ABSTRACT
Introduction
Pseudoviruses are recombinant, replication-incompetent, viral particles designed to mimic the surface characteristics of native enveloped viruses. They are a safer, and cost-effective research alternative to live viruses. With the potential emergence of the next major infectious disease, more vaccine scientists must become familiar with the pseudovirus platform as a vaccine development tool to mitigate future outbreaks.
Areas Covered
This review aims at vaccine developers to provide a basic understanding of pseudoviruses, list their production methods, and discuss their utility to assess vaccine efficacy against enveloped viral pathogens. We further illustrate their usefulness as wet-lab simulators for emerging mutant variants, and new viruses to help prepare for current and future viral outbreaks, minimizing the need for gain-of-function experiments with highly infectious or lethal enveloped viruses.
Expert opinion
With this platform, researchers can better understand the role of virus–receptor interactions and entry in infections, prepare for dangerous mutations, and develop effective vaccines.
1. Introduction
Most zoonotic viruses that cause human diseases, such as Ebola virus, Marburg virus, Nipah virus, Chikungunya virus, smallpox virus, monkeypox virus, coronaviruses, rabies virus, and influenza viruses are enveloped viruses [Citation1]. Cultivation of the live (wild type) pathogenic viruses can be dangerous, and gaining access to these virus strains is often a complex and time-consuming process, demanding Bio Safety Level 3 (BSL-3) or Biosafety Level 4 (BSL-4) laboratories. These limitations further hinder the number of laboratories that study pathogenic viruses, thus impeding research progress toward antivirals and vaccines. Pseudotypes of these enveloped viral pathogens act as valuable surrogates for studying their structural and functional interactions with host organisms and offer an excellent platform for testing vaccine efficacy [Citation2].
A pseudovirus, defined as ‘a defective nonpathogenic enveloped virus that displays a heterologous viral envelope protein of interest,’ can enter a cell, and mimic the initial stages of infection, but cannot complete a full replication cycle to produce new infectious particles. To make them replication-defective and to facilitate the incorporation of the heterologous viral envelope protein, the native virus envelope gene is deleted, and other genes involved in pathogenicity are also removed. What remains are the structural and enzymatic proteins and genomic elements required for virion assembly, host cell entry, and gene expression. Pseudoviruses can be produced at large scale in vitro, by co-transfecting producer cells with plasmids expressing the envelope protein from the viral pathogen of interest, viral packaging plasmids to express structural proteins, and a defective viral genome that often includes a reporter gene for ease of quantifying infection. The incorporation of the heterologous viral envelope protein allows the pseudovirus to utilize the same pathway that its native pathogenic virus uses to enter cells [Citation2], and through selective expression of envelop proteins, these pseudoviruses can facilitate systematic studies on the mechanisms of viral entry into host cells and allow research into entry inhibitors as antivirals.
The pseudovirus platform can serve as a well-controlled model to evaluate the virus-neutralization efficacy of antibodies, making it a valuable tool for the preclinical phase of vaccine development [Citation3]. Pseudoviruses can be prepared using a wide range of enveloped viral genomes as a packaging backbone, allowing researchers to tailor their design to specific viral targets of interest [Citation4]. Also, they can express many viral proteins, including those from different viruses or variants of the same virus, making them a versatile platform for studying how viral proteins interact with host cells [Citation5]. Thus, pseudoviruses help model the interactions between a virus and its cellular receptor(s) [Citation6]. They can be concentrated to high titers and employed in place of wild-type viruses that are difficult to culture. They can also be engineered to possess a broadened host range, which helps to study cellular, tissue, or organ tropism [Citation7]. However, while pseudotyping a virus is essential to keep the protein in its most representative state to the live virus for its use in neutralization assays. This can be achieved by using a vector platform that is structurally similar to native virus. Though pseudovirus envelope proteins can largely mediate viral entry in a fashion like that of the wildtype virus, they can only replicate for one round and may not always induce pathogenesis as their wild-type counterparts do. Therefore, results from assays using pseudotyped viruses should be compared and validated against the live virus‐based assay, which remains the golden standard.
Over the past two decades, several pseudovirus systems have been tested, encompassing a wide range of zoonotic viruses. Examples can be found for viruses belonging to multiple viral families (), including Arenaviridae (e.g. Lassa virus [Citation8]), Coronaviridae (e.g. Severe Acute Respiratory Syndrome Coronavirus SARS-CoV-1 [Citation20], and SARS-CoV-2 [Citation23]), Filoviridae (e.g. Ebola [Citation6] and Marburg virus [Citation2]), Flaviviridae (e.g. Dengue virus [Citation1], and Zika virus), Hantaviridae (e.g. Hantavirus [Citation40]), Hepeviridae (e.g. Hepatitis E virus [Citation42]), Nairoviridae (e.g. Crime-Congo Hemorrhagic Fever Virus [Citation44]), Orthomyxoviridae (e.g. Influenza A virus [Citation5]), Paramyxoviridae (e.g. Nipah virus [Citation35]), Peribunyaviridae (e.g. La Crosse Encephalitis virus [Citation52] and Rift Valley fever virus [Citation53]), Pneumoviridae (human Respiratory Syncytial Virus RSV [Citation66]), Retroviridae (e.g. Human Immunodeficiency Virus [Citation67]), Rhabdoviridae (e.g Rabies virus [Citation60] and Togaviridae (e.g. Chikungunya virus [Citation61] and Ross River virus [Citation65]). These pseudoviruses have been used for studying viral entry, and in some cases, they have been applied to evaluate antibody-mediated neutralization. Notably, for the many viruses that still lack effective vaccines, pseudovirus platforms can be employed to accelerate further research.
Table 1. List of viral zoonoses for which envelope pseudovirus systems have been established.
In addition, pseudoviruses can be valuable for studying mutations in the envelope gene of an emerging virus that enhances infectivity, transmission, or disease. Once the mutated envelope sequence of a pathogenic virus is identified, a DNA plasmid expressing the viral envelope protein can be synthesized, facilitating the rapid development of pseudoviruses. This makes it easier and faster for research groups worldwide to participate in viral research without having to invest in expensive laboratory infrastructure. Hence, pseudovirus platforms have the potential to significantly accelerate research efforts during viral outbreaks and expedite the development of effective countermeasures such as antivirals and vaccines.
2. Pseudovirus production and development
Transient transfection is the most common method for producing pseudoviruses. Several types of pseudovirus systems have been established using different pseudovirus packaging systems, transduction reporters and production cell lines. As can be learned from the abundance of literature on pseudovirus systems for SARS-CoV-2, different systems may be applicable to study the same virus and the researcher’s choice most often depends on previous experiences, and the availability of resources [Citation2]. However, it is important to understand the characteristics and limitations of each system of choice and optimize the pseudovirus production parameters conducive to the target pathogen envelope protein.
2.1. Pseudovirus packaging systems
Pseudoviruses can be categorized based on the source of their core packaging genome. Among the most frequently employed envelope pseudotyping platforms are Vesicular Stomatitis Virus (VSV), Human Immunodeficiency Virus-1 (HIV-1) and Murine Leukemia Virus (MLV). Additional examples encompass Simian Immunodeficiency Virus (SIV), Feline Immunodeficiency Virus (FIV), and West Nile Virus (WNV). The construction of these viral platforms has been effectively achieved through reverse genetic systems, and a detailed molecular understanding of the regulation of the viral replication cycles.
VSV is an enveloped RNA virus that has been applied for multiple reverse genetics approaches established for negative-strand RNA viruses. With its non-segmented genome of approximately 11 kb, VSV infects a wide range of mammalian cell types and yields high viral quantities within a short period, thus making it a valuable viral vector for research applications. Notably, VSV has shown promise as a vaccine platform, exemplified by the development of the first Ebola vaccine using VSV [Citation68]. Through plasmid-based methods, recombinant VSV-based pseudoviruses with substitutions of envelope glycoproteins from other viruses can be generated, enabling the creation of vaccine candidates targeting various human pathogens [Citation64].
MLV is a positive-strand retrovirus that causes cancers in mice. The molecular dissection of defective MLV genomes harboring oncogenes that can be packaged by a helper virus has been instructive for the development of retroviral and lentiviral vectors for gene therapy [Citation69]. MLV-based heterologous envelope protein pseudoviruses are produced similarly to HIV-based vector pseudoviruses, where MLV structural genes gag and pol, along with a gene encoding a heterologous virus envelope protein (env), and a transfer vector with transgene are transfected into cells [Citation2]. Following virion assembly and incorporation of the heterologous viral envelope protein and packaging of the reporter vector, the cells secrete pseudovirus particles carrying the heterologous virus envelope protein into the cell culture medium.
Lentiviral vectors derived from HIV-1 are an increasingly popular model for developing pseudo and chimeric viruses due to their ease of use, stability, and long-term expression of transgenes. Their ability to transduce cells independent of their division status makes lentiviral vectors ideal for gene therapy of highly differentiated, postmitotic cells. The HIV-1 packaging system typically involves dividing the HIV-1 backbone into separate packaging and gene transfer vector plasmids. These plasmids can then be co-expressed with a viral envelope expression plasmid to generate infectious pseudovirions. The packaging plasmid expresses Gag structural and Pol enzymatic proteins for virion assembly, reverse transcription of viral RNA into double stranded DNA and integration into host cell DNA, while the transfer plasmid contains the minimal viral cis-elements required to package a RNA with transgene cassette controlled by a heterologous promoter that can be subsequently reverse transcribed into DNA, integrated, and expressed in target cells [Citation2].
Other lentiviral platforms have also been utilized to generate Enveloped pseudovirions. SIV, based on the viral genome of SIVmac251, has been utilized as a packaging system for pseudoviruses to study coronaviruses [Citation70]. SIV-based pseudoviruses have shown high transduction efficiency in primary blood lymphocytes and CD34+ cells derived from humans or macaques [Citation71]. Feline Immunodeficiency Virus (FIV) has successfully been used for studying EBOV, SARS-CoV-1, Vesicular stomatitis virus, Rabies Virus, Ross River virus, West Nile Virus, and Dengue virus [Citation72], producing pseudoviruses with high titers [Citation27].
From a biosafety perspective, all pseudovirus vectors are either based on the genome of viruses with low biological risk (e.g. MLV and VSV) or in the case of moderate-risk viruses like HIV, they are extensively modified to reduce the chance of recombination occurring to reform replication-competent virus. Separate plasmid vectors with heterogeneous promoters are used to co-express the envelope protein of the high-risk virus to be pseudotyped with the vectors [Citation73]. To enhance the safety of the packaging systems, non-essential genes are deleted from the core genome, and the components necessary for virus production can be split across multiple plasmids, as is the case with the 2nd and 3rd-generation lentiviral vector packaging systems. This separation of transfer, envelope, and packaging components of the virus onto different vectors () eliminates the potential for the generation of replication-competent viruses. Also, in the case of lentiviral and retroviral vectors, many of these vectors are self-inactivating vectors with a 3’ LTR U3 deletion that abolishes transcription from the 5’ LTR after the vector genome has reverse-transcribed and integrated into a host cell’s genome [Citation73].
Figure 1. Schematic representation of production of the lentivirus-based pseudovirus particles (example: HIV, MLV, SIV, FIV). After co-transfection of producer cells (example: HEK293T) using different plasmids, viral core particles with reporter gene are formed. Simultaneously, envelope spikes (red rod like structures) are first translated inside the cell before being translocated to the membrane, where they are integrated with the core particles and are secreted into the supernatant as budding pseudoviruses.
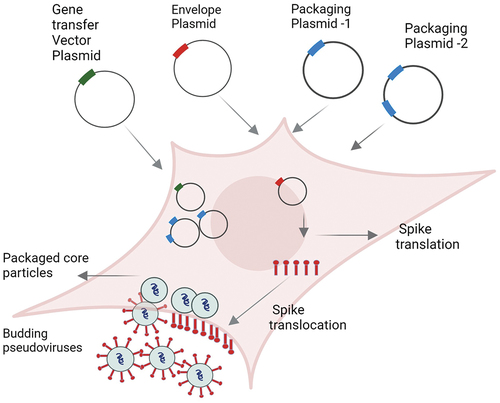
2.2. Reporter genes
The addition of reporter gene makes pseudoviruses a more sensitive platform for rapid quantitative analyses compared to wild-type viruses. The choice of the reporter gene depends on the experimental goal, equipment available, and the desired output, as each reporter () has its strengths and limitations. Most common reporter genes used are luminescence-based like luciferase, or florescence-based like Green Florescent Protein. Luminescence reporter assays have lower background noise and higher sensitivity but require laborious and expensive data acquisition and analysis. On the other hand, assays using fluorescent proteins are cheaper and easier but may have higher background noise and lower sensitivity. Additional fluorescent reporters with different emission spectra, such as mCherry (red), YFP (yellow) or CFP (cyan), offer multiplexing options. Colorimetry-based reporters, such as β-galactosidase (LacZ) and Secreted Alkaline Phosphatase (SEAP) are advantageous for researchers to repeatedly examine the same samples to detect any changes that might occur over a period of time (longitudinal studies) as they do not require cell lysis and can be used to monitor transduction over time using the same cell populations. Sensitive, high-throughput neutralization assays using pseudoviruses carrying a SEAP reporter gene were developed and validated for human papillomavirus HPV16, HPV18, and bovine papillomavirus-1 (BPV1) [Citation84].
Table 2. List of established reporter genes to characterize pseudovirus systems.
2.3. Production cell lines
When producing pseudoviruses, the selection of a suitable production cell line is crucial to ensure efficient transfection and elevated levels of viral protein expression. The choice of the production cell line may depend on the specific type of pseudovirus being produced and as well its intended downstream application ().
Table 3. Common production cell lines used for transient transfection and pseudovirus generation.
The most prominent choice for pseudovirus production involves the utilization of human embryonic kidney 293 cells, which have been genetically modified to express the SV40 T antigen (HEK293T). HEK293T cells demonstrate competence in replicating vectors containing the SV40 replication region, resulting in consistently high titers during lentivirus production [Citation85]. Optimized cell lines from HEK293T include HEK293FT, recommended for its faster growing and high transfection efficiency [Citation86]. HEK 293GP, another specialized cell line derived from HEK293 cells, expresses the Gag and Pol proteins of the Moloney Murine Leukemia Virus [Citation87], and is most suited for producing retroviral vectors. For a large-scale pseudovirus production, Expi293F cells are a popular option. These cells are derived from the HEK293F cell line, and grow in suspension culture [Citation88]. In specific cases, other cell lines may be preferred. For example, Huh-7, KHK-21, Vero E6, Calu-3, CHO, MDCK, or HepG2 cell lines have been described in literature () [Citation89,Citation90].
Additionally, some groups have established knockout cell lines that were very helpful to optimize the titers of pseudoviruses [Citation91].
2.4. Pseudovirus characterization and stability
Different techniques have been described to characterize pseudoviruses to assess their suitability for further downstream applications. The most basic characterization is to titrate the virus by serial dilution and measure its infectivity. This does not equal the absolute number of viral particles, because, in practice, not all virions will successfully cause infection. Aside from the number of infectious particles, the infection rate is dependent on experimental conditions such as the temperature or acidity of the medium and the host cell type. Traditionally, the virus titration is expressed by the 50% Tissue Culture Infectious Dose assay (TCID50 assay) [Citation77,Citation92] which determines the dilution factor of the virus stock at which 50% of the infected host cells display cytopathic effects. When using pseudoviruses and the pseudo-infection does not cause cell death, then a reporter gene is used to score the transduction (infection) efficiency. To determine the TCID50, serial dilutions of a pseudovirus are added onto monolayers of cells and incubated until they can be scored for infection using reporter readout values. The amount of read out signal acquired is directly proportional to the number of infected cells. These values at various serial dilutions can then be plotted in a continuous non-linear regression dose–response curve, which will enable to extrapolate accurate TCID50 values. A rough estimate of the number of infectious viral particles can be made by multiplying the TCID50 number by 0.7 [Citation93]. This estimate is based on the Poisson distribution applied to viral infection which states that, in a fully permissive cell line, the probability of reaching 50% infection is achieved by a multiplicity of infection (MOI) of approximately 0.7 [Citation94,Citation95]. In addition to electron microscopy for visual characterization of the morphology of pseudovirus particles, Nano particle Tracking Analysis (NTA) [Citation96–98] can be used to measure the concentration and size distribution of pseudoviruses in liquid suspension. Indirect methods like Quantitative PCR (viral DNA) or reverse-transcription quantitative PCR (for viral RNA) offer a way to measure the amount of viral DNA or RNA in target cells leading to indirect quantification of the pseudoviruses [Citation75]. Methods to quantify the amount of envelope protein incorporated into the viral particles include flow cytometry, immuno-fluorescence, and immune-blot analysis where envelope protein antibodies are employed to study the transduction efficiency of pseudoviruses.
Aliquots of pseudoviruses can be stored in −80°C freezers and remain stable for many months. Limited lyophilization experiments with lentiviral-based pseudoviruses have recently been reported [Citation99], which may offer better storage and shipping considerations allowing for wider deployment potential.
3. Applications of pseudoviruses for vaccine development
3.1. Systematic study of the mechanisms of viral entry, entry inhibitors and infection
Pseudovirus systems offer versatile applications in both fundamental and applied virology. By utilizing the same cell entry mechanism as the actual pathogen, pseudoviruses are valuable functional tools for investigating virus-host cell receptor binding and entry [Citation76] and cell tropism [Citation6,Citation23]. For example, Lassa Virus glycoprotein complex (LASV-GPC) pseudoviruses co-labeled with viral content marker and an internal pH sensor enabled detection of single virus fusion events and allowed in-depth study of viral entry dependence on low pH while infecting human and avian host cells [Citation100]. By leveraging pseudoviruses in systematic studies of viral infection mechanisms, researchers can accelerate the discovery and selection of new vaccine antigens.
3.2. Antibody-based virus neutralization
For many viruses, neutralizing antibodies, whether acquired through natural infection or vaccination, can effectively inhibit viral pathogen infectivity in host cells. Using isolated live virus cultures, neutralizing antibodies can be measured through in vitro assays such as PRNT (Plaque Reduction Neutralization Test), FRNT (Focus Reduction Neutralization Test), and VRNT (Virus Reduction Neutralization Test). Despite their effectiveness, the use of replication-competent viruses in these assays poses significant health hazards and requires the use of high-level containment. Studies with influenza virus [Citation101], Ebola virus, Marburg virus [Citation102], SARS-CoV-2 [Citation103,Citation104] and for other viruses as reviewed [Citation105], have shown that neutralizing titers of sera tested against pseudoviruses are highly correlated with results obtained when using live viruses. However, choice of pseudo-viral vector influences the neutralization capacity for some pathogens, as in the case of Ebola virus where comparative studies between HIV-1 and VSV-based pseudovirus platforms to measure the neutralizing ability revealed better alignment of VSV-based pseudovirus results with live virus neutralization data [Citation68]. Therefore, if new pseudotypes are to be generated, they must be characterized and compared to a live virus assay, to fully verify the pseudovirus vector system suitability for the specific virus [Citation106]. Thus, use of pseudoviruses has emerged as a safer alternative for evaluating and quantifying virus neutralizing antibody titers.
To measure neutralizing antibodies, serum samples from patients or test animals are serially diluted to different concentrations, mixed with pseudoviruses, and incubated, allowing the antibodies in the serum to interact with the pseudoviruses. Cells expressing the receptor for the pseudovirus are added to the mixture and incubated further. The level of cell infection is measured, often by quantifying a reporter signal produced by the pseudovirus upon successful entry, and expression of the reporter gene. By comparing the infection levels in the presence of serum samples to control samples without antibodies, the reduction in infection indicates the neutralizing capacity of the antibodies. In vitro vaccine efficacy studies have been successfully conducted based on the pseudotyped H7N9 virus [Citation46], Rift Valley fever virus [Citation53], Nipah virus [Citation50], and rabies virus [Citation107]. The Human Papilloma Virus (HPV) pseudovirus neutralization assays were helpful to study the immunogenicity of HPV vaccines (CervarixTM and Gardasil®) [Citation108,Citation109].
3.3. The role of pseudoviruses during COVID and preparing for the next viral outbreak
Coronaviruses (CoV) present a significant threat to human health, as experienced by the three highly pathogenic and lethal CoVs, namely SARS-CoV-1, MERS-CoV and SARS-CoV-2. While SARS and MERS were contained early through stringent public health measures and research efforts, SARS-CoV-2, responsible for COVID-19, led to the first worldwide pandemic of the 21st century. The ongoing risk of new coronavirus strains emerging underscores the importance of preparedness for virologists and vaccine developers. Multiple pseudovirus systems for CoV have been developed and employed to advance our understanding of these viruses, significantly contributing to studies pertaining to the recognition of host cell receptors, viral binding, and entry mechanisms. These virus models have further been exploited by vaccine developers screening for cross-neutralizing antibodies in the sera of vaccinated test subjects. These pseudovirus systems have been adapted using various platforms, including VSV, HIV, and MLV-based vectors, to mimic receptor binding and entry of CoVs. To improve global collaboration and standardization in the study of coronaviruses, international serum standards were generated. These standards, distributed by National Institute for Biological Standards and Control (NIBSC) and the World Health Organization (WHO), played a crucial role in harmonizing methodologies and facilitating a unified understanding of correlates of protection, ensuring that research efforts and findings related to pseudovirus systems and vaccine development align with internationally recognized benchmarks.
To facilitate the study of SARS-CoV-1 host range and entry mechanisms, Nie et al. [Citation22] created highly infectious SARS-CoV-1 pseudotyped viruses by incorporating the humanized SARS-CoV-1 spike glycoprotein into HIV particles. These pseudotypes were effectively neutralized by sera from convalescent SARS-CoV-1 patients, revealed pH-dependent entry, and demonstrated susceptibility in various tissue-derived cell lines, aligning with the expression pattern of the virus receptor, angiotensin-converting enzyme 2 (ACE2). Fujioka et al. [Citation20] introduced a modified protocol for producing high-yield VSV pseudotyped with SARS-CoV-1 spike proteins. They achieved efficient production of pseudoviruses by establishing stable cell lines with optimal spike protein expression levels, which is expected to facilitate research on coronavirus entry and the development of antiviral agents.
Pseudoviruses expressing the spike protein of the MERS-CoV coronavirus have been optimized [Citation15] and used to elucidate viral entry mechanisms and evaluating vaccine candidates [Citation17,Citation110]. MERS-CoV shares a close phylogenetic relationship with bat coronaviruses HKU4 and HKU5. MERS-CoV’s viral spike protein contains an RBD that interacts with human CD26 for viral entry. Using pseudovirus models, Wang et al. [Citation16] discovered that while HKU4-RBD binds to hCD26, HKU5-RBD does not, and pseudotyped viruses with the HKU4 spike can infect cells through hCD26 recognition, though with lower affinity compared to MERS-RBD, indicating a potential bat origin for MERS-CoV.
During the COVID-19 pandemic, pseudoviruses have been used to investigate SARS-CoV-2’s recognition of host cell receptors and entry mechanisms, aiding in the rapid development, and testing of vaccines. They played a pivotal role in screening for cross-neutralizing antibodies [Citation111], evaluating monoclonal antibodies, and assessing vaccine titers, particularly in the context of emerging and mutated variants of the virus [Citation112,Citation113]. Among them, Crawford et al. [Citation114] showed in detail, the process of packaging the SARS-CoV-2 pseudoviruses with HIV, while Nie et al. and Al-Mahbub et al. chose to package the SARS-CoV-2 pseudoviruses with VSV [Citation92,Citation115]. SARS-CoV-2 spike was also pseudotyped successfully with an MLV-based vector system [Citation116]. Johnson et al. [Citation117] identified HIV and VSV systems to be compatible for creating surrogate pseudoviruses of SARS-CoV-2. Additionally, natural mutations at certain spike positions have been found to determine tropism of coronaviruses and their transmission between humans and animals [Citation118]. Mutations in these positions can therefore have implications for cross-species and/or intra-species transmission of SARS-CoVs.
Spike pseudovirus systems allow for genetic tractability [Citation2]. Expression libraries of SARS-CoV-2 spike mutants can be incorporated into pseudovirions to neutralize escape mutations and variant spike genes can be rapidly engineered to investigate alterations in tropism. Unlike real viruses, which undergo changes with each interaction with a new host beyond our control, pseudoviruses can be manipulated and controlled by utilizing single copy clones of the gene of interest. This characteristic enabled for a greater control over variations and offered a faster and scalable model for generating and screening new variants of SARS-CoV-2 [Citation119]. One of the significant advantages of pseudoviruses is their ease of manipulation in vitro, enabling the prediction, production, and screening of emerging variants in response to specific host cell types or culture conditions. For instance, in the case of Coronaviruses, pseudoviruses have provided a faster model for studying new mutations in the spike protein [Citation120]. In this study, a diverse array of spike protein variants, including region-specific mutants, was designed using pseudoviruses in combination with a broader set of 12 ACE2 receptors sourced from various primate and other animal origins. By utilizing these engineered spike-ACE2 combinations for neutralizing antibody assays, valuable information was obtained, such as the extent of immune evasion, if any, in previously vaccinated or convalescent individuals, as well as prospective inter-genus transmission (heterologous ACE2 receptor binding) resulting from these mutations. Similarly, for any other pathogenic virus, it is possible to conduct similar studies on emerging variants and their resistance to neutralization by current vaccines by engineering variant envelope versions in vitro.
Preparing for the next pandemic and addressing the potential threats posed by emergent infectious diseases are crucial. Coronavirus diseases, known for their rapid evolution and cross-species transmission, are expected to remain highly prevalent, followed by influenza and Ebola. The persistence of coronaviruses in humans, as seen with 229E, OC43, NL63, and HKU1 [Citation121], MERS, and SARS-related CoVs and the zoonotic disease transmission potential of Alphacoronavirus 1 and other examples of people being infected with novel canine CoV [Citation122] emphasize the need for vigilance [Citation123]. Genetic recombination events among existing subtypes of influenza viruses can lead to future pandemics, while the high fatality rates and human-to-human transmission of Ebola virus pose significant risks.
To effectively prepare for emerging pathogens and pandemics, it is essential to focus on tools such as immunogenicity evaluation assays, clinical methods, and experimental models/platforms to aid in vaccine development. Pseudoviruses, with their safety and inability to undergo multiple rounds of replication like wild type viruses, offer a viable alternative way to gain valuable insights. Pseudovirus platforms have been established for coronaviruses, influenza viruses, Ebola viruses, and other emerging viruses, and played a vital role in evaluating vaccines and constructing mutational libraries (to the tune of 10,000 mutations per preparation) of entry proteins for high-risk pathogens. They enable the identification of virulent strain escape mutants and the evaluation of vaccine-induced neutralizing antibodies. In 2023, Dadonite et al. [Citation23] reported how a pseudovirus system has enabled deep mutational scanning of the complete SARS-CoV-2 spike. They used a lentiviral pseudovirus platform to produce libraries of Omicron BA.1 and Delta spikes and map the escape mutations against those libraries and how they evade neutralization. Similar pseudovirus platforms can be applied to the entry proteins of any other viruses. Pseudoviruses have also found utility in the generation of machine learning models for Antibody Mediated Prevention Trials (AMP), as demonstrated by studies predicting the sensitivity of HIV-1 pseudoviruses to neutralizing antibodies [Citation124].
4. Conclusion
Pseudoviruses have emerged as invaluable tools in the arena of vaccine development for enveloped viruses. Their flexibility in accommodating various envelope proteins allows for extensive applications, from understanding the biology of known viral pathogens to the early characterization of emergent, potentially zoonotic viruses. Their usage extends from preclinical evaluation of vaccine candidates, assessing the neutralizing capacity of vaccine-induced antibodies, to monitoring vaccine efficacy against evolving viral variants and has expedited the development of crucial countermeasures against important disease like COVID-19 [Citation125,Citation126]. Their non-replicating nature eliminates the need for BSL3 or BSL4 laboratories, reducing the cost and need for special equipment [Citation13,Citation127]. Pseudoviruses continue to play significant role in the development of vaccines for viruses that are difficult to vaccinate against, such as HIV and HCV. Safety and ease of pseudovirus platform makes vaccine research more accessible to scientists around the world, accelerating the pace of discoveries spanning across various fields of virology and vaccine development. However, it is important to note that pseudovirus systems are not always a good surrogate system for live viruses [Citation106]. For example, due to viral morphology differences, pseudotyped Filoviruses made in lentiviral systems do not correlate well with their live virus counterpart. Better mimics of Filoviruses can be made with a VSV-type pseudovirus [Citation68]. Therefore, if new pseudotypes are to be generated, they must be characterized and compared to a live virus assay, to fully verify whether the pseudovirus system can be used as a surrogate platform.
5. Expert opinion
Considering all the zoonotic viruses that could lead to diseases, attempts to develop corresponding pseudovirus systems for some of these pathogens are sparse. All the latest technological advancements that were utilized for quick mitigation of the SARS-CoV-2 virus during the COVID-19 pandemic can be successfully applied to emerging enveloped viral pathogens as well.
As technical capabilities in cell biology, genetic engineering and single cell sequencing improve, scientists might be able to create more complex pseudoviruses for a broader range of viral diseases with multiple viral proteins, that enable studies of co-infection patterns. This may help to produce broad spectrum/multivalent/universal vaccines for different strains of a pathogen, or for multiple pathogens. A pseudovirus platform is valuable for testing and validating the next generation vaccine models for epitope mapping for immunogenicity before they can go to in vivo testing or clinical trials. Another speculative but potential advancement in the future of pseudoviruses could be their contribution to personalized vaccines, incorporating tailored, patient-specific antigens into pseudoviruses to induce unique immune responses.
Pseudoviruses are commonly preserved in −80°C freezers, where they can maintain stability for extended periods, typically months. Recent experiments involving lyophilization of lentiviral-based pseudoviruses have shown promise [Citation99], potentially improving storage and shipping coordination for broader deployment. The establishment of a thermostable lyophilization system for pseudoviruses could facilitate cost-effective access and rapid screening of vaccine efficacies for future pandemics, enabling quick assessment of region-specific emerging pathogen serotypes and mutants during outbreaks.
Despite their merit in various applications, pseudoviruses do have certain limitations. They cannot be employed to study immune escape mechanisms or the effects of therapeutics that target non-envelope protein genes. Moreover, the density of envelope proteins or the structure of pseudovirus may not precisely mimic that of live viruses for certain pathogens. Though such potential discrepancies exist in the action of pseudoviruses compared to their authentic counterparts, advancements in pseudovirus production techniques leading to improved pseudoviral titers and increased transduction efficiencies increase the robustness of neutralization assays, thereby extending their utility. Progress toward automated sample handling and better readout techniques can increase the speed and efficiency of these assays. Improving pseudoviruses to better screen for mutant variants involves optimizing the processes of design, cloning, and validation of new pseudoviruses. Integrating pseudovirus studies with bioinformatics can improve our ability to predict and sort the effect of mutations on viral function, helping to focus screening efforts on the most important/high priority variants. Developing standardized protocols and superior quality control measures can improve the reliability and reproducibility of results, making it easier to compare results between different variants and different labs. By sharing data with other researchers around the globe, we can rapidly gather information on a wide range of variants, increasing our collective ability to respond to new threats.
As discussed in this review, scientists already have enlisted pseudovirus platform as a successful validation model for in-silico-generated epitope modeling. Moving forward, pseudovirus platforms may seamlessly integrate with emerging technologies such as artificial intelligence to predict vaccine efficacy; machine learning and gene editing to optimize pseudovirus design; and microfluidics, nanotechnology and computational modeling for high-throughput and rapid testing. Efforts to standardize the methods for production and storage of pseudoviruses, and development of clear regulatory guidelines for their utilization would ensure the reliability and comparability of results across different studies, labs, and geographical regions and thus, could facilitate the wider adoption of this platform in both academic and industrial settings.
As the field of vaccine development rapidly progresses, various cutting-edge technologies are being employed, such as viral vector vaccines, mRNA vaccines, nano-particle vaccines, and peptide-based vaccines. By leveraging these innovative techniques, scientists and researchers are accelerating the discovery and refinement of vaccines against various diseases. Pseudovirus platforms hold great promise as they offer a safe and efficient means to thorough optimize and evaluate these next generation vaccine candidates.
Article highlights
Replication incompetent pseudoviruses have been established for many enveloped viruses.
Pseudoviruses allow for safer research on virus entry, cell tropism, and neutralizing antibodies.
Pseudoviruses can be used to screen for anti-viral solutions before major outbreaks happen.
Declaration of interest
The authors have no relevant affiliations or financial involvement with any organization or entity with a financial interest in or financial conflict with the subject matter or material discussed in the manuscript. This includes employment, consultancies, honoraria, stock ownership or options, expert testimony, grants or patents received or mending, or royalties.
Reviewer disclosures
Reviewers on this manuscript have received honoraria for their review work. Peer reviewers on this manuscript have no relevant financial or other relationships to disclose.
Acknowledgments
The authors express sincere gratitude to Dr. Ulrich Strych for proof reading the manuscript and providing valuable suggestions.
Additional information
Funding
References
- Valero-Rello A, Sanjuán R. Enveloped viruses show increased propensity to cross-species transmission and zoonosis. Proc Natl Acad Sci. 2022;119(50):e2215600119. doi: 10.1073/pnas.2215600119
- Xiang Q; Li L; Wu J; Tian M; Fu Y. Application of pseudovirus system in the development of vaccine, Antiviral-Drugs, and neutralizing antibodies. Microbiol Res. 2022;258:126993. doi: 10.1016/j.micres.2022.126993
- Kalkeri R, Cai Z, Lin S, et al. SARS-CoV-2 Spike Pseudoviruses: a useful tool to study virus entry and address emerging neutralization escape phenotypes. Microorganisms. 2021;9(8):1744. doi: 10.3390/microorganisms9081744
- Chen M, Zhang X-E. Construction and applications of SARS-CoV-2 pseudoviruses: a mini review. Int J Biol Sci. 2021;17(6):1574–1580. doi: 10.7150/ijbs.59184
- Cheresiz SV, Kononova AA, Razumova Y, et al. A Vesicular Stomatitis Pseudovirus Expressing the Surface Glycoproteins of Influenza A Virus. Arch Virol. 2014;159(10):2651–2658. doi: 10.1007/s00705-014-2127-y
- Wool-Lewis RJ, Bates P. Characterization of ebola virus entry by using pseudotyped viruses: identification of receptor-deficient cell lines. J Virol. 1998;72(4):3155–3160. doi: 10.1128/JVI.72.4.3155-3160.1998
- Najafi Fard S, Petrone L, Petruccioli E, et al. In vitro models for studying entry, tissue tropism, and therapeutic approaches of highly pathogenic coronaviruses. Biomed Res Int. 2021;2021:e8856018. doi: 10.1155/2021/8856018
- Cosset F-L, Marianneau P, Verney G, et al. Characterization of lassa virus cell entry and neutralization with lassa virus pseudoparticles. J Virol. 2009;83(7):3228–3237. doi: 10.1128/JVI.01711-08
- Kunz S, Rojek JM, Perez M, et al. Characterization of the interaction of lassa fever virus with its cellular receptor α-dystroglycan. J Virol. 2005;79(10):5979–5987. doi: 10.1128/JVI.79.10.5979-5987.2005
- Robinson JE, Hastie KM, Cross RW, et al. Most neutralizing human monoclonal antibodies target novel epitopes requiring both lassa virus glycoprotein subunits. Nat Commun. 2016;7(1):11544. doi: https://doi.org/10.1038/ncomms11544
- Klewitz C, Klenk H-D, Ter Meulen J. Amino acids from both N-Terminal hydrophobic regions of the lassa virus envelope glycoprotein GP-2 are critical for pH-Dependent membrane fusion and infectivity. J Gen Virol. 2007;88(Pt 8):2320–2328. doi: 10.1099/vir.0.82950-0
- Radoshitzky SR; Abraham J; Spiropoulou CF; Kuhn JH; Nguyen D; Li W; Nagel J; Schmidt PJ; Nunberg JH; Andrews NC; Farzan M; Choe H. Transferrin Receptor 1 Is a Cellular Receptor for New World Haemorrhagic Fever Arenaviruses. Nature. 2007;446(7131):92–96. doi: 10.1038/nature05539
- Welch SR; Guerrero LW; Chakrabarti AK; McMullan LK; Flint M; Bluemling GR; Painter GR; Nichol ST; Spiropoulou CF; Albariño CG. Lassa and ebola virus inhibitors identified using minigenome and recombinant virus reporter systems. Antiviral Res. 2016;136:9–18. doi: 10.1016/j.antiviral.2016.10.007
- Steffen I, Simmons G. Pseudotyping viral vectors with emerging Virus envelope proteins. Curr Gene Ther. 2016;16(1):47–55. doi: 10.2174/1566523216666160119093948
- Grehan K, Ferrara F, Temperton N. An optimised method for the production of MERS-CoV spike expressing viral pseudotypes. MethodsX. 2015;2:379–384. doi: 10.1016/j.mex.2015.09.003
- Wang Q, Qi J, Yuan Y, et al. Bat origins of MERS-CoV supported by bat coronavirus HKU4 usage of human receptor CD26. Cell Host Microbe. 2014;16(3):328–337. doi: 10.1016/j.chom.2014.08.009
- Zhao G, Du L, Ma C, et al. A safe and convenient pseudovirus-based inhibition assay to detect neutralizing antibodies and screen for viral entry inhibitors against the novel human coronavirus MERS-CoV. Virol J. 2013;10:266. doi: 10.1186/1743-422X-10-266
- Yang Y, Du L, Liu C, et al. Receptor usage and cell entry of bat coronavirus HKU4 provide insight into bat-to-human transmission of MERS coronavirus. Proc Natl Acad Sci U S A. 2014;111(34):12516–12521. doi: 10.1073/pnas.1405889111
- Du L, Zhao G, Yang Y, et al. A conformation-dependent neutralizing monoclonal antibody specifically targeting receptor-binding domain in middle east respiratory syndrome coronavirus spike protein. J Virol. 2014;88(12):7045–7053. doi: 10.1128/JVI.00433-14
- Fujioka Y, Kashiwagi S, Yoshida A, et al. A method for the generation of pseudovirus particles bearing SARS coronavirus spike protein in high yields. Cell Struct Funct. 2022;47(1):43–53. doi: 10.1247/csf.21047
- He Y, Zhu Q, Liu S, et al. Identification of a critical neutralization determinant of severe acute respiratory syndrome (SARS)-associated coronavirus: importance for designing SARS vaccines. Virology. 2005;334(1):74–82. doi: 10.1016/j.virol.2005.01.034
- Nie Y, Wang P, Shi X, et al. Highly infectious SARS-CoV pseudotyped virus reveals the cell tropism and its correlation with receptor expression. Biochem Biophys Res Commun. 2004;321(4):994–1000. doi: 10.1016/j.bbrc.2004.07.060
- Dadonaite B, Crawford KHD, Radford CE, et al. A pseudovirus system enables deep mutational scanning of the full SARS-CoV-2 Spike. Cell. 2023;186(6):1263–1278.e20. doi: 10.1016/j.cell.2023.02.001
- Donofrio G; Franceschi V; Macchi F; Russo L; Rocci A; Marchica V; Costa F; Giuliani N; Ferrari C; Missale G. A Simplified SARS-CoV-2 pseudovirus neutralization assay. Vaccines. 2021;9(4):389. doi: 10.3390/vaccines9040389
- Yang P; Yang Y; Wu Y; Huang C; Ding Y; Wang X; Wang S. An optimized and robust SARS-CoV-2 pseudovirus system for viral entry research. J Virol Methods. 2021;295:114221. doi: 10.1016/j.jviromet.2021.114221
- Fu X; Tao L; Zhang X. Comprehensive and systemic optimization for improving the yield of SARS-CoV-2 spike pseudotyped virus. Mol Ther - Methods Clin Dev. 2021;20:350–356. doi: 10.1016/j.omtm.2020.12.007
- Kobinger GP; Weiner DJ; Yu QC; Wilson JM. Filovirus-pseudotyped lentiviral vector can efficiently and stably transduce airway epithelia in vivo. Nat Biotechnol. 2001;19(3):225–230. doi: 10.1038/85664
- Takada A, Robison C, Goto H, et al. A system for functional analysis of ebola virus glycoprotein. Proc Natl Acad Sci U S A. 1997;94(26):14764–14769. doi: 10.1073/pnas.94.26.14764
- Urbanowicz RA, McClure CP, King B, et al. Novel functional hepatitis C virus glycoprotein isolates identified using an optimized viral pseudotype entry assay. J Gen Virol. 2016;97(9):2265–2279. doi: 10.1099/jgv.0.000537
- Bi J; Wang H; Pei H; Han Q; Feng N; Wang Q; Wang X; Wang Z; Wei S; Ge L; Wu M; Liang H; Yang S; Yan F; Zhao Y; Xia X. A novel and secure pseudovirus reporter system based assay for neutralizing and enhancing antibody assay against marburg virus. Front Microbiol. 2022;13. doi: 10.3389/fmicb.2022.927122
- Chan SY, Speck RF, Ma MC, et al. Distinct mechanisms of entry by envelope glycoproteins of marburg and ebola (Zaire) viruses. J Virol. 2000;74(10):4933–4937. doi: 10.1128/jvi.74.10.4933-4937.2000
- Barrientos LG; Lasala F; Otero JR; Sanchez A; Delgado R. In vitro evaluation of cyanovirin-N antiviral activity, by use of lentiviral vectors pseudotyped with filovirus envelope glycoproteins. J Infect Dis. 2004;189(8):1440–1443. doi: 10.1086/382658
- Cruz-Oliveira C, Freire JM, Conceição TM, et al. Receptors and routes of dengue virus entry into the Host cells. FEMS Microbiol Rev. 2015;39(2):155–170. doi: 10.1093/femsre/fuu004
- Tani H; Shiokawa M; Kaname Y; Kambara H; Mori Y; Abe T; Moriishi K; Matsuura Y. Involvement of ceramide in the propagation of Japanese encephalitis virus. J Virol. 2010;84(6):2798–2807. doi: 10.1128/JVI.02499-09
- Negrete OA; Levroney EL; Aguilar HC; Bertolotti-Ciarlet A; Nazarian R; Tajyar S; Lee B. EphrinB2 is the entry receptor for nipah virus, an emergent deadly paramyxovirus. Nature. 2005;436(7049):401–405. doi: 10.1038/nature03838
- Pierson TC, Sánchez MD, Puffer BA, et al. A rapid and quantitative assay for measuring antibody-mediated neutralization of west Nile virus infection. Virology. 2006;346(1):53–65. doi: 10.1016/j.virol.2005.10.030
- Mercier-Delarue S, Durier C, Colin de Verdière N, et al. Screening test for neutralizing antibodies against yellow fever virus, based on a flavivirus pseudotype. PLoS One. 2017;12(5):e0177882. doi: 10.1371/journal.pone.0177882
- Kretschmer M, Kadlubowska P, Hoffmann D, et al. Zikavirus prME envelope pseudotyped human immunodeficiency virus type-1 as a novel tool for Glioblastoma-Directed Virotherapy. Cancers (Basel). 2020;12(4):1000. doi: 10.3390/cancers12041000
- Rana J, Slon Campos JL, Leccese G, et al. Role of Capsid Anchor in the morphogenesis of Zika Virus. J Virol. 2018;92(22): doi: 10.1128/jvi.01174-18
- Higa MM, Petersen J, Hooper J, et al. Efficient Production of hantaan and puumala pseudovirions for viral tropism and neutralization studies. Virology. 2012;423(2):134–142. doi: 10.1016/j.virol.2011.08.012
- Lee B-H; Yoshimatsu K; Araki K; Okumura M; Nakamura I; Arikawa J. A pseudotype vesicular stomatitis virus containing hantaan virus envelope glycoproteins G1 and G2 as an alternative to hantavirus vaccine in mice. Vaccine. 2006;24(15):2928–2934. doi: 10.1016/j.vaccine.2005.12.040
- Renoux VMG, Fleury MJJ, Bousarghin L, et al. Induction of antibody response against hepatitis E virus (HEV) with recombinant human papillomavirus pseudoviruses expressing truncated HEV capsid proteins in mice. Vaccine. 2008;26(51):6602–6607. doi: 10.1016/j.vaccine.2008.09.035
- Di Genova C; Sutton G; Paillot R; Temperton N; Pronost S; Scott SD. Studying longitudinal neutralising antibody levels against equid herpesvirus 1 in experimentally infected horses using a novel pseudotype based assay. Virus Res. 2024;339:199262. doi: 10.1016/j.virusres.2023.199262
- Suda Y; Fukushi S; Tani H; Murakami S; Saijo M; Horimoto T; Shimojima M. Analysis of the entry mechanism of crimean-congo hemorrhagic fever virus, using a vesicular stomatitis virus pseudotyping system. Arch Virol. 2016;161(6):1447–1454. doi: 10.1007/s00705-016-2803-1
- Zimmer G, Locher S, Berger Rentsch M, et al. Pseudotyping of vesicular stomatitis virus with the envelope glycoproteins of highly pathogenic avian influenza viruses. J Gen Virol. 2014;95(Pt 8):1634–1639. doi: 10.1099/vir.0.065201-0
- Tian Y; Zhao H; Liu Q; Zhang C; Nie J; Huang W; Li C; Li X; Wang Y. Development of in vitro and in vivo neutralization assays based on the pseudotyped H7N9 virus. Sci Rep. 2018;8(1):8484. doi: 10.1038/s41598-018-26822-6
- Wang W; Butler EN; Veguilla V; Vassell R; Thomas JT; Moos M; Ye Z; Hancock K; Weiss CD. Establishment of retroviral pseudotypes with influenza hemagglutinins from H1, H3, and H5 subtypes for sensitive and specific detection of neutralizing antibodies. J Virol Methods. 2008;153(2):111–119. doi: 10.1016/j.jviromet.2008.07.015
- Nie J, Liu L, Wang Q, et al. Nipah pseudovirus system enables evaluation of vaccines in vitro and in vivo using non-BSL-4 facilities. Emerg Microbes Infect. 2019;8(1):272–281. doi: 10.1080/22221751.2019.1571871
- Kaku Y; Noguchi A; Marsh GA; Barr JA; Okutani A; Hotta K; Bazartseren B; Fukushi S; Broder CC; Yamada A; Inoue S; Wang L-F. Second generation of pseudotype-based serum neutralization assay for nipah virus antibodies: sensitive and high-throughput analysis utilizing secreted alkaline phosphatase. J Virol Methods. 2012;179(1):226–232. doi: 10.1016/j.jviromet.2011.11.003
- Tamin A; Harcourt BH; Lo MK; Roth JA; Wolf MC; Lee B; Weingartl H; Audonnet J-C; Bellini WJ; Rota PA. Development of a neutralization assay for nipah virus using pseudotype particles. J Virol Methods. 2009;160(1–2):1–6. doi: 10.1016/j.jviromet.2009.02.025
- Kaku Y; Noguchi A; Marsh GA; McEachern JA; Okutani A; Hotta K; Bazartseren B; Fukushi S; Broder CC; Yamada A; Inoue S; Wang L-F. A neutralization test for specific detection of nipah virus antibodies using pseudotyped vesicular stomatitis virus expressing green fluorescent protein. J Virol Methods. 2009;160(1–2):7–13. doi: 10.1016/j.jviromet.2009.04.037
- Ma M, Kersten DB, Kamrud KI, et al. Murine leukemia virus pseudotypes of La Crosse and Hantaan Bunyaviruses: a system for analysis of cell tropism. Virus Res. 1999;64(1):23–32. doi: 10.1016/s0168-1702(99)00070-2
- Ma J, Chen R, Huang W, et al. In vitro and in vivo efficacy of a rift valley fever Virus vaccine based on pseudovirus. Hum Vaccines Immunother. 2019;15(10):2286–2294. doi: 10.1080/21645515.2019.1627820
- Haid S, Grethe C, Bankwitz D, et al. Identification of a human respiratory syncytial virus cell entry inhibitor by using a novel lentiviral pseudotype system. J Virol. 2016;90(6):3065–3073. doi: 10.1128/JVI.03074-15
- Chen Q, Nie J, Huang W, et al. Development and optimization of a sensitive pseudovirus-based assay for HIV-1 neutralizing antibodies detection using A3R5 cells. Hum Vaccines Immunother. 2017;14(1):199–208. doi: 10.1080/21645515.2017.1373922
- Louder MK, Sambor A, Chertova E, et al. HIV-1 envelope pseudotyped viral vectors and infectious molecular clones expressing the same envelope glycoprotein have a similar neutralization phenotype, but culture in peripheral blood mononuclear cells is associated with decreased neutralization sensitivity. Virology. 2005;339(2):226–238. doi: 10.1016/j.virol.2005.06.003
- Chan E, Heilek-Snyder G, Cammack N, et al. Development of a moloney murine leukemia virus-based pseudotype anti-HIV assay suitable for accurate and rapid evaluation of HIV entry inhibitors. J Biomol Screen. 2006;11(6):652–663. doi: 10.1177/1087057106288881
- Wang W, Nie J, Prochnow C, et al. A systematic study of the N-Glycosylation sites of HIV-1 envelope protein on infectivity and antibody-mediated neutralization. Retrovirology. 2013;10(1):14. doi: https://doi.org/10.1186/1742-4690-10-14
- Himes JE, Ho C, Nguyen QN, et al. Characterization of simian immunodeficiency virus variants anatomically compartmentalized in plasma and milk in chronically infected African green monkeys. J Virol. 2016;90(19):8795–8808. doi: 10.1128/JVI.00701-16
- Nie J, Wu X, Ma J, et al. Development of in vitro and in vivo rabies virus neutralization assays based on a high-titer pseudovirus system. Sci Rep. 2017;7(1):42769. doi: 10.1038/srep42769
- Salvador B, Zhou Y, Michault A, et al. Characterization of Chikungunya pseudotyped viruses: identification of refractory cell lines and demonstration of cellular tropism differences mediated by mutations in E1 glycoprotein. Virology. 2009;393(1):33–41. doi: 10.1016/j.virol.2009.07.013
- Hu D, Zhang J, Wang H, et al. Chikungunya Virus Glycoproteins Pseudotype with Lentiviral Vectors and Reveal a Broad Spectrum of Cellular Tropism. PLoS One. 2014;9(10):e110893. doi: 10.1371/journal.pone.0110893
- Kishishita N; Takeda N; Anuegoonpipat A; Anantapreecha S. Development of a pseudotyped-lentiviral-vector-based neutralization assay for Chikungunya virus infection. J Clin Microbiol. 2013;51(5):1389–1395. doi: 10.1128/JCM.03109-12
- Tong W, Yin X-X, Lee B-J, et al. Preparation of vesicular stomatitis virus pseudotype with Chikungunya virus envelope protein. Acta Virol. 2015;59(2):189–193. doi: 10.4149/av_2015_02_189
- Sharkey CM; North CL; Kuhn RJ; Sanders DA. Ross river virus glycoprotein-pseudotyped retroviruses and stable cell lines for their production. J Virol. 2001;75(6):2653–2659. doi: 10.1128/JVI.75.6.2653-2659.2001
- Hurwitz JL. Respiratory syncytial virus vaccine development. Expert Rev Vaccines. 2011;10(10):1415–1433. doi: 10.1586/erv.11.120
- Zhao J; Nie J; Jiao Y; Li L; Zhang T; Liu Q; Huang W; Wu H; Wang Y. Effect of the maturation of neutralizing antibodies on human immunodeficiency virus (HIV) envelope evolution in HIV-Infected subjects. Infect Genet Evol J Mol Epidemiol Evol Genet Infect Dis. 2016;38:82–89. doi: 10.1016/j.meegid.2015.12.012
- Steeds K, Hall Y, Slack GS, et al. Pseudotyping of VSV with ebola virus glycoprotein is superior to HIV-1 for the assessment of neutralising antibodies. Sci Rep. 2020;10(1):14289. doi: https://doi.org/10.1038/s41598-020-71225-1
- Bulcha JT, Wang Y, Ma H, et al. Viral vector platforms within the gene therapy landscape. Signal Transduct Target Ther. 2021;6(1):1–24. doi: 10.1038/s41392-021-00487-6
- Moore MJ; Dorfman T; Li W; Wong SK; Li Y; Kuhn JH; Coderre J; Vasilieva N; Han Z; Greenough TC; Farzan M; Choe H. Retroviruses pseudotyped with the severe acute respiratory syndrome coronavirus spike protein efficiently infect cells expressing angiotensin-converting enzyme 2. J Virol. 2004;78(19):10628–10635. doi: 10.1128/JVI.78.19.10628-10635.2004
- Sandrin V, Boson B, Salmon P, et al. Lentiviral vectors pseudotyped with a modified RD114 envelope glycoprotein show increased stability in sera and augmented transduction of primary lymphocytes and CD34+ cells derived from human and nonhuman primates. Blood. 2002;100(3):823–832. doi: 10.1182/blood-2001-11-0042
- Li Q, Liu Q, Huang W, et al. Current status on the development of pseudoviruses for enveloped viruses. Rev Med Virol. 2018;28:1. doi: 10.1002/rmv.1963
- Labbé RP, Vessillier S, Rafiq QA. Lentiviral vectors for T cell engineering: clinical applications, bioprocessing and future perspectives. Viruses. 2021;13(8):8. doi: https://doi.org/10.3390/v13081528
- Pollet J; Strych U; Chen W-H; Versteeg L; Keegan B; Zhan B; Wei J; Liu Z; Lee J; Kundu R; Adhikari R; Poveda C; Jose Villar M; Rani Thimmiraju S; Lopez B; Gillespie PM; Ronca S; Kimata JT; Reers M; Paradkar V; Hotez PJ; Elena Bottazzi M. Receptor-binding domain recombinant protein on alum-CpG induces broad protection against SARS-CoV-2 variants of concern. Vaccine. 2022;40(26):3655–3663. doi: 10.1016/j.vaccine.2022.05.007
- Wu X; Fang N; Liang Z; Nie J; Lang S; Fan C; Liang C; Huang W; Wang Y. Development of a Bioluminescent Imaging Mouse Model for SARS-CoV-2 Infection Based on a Pseudovirus System. Vaccines. 2023;11(7):1133. doi: 10.3390/vaccines11071133
- Zhou Y, Agudelo J, Lu K, et al. Inhibitors of SARS-CoV entry – identification using an internally-controlled dual envelope pseudovirion assay. Antiviral Res. 2011;92(2):187–194. doi: 10.1016/j.antiviral.2011.07.016
- Cruz-Cardenas JA, Gutierrez M, López-Arredondo A, et al. A pseudovirus-based platform to measure neutralizing antibodies in Mexico using sarS-CoV-2 as Proof-of-Concept. Sci Rep. 2022;12(1):17966. doi: 10.1038/s41598-022-22921-7
- Liu S, Selvaraj P, Lien CZ, et al. The PRRA insert at the S1/S2 site modulates cellular tropism of SARS-CoV-2 and ACE2 usage by the closely related bat RaTG13. J Virol. 2021;95(11):e01751–20. doi: 10.1128/JVI.01751-20
- Neerukonda SN, Vassell R, Herrup R, et al. Establishment of a well-characterized SARS-CoV-2 lentiviral pseudovirus neutralization assay using 293T cells with stable expression of ACE2 and TMPRSS2. PLoS One. 2021;16(3):e0248348. doi: 10.1371/journal.pone.0248348
- Valencia-Reséndiz DG, Palomino-Vizcaino G, Tapia-Vieyra JV, et al. Inhibition of human papillomavirus type 16 infection using an RNA aptamer. Nucleic Acid Ther. 2018;28(2):97–105. doi: 10.1089/nat.2017.0687
- Nie J; Liu Y; Huang W; Wang Y. Development of a triple-color pseudovirion-based assay to detect neutralizing antibodies against human papillomavirus. Viruses. 2016;8(4):107. doi: 10.3390/v8040107
- Ma Y; Mao G; Wu G; Chen M; Qin F; Zheng L; Zhang X-E. Dual-fluorescence labeling pseudovirus for real-time imaging of single SARS-CoV-2 entry in respiratory epithelial cells. ACS Appl Mater Interfaces. 2021;13(21):24477–24486. doi: 10.1021/acsami.1c03897
- Burkard C, Bloyet L-M, Wicht O, et al. Dissecting virus entry: replication-independent analysis of virus binding, internalization, and penetration using minimal complementation of β-galactosidase. PLoS One. 2014;9(7):e101762. doi: 10.1371/journal.pone.0101762
- Pastrana DV, Buck CB, Pang Y-YS, et al. Reactivity of human sera in a sensitive, high-throughput pseudovirus-based papillomavirus neutralization assay for HPV16 and HPV18. Virology. 2004;321(2):205–216. doi: 10.1016/j.virol.2003.12.027
- Bae DH; Marino M; Iaffaldano B; Fenstermaker S; Afione S; Argaw T; McCright J; Kwilas A; Chiorini JA; Timmons AE; Reiser J. Design and testing of vector-producing HEK293T cells bearing a genomic deletion of the SV40 T antigen coding region. Mol Ther Methods Clin Dev. 2020;18:631–638. doi: 10.1016/j.omtm.2020.07.006
- Li M; Huang J; Zhu Y; Huang Z; Zhang G; Huang J. Anti-L1 antibody-bound HPV16 pseudovirus is degraded intracellularly via TRIM21/Proteasomal pathway. Virol J. 2022;19(1):90. doi: 10.1186/s12985-022-01826-x
- Liu ML; Winther BL; Kay MA. Pseudotransduction of hepatocytes by using concentrated pseudotyped vesicular stomatitis virus G glycoprotein (VSV-G)-moloney murine leukemia virus-derived retrovirus vectors: comparison of VSV-G and amphotropic vectors for hepatic gene transfer. J Virol. 1996;70(4):2497–2502. doi: 10.1128/jvi.70.4.2497-2502.1996
- Zhang J, Cai Y, Lavine CL, et al. Structural and functional impact by SARS-CoV-2 Omicron Spike Mutations. Cell Rep. 2022;39(4):110729. doi: 10.1016/j.celrep.2022.110729
- Xiong H-L, Wu Y-T, Cao J-L, et al. Robust neutralization assay based on SARS-CoV-2 S-Protein-Bearing Vesicular Stomatitis Virus (VSV) pseudovirus and ACE2-overexpressing BHK21 cells. Emerg Microbes Infect. 2020;9(1):2105–2113. doi: 10.1080/22221751.2020.1815589
- Korber B, Fischer WM, Gnanakaran S, et al. Tracking changes in SARS-CoV-2 Spike: evidence that D614G increases infectivity of the COVID-19 virus. Cell. 2020;182(4):812. doi: 10.1016/j.cell.2020.06.043
- Kalemera MD, Capella-Pujol J, Chumbe A, et al. Optimized cell systems for the investigation of hepatitis C virus E1E2 glycoproteins. J Gen Virol. 2020;102(1):jgv001512. doi: 10.1099/jgv.0.001512
- Nie J; Li Q; Wu J; Zhao C; Hao H; Liu H; Zhang L; Nie L; Qin H; Wang M; Lu Q; Li X; Sun Q; Liu J; Fan C; Huang W; Xu M; Wang Y. Quantification of SARS-CoV-2 neutralizing antibody by a pseudotyped virus-based assay. Nat Protoc. 2020;15(11):3699–3715. doi: 10.1038/s41596-020-0394-5
- Hierholzer JC, Killington RA. Virus Isolation and Quantitation. Virol Methods Man. 1996:25–46. doi: 10.1016/B978-012465330-6/50003-8
- Savoie C, Lippé R. Optimizing human coronavirus OC43 growth and titration. PeerJ. 2022;10:e13721. doi: 10.7717/peerj.13721
- Dallner M; Harlow J; Nasheri N. Human coronaviruses do not transfer efficiently between surfaces in the absence of organic materials. Viruses. 2021;13(7):1352. doi: 10.3390/v13071352
- Kramberger P, Ciringer M, Štrancar A, et al. Evaluation of nanoparticle tracking analysis for total virus particle determination. Virol J. 2012;9(1):265. doi: https://doi.org/10.1186/1743-422X-9-265
- Gast M; Sobek H; Mizaikoff B. Nanoparticle tracking of adenovirus by light scattering and fluorescence detection. Hum Gene Ther Methods. 2019;30(6):235–244. doi: 10.1089/hgtb.2019.172
- Wu C; Xu Q; Wang H; Tu B; Zeng J; Zhao P; Shi M; Qiu H; Huang Y. Neutralization of SARS-CoV-2 Pseudovirus Using ACE2-Engineered Extracellular Vesicles. Acta Pharm Sin B. 2022;12(3):1523–1533. doi: 10.1016/j.apsb.2021.09.004
- Di Genova C, Sampson A, Scott S, et al. Production, titration, neutralisation, storage and lyophilisation of severe acute respiratory syndrome coronavirus 2 (SARS-CoV-2) lentiviral pseudotypes. Bio-Protoc. 2021;11(21):e4236. doi: 10.21769/BioProtoc.4236
- Zhang Y, Torre JCDL, Melikyan GB, et al. Human LAMP1 accelerates lassa virus fusion and potently promotes fusion pore dilation upon forcing viral fusion with non-endosomal membrane. PLOS Pathog. 2022;18(8):e1010625. doi: 10.1371/journal.ppat.1010625
- Weiss CD, Wang W, Lu Y, et al. Neutralizing and neuraminidase antibodies correlate with protection against influenza during a late season A/H3N2 Outbreak among unvaccinated military recruits. Clin Infect Dis Off Publ Infect Dis Soc Am. 2019;71(12):3096–3102. doi: 10.1093/cid/ciz1198
- Bi J, Wang H, Han Q, et al. A rabies virus-vectored vaccine expressing two copies of the marburg virus glycoprotein gene induced neutralizing antibodies against marburg virus in humanized mice. Emerg Microbes Infect. 2023;12(1):2149351. doi: 10.1080/22221751.2022.2149351
- Sholukh AM, Fiore-Gartland A, Ford ES, et al. Evaluation of cell-based and surrogate SARS-CoV-2 neutralization assays. J Clin Microbiol. 2021;59(10):e00527–21. doi: 10.1128/JCM.00527-21
- Condor Capcha JM; Lambert G; Dykxhoorn DM; Salerno AG; Hare JM; Whitt MA; Pahwa S; Jayaweera DT; Shehadeh LA. Generation of SARS-CoV-2 spike pseudotyped virus for viral entry and neutralization assays: a 1-week protocol. Front Cardiovasc Med. 2021;7:7. doi: 10.3389/fcvm.2020.618651
- Plotkin SA. Correlates of protection induced by vaccination. Clin Vaccine Immunol. 2010;17(7):1055–1065. doi: 10.1128/CVI.00131-10
- Cantoni D; Wilkie C; Bentley EM; Mayora-Neto M; Wright E; Scott S; Ray S; Castillo-Olivares J; Heeney JL; Mattiuzzo G; Temperton NJ. Correlation between pseudotyped virus and authentic virus neutralisation assays, a systematic review and meta-analysis of the literature. Front Immunol. 2023;14:1184362. doi: 10.3389/fimmu.2023.1184362
- Bentley EM; Mather ST; Temperton NJ. The use of pseudotypes to study viruses, virus sero-epidemiology and vaccination. Vaccine. 2015;33(26):2955–2962. doi: 10.1016/j.vaccine.2015.04.071
- Einstein MH, Baron M, Levin MJ, et al. Comparison of the immunogenicity and safety of CervarixTM and Gardasil® human papillomavirus (HPV) cervical cancer vaccines in healthy women aged 18–45 years. Hum Vaccin. 2009;5(10):705–719. doi: 10.4161/hv.5.10.9518
- Draper E, Bissett SL, Howell-Jones R, et al. A randomized, observer-blinded immunogenicity trial of cervarix® and gardasil® human papillomavirus vaccines in 12-15 year old girls. PLoS One. 2013;8(5):e61825. doi: 10.1371/journal.pone.0061825
- Millet JK, Whittaker GR. Murine leukemia virus (MLV)-based coronavirus spike-pseudotyped particle production and infection. Bio-Protoc. 2016;6(23):e2035. doi: 10.21769/BioProtoc.2035
- Pollet J, Chen W-H, Versteeg L, et al. SARS‑CoV-2 RBD219-N1C1: A Yeast-Expressed SARS-CoV-2 Recombinant Receptor-Binding Domain Candidate Vaccine Stimulates Virus Neutralizing Antibodies and T-Cell Immunity in Mice. Hum Vaccines Immunother. 2021;17(8):2356–2366. doi: https://doi.org/10.1080/21645515.2021.1901545
- Kuzmina A, Khalaila Y, Voloshin O, et al. SARS-CoV-2 spike variants exhibit differential infectivity and neutralization resistance to convalescent or post-vaccination sera. Cell Host Microbe. 2021;29(4):522–528.e2. doi: 10.1016/j.chom.2021.03.008
- Sun H; Xu J; Zhang G; Han J; Hao M; Chen Z; Fang T; Chi X; Yu C. Developing pseudovirus-based neutralization assay against omicron-included SARS-CoV-2 variants. Viruses. 2022;14(6):1332. doi: 10.3390/v14061332
- Crawford KHD, Eguia R, Dingens AS, et al. Protocol and reagents for pseudotyping lentiviral particles with SARS-CoV-2 spike protein for neutralization assays. Viruses. 2020;12(5):513. doi: 10.3390/v12050513
- Almahboub SA; Algaissi A; Alfaleh MA; ElAssouli M-Z; Hashem AM. Evaluation of neutralizing antibodies against highly pathogenic coronaviruses: a detailed protocol for a rapid evaluation of neutralizing antibodies using vesicular stomatitis virus pseudovirus-based assay. Front Microbiol. 2020;11:11. doi: 10.3389/fmicb.2020.02020
- Zheng Y, Larragoite ET, Williams ESCP, et al. Neutralization assay with SARS-CoV-1 and SARS-CoV-2 spike pseudotyped murine leukemia virions. Virol J. 2021;18(1):1. doi: 10.1186/s12985-020-01472-1
- Johnson MC, Lyddon TD, Suarez R, et al. Optimized pseudotyping conditions for the SARS-COV-2 spike glycoprotein. J Virol. 2020;94(21):e01062–20. doi: 10.1128/JVI.01062-20
- Nova N. Cross-species transmission of coronaviruses in humans and domestic mammals, what are the ecological mechanisms driving transmission, spillover, and disease emergence? Front Public Health. 2021;9:717941. doi: 10.3389/fpubh.2021.717941
- Zhang Y, Wei M, Wu Y, et al. Cross-species tropism and antigenic landscapes of circulating SARS-CoV-2 variants. Cell Rep. 2022;38(12):110558. doi: 10.1016/j.celrep.2022.110558
- Wang H; Zhang L; Liang Z; Nie J; Wu J; Li Q; Ding R; Zhang Y; Chen G; Wang Y; Wang H; Huang W. Infectivity and antigenicity of pseudoviruses with high-frequency mutations of SARS-CoV-2 identified in portugal. Arch Virol. 2022;167(2):459–470. doi: 10.1007/s00705-021-05327-0
- Liu DX, Liang JQ, Fung TS. Human Coronavirus-229E, -OC43, -NL63, and -HKU1 (Coronaviridae). Encycl virol. 2021:428–440. doi: 10.1016/B978-0-12-809633-8.21501-X
- Vlasova AN, Diaz A, Damtie D, et al. Novel canine coronavirus isolated from a hospitalized patient with pneumonia in East Malaysia. Clin Infect Dis. 2022;74(3):446–454. doi: 10.1093/cid/ciab456
- Sánchez CA; Li H; Phelps KL; Zambrana-Torrelio C; Wang L-F; Zhou P; Shi Z-L; Olival KJ; Daszak P. A strategy to assess spillover risk of bat SARS-related coronaviruses in Southeast Asia. Nat Commun. 2022;13(1):4380. doi: 10.1038/s41467-022-31860-w
- Mkhize NN, Yssel AEJ, Kaldine H, et al. Neutralization profiles of HIV-1 viruses from the VRC01 antibody mediated prevention (AMP) trials. PLOS Pathog. 2023;19(6):e1011469. doi: 10.1371/journal.ppat.1011469
- Al-Fattah Yahaya AA; Khalid K; Lim HX; Poh CL. Development of next generation vaccines against SARS-CoV-2 and variants of concern. Viruses. 2023;15(3):624. doi: 10.3390/v15030624
- Kumari M; Su S-C; Liang K-H; Lin H-T; Lu Y-F; Chen K-C; Chen W-Y; Wu H-C. Bivalent mRNA vaccine effectiveness against SARS-CoV-2 variants of concern. J Biomed Sci. 2023;30(1):46. doi: 10.1186/s12929-023-00936-0
- Sehr P, Rubio I, Seitz H, et al. High-throughput pseudovirion-based neutralization assay for analysis of natural and vaccine-induced antibodies against human papillomaviruses. PLoS One. 2013;8(10):e75677. doi: 10.1371/journal.pone.0075677