ABSTRACT
We propose a novel one-leg standardised rebound side-hop test (SRSH) specifically designed for detailed analysis of landing mechanics. Anterior cruciate ligament reconstructed persons (ACLR, n = 30) and healthy-knee controls (CTRL, n = 30) were tested for within-session and test-retest (CTRL only, n = 25) reliability and agreement. Trunk, hip and knee angles and moments in sagittal, frontal, and transversal planes during landing, including time to stabilisation (TTS), were evaluated using intra-class correlations (ICCs), average within-person standard deviations (SW) and minimal differences. Excellent within-session reliability were found for angles in both groups (most ICCs > 0.90, SW ≤ 5°), and excellent to good for moments (most ICCs > 0.80, SW ≤ 0.34 Nm/kg). Only knee internal rotation moment showed poor reliability (ICC < 0.4). Test-retest results were excellent to fair for all angles and moments (ICCs 0.47–0.91, SW < 5° and ≤ 0.25 Nm/kg), except for peak trunk lateral bending angle and knee internal rotation moment. TTS showed excellent to fair within-session reliability but poor test-retest results. These results, with a few exceptions, suggest promising potential of evaluating landing mechanics during the SRSH for ACLR and CTRL, and emphasise the importance of joint-specific movement control variables in standardised tasks.
Introduction
Three-dimensional (3D) analysis for increased understanding of movement control in relation to sports injury rehabilitation is increasingly used in research and clinics. The purposes are often to evaluate consequences of injuries (Oberländer, Brüggemann, Höher, & Karamanidis, Citation2013), to inform and monitor rehabilitation (Chua et al., Citation2016), or to provide guidelines for sports injury prevention (Kristianslund & Krosshaug, Citation2013; Taylor, Ford, Nguyen, & Shultz, Citation2016). Such measures are, however, seldom evaluated for reliability and agreement, which is imperative for their usefulness. A research field with increasing application of 3D analysis over the last decade is focusing on injury of the anterior cruciate ligament (ACL), where athletes are screened for lower limb biomechanics potentially associated with higher injury risk (Imwalle, Myer, Ford, & Hewett, Citation2009; Nagano, Ida, Akai, & Fukubayashi, Citation2009; Shimokochi, Ambegaonkar, Meyer, Lee, & Shultz, Citation2013; VandenBerg et al., Citation2017). An injury to the ACL often occurs during side-cutting or one-leg landing manoeuvres with the knee near full extension and with increased abduction (knee valgus) and internal rotation (Cochrane, Lloyd, Buttfield, Seward, & McGivern, Citation2007; Walden et al., Citation2015). It is one of the most common injuries in sports characterised by such side-to-side manoeuvre including football, floorball and basketball; roughly 3% of amateur athletes and up to 15% of elite athletes injure their ACL annually (Moses, Orchard, & Orchard, Citation2012). Recent statistics further show that 23% of athletes younger than 25 years suffer re-injury when returning to sport after ACL reconstruction (ACLR), equalling a 30–40 times greater risk relative to their non-injured counterparts (Wiggins et al., Citation2016). These injury rates call for implementation of valid and reliable tests that simulate challenging sport-like situations, to be used in screening and rehabilitation to ensure safer practise and return to sports, and that may further elucidate injury mechanisms.
One-leg landing tasks are commonly used in clinical settings to evaluate knee function, although argued not to sufficiently challenge ACL-injured limbs to the same extent as during sports participation (Taylor et al., Citation2016; Thomee et al., Citation2011). Sidestep cutting induces greater external and internal tibial rotation, and faster internal tibial rotation compared to single-limb landings (Nagano et al., Citation2009), and implies greater knee abduction and internal rotation angles and moments compared to bilateral drop jumps (Kristianslund & Krosshaug, Citation2013). Thus, for a successful return post-injury to sports or leisure activities that place considerable load on the lower limb (and in particular the knee joint), individuals should demonstrate sufficient lower limb control during tasks that involve cutting and side-to-side manoeuvres. This requires tests that are specifically designed to evaluate landing mechanics during such conditions, both of the lower limb but also of the trunk due to interrelationship of joint motion (Markström, Tengman, & Häger, Citation2017; Shimokochi et al., Citation2013). However, no study to date have evaluated reliability and agreement of trunk, hip, and knee landing mechanics during such side-to-side tasks for ACLR persons. The few reliability studies that exist have evaluated sidestep cutting tasks for healthy-knee athletes (Besier, Lloyd, Cochrane, & Ackland, Citation2001; Alenezi, Herrington, Jones, & Jones, Citation2016; Marshall et al., Citation2014; Mok, Bahr, & Krosshaug, Citation2018; Sankey et al., Citation2015; Sigward & Powers, Citation2006). In comparison to side- or cross-cutting tasks, a one-leg side-hop task has the advantage of being easier to administer, requires little space, eliminates compensational strategies of the contralateral leg and provides a direct between-leg comparison, especially if well standardised, which is an important aspect (Chua et al., Citation2016). Studies that have investigated reliability of the conventionally used side hop have focused only on capacity, i.e., the number of hops performed during 30 s over a distance of 40 cm (Gustavsson et al., Citation2006; Kockum & Heijne, Citation2015) or the time to complete 10 hops over a distance of 30 cm (Ortiz, Olson, Roddey, & Morales, Citation2005). These tests were designed to particularly target endurance, especially for the 30 s version, where fatigue is known to negatively affect knee stability (Melnyk & Gollhofer, Citation2007). Therefore, we propose the one-leg standardised rebound side hop test (SRSH), with the purpose of enabling reliable evaluation of joint-specific angles and moments and that may be used in a non-fatigued or fatigued state.
The primary aim was to evaluate within-session reliability and agreement for trunk, hip and knee angles and moments for ACLR persons in end phase of or post-rehabilitation and healthy-knee controls during SRSH landings. Further, we aimed to evaluate test-retest reliability and agreement for controls. Finally, we assessed reliability and agreement of Time to Stabilisation (TTS), another measure used to evaluate knee function in ACL-injured persons (Phillips & van Deursen, Citation2008; Webster & Gribble, Citation2010). Based on previous research for sidestep cutting (Besier et al., Citation2001; Alenezi et al., Citation2016; Marshall et al., Citation2014; Mok et al., Citation2018; Sankey et al., Citation2015; Sigward & Powers, Citation2006), we hypothesised that there would be excellent within-session reliability for angles and moments in the sagittal and frontal planes, and good reliability in transversal plane for both groups. We further hypothesised that TTS would generally show lower reliability than trunk, hip and knee angles and moments.
Methods
Participants
Sixty participants, 30 ACLR persons and 30 healthy-knee controls (CTRL) were included in this study for within-session analyses (). In addition, we evaluated test-retest reliability for 25 persons in CTRL (all who agreed to participate twice) on two separate occasions [mean (range), 16.4 (7–30) days]. The CTRL group consisted of both athletes and active non-athletes to represent the diversity of persons who suffer an ACL injury. All ACLR persons had suffered a unilateral ACL injury and undergone hamstring graft surgery [median (range), 19.0 (122.0) months before testing]. All had returned to physical activity after recommendation from physiotherapist. Persons were not included in any of the groups if they were not in the ages of 17–34 or had any neurological or musculoskeletal condition that would affect their ability to execute the SRSH. For sample size, at least 22 participants per group were needed in test-retest analyses to achieve an ICC of 0.8 as previously shown for the same angles and moments (Alenezi et al., Citation2016; Marshall et al., Citation2014; Mok et al., Citation2018) that differ from an ICC of 0.5 with a type I error of 0.05 and type II error of 0.20 (Walter, Eliasziw, & Donner, Citation1998). Hence, 30 participants in each group were included for within-session analyses and 25 participants in the CTRL group were considered sufficient for test-retest analyses. The project was approved by the Regional Ethical Review Board in Umeå, Sweden (Dnr. 2015/67–31) and all participants provided written informed consent in accordance with the declaration of Helsinki before partaking in the study.
Table 1. Background data for anterior cruciate ligament reconstructed group (ACLR) and control group (CTRL)
Procedures
An experienced physiotherapist performed knee examinations on all persons before testing, including a knee laxity assessment with a KT-1000 arthrometer (Medmetric Corporation, San Diego, CA, USA). Controls in test-retest were also asked about any potential reasons that might influence test outcome at the second test. Knee laxity data could not be obtained from one person in ACLR and three persons in CTRL due to absence of the physiotherapist performing all of these tests in the study, or in one case that the KT1000 was inaccessible. Passive spherical markers were attached with double-coated adhesive tape bilaterally on the acromion, lateral epicondyles of the humerus, anterior between the styloid processes of the radius and ulna, iliac crests, anterior superior iliac spines, greater trochanters, midpoint between the posterior superior iliac spines, lateral and medial femoral epicondyles, tibial tuberosities, fibular heads, lateral and medial malleoli, sustentaculum tali, lateral calcanei, proximal, and distal aspect of the calcanei (posterior), heads of the 5th metatarsal, medial aspect of the 1st metatarsal heads, and on the proximal base of the 1st metatarsals. Participants had rigid clusters with four markers on thighs and three markers on shanks and wore a head band with one marker on the forehead and one marker on both the right and left side of the head (cf. ). The clusters on the thighs were used to improve construct validity (reducing effects of soft tissue artefacts) and thereby increase reliability and precision (Collins, Ghoussayni, Ewins, & Kent, Citation2009). Hip joint centres were calculated using the thigh clusters based on a functional joint method during a hip circumduction movement (Schwartz & Rozumalski, Citation2005), and knee and ankle joint centres were calculated as the mid-point between the marker placements on femur epicondyles and malleoli, respectively. A stationary standing trial was recorded for anatomical modelling before removal of placement-sensitive markers (markers in dark grey in ).
Figure 1. Passive spherical markers were attached with double-coated adhesive tape on specific landmarks on the body to define 3D coordinates of the body segments
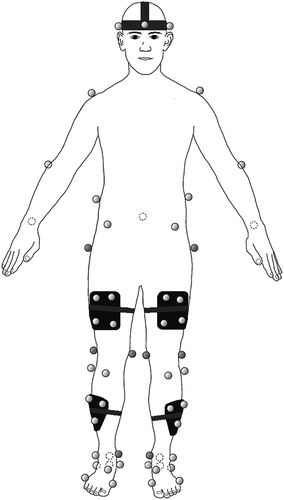
Kinematics were captured at 240 Hz using an eight camera motion capture system (Oqus®, Qualisys AB, Gothenburg, Sweden). Ground reaction forces (GRF) were recorded at 1200 Hz with two Kistler force plates (model 9260AA, Kistler Instrument AG, Winterthur, Switzerland) that were time synchronised with the motion capture system. Modular walkway elements (Kistler) were placed around the force plates, and were of the same colour and at the same level as the force plates, thus masking them to participants while providing additional surface area. The same person was test leader and applied markers and instructed participants on all test occasions.
The one-leg standardised rebound side hop (SRSH)
Participants performed the SRSH barefoot while holding a short rope (25 cm, with knots at each end) with both hands behind their back for standardisation and to emphasise lower limb landing control and engagement of the knee, as earlier shown for the squat jump (Hara, Shibayama, Takeshita, & Fukashiro, Citation2006) (see ). The distance to hop was standardised to 25% of body height based on the common 40 cm distance used in the conventional side hop test (Gustavsson et al., Citation2006; Kockum & Heijne, Citation2015) for a woman with a height of 160 cm. Such a normalisation provides a similar individual challenge indifferent of body height. The tailored distance was marked by two parallel tape strips on the floor. Participants stood on one leg on the side of one tape and were instructed to laterally hop over and land on the other side of the other tape, and to as fast as possible rebound back to the starting position. They were told to control the 2nd Landing and achieve a stable posture as quickly as possible and keeping the foot of the landing leg still on the floor. One or two practise trials were performed on each leg for familiarisation before 10 hops/leg were recorded. Trials were deemed successful provided that: the participant accomplished 3 s of single leg stance after landing without letting go of the rope, did not put the contralateral foot on the floor, and did not make significant adjustments with the ipsilateral foot in order to maintain balance. Participants alternated between legs every trial to avoid fatigue, starting on the non-affected leg for ACLR and dominant leg (defined as the self-preferred leg for kicking a ball) for CTRL. The time between each trial was approximately 5 s.
Figure 2. Illustration of the novel one-leg standardised rebound side hop (SRSH). The task was performed by: (1) standing upright on one leg on a force plate before hopping laterally with respect to the standing leg over a distance normalised to 25% of body height marked by tape, and landing on another force plate for (2), a rebound back to the starting point (3), 2nd Landing, and ‘stick’ the landing
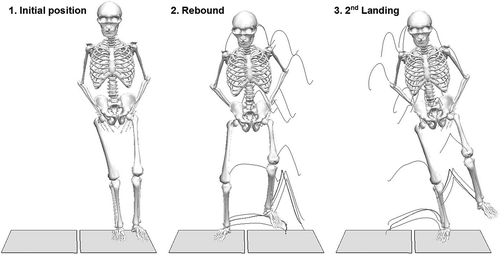
Data analyses
The software Qualisys Track Manager (v.2.2, Qualisys AB, Gothenborg, Sweden) and Visual3D (v.5.02.19, C-Motion Inc., Germantown, MD, USA) were used for data processing and calculation. Angles and moments for the trunk, hip, and knee were calculated using joint coordinate systems with the Cardan rotation sequence of X (mediolateral axis), Y (anteroposterior axis) and Z (longitudinal axis). Trunk angles were defined relative to the vertical axis of the lab coordinate system, hip joint angles were defined from movement of the thigh relative to the pelvis and knee joint angles as movement of the shank relative to the thigh. Hip and knee joint moments were calculated using inverse dynamics and normalised to body mass. Joint moments were presented as external moments in this study, e.g., an external knee flexion moment will tend to bend the knee. Kinematic and kinetic data were filtered with a fourth-order bidirectional low-pass Butterworth digital filter with a cut-off frequency of 15 Hz.
The two landings were each investigated for reliability and agreement, Rebound and 2nd Landing, both defined from initial contact (IC, vertical GRF > 20 N) to peak knee flexion, a particularly interesting period since the majority of ACL injuries occur in the eccentric phase of landings (Cochrane et al., Citation2007; Walden et al., Citation2015). The outcome variables were angles at IC and peak angles of trunk flexion and trunk lateral bending, as well as angles at IC, peak angles, and peak moments of hip flexion, hip adduction, hip internal rotation, knee flexion, knee abduction and knee internal rotation. Analyses for only the affected leg of persons in ACLR and for the dominant leg in CTRL were performed since no clear differences of reliability and agreement were shown between the legs within groups.
Calculation of TTS (Phillips & van Deursen, Citation2008; Webster & Gribble, Citation2010) was performed for 2nd Landing by filtering the GRF velocity using a second-order, recursive low-pass Butterworth digital filter with a cut-off frequency of 40 Hz. It was calculated in anteroposterior (AP) and mediolateral (ML) directions by differentiating their excursion signals from peak GRF, then rectified and filtered using a moving average window of 0.25 s. The TTS reflects the time taken for the GRF velocity in each direction to reach and remain below a stability threshold for at least 0.5 s. A resultant value R was also calculated by taking the square root of the sum of AP2 and ML2 (Fransz, Huurnink, de Boode, Kingma, & van Dieën, Citation2015). The threshold was calculated from a one-leg standing balance test for 10 s (with a 5 s pre-stabilisation period) from three trials for 10 controls. The overall standard deviation (SD) from the GRF velocity was averaged across trials and participants, with the threshold set at 2.5 SD in line with previous work (Phillips & van Deursen, Citation2008; Webster & Gribble, Citation2010).
Statistics
Reliability were investigated using intra-class correlations (ICC), for within-session using model ICC(3, 5) based on the first five successful trials, and in test-retest with model ICC(3, 1) using the average of these five trials for each test session. The classification of Fleiss (Fleiss, Citation1986) was adapted for interpretation (ICC < 0.4 = poor, ICC 0.4–0.75 = fair to good, ICC > 0.75 = excellent). Bland-Altman plots were used to screen for systematic bias, outliers and heteroscedasticity for test-retest data. Agreement was evaluated with the within-person SD (denoted SW) calculated by taking the square root of the average variances on group level, to provide clinically relevant values for expected errors in an individual (Bland & Altman, Citation1996).
Minimal differences (MD) for each variable was also assessed in test-retest by constructing a 95% confidence interval for the SW that take both test sessions into account. The level for statistical significance was set at 0.05 a priori. All statistical analyses were conducted using the Statistical Package for the Social Sciences (v.23, IBM SPSS Statistics, Armonk, New York, USA).
Results
Of the 10 hops performed, ACLR successfully performed an average of 8.2 hops (median: 8, range: 5–10) and CTRL an average of 8.9 hops (median: 9, range: 5–10). For the second test session, CTRL successfully performed an average of 9.0 hops (median: 9, range: 6–10). As for general movement strategies for both groups, in Rebound, persons landed and went into trunk lateral bending and flexion, with the hip in adduction and internal rotation, and with the knee going from abduction to adduction while externally rotated. Similar movement patterns were shown in 2nd Landing, although with both the hip and knee more in adduction and with less flexion moments (see ).
Figure 3. Ensemble trunk, hip, and knee kinematics (A) and kinetics (B) during the time-normalised Rebound for the affected leg of the ACLR group (solid line) and the dominant leg of the CTRL group (dotted line). Grey areas show the SD of the values for each group along the curve, and dark grey area shows where the SDs overlap between the groups. The horizontal line denotes the value of 0
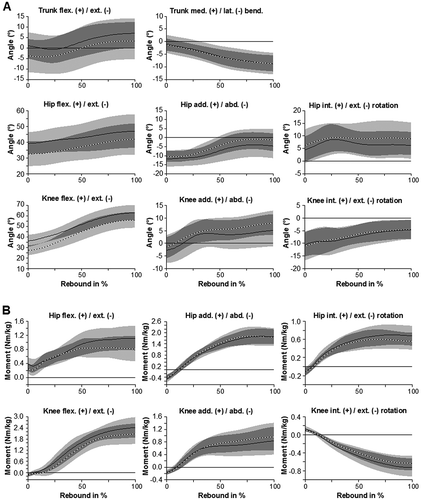
Figure 4. Ensemble trunk, hip, and knee kinematics (A) and kinetics (B) during the time-normalised 2nd Landing for the affected leg of the ACLR group (solid line) and the dominant leg of the CTRL group (dotted line). Grey areas show the SD of the values for each group along the curve, and dark grey area shows where the SDs overlap between the groups. The horizontal line denotes the value of 0
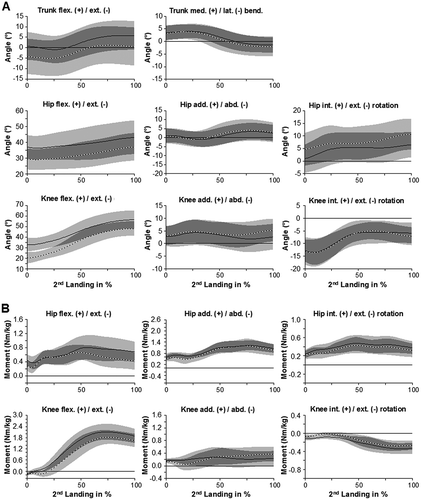
Within-session reliability
Within-session reliability for ACLR was excellent for all angles, except for trunk lateral bending at IC (ICC = 0.74), and excellent for 10 of 12 moments. Only moments of hip adduction and knee internal rotation had lower ICCs (0.68 and 0.43 in 2nd Landing, respectively). CTRL also showed excellent within-session reliability for most angles and moments. The few exceptions were for trunk lateral bending in 2nd Landing (ICCs of 0.67 at IC and 0.69 for peak angle), peak knee abduction moment in Rebound (ICC = 0.73), peak hip adduction moment in 2nd Landing (ICC = 0.74) and peak knee internal rotation moment in 2nd Landing (ICC = 0.23). All SW for ACLR and CTRL were ≤ 5.2° for angles and ≤ 0.34 Nm/kg for moments (cf. ). Reliability analyses of TTS showed excellent to good ICCs for ACLR in all directions (AP: 0.81, ML: 0.71, R: 0.80) but only fair ICCs for CTRL (AP: 0.58, ML: 0.51, R: 0.56). High relative (to mean values) SW were shown for both groups with values ≥ 37%.
Table 2. Within-session reliability with 95% CI and agreement for angles and moments (test session 1)
Test-retest reliability
Test-retest reliability was excellent to fair for all angles and moments for CTRL, with the two exceptions of peak trunk lateral bending angle (ICC = 0.36) and peak knee internal rotation moment (ICC = 0.07) in 2nd Landing (cf. ). All SW were ≤ 4.5° (MDs ≤ 12.6°) for angles and ≤ 0.25 Nm/kg (MDs ≤ 0.68 Nm/kg) for moments in test-retest. Regarding TTS, fair to poor reliability was found with ICCs of 0.46 in AP, 0.34 in ML and 0.40 in R, with high relative MDs ≥ 57% of mean values for both test sessions.
Table 3. Test-retest reliability with 95% CI and agreement for angles and moments for controls (dominant leg)
Discussion and implications
This study proposed and evaluated a novel one-leg standardised rebound side hop (SRSH) aimed for biomechanical evaluation of populations with ACL injury or healthy athletes. The test generally proved to have satisfactory results of reliability and agreement of trunk, hip, and knee angles and moments, both within-session for ACLR and CTRL, and in test-retest for CTRL. Only peak trunk lateral bending angle and knee internal rotation moment had poor reliability in one of two landing phases. Further, TTS had generally good within-session reliability, although poor test-retest reliability and agreement.
The design of the SRSH with the purpose of enabling reliable biomechanical evaluation of lower limb function includes four main strengths: First, the standardisation of the test, as recommended for sport-specific tasks (Chua et al., Citation2016) with a normalised hop distance, restricted arm movements, and alternating legs during test performance. Second, the side-to-side movement, that simulates sport specific situations and particularly challenges the lower limbs, especially for the rebound landing. The fast movement to the side followed by an immediate rebound, occurs during changes of directions in sports. This movement is also comparable to evaluated side-cutting movements (Besier et al., Citation2001; Alenezi et al., Citation2016; Marshall et al., Citation2014; Mok et al., Citation2018; Sankey et al., Citation2015; Sigward & Powers, Citation2006). Third, the one-leg performance eliminates the possibility of compensating with the contralateral leg as previously shown for ACLR persons (Roos, Button, & van Deursen, Citation2014). Fourth, the small space that is required to perform the test, as compared to side cutting which has been limited to analyses of only one direction due to restricted lab-space (Alenezi et al., Citation2016; Jones, Herrington, Munro, & Graham-Smith, Citation2014).
This is the first study that comprehensively have evaluated reliability and agreement for 3D angles and moments of multiple joints in a sport-specific task for a relatively large sample size of both ACLR and healthy knee persons. Our results of excellent to good reliability for discrete trunk, hip and knee angles and moments for both ACLR and CTRL, without apparent differences in either ICCs or SW between groups, indicate that our findings may be representative of both injured and healthy populations. These results extend on those of the few existing and recently published reliability studies that have evaluated sidestep cutting for healthy knee athletes (Alenezi et al., Citation2016; Marshall et al., Citation2014; Mok et al., Citation2018; Sankey et al., Citation2015). More specifically, within-session ICCs ≥ 0.75 were presented for hip and knee angles (either at IC or peak values) with measures of agreement ≤ 4.1°, and ICCs for peak moments ≥ 0.89, similar to our results (Mok et al., Citation2018). For test-retest of those studies, most hip and knee angles and moments had fair to excellent ICCs, while knee internal rotation angle and moment generally displayed lower ICCs (Alenezi et al., Citation2016; Marshall et al., Citation2014; Mok et al., Citation2018). Measures of agreement were, however, generally somewhat greater than those that we found, especially for moments of ≤ 0.56 Nm/kg. The inter-trial, inter-session and inter-observer (for marker placements) variability for hip and knee angles has also been shown to be ≤ 5.5° for full waveforms, with inter-trial variability being lower than inter-session and inter-observer variability (Sankey et al., Citation2015), further corroborated by our findings of within versus test-retest reliability. It is, however, important to consider the use of different models to calculate ICCs and measures of agreement, time between test-retest, number of trials analysed and the specific task performed when contrasting findings between research reports. However, the general lower reliability that we observed for moments compared to angles, especially for knee internal rotation moment, is congruent with earlier papers (Alenezi et al., Citation2016; Mok et al., Citation2018; Sankey et al., Citation2015). Indeed, large variability for knee joint moments during cutting, especially in early stance phase, have resulted in advice of cautious interpretation (Sankey et al., Citation2015). Potential reasons, as also discussed by Sankey and colleagues, may be the dynamic nature of the SRSH with side-to-side movement. The lower reliability may be explained by inconsistencies in technique with variation in foot placement and/or postural control (especially due to restricted arms) that affects the ground reaction forces of the landing phase. Such variation may have a greater effect on joint moments (and on TTS) while joint angles remain roughly similar. Further, our estimates from test-retest with (dependent on landing) MDs of 5.0–6.0° for peak knee abduction angle, and 7.1–7.8° for peak knee internal rotation angle, should be considered when assessing their contribution in terms of ACL injury risk, or when comparing different populations.
Compared to joint-specific angles and moments, TTS may instead be assumed to reflect whole body coordination for landing control. However, our results of variable within-session reliability for ACLR and CTRL and poor test-retest reliability for CTRL question the usefulness of this measure. Similar results of only fair within-session reliability for TTS have in fact been demonstrated with ICCs of 0.64 and 0.41, respectively, during the forward running-stop and the one-leg hop for distance (Phillips & van Deursen, Citation2008). There is also no consensus of how to best determine and calculate TTS, which is often based on GRF velocity, centre of pressure velocity or their derivatives (Fransz et al., Citation2015; Phillips & van Deursen, Citation2008; Webster & Gribble, Citation2010). Large variation between different calculation methods have also been reported (Fransz et al., Citation2015), thus questioning the validity of such measures. In relation to our results from test-retest analyses, the TTS seem to be a poor measure to reflect lower limb function and control. If, however, using TTS, the velocity is recommended to detect differences in postural sway between groups (Masani, Vette, Abe, & Nakazawa, Citation2014). Methods other than 3D angles and moments that more directly estimate knee stability and control, particular in relation to rotation, after ACLR or other lower limb injuries are probably more suitable than TTS. A finite helical axis approach (Grip & Häger, Citation2013; Grip, Tengman, & Häger, Citation2015) and an inverted pendulum method (Oberländer et al., Citation2013) are relevant methodologies that should be further explored in this context. Moreover, instead of focusing solely on discrete variables for evaluation of biomechanics as in the present study, waveform analyses include more data over the time-period of interest. Recent attempts of such analyses (e.g., Coffey, Harrison, Donoghue, & Hayes, Citation2011; Hebert-Losier, Schelin, Tengman, Strong, & Häger, Citation2018) evaluated kinematic curves during running for persons with chronic Achilles tendon injury, and during hop tasks for persons with ACL injury in the long-term perspective, respectively. Current methods used to investigate reliability of curve data (e.g., the coefficient of multiple correlation) have been criticised (Roislien, Skare, Opheim, & Rennie, Citation2012), and more sophisticated methods are being discussed (Coffey et al., Citation2011; Roislien, Rennie, & Skaaret, Citation2012). Such waveform analyses show potential for advanced biomechanical evaluations, which our proposed SRSH is designed for.
From a clinical perspective, the main difficulty of a biomechanical evaluation as performed in the current study is the technical demands, which are substantial and not currently clinician friendly. The greater standardisation of the SRSH compared to the commonly evaluated SH does, however, enable biomechanical analyses without having to consider the effects of fatigue or arm usage that each may cause large variations. Such an approach could enable simpler software analysis of angles even based on videos. The feasibility of the test also need consideration in clinical testing or when planning for future data collection of the SRSH for detailed biomechanical analyses (due to the success rate being 50% for a few ACLR and CTRL persons).
Some limitations of this study need to be mentioned. Common for all studies using motion capture is the variability in marker placement that may affect test-retest reliability. To minimise such effects, we used a standardised test protocol and the same person applied the markers for all participants. Possible soft tissue artefacts (skin relative to bone motion) also need attention, especially for frontal and transversal plane angles and moments. We used rigid clusters to improve construct validity (reducing effects of soft tissue artefacts) and thereby increase reliability and precision (Collins et al., Citation2009). The present study did not address the test-retest reliability for ACLR persons. However, the comparable within-session reliability for ACLR and CTRL may argue that similar results may also be seen for test-retest reliability. Further, the time duration between test-retest varied in our study, but did not influence reliability and agreement (ICCs or within-person SDs). We included both sexes for better extrapolation of our results to the ACLR population in general, even if more women participated and we were not able to address sex differences.
Conclusion
This novel one-leg SRSH is a reliable test to evaluate trunk, hip and knee angles and moments in two eccentric landing phases for both ACL reconstructed persons and healthy-knee controls, with the exception of peak trunk lateral bending angle and knee internal rotation moment. Joint-specific movement control variables should most likely be used in favour of more general performance measures such as TTS, due to questionable validity, reliability and agreement, in order to evaluate lower limb landing control in sports and rehabilitation.
Ethical approval
The Regional Ethical Review board in Umeå approved the study (reference: 2015/67-31).
Acknowledgments
We are grateful to the participants of this study, and would like to acknowledge Jonas Selling (M.Eng.) for technical assistance, Eva Tengman (PT, PhD) for clinical examination of the participants and Andrew Strong (PhD student) for help with data collection and proofreading. No financial or personal relationship exists that may have influenced this manuscript, and there are no conflicts of interest that could have either influenced the study or preparation of the manuscript.
Disclosure statement
No potential conflict of interest was reported by the authors.
Additional information
Funding
References
- Alenezi, F., Herrington, L., Jones, P., & Jones, R. (2016). How reliable are lower limb biomechanical variables during running and cutting tasks. Journal of Electromyography and Kinesiology, 30, 137–142. doi:10.1016/j.jelekin.2016.07.001
- Besier, T. F., Lloyd, D. G., Cochrane, J. L., & Ackland, T. R. (2001). External loading of the knee joint during running and cutting maneuvers. Medicine and Science in Sports and Exercise, 33, 1168–1175, PMID:11445764.
- Bland, J. M., & Altman, D. G. (1996). Measurement error. British Medical Journal, 312, 1654. doi:10.1136/bmj.312.7047.1654
- Chua, E. N., Yeung, M. Y., Fu, S. C., Yung, P. S., Zhang, Y., Feng, H., & Chan, K. M. (2016). Motion task selection for kinematic evaluation after anterior cruciate ligament reconstruction: A systematic review. Arthroscopy, 32, 1453–1465. doi:10.1016/j.arthro.2016.01.057
- Cochrane, J. L., Lloyd, D. G., Buttfield, A., Seward, H., & McGivern, J. (2007). Characteristics of anterior cruciate ligament injuries in Australian football. Journal of Science and Medicine in Sport, 10, 96–104. doi:10.1016/j.jsams.2006.05.015
- Coffey, N., Harrison, A. J., Donoghue, O. A., & Hayes, K. (2011). Common functional principal components analysis: A new approach to analyzing human movement data. Human Movement Science, 30, 1144–1166. doi:10.1016/j.humov.2010.11.005
- Collins, T. D., Ghoussayni, S. N., Ewins, D. J., & Kent, J. A. (2009). A six degrees-of-freedom marker set for gait analysis: Repeatability and comparison with a modified Helen Hayes set. Gait and Posture, 30, 173–180. doi:10.1016/j.gaitpost.2009.04.004
- Fleiss, J. (1986). The design and analysis of clinical experiments. New York: Wiley.
- Fransz, D. P., Huurnink, A., de Boode, V. A., Kingma, I., & van Dieën, J. H. (2015). Time to stabilization in single leg drop jump landings: An examination of calculation methods and assessment of differences in sample rate, filter settings and trial length on outcome values. Gait and Posture, 41, 63–69. doi:10.1016/j.gaitpost.2014.08.018
- Grip, H., & Häger, C. (2013). A new approach to measure functional stability of the knee based on changes in knee axis orientation. Journal of Biomechanics, 46, 855–862. doi:10.1016/j.jbiomech.2012.12.015
- Grip, H., Tengman, E., & Häger, C. K. (2015). Dynamic knee stability estimated by finite helical axis methods during functional performance approximately twenty years after anterior cruciate ligament injury. Journal of Biomechanics, 48, 1906–1914. doi:10.1016/j.jbiomech.2015.04.016
- Gustavsson, A., Neeter, C., Thomee, P., Silbernagel, K. G., Augustsson, J., Thomee, R., & Karlsson, J. (2006). A test battery for evaluating hop performance in patients with an ACL injury and patients who have undergone ACL reconstruction. Knee Surgery, Sports Traumatology, Arthroscopy, 14, 778–788. doi:10.1007/s00167-006-0045-6
- Hara, M., Shibayama, A., Takeshita, D., & Fukashiro, S. (2006). The effect of arm swing on lower extremities in vertical jumping. Journal of Biomechanics, 39, 2503–2511. doi:10.1016/j.jbiomech.2005.07.030
- Hebert-Losier, K., Schelin, L., Tengman, E., Strong, A., & Häger, C. K. (2018). Curve analyses reveal altered knee, hip, and trunk kinematics during drop-jumps long after anterior cruciate ligament rupture. The Knee, 25, 226–239. doi:10.1016/j.knee.2017.12.005
- Imwalle, L. E., Myer, G. D., Ford, K. R., & Hewett, T. E. (2009). Relationship between hip and knee kinematics in athletic women during cutting maneuvers: A possible link to noncontact anterior cruciate ligament injury and prevention. Journal of Strength and Conditioning Research, 23, 2223–2230. doi:10.1519/JSC.0b013e3181bc1a02
- Jones, P. A., Herrington, L. C., Munro, A. G., & Graham-Smith, P. (2014). Is there a relationship between landing, cutting, and pivoting tasks in terms of the characteristics of dynamic valgus? American Journal of Sports Medicine, 42, 2095–2102. doi:10.1177/0363546514539446
- Kockum, B., & Heijne, A. I. (2015). Hop performance and leg muscle power in athletes: Reliability of a test battery. Physical Therapy in Sport, 16, 222–227. doi:10.1016/j.ptsp.2014.09.002
- Kristianslund, E., & Krosshaug, T. (2013). Comparison of drop jumps and sport-specific sidestep cutting: Implications for anterior cruciate ligament injury risk screening. American Journal of Sports Medicine, 41, 684–688. doi:10.1177/0363546512472043
- Markström, J. L., Tengman, E., & Häger, C. K. (2017). ACL-reconstructed and ACL-deficient individuals show differentiated trunk, hip, and knee kinematics during vertical hops more than 20 years post-injury. Knee Surgery, Sports Traumatology, Arthroscopy, 26, 358–367. doi:10.1007/s00167-017-4528-4
- Marshall, B. M., Franklyn-Miller, A. D., King, E. A., Moran, K. A., Strike, S. C., & Falvey, E. C. (2014). Biomechanical factors associated with time to complete a change of direction cutting maneuver. Journal of Strength and Conditioning Research, 28, 2845–2851. doi:10.1519/JSC.0000000000000463
- Masani, K., Vette, A. H., Abe, M. O., & Nakazawa, K. (2014). Center of pressure velocity reflects body acceleration rather than body velocity during quiet standing. Gait and Posture, 39, 946–952. doi:10.1016/j.gaitpost.2013.12.008
- Melnyk, M., & Gollhofer, A. (2007). Submaximal fatigue of the hamstrings impairs specific reflex components and knee stability. Knee Surgery, Sports Traumatology, Arthroscopy, 15, 525–532. doi:10.1007/s00167-006-0226-3
- Mok, K. M., Bahr, R., & Krosshaug, T. (2018). Reliability of lower limb biomechanics in two sport-specific sidestep cutting tasks. Sports Biomechanics, 1–11. doi:10.1080/14763141.2016.1260766.
- Moses, B., Orchard, J., & Orchard, J. (2012). Systematic review: Annual incidence of ACL injury and surgery in various populations. Research in Sports Medicine, 20, 157–179. doi:10.1080/15438627.2012.680633
- Nagano, Y., Ida, H., Akai, M., & Fukubayashi, T. (2009). Biomechanical characteristics of the knee joint in female athletes during tasks associated with anterior cruciate ligament injury. The Knee, 16, 153–158. doi:10.1016/j.knee.2008.10.012
- Oberländer, K. D., Brüggemann, G. P., Höher, J., & Karamanidis, K. (2013). Altered landing mechanics in ACL-reconstructed patients. Medicine and Science in Sports and Exercise, 45, 506–513. doi:10.1249/MSS.0b013e3182752ae3
- Ortiz, A., Olson, S. L., Roddey, T. S., & Morales, J. (2005). Reliability of selected physical performance tests in young adult women. Journal of Strength and Conditioning Research, 19, 39–44. doi:10.1519/14163.1
- Phillips, N., & van Deursen, R. W. (2008). Landing stability in anterior cruciate ligament deficient versus healthy individuals: A motor control approach. Physical Therapy in Sport, 9, 193–201. doi:10.1016/j.ptsp.2008.06.010
- Roislien, J., Rennie, L., & Skaaret, I. (2012). Functional limits of agreement: A method for assessing agreement between measurements of gait curves. Gait and Posture, 36, 495–499. doi:10.1016/j.gaitpost.2012.05.001
- Roislien, J., Skare, O., Opheim, A., & Rennie, L. (2012). Evaluating the properties of the coefficient of multiple correlation (CMC) for kinematic gait data. Journal of Biomechanics, 45, 2014–2018. doi:10.1016/j.jbiomech.2012.05.014
- Roos, P. E., Button, K., & van Deursen, R. W. (2014). Motor control strategies during double leg squat following anterior cruciate ligament rupture and reconstruction: An observational study. Journal of NeuroEngineering and Rehabilitation, 11, 19. doi:10.1186/1743-0003-11-19
- Sankey, S. P., Raja Azidin, R. M., Robinson, M. A., Malfait, B., Deschamps, K., Verschueren, S., … Vanrenterghem, J. (2015). How reliable are knee kinematics and kinetics during side-cutting manoeuvres? Gait and Posture, 41, 905–911. doi:10.1016/j.gaitpost.2015.03.014
- Schwartz, M. H., & Rozumalski, A. (2005). A new method for estimating joint parameters from motion data. Journal of Biomechanics, 38, 107–116. doi:10.1016/j.jbiomech.2004.03.009
- Shimokochi, Y., Ambegaonkar, J. P., Meyer, E. G., Lee, S. Y., & Shultz, S. J. (2013). Changing sagittal plane body position during single-leg landings influences the risk of non-contact anterior cruciate ligament injury. Knee Surgery, Sports Traumatology, Arthroscopy, 21, 888–897. doi:10.1007/s00167-012-2011-9
- Sigward, S., & Powers, C. M. (2006). The influence of experience on knee mechanics during side-step cutting in females. Clinical Biomechanics, 21, 740–747. doi:10.1016/j.clinbiomech.2006.03.003
- Taylor, J. B., Ford, K. R., Nguyen, A. D., & Shultz, S. J. (2016). Biomechanical Comparison of Single- and Double-Leg Jump Landings in the Sagittal and Frontal Plane. Orthopaedic Journal of Sports Medicine, 4, 2325967116655158. doi:10.1177/2325967116655158
- Thomee, R., Kaplan, Y., Kvist, J., Myklebust, G., Risberg, M. A., Theisen, D., … Witvrouw, E. (2011). Muscle strength and hop performance criteria prior to return to sports after ACL reconstruction. Knee Surgery, Sports Traumatology, Arthroscopy, 19, 1798–1805. doi:10.1007/s00167-011-1669-8
- VandenBerg, C., Crawford, E. A., Sibilsky Enselman, E., Robbins, C. B., Wojtys, E. M., & Bedi, A. (2017). Restricted hip rotation is correlated with an increased risk for anterior cruciate ligament injury. Arthroscopy, 33, 317–325. doi:10.1016/j.arthro.2016.08.014
- Walden, M., Krosshaug, T., Bjorneboe, J., Andersen, T. E., Faul, O., & Hagglund, M. (2015). Three distinct mechanisms predominate in non-contact anterior cruciate ligament injuries in male professional football players: A systematic video analysis of 39 cases. British Journal of Sports Medicine, 49, 1452–1460. doi:10.1136/bjsports-2014-094573
- Walter, S. D., Eliasziw, M., & Donner, A. (1998). Sample size and optimal designs for reliability studies. Statistics in Medicine, 17, 101–110. PMID:9463853.
- Webster, K. A., & Gribble, P. A. (2010). Time to stabilization of anterior cruciate ligament-reconstructed versus healthy knees in national collegiate athletic association division I female athletes. Journal of Athletic Training, 45, 580–585. doi:10.4085/1062-6050-45.6.580
- Wiggins, A. J., Grandhi, R. K., Schneider, D. K., Stanfield, D., Webster, K. E., & Myer, G. D. (2016). Risk of secondary injury in younger athletes after anterior cruciate ligament reconstruction: A systematic review and meta-analysis. American Journal of Sports Medicine, 44, 1861–1876. doi:10.1177/0363546515621554