ABSTRACT
We compared knee landing mechanics with presumed relation to risk of anterior cruciate ligament (ACL) injury among three single-leg hop tests and between legs in individuals with unilateral ACL reconstruction. Thirty-four participants (>10 months’ post-surgery, 23 females) performed the standardised rebound side hop (SRSH), maximal hop for distance (OLHD) and maximal vertical hop (OLVH). We calculated the following knee outcomes from motion capture and force plate data: finite helical axis inclination angles (approximates knee robustness), frontal and transversal plane angles at initial contact, peak angles of abduction and internal rotation during landing, and peak external moments of flexion, abduction and internal rotation during landing. Repeated-measures MANOVA analysis (‘sex’ as covariate) confirmed that SRSH induced greater angles and moments, particularly in the frontal plane, compared to OLHD and OLVH. There was between-leg asymmetry for peak knee flexion moment for males during OLHD and OLVH, and for females during SRSH. Our results advocate the SRSH over OLHD and OLVH for assessment of knee landing control to screen for movement patterns potentially related to ACL injury risk. However, clear differences in both knee kinematics and kinetics between OLHD and SRSH motivate the use of both tests to evaluate different aspects of landing control.
Introduction
Rupture of the anterior cruciate ligament (ACL) is a serious and unfortunately common knee injury that particularly occur during sports participation. (Dai et al., Citation2012; Griffin et al., Citation2000) Despite treatment with ACL reconstruction, rerupture is a major problem with nearly one out of four (23%) athletes below 25 years of age who suffers either a rupture of the graft or of the contralateral ACL after returning to sports. (Wiggins et al., Citation2016) Screening procedures of knee control are considered important to decrease the risk of primary ACL injury and that of reinjury, and landings of single-leg hop tests are commonly evaluated for this purpose. (Hewett & Bates, Citation2017) A primary and particularly secondary ACL injury is devastating for the athlete and is associated with high societal costs for the health-care system. Incentives for decreasing the amount of ACL injuries are therefore substantial.
ACL injuries mainly occur early after ground contact in non-contact landing situations with a side-to-side manoeuvre during sports. (Boden et al., Citation2000; Cochrane et al., Citation2007; Johnston et al., Citation2018; Koga et al., Citation2010; Olsen et al., Citation2004; Walden et al., Citation2015) The knee is often unfavourably positioned in abduction and internal rotation with full loading on one leg at the time of injury. (Johnston et al., Citation2018; Koga et al., Citation2010; Krosshaug et al., Citation2007; Olsen et al., Citation2004; Renstrom et al., Citation2008; Walden et al., Citation2015) Experimental research that simulates landing situations demonstrates that the ACL strain is highest during the combined external loadings of knee abduction and internal tibial rotation moments. (Bates et al., Citation2019; Kiapour et al., Citation2015) The peak knee abduction moment during a drop vertical jump task also predicts ACL injury with a high degree (>70%) of specificity and sensitivity. (Hewett et al., Citation2005) These results highlight the importance of evaluating knee frontal and transversal plane movement control during single-leg hop landings among sports people to implement actions that may decrease the risk of ACL injury or reinjury. However, there is still no consensus of which specific hop test or tests that should be used for this purpose.
The maximal one-leg hop for distance (OLHD) and one-leg vertical hop (OLVH) are frequently used single-leg hop tests to evaluate lower limb functional performance and movement patterns as a consequence of ACL injury. (Hegedus et al., Citation2015) However, their primary anteroposterior and vertical hop directions are not accurately simulating sports manoeuvres where ACL injuries are common, (Patterson & Delahunt, Citation2013; Thomee et al., Citation2011) and may therefore be less suitable tests when screening for outcomes presumably related to increased risk of ACL injury or reinjury. A recently developed test that may be more relevant for this purpose is the standardised rebound side hop (SRSH). The SRSH shows high reliability for evaluation of knee kinematic and kinetic parameters during landing, (Markström, Schelin et al., Citation2018) and compared to e.g., side or crosscutting tasks the SRSH requires less space (sidestep cutting has been limited to analyses of only one direction due to restricted lab-space), (Alenezi et al., Citation2016; Jones et al., Citation2014) excludes possible compensational strategies of the contralateral leg in preparation to the cutting, and allows a direct between-leg comparison for asymmetry. The SRSH may reveal landing mechanics that are more aligned with situations related to ACL injury and reinjury compared to OLHD and OLVH, despite the latter tests being performed with maximal effort. However, there are currently no studies confirming this, and evidence is scarce of detailed landing mechanics among hop tests. Previous findings show that single-leg hop tests with substantial side-to-side movement induces greater knee abduction angles and moments than bilateral hop tests. (Kristianslund & Krosshaug, Citation2013; Pappas et al., Citation2007; Taylor et al., Citation2016) A few studies also indicate greater angles and moments of knee abduction and internal rotation when adding a side-to-side component to single-leg landing tasks in anteroposterior or vertical change of directions. (Besier et al., Citation2001; Sell et al., Citation2006; Taylor et al., Citation2016) Knee frontal and transversal plane outcomes, therefore, seem to differ between single-leg hop landings performed in different directions, but further investigations are needed for consensus and particularly for ACL-reconstructed individuals for population validity. There is also a need to determine the discriminative ability for asymmetry in knee-specific outcomes between hop test landings among ACL-reconstructed persons.
The current aim was to compare knee frontal and transversal plane kinematic and kinetic outcomes presumed to be related to risk of ACL injury, and between-leg asymmetry, during landings among the hop tests OLHD, OLVH and SRSH for ACL-reconstructed individuals. We hypothesised that the SRSH, despite being submaximal, would induce greater knee angles and moments in frontal and transversal planes with presumed risk for ACL injury and be more discriminative for asymmetry between legs than the OLHD and OLVH.
Materials and methods
Participants
Thirty-four ACL-reconstructed individuals (all hamstring graft) with a minimum of 10 months between surgery and testing between 17 and 34 years of age participated in this study (). They were prospectively and sequentially recruited over a period of ~2 years, mainly from the orthopaedic clinic of the regional hospital but also in a few cases from another local sports medicine clinic and from advertisements around the university and hospital campus. Individuals had the following inclusion criteria: unilateral ACL injury, no other musculoskeletal or neurological pathology that might affect hop test results, and confidence in performing the different hop tests without discomfort. Minor surgery for the meniscus and minor injury to collateral ligaments were allowed, but any complete collateral ligament tear or any major surgical procedure resulted in exclusion. All participants were interviewed regarding inclusion criteria over the phone before participating and again questioned about previous injuries and knee pain that might influence test outcome before testing. They were either actively performing or had completed rehabilitation programmes including exercises that aim to improve lower limb strength and knee control. Participants were recruited from the university hospital orthopaedic clinic, through adverts around the university area, and through sports clubs. For sample size, we performed power analyses on data from six individuals with ACL-reconstruction (three males and three females) for the three tasks for peak knee abduction angle and moment using G*Power (version 3.1.9.3). These analyses were conducted for repeated-measures ANOVAs and both revealed the need for total group sample sizes of six individuals with a wanted power of 0.8. The six individuals we included in the power analyses would therefore be sufficient for repeated measures ANOVAs for these specific outcomes. However, since we aimed to perform a repeated measure MANOVA, we adhered to a sample of at least 30 individuals with ACL reconstruction, since this was the sample size we aimed for in the overall project (Markström, Grip, Schelin, Häger et al., Citation2019b; Markström, Schelin et al., Citation2018). This study was approved by the regional ethical review board in Umeå, Sweden (Dnr. 2015/67-31). All persons provided written informed consent in accordance with the declaration of Helsinki before partaking.
Table 1. Group characteristics
Test procedure
Testing was performed inU-motion lab, Umeå, Sweden, where participants first answered the 2000 International Knee Documentation Committee Subjective Knee Form, Knee injury and Osteoarthritis Outcome Score subscales, Lysholm scale and Tegner activity scale. They then had their knees clinically examined by an experienced physiotherapist including a knee laxity assessment with a KT-1000 arthrometer (Medmetric Corporation) with a 30-lb (13.5-kg) load of anterior pull force. Markers were then applied and the hop tests were performed in the pre-decided order of OLHD, OLVH and SRSH with landings on force plates masked by modular walkway elements (). The rational for this order was to have the same increase in balance difficulty across the hop test landings (Liu & Heise, Citation2013; Wikstrom et al., Citation2008; also confirmed at pilot testing) to have the participants similarly prepared for the tasks. The OLHD was performed with participants initially standing upright on the testing leg before hopping straight forward as far as possible and landing on the same leg. They were instructed to try to ‘stick’ the landing and regain control as fast as possible. The OLVH was performed in the same manner with participants initially standing upright on the testing leg before hopping upward as high as possible and landing on the same leg, and with the same instructions at landing. The SRSH was performed in the same manner with participants initially standing upright on the testing leg before hopping straight laterally (with respect to the hopping leg) over a distance of 25% of body height, followed by an immediate rebound back to the starting position for the same leg. This rebound landing was analysed. All hops were performed barefoot and with arms behind the back holding a short rope (25 cm with knots on each side) for standardisation, to avoid obstructing the reflective markers and to emphasise lower limb landing control. One or two test trials for each leg was allowed for all tests before data were collected. Trials in all tests were deemed successful following 3 s of single-leg stance after landing without participants letting go of the rope or losing their balance by putting the contralateral foot down or making significant adjustments with the stance leg to maintain balance. For the OLHD and OLVH, trials were performed until three hops were approved although with maximal five trials allowed on each leg to avoid onset of fatigue (considering the maximal effort). For the SRSH 10 trials were performed irrespective of how many that were successful. Participants alternated between legs every trial for all tests to minimise any fatigue, and had 5-s rest between trials and ~5-min rest between tests.
Figure 1. Schematic descriptions of the hop tests performed from left to right. Arm movement were restricted by holding a 25 cm rope behind the back for all three tests. In A, the one-leg hop for distance (OLHD), the participant hopped straight forward as far as possible in this illustrated case with the right leg and landed on the same leg. In B, the one-leg vertical hop (OLVH), the participant hopped straight up as high as possible with in this case the right leg and landed on the same leg. In C, the standardised rebound side hop (SRSH), the participant shown hopped with the left leg straight laterally (to the left) over a distance of 25% of body height and then immediately back again (to the right) and landed on the same leg. The landings that were analysed are circled. The lines on the ground indicate tape that marked the starting position and the distance to hop over (in SRSH)
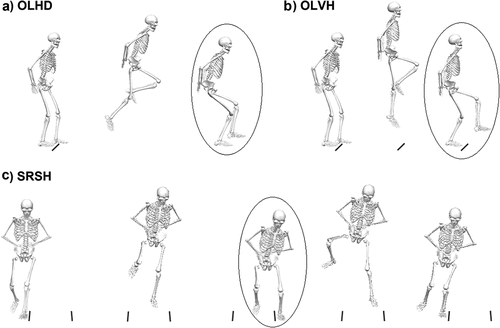
Instrumentation and data processing
An eight-camera motion capture system (Oqus 300, Qualisys AB, Gothenburg, Sweden) registered kinematics at 240 Hz. Two Kistler force plates (model 9260AA, Winterthur, Switzerland) were time synchronised with the motion capture system and recorded ground reaction forces at 1200 Hz. Passive reflective markers were attached to the skin at anatomical landmarks to construct a six degree-of-freedom full-body model. (Markström, Schelin et al., Citation2018) Relevant markers to attain knee angles and moments were markers placed bilaterally on the highest point on the iliac crests, anterior superior iliac spines, the midpoint between the posterior superior iliac spines, greater trochanters, lateral and medial femoral epicondyles, tibial tuberosities, fibular heads, lateral and medial malleoli, proximal and distal posterior aspects of the calcanei, lateral calcanei, heads of the 5th metatarsal, medial aspect of the 1st metatarsal heads and on the proximal base of the 1st metatarsals. Participants wore rigid clusters with four markers on thighs to improve construct validity by reducing soft tissue artefacts and thereby increase reliability and precision of tracking. (Collins et al., Citation2009) The thigh was defined with the origin of the local coordinate system set to the location of the hip joint centre calculated with a functional joint method, Schwartz & Rozumalski (Citation2005) using the 4-marker thigh cluster and Pelvis segment as reference. The Z-axis (longitudinal axis) was set along the axis passing from the mid-point between the lateral and medial femoral epicondyles to the hip joint centre, the Y-axis (anteroposterior axis) was made perpendicular to the plane formed by the hip joint centre and the femoral epicondyles, and the X-axis (mediolateral axis) was made perpendicular to both the Y-axis and Z-axis to form a right-handed coordinate system. The shank was defined with the origin of the local coordinate system set to the mid-point between the lateral and medial femoral epicondyles (i.e. the knee joint centre). The Z-axis was set along the axis passing from the mid-point between the lateral and medial malleoli to the knee joint centre, the Y-axis was set perpendicular to a least squares plane fit of the femoral epicondyles and the malleoli, and the X-axis was made perpendicular to both the Y-axis and Z-axis to form a right-handed coordinate system. Knee joint angles were defined by movement of the shank in relation to the thigh. After a stationary recording in standing for modelling purposes, placement sensitive markers were removed. The same test leader palpated for marker placements and instructed participants at all tests.
Qualisys Track Manager software (v.2.2, Qualisys AB, Gothenburg, Sweden) was used for capturing, construction of three-dimensional (3D) marker coordinates and for interpolation and identification of markers. The marker data were exported to Visual3D software (v.5.02.19, C-Motion Inc. Germantown, MD, USA) and filtered at 15 Hz with a critically damped digital filter before the outcome variables were calculated. Inverse dynamics was then used to calculate joint moments normalised to body mass, which were described as external moments in this study (e.g., an external knee abduction moment will tend to put the knee in abduction). The mass of each segment was calculated based on the proportions of Dempster (Dempster, Citation1955). The angle and moment data were then filtered with a fourth-order bidirectional low-pass Butterworth digital filter with a cut-off frequency of 15 Hz. The Cardan rotation sequence XYZ was used (Cole et al., Citation1993).
Outcome variables
There were in total nine discrete knee-specific outcome variables extracted in each of the three hop landings for this study: finite helical axis (FHA) inclination angles (Grip & Häger, Citation2013; Grip et al., Citation2015; Markström, Grip, Schelin, Häger et al., Citation2019a) that estimate how robust (the ability to tolerate perturbation and maintain joint configuration (Reeves et al., Citation2007)) the knee is during landing, frontal and transversal plane angles at initial contact, peak angles of abduction and internal rotation, and external peak moments of flexion, abduction and internal rotation. Excellent trial-to-trial reliability (intraclass correlation coefficients of 0.83–0.98) have recently been reported for these eight outcomes for the SRSH among ACL-reconstructed individuals (Markström, Grip, Schelin, Häger et al., Citation2019b; Markström, Schelin et al., Citation2018). The landing phase of interest was defined from initial contact (vertical force >20 N) to maximal knee flexion.
The discrete FHA inclination angles (0–90°) were calculated as the knee helical axis relative to the knee flexion-extension axis, for helical rotation intervals of 10° from initial contact throughout the landing phases across the hop tests. This procedure computes how much the knee motion differed from a pure flexion-extension movement over the 10° knee motion intervals, regardless of whether this difference resulted from adduction/abduction and/or internal/external rotation. More specifically, greater knee motion in the frontal or transversal planes relative to the sagittal plane during one 10° motion interval results in the FHA being directed more along the anterior-posterior or vertical axis, respectively. This results in an FHA inclination angle >45° representing a less robust knee, which is considered as poor knee control. The 10° interval was set to be small enough to capture movement changes over short time intervals but still to be within acceptable error levels from error simulations. The first two consecutive motion intervals of 10° were analysed to include the initial knee motion after initial contact, since ACL injuries seem to occur shortly after initial contact. (Koga et al., Citation2010; Krosshaug et al., Citation2007) These motion intervals were numbered as FHA-1 and FHA-2, respectively.
Statistical analyses
This study complied with the recent published recommendations for statistical analysis in Sports Biomechanics by Harrison and colleagues. (Harrison et al., Citation2020) The mean value was calculated for each outcome variable from the successful trials for each test, which were for OLHD 2.9 ± 0.6 trials for both the ACL-reconstructed and non-injured legs, for OLVH 2.9 ± 0.7 and 2.8 ± 0.6 trials for the ACL-reconstructed and non-injured legs, respectively, and for SRSH 7.9 ± 1.6 and 8.0 ± 1.3 trials for the ACL-reconstructed and non-injured legs, respectively. These mean values for the nine knee-specific outcome variables for each leg were included in a repeated-measure MANOVA to investigate the effect of ‘test’ (OLHD, OLVH, SRSH), ‘leg’ (ACL-reconstructed leg, non-injured leg) and the interaction between ‘test’ and ‘leg’, with ‘sex’ (male, female) as a covariate. A significant main effect was followed with univariate analyses and pairwise comparisons using Bonferroni adjustment. Data were screened for normality using Shapiro–Wilk test and histograms, and for sphericity using Mauchly´s test with degrees of freedom for the F-ratio corrected when necessary. Partial eta-squared values as effect sizes (ESs; 0.01 = small, 0.1 = medium and 0.25 = large) (Vacha-Haase & Thompson, Citation2004) were presented for main effects. A 5% α level for statistical significance was applied for all analyses, performed with SPSS (v 25, IBM).
Results
The repeated-measure MANOVA analysis confirmed that there were significant multivariate effects for ‘test’ (F (18, 15) = 24.8, P <.001, ES = 0.97 [large]) and ‘leg’ (F (9, 24) = 6.9, P < .001, ES = 0.72 [large]), but not for their interaction (F (18, 15) = 1.5, P = .201). Univariate analyses showed significant differences between tests for FHA-1 inclination angle during landing (P < .001, ES = 0.50 [large]), frontal plane angle at initial contact (P < .001, ES = 0.54 [large]), transversal plane angle at initial contact (P = .017, ES = 0.12 [medium]), peak abduction angle during landing (P < .001, ES = 0.56 [large]), peak internal rotation angle during landing (P = .018, ES = 0.12 [medium]), peak flexion moment during landing (P < .001, ES = 0.66 [large]), peak abduction moment during landing (P < .001, ES = 0.47 [large]), and peak internal rotation moment during landing (P < .001, ES = 0.81 [large]). Descriptive data for ACL-reconstructed and non-injured legs are found in Supplementary material (Table S1). Specifically, the SRSH landing induced greater FHA-1 inclination angle ()), greater abduction angle at initial contact (-d)), greater peak abduction angle ()), greater peak abduction moment ()), and greater internal rotation moments ()) compared to both the maximal OLHD and OLVH landings. Additionally, the OLHD landing induced greater adduction angle at initial contact than the OLVH ()), more external rotation at initial contact than the SRSH ()), lower peak abduction angle than the OLVH ()), greater peak internal rotation angle than the OLVH ()), greater peak flexion moment than both OLVH and SRSH ()), and lower peak abduction moment than the OLVH ()).
Figure 2. Mean values with 95% confidence interval error bars for the knee kinematic outcomes for both legs, with FHA-1 inclination angle in (a), FHA-2 inclination angle in (b), adduction/abduction angle at initial contact in (c), internal/external rotation angle at initial contact in (d), peak abduction angle during landing in (e), and peak internal rotation angle during landing in (f). Positive values are shown for adduction and internal rotation, negative values for abduction and external rotation. The horizontal line shows the value of 0. ACL, anterior cruciate ligament; IC, initial contact; FHA, finite helical axis; OLHD, one-leg hop for distance; OLVH, one-leg vertical hop; SRSH, standardised rebound side hop
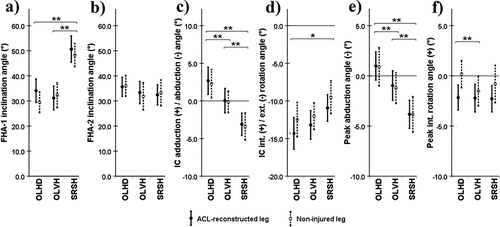
Figure 3. Mean values with 95% confidence interval error bars for the knee kinetic outcomes for both legs, with external peak flexion moment during landing in (a), external peak abduction moment during landing in (b), and external peak internal rotation moment during landing in (c). Positive values are shown for adduction and internal rotation, negative values for abduction and external rotation. The horizontal line shows the value of 0. ACL, anterior cruciate ligament; OLHD, one-leg hop for distance; OLVH, one-leg vertical hop; SRSH, standardised rebound side hop
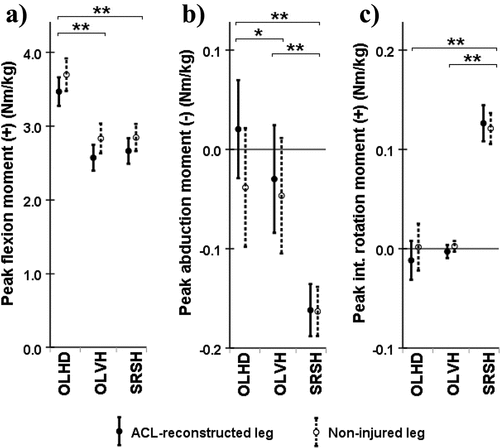
The covariate ‘sex’ had a significant effect on ‘leg’ (F (9, 24) = 3.0, P = .016, ES = 0.53 [large]) with univariate significant findings for FHA-2 inclination angle (P = .023, ES = 0.15 [medium]) and peak flexion moment (P = .013, ES = 0.18 [medium]), but not for the remaining outcomes (P ≥ .284). Males had significantly lower FHA-2 inclination angles compared to females for the non-injured leg during OLVH (mean±SD: males 24.1 ± 12.7° and females 35.6 ± 15.9°, P = .045) and during SRSH (males 25.5 ± 8.5° and females 36.9 ± 16.0°, P = .035), but not during OLHD or for the ACL-reconstructed leg for any test or between legs (P ≥ .101). For peak flexion moment, both males and females displayed lesser moments for the ACL-reconstructed leg compared to their non-injured leg, although for different tests (males: OLHD and OLVH, P = .003 and .005, respectively; females: SRSH, P = .002) (). Males also had greater flexion moments than females for the non-injured leg during OLHD (males 4.05 ± 0.63 Nm/kg and females 3.53 ± 0.57 Nm/kg, P = .021), but not during OLVH or SRSH or for the ACL-reconstructed leg for any test (P ≥ .137). Asymmetry was also found for peak abduction moment (P = .015, ES = 0.157 [medium]), with lower moment for the ACL-reconstructed leg compared to the non-injured leg irrespective of sex.
Figure 4. Differences in peak knee flexion moment between the ACL-reconstructed leg and the non-injured leg for males and females shown with mean values and 95% confidence interval error bars. ACL, anterior cruciate ligament; OLHD, one-leg hop for distance; OLVH, one-leg vertical hop; SRSH, standardised rebound side hop
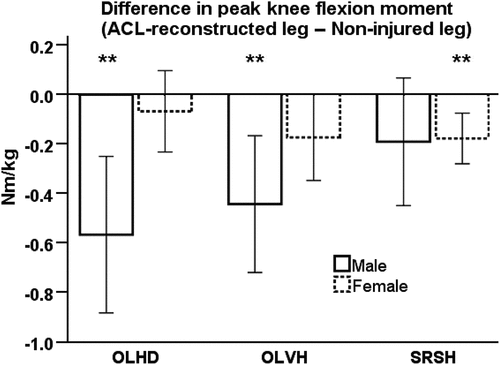
Discussion and implications
We compared knee kinematic and kinetic outcomes presumed to be related to risk of ACL injury, and between-leg asymmetry of these outcomes, during landings between three single-leg hop tests (OLHD, OLVH, SRSH) for ACL-reconstructed individuals. As hypothesised we found that SRSH landings induced greater angles and moments with presumed risk of ACL injury as evident by greater FHA-1 inclination angle (indicative of less robust knees), the knee positioned more in abduction both at initial contact and during landing, and by greater external moments of peak abduction and internal rotation during landing. Asymmetry between legs with lower external peak flexion moment for the ACL-reconstructed leg differed between sexes across the tests (males: OLHD and OLVH; females: SRSH), while lower external peak knee abduction moment was seen for the ACL-reconstructed leg irrespective of sex.
Our results support the few existing and quite recently published studies that evaluated knee joint-specific landing mechanics between single-leg tasks. Among them, a report from 201425 investigated relationships between the single-leg drop landing, running with 90° cutting, and running with 180° pivoting for 20 asymptomatic female soccer players. Their findings showed that both cutting and pivoting tasks resulted in greater peak knee abduction angles and moments than single-leg drop landing. A study from 201647 compared 2 two-legged and two single-leg landing tasks in either sagittal (distance to hop = leg length) or frontal (distance to hop = half leg length) planes with a subsequent maximal vertical hop, for 15 recreationally active females. The two single-leg tasks were unfortunately not contrasted, but the frontal plane landings had lower peak angles of knee internal rotation although greater peak moments of knee abduction and internal rotation than the sagittal plane landings. Finally, another study from 201653 compared a lateral shuffle task (180° change of direction, similar to the SRSH) and the 45° side-step among a heterogeneous group of 78 patients (mixed sex and pathology with different diagnoses of lower limb injury, pain or instability but also back pain) and one group of 34 asymptomatic persons (mixed sex). The lateral shuffle displayed greater peak knee abduction angles although similar abduction moments, for both groups. Summarising these findings it seems that single-leg landings including a side-to-side manoeuvre provoke knee motions thought to increase the risk of ACL injury (Bates et al., Citation2019; Kiapour et al., Citation2015) more than landings without such manoeuvres. None of these studies did, however, include a homogeneous group of individuals with ACL reconstruction which is important for issues of population validity. Our study adds important information for individuals with ACL reconstruction in this regard.
Our results of greater frontal and transversal plane angles and moments with presumed risk to ACL injury during SRSH relative OLHD and OLVH corroborate the above-mentioned study findings. We particularly consider the high FHA-1 inclination angle as important to highlight since it is clinically relevant with regard to two major issues of ACL injury occurrence: the timing of ACL rupture shortly after impact (Koga et al., Citation2010; Krosshaug et al., Citation2007) and the frontal and transversal plane motion related to ACL strain and injury. (Bates et al., Citation2019; Kiapour et al., Citation2015; Koga et al., Citation2010; Krosshaug et al., Citation2007; Renstrom et al., Citation2008) The FHA-1 inclination angle group mean values of 50.6°/48.2° (ACL-reconstructed knee/non-injured knee) during SRSH shows that more knee movement occurred in the frontal and/or transversal planes relative the sagittal plane (since >45°) for the first 10° motion interval after initial contact ()). This was not the case for OLHD (34.1°/29.4°) and OLVH (31.2°/32.3°) landings. The knee control was however more robust for the second 10° motion interval for all three hop test landings and for both legs with similar group mean FHA-2 inclination angles ranging between 31.9° and 36.0° ()). The greater FHA-1 inclination angle in relation to the greater abduction angle at initial contact and the greater external peak moments of abduction and internal rotation moment (which tends to place the knee in more abducted and internally rotated positions) during SRSH compared to both OLHD and OLVH collectively suggests a more ACL-injury prone movement pattern for the knee. Although no research has investigated if these outcomes during SRSH is related to ACL injury or reinjury risk, our results show promising potential considering that frontal and transversal plane motion are related to ACL strain and injury. (Bates et al., Citation2019; Kiapour et al., Citation2015; Koga et al., Citation2010; Krosshaug et al., Citation2007; Renstrom et al., Citation2008) Nevertheless, the different knee landing mechanics for OLHD, OLVH and SRSH motivate that these tests may complement each other in a test battery for proper evaluation of knee landing control. The same argument has been stated before for OLHD, OLVH and the ordinary side hop test for endurance (30s version), although for evaluation of symmetry in hop performances among individuals with ACL reconstruction. (Gustavsson et al., Citation2006) Indeed, it is recommended to use multiple tests with different characteristics regarding testing and training for injury prevention purposes, and for ACL-injured persons during rehabilitation before recommending a return to sport to decrease the risk of reinjury. (Ardern et al., Citation2016; Thomee et al., Citation2011) The SRSH has also been shown to elicit different movement strategies for the trunk, hip and knee between ACL-reconstructed individuals and matched asymptomatic individuals, (Markström, Grip, Schelin, Häger et al., Citation2019b) thus having a discriminant function for group comparison testing. Our data may also be interpreted such that single-leg side-to-side hop tests may be performed at a later stage of rehabilitation than single-leg hop tests in forward or vertical directions, to avoid overloading the graft during ongoing maturation and healing after ACL reconstruction. (Nagelli & Hewett, Citation2017) Further research are however required to substantiate this argument.
A clear difference in execution between the OLHD, OLVH and SRSH were the requirement of landing stabilisation and the maximal effort with associated hop distances in the former tests, while the SRSH instead included a rebound hop that required a plyometric component and had a standardised hop distance. These differences introduce two factors that need to be considered in relation to our results. First, the rebound hop in SRSH results in participants preparing for the second hop, which may in itself result in greater frontal and transversal plane angles and moments. Tendencies of such findings were found for a forward bilateral drop landing with (compared to without) a subsequent vertical jump, which resulted in ~1.5° more knee abduction, although with no differences for knee abduction moment, for recreational athlete females. (Ishida et al., Citation2018) Male athletes did however not display any differences in knee abduction angles or moments in that study. Our significant results of differences between SRSH to OLHD and OLVH for peak knee abduction angles were ~3-5°, thus two to three times larger than the differences found in the previous study for females. This suggests an actual effect not fully explained by the rebound in SRSH. Greater peak moments of knee abduction and internal rotation have also been found for frontal plane landings compared to sagittal plane landings including subsequent maximal vertical jumps. (Taylor et al., Citation2016) A rebound landing at the transition between the first to the second hop of a single-leg triple hop test also show knee angles and moments of adduction, (Amir Curcio et al., Citation2015) similar to our results for OLHD and OLVH. As such, the collective differences in kinematic and kinetic outcomes between landings in this study suggest a substantially greater need for knee frontal and transversal plane landing control during SRSH even if a rebound hop is added to the other landings. Most sports manoeuvres also include a rebound movement utilising the stretch-shortening cycle of the lower limbs, thus proposing improved ecological validity for the SRSH compared to the OLHD or OLVH. Second, we chose to compare the SRSH to the conventional maximal-effort OLHD and OLVH since these tests are commonly performed and recommended in clinical environments (thus for ecological validity). (Hegedus et al., Citation2015; Thomee et al., Citation2011) Similar data that we show for OLHD and OLVH with the knee in adduction or in neutral position have also been reported for a 30 cm forward hop (Deneweth et al., Citation2010) and for OLVH performed by middle-aged persons with and without ACL reconstruction both during take-off and landing phases. (Markström, Tengman et al., Citation2018) As such, the hop tests OLHD, OLVH and SRSH seem to show different landing mechanics mainly due to the different hop directions. A further explanation to the diverse results between tests may be an increased demand of medial-lateral trunk control during SRSH. Increased lateral trunk flexion results in more knee abduction during single-leg landings (Saito et al., Citation2020) and greater external knee abduction moment during side-cutting. (Jamison et al., Citation2012) It is also common with emphasised lateral trunk motion towards the ACL-injured knee at time of injury. (Stuelcken et al., Citation2016) A hop test performed in medial-to-lateral direction may emphasise trunk rotation towards the testing leg and lateral trunk flexion at landing to efficiently complete the subsequent lateral hop, and as a result show increased knee abduction angle and moment. A recent study reported that a bilateral landing with mid-flight trunk rotation to the testing leg resulted in greater angles of knee abduction and internal rotation, and greater external abduction moment, when compared to landing without mid-flight rotation. (Critchley et al., Citation2020) A unilateral hop landing test in medial-to-lateral direction may therefore be even more challenging and suitable to screen for excessive knee abduction angles and moments for ACL injury prevention purposes.
The difference in asymmetry between males and females for external peak flexion moment among the three tests are also worth mentioning (). The larger asymmetry shown for males for OLHD and OLVH may be related to a higher level of physical capacity in relation to a generally shorter time after surgery at testing compared to females in our study (median: males 12.5 months, females 20.0 months). Thus, males may not have been recovered to the same extent as females, which would become more evident in the maximal effort tests OLHD and OLVH than for the SRSH. However, the difference between males and females in asymmetry for SRSH may be due to the difference in samples sizes (11 males, 23 females), since the mean values of asymmetry was the same but the confidence intervals differed. If calculating a 95% confidence interval of asymmetry for males during SRSH with the current standard deviation (this would likely be smaller in reality) but using a sample size of 23 males, significant asymmetry is indeed shown (95% CI: −0.35 to −0.03 Nm/kg). These results highlight that the three tests, but particularly OLHD and SRSH that differed the most, may be used together in a test battery to evaluate different aspects of knee control among individuals with ACL reconstruction.
This study has some methodological considerations that need mentioning. First, motion capture systems with skin-attached markers only provide an estimation of joint kinematics and kinetics, where soft tissue artefacts unavoidably introduce error to the data. Knee kinematics and kinetics in the transversal plane are particularly susceptible to errors. (Kernozek et al., Citation2006; Sankey et al., Citation2015) Since we evaluated the within-person difference between tests (utilising the same marker setup) these results are not as prone to marker-placement errors since this would affect all landings. We also tried to limit these artefacts by using rigid clusters to increase reliability and precision, (Collins et al., Citation2009) and by standardising marker placements applied by the same test leader. Our approach to calculate knee FHA inclination angles for the two first consecutive helical motion intervals of 10° is considered a strength, since this captures 3D knee motion as the main outcome of interest. Motion capture systems are however difficult to implement in clinical environments given high costs and the need for expertise and time for data processing. Simpler 2D analysis of frontal plane projection angles can instead be performed for the SRSH using video recording given that the test is easy to administer and is time-efficient while requiring minimal space. Our protocol (barefoot, restricted arms) also need consideration when contrasting our results to those from other studies using different protocols, seeing that shoes and unrestricted arms aid with maintaining balance at landing. Finally, all participants had surgery with hamstring grafts which may restrict the generalisation of our results to ACL-reconstructed individuals with other grafts.
In summary, a submaximal single-leg lateral-to-medial side-hop landing induced greater frontal and transversal plane angles and moments with presumed risk to ACL injury than maximal single-leg forward and vertical hop landings, among ACL-reconstructed individuals with a minimum of 10 months between surgery and testing. These included greater FHA inclination angles for the first 10° of helical knee motion from initial contact (indicative of less robust knees when most clinically important), larger knee abduction angles both at initial contact and during landing, and greater external moments of peak abduction and internal rotation during landing. Between-leg asymmetry with lower external peak flexion moment for the ACL-reconstructed leg was shown for males during OLHD and OLVH, and for females during SRSH. When assessing knee landing control to screen and correct for movement patterns potentially related to ACL injury risk, the SRSH may be preferred to OLHD and OLVH. However, the clear differences in both knee kinematics and kinetics between particularly the OLHD and SRSH motivate the use of both these tests to evaluate different aspects of landing control.
Ethical approval
The Regional Ethical Review board in Umeå approved the study (reference: 2015/67-31).
Supplemental Material
Download PDF (88.3 KB)Acknowledgments
The authors are grateful to the participants of this study. We also acknowledge Jonas Selling (M.Eng.) for technical assistance, Ashokan Arumugam (PT, PhD) for clinical examination of some of the participants when stepping in for Eva Tengman (co-author), and Andrew Strong (PhD student) for help with the data collection.
Disclosure statement
No potential conflict of interest was reported by the author(s).
Supplementary material
Supplemental data for this article can be accessed here.
Additional information
Funding
References
- Alenezi, F., Herrington, L., Jones, P., & Jones, R. (2016). How reliable are lower limb biomechanical variables during running and cutting tasks. Journal of Electromyography and Kinesiology, 30, 137–142. https://doi.org/10.1016/j.jelekin.2016.07.001
- Amir Curcio, D. R., João Carlos, F. C., André Serra, B., Nayra Deise, D. A. R., Thiago Yukio, F., & Paulo Roberto, G. L. (2015). Kinematic and kinetic analysis of the single-leg triple hop test in women with and without patellofemoral pain. Journal of Orthopaedic & Sports Physical Therapy, 45(10), 799–807. https://doi.org/10.2519/jospt.2015.5011
- Ardern, C. L., Glasgow, P., Schneiders, A., Witvrouw, E., Clarsen, B., Cools, A., Gojanovic, B., Griffin, S., Khan, K. M., Moksnes, H., Mutch, S. A., Phillips, N., Reurink, G., Sadler, R., Grävare Silbernagel, K., Thorborg, K., Wangensteen, A., Wilk, K. E., & Bizzini, M. (2016). 2016 consensus statement on return to sport from the first world congress in sports physical therapy, bern. British Journal of Sports Medicine, 50(14), 853–864. https://doi.org/10.1136/bjsports-2016-096278
- Bates, N. A., Schilaty, N. D., Nagelli, C. V., Krych, A. J., & Hewett, T. E. (2019). Multiplanar loading of the knee and its influence on anterior cruciate ligament and medial collateral ligament strain during simulated landings and noncontact tears. The American Journal of Sports Medicine, 47(8), 1844–1853. https://doi.org/10.1177/0363546519850165
- Besier, T. F., Lloyd, D. G., Cochrane, J. L., & Ackland, T. R. (2001). External loading of the knee joint during running and cutting maneuvers. Medicine and Science in Sports and Exercise, 33(7), 1168–1175. https://doi.org/10.1097/00005768-200107000-00014
- Boden, B. P., Dean, G. S., Feagin, Jr, J. A., & Garrett, Jr, W. E. (2000). Mechanisms of anterior cruciate ligament injury. Orthopedics, 23(6), 573–578. https://doi.org/10.3928/0147-7447-20000601-15
- Cochrane, J. L., Lloyd, D. G., Buttfield, A., Seward, H., & McGivern, J. (2007). Characteristics of anterior cruciate ligament injuries in Australian football. Journal of Science and Medicine in Sport, 10(2), 96–104. https://doi.org/10.1016/j.jsams.2006.05.015
- Cole, G. K., Nigg, B. M., Ronsky, J. L., & Yeadon, M. R. (1993). Application of the joint coordinate system to three-dimensional joint attitude and movement representation: A standardization proposal. Journal of Biomechanical Engineering, 115(4a), 344–349. https://doi.org/10.1115/1.2895496
- Collins, T. D., Ghoussayni, S. N., Ewins, D. J., & Kent, J. A. (2009). A six degrees-of-freedom marker set for gait analysis: Repeatability and comparison with a modified Helen Hayes set. Gait & Posture, 30(2), 173–180. https://doi.org/10.1016/j.gaitpost.2009.04.004
- Critchley, M. L., Davis, D. J., Keener, M. M., Layer, J. S., Wilson, M. A., Zhu, Q., & Dai, B. (2020). The effects of mid-flight whole-body and trunk rotation on landing mechanics: Implications for anterior cruciate ligament injuries. Sports Biomechanics, 19(4), 421–437. https://doi.org/10.1080/14763141.2019.1595704
- Dai, B., Herman, D., Liu, H., Garrett, W. E., & Yu, B. (2012). Prevention of ACL injury, part I: Injury characteristics, risk factors, and loading mechanism. Research in Sports Medicine, 20(3–4), 180–197. https://doi.org/10.1080/15438627.2012.680990
- Dempster, W. T. (1955). Space requirements of the seated operator. In Wright-patterson air force base (pp. 55–159). U.S.A. WADC Technical Report.
- Deneweth, J. M., Bey, M. J., McLean, S. G., Lock, T. R., Kolowich, P. A., & Tashman, S. (2010). Tibiofemoral joint kinematics of the anterior cruciate ligament-reconstructed knee during a single-legged hop landing. The American Journal of Sports Medicine, 38(9), 1820–1828. https://doi.org/10.1177/0363546510365531
- Griffin, L. Y., Agel, J., Albohm, M. J., Arendt, E. A., Dick, R. W., Garrett, W. E., Garrick, J. G., Hewett, T. E., Huston, L., Ireland, M. L., Johnson, R. J., Kibler, W. B., Lephart, S., Lewis, J. L., Lindenfeld, T. N., Mandelbaum, B. R., Marchak, P., Teitz, C. C., & Wojtys, E. M. (2000). Noncontact anterior cruciate ligament injuries: Risk factors and prevention strategies. Journal of the American Academy of Orthopaedic Surgeons, 8(3), 141–150. https://doi.org/10.5435/00124635-200005000-00001
- Grip, H., & Häger, C. (2013). A new approach to measure functional stability of the knee based on changes in knee axis orientation. Journal of Biomechanics, 46(5), 855–862. https://doi.org/10.1016/j.jbiomech.2012.12.015
- Grip, H., Tengman, E., & Häger, C. K. (2015). Dynamic knee stability estimated by finite helical axis methods during functional performance approximately twenty years after anterior cruciate ligament injury. Journal of Biomechanics, 48(10), 1906–1914. https://doi.org/10.1016/j.jbiomech.2015.04.016
- Gustavsson, A., Neeter, C., Thomee, P., Grävare Silbernagel, K., Augustsson, J., Thomeé, R., & Karlsson, J. (2006). A test battery for evaluating hop performance in patients with an ACL injury and patients who have undergone ACL reconstruction. Knee Surgery, Sports Traumatology, Arthroscopy, 14(8), 778–788. https://doi.org/10.1007/s00167-006-0045-6
- Harrison, A. J., McErlain-Naylor, S. A., Bradshaw, E. J., Dai, B., Nunome, H., Hughes, G. T. G., Kong, P. W., Vanwanseele, B., Vilas-Boas, J. P., & Fong, D. T. P. (2020). Recommendations for statistical analysis involving null hypothesis significance testing. Sports Biomechanics, 19(5), 561–568. https://doi.org/10.1080/14763141.2020.1782555
- Hegedus, E. J., McDonough, S. M., Bleakley, C., Baxter, D., & Cook, C. E. (2015). Clinician-friendly lower extremity physical performance tests in athletes: A systematic review of measurement properties and correlation with injury. Part 2—the tests for the hip, thigh, foot and ankle including the star excursion balance test. British Journal of Sports Medicine, 49(10), 649–656. https://doi.org/10.1136/bjsports-2014-094341
- Hewett, T. E., Myer, G. D., Ford, K. R., Heidt, R. S., Colosimo, A. J., McLean, S. G., van den Bogert, A. J., Paterno, M. V., & Succop, P. (2005). Biomechanical measures of neuromuscular control and valgus loading of the knee predict anterior cruciate ligament injury risk in female athletes: A prospective study. The American Journal of Sports Medicine, 33(4), 492–501. https://doi.org/10.1177/0363546504269591
- Hewett, T. E., & Bates, N. A. (2017). Preventive biomechanics: A paradigm shift with a translational approach to injury prevention. The American Journal of Sports Medicine, 45(11), 2654–2664. https://doi.org/10.1177/0363546516686080
- Ishida, T., Koshino, Y., Yamanaka, M., Ueno, R., Taniguchi, S., Samukawa, M., Saito, H., Matsumoto, H., Aoki, Y., & Tohyama, H. (2018). The effects of a subsequent jump on the knee abduction angle during the early landing phase. BMC Musculoskeletal Disorders, 19(1), 379. https://doi.org/10.1186/s12891-018-2291-4
- Jamison, S. T., Pan, X., & Chaudhari, A. M. (2012). Knee moments during run-to-cut maneuvers are associated with lateral trunk positioning. Journal of Biomechanics, 45(11), 1881–1885. https://doi.org/10.1016/j.jbiomech.2012.05.031
- Johnston, J. T., Mandelbaum, B. R., Schub, D., Rodeo, S. A., Matava, M. J., Silvers-Granelli, H. J., Cole, B. J., ElAttrache, N. S., McAdams, T. R., & Brophy, R. H. (2018). video analysis of anterior cruciate ligament tears in professional american football athletes. The American Journal of Sports Medicine, 46(4), 862–868. https://doi.org/10.1177/0363546518756328
- Jones, P. A., Herrington, L. C., Munro, A. G., & Graham-Smith, P. (2014). Is there a relationship between landing, cutting, and pivoting tasks in terms of the characteristics of dynamic valgus? The American Journal of Sports Medicine, 42(9), 2095–2102. https://doi.org/10.1177/0363546514539446
- Kernozek, T., Torry, M., Shelburne, K., Durall, C. J., & Willson, J. (2006). From the gait laboratory to the rehabilitation clinic: Translation of motion analysis and modeling data to interventions that impact anterior cruciate ligament loads in gait and drop landing. Critical Reviews in Biomedical Engineering, 14(8), 243–258. https://doi.org/10.1615/CritRevBiomedEng.2014010676
- Kiapour, A. M., Demetropoulos, C. K., Kiapour, A., Quatman, C. E., Wordeman, S. C., Goel, V. K., & Hewett, T. E. (2015). Strain response of the anterior cruciate ligament to uniplanar and multiplanar loads during simulated landings: Implications for injury mechanism. The American Journal of Sports Medicine, 49(10), 2087–2096. https://doi.org/10.1177/0363546516640499
- Koga, H., Nakamae, A., Shima, Y., Iwasa, J., Myklebust, G., Engebretsen, L., Bahr, R., & Krosshaug, T. (2010). Mechanisms for noncontact anterior cruciate ligament injuries: Knee joint kinematics in 10 injury situations from female team handball and basketball. The American Journal of Sports Medicine, 38(11), 2218–2225. https://doi.org/10.1177/0363546510373570
- Kristianslund, E., & Krosshaug, T. (2013). Comparison of drop jumps and sport-specific sidestep cutting: Implications for anterior cruciate ligament injury risk screening. The American Journal of Sports Medicine, 41(3), 684–688. https://doi.org/10.1177/0363546512472043
- Krosshaug, T., Nakamae, A., Boden, B. P., Engebretsen, L., Smith, G., Slauterbeck, J. R., Hewett, T. E., & Bahr, R. (2007). Mechanisms of anterior cruciate ligament injury in basketball: Video analysis of 39 cases. The American Journal of Sports Medicine, 35(3), 359–367. https://doi.org/10.1177/0363546506293899
- Liu, K., & Heise, G. D. (2013). The effect of jump-landing directions on dynamic stability. Journal of Applied Biomechanics, 29(5), 634–638. https://doi.org/10.1123/jab.29.5.634
- Markström, J. L., Schelin, L., & Häger, C. K. (2018 December 10). A novel standardised side hop test reliably evaluates landing mechanics for anterior cruciate ligament reconstructed persons and controls. Sports Biomechanics. Epub ahead of print. https://doi.org/10.1080/14763141.2018.1538385
- Markström, J. L., Tengman, E., & Häger, C. K. (2018). ACL-reconstructed and ACL-deficient individuals show differentiated trunk, hip, and knee kinematics during vertical hops more than 20 years post-injury. Knee Surgery, Sports Traumatology, Arthroscopy, 26(2), 358–367. https://doi.org/10.1007/s00167-017-4528-4
- Markström, J. L., Grip, H., Schelin, L., & Häger, C. K. (2019a). Dynamic knee control and movement strategies in athletes and non-athletes in side hops: Implications for knee injury. Scandinavian Journal of Medicine & Science in Sports, 29(8), 1181–1189. https://doi.org/10.1111/sms.13432
- Markström, J. L., Grip, H., Schelin, L., & Häger, C. K. (2019b). Individuals with an anterior cruciate ligament–reconstructed knee display atypical whole body movement strategies but normal knee robustness during side-hop landings: A finite helical axis analysis. The American Journal of Sports Medicine, 47(8), 1117–1126. https://doi.org/10.1177/0363546520910428
- Nagelli, C. V., & Hewett, T. E. (2017). Should return to sport be delayed until 2 years after anterior cruciate ligament reconstruction? Biological and functional considerations. Sports Medicine, 47(2), 221–232. https://doi.org/10.1007/s40279-016-0584-z
- Olsen, O.-E., Myklebust, G., Engebretsen, L., & Bahr, R. (2004). Injury mechanisms for anterior cruciate ligament injuries in team handball: A systematic video analysis. The American Journal of Sports Medicine, 32(4), 1002–1012. https://doi.org/10.1177/0363546503261724
- Pappas, E., Hagins, M., Sheikhzadeh, A., Nordin, M., & Rose, D. (2007). Biomechanical differences between unilateral and bilateral landings from a jump: Gender differences. Clinical Journal of Sport Medicine, 17(4), 263–268. https://doi.org/10.1097/JSM.0b013e31811f415b
- Patterson, M. R., & Delahunt, E. (2013). A diagonal landing task to assess dynamic postural stability in ACL reconstructed females. The Knee, 20(6), 532–536. https://doi.org/10.1016/j.knee.2013.07.008
- Reeves, N. P., Narendra, K. S., & Cholewicki, J. (2007). Spine stability: The six blind men and the elephant. Clinical Biomechanics, 22(3), 266–274. https://doi.org/10.1016/j.clinbiomech.2006.11.011
- Renstrom, P., Ljungqvist, A., Arendt, E., Beynnon, B., Fukubayashi, T., Garrett, W., Georgoulis, T., Hewett, T. E., Johnson, R., Krosshaug, T., Mandelbaum, B., Micheli, L., Myklebust, G., Roos, E., Roos, H., Schamasch, P., Shultz, S., Werner, S., Wojtys, E., & Engebretsen, L. (2008). Non-contact ACL injuries in female athletes: An International Olympic Committee current concepts statement. British Journal of Sports Medicine, 42(6), 394–412. https://doi.org/10.1136/bjsm.2008.048934
- Saito, A., Okada, K., Sasaki, M., & Wakasa, M. (2020). Influence of the trunk position on knee kinematics during the single-leg landing: Implications for injury prevention. Sports Biomechanics.
- Sankey, S. P., Raja Azidin, R. M., Robinson, M. A., Malfait, B., Deschamps, K., Verschueren, S., Staes, F., & Vanrenterghem, J. (2015). How reliable are knee kinematics and kinetics during side-cutting manoeuvres? Gait & Posture, 41(4), 905–911. https://doi.org/10.1016/j.gaitpost.2015.03.014
- Schwartz, M. H., & Rozumalski, A. (2005). A new method for estimating joint parameters from motion data. Journal of Biomechanics, 38(1), 107–116. https://doi.org/10.1016/j.jbiomech.2004.03.009
- Sell, T. C., Ferris, C. M., Abt, J. P., Tsai, Y.-S., Myers, J. B., Fu, F. H., & Lephart, S. M. (2006). The effect of direction and reaction on the neuromuscular and biomechanical characteristics of the knee during tasks that simulate the noncontact anterior cruciate ligament injury mechanism. The American Journal of Sports Medicine, 34(1), 43–54. https://doi.org/10.1177/0363546505278696
- Stuelcken, M. C., Mellifont, D. B., Gorman, A. D., & Sayers, M. G. (2016). Mechanisms of anterior cruciate ligament injuries in elite women’s netball: A systematic video analysis. Journal of Sports Sciences, 34(16), 1516–1522. https://doi.org/10.1080/02640414.2015.1121285
- Taylor, J. B., Ford, K. R., Nguyen, A.-D., & Shultz, S. J. (2016). Biomechanical comparison of single- and double-leg jump landings in the sagittal and frontal plane. Orthopaedic Journal of Sports Medicine, 4(6), 232596711665515. https://doi.org/10.1177/2325967116655158
- Thomee, R., Kaplan, Y., Kvist, J., Myklebust, G., Risberg, M. A., Theisen, D., Tsepis, E., Werner, S., Wondrasch, B., & Witvrouw, E. (2011). Muscle strength and hop performance criteria prior to return to sports after ACL reconstruction. Knee Surgery, Sports Traumatology, Arthroscopy, 19(11), 1798–1805. https://doi.org/10.1007/s00167-011-1669-8
- Vacha-Haase, T., & Thompson, B. (2004). How to estimate and interpret various effect sizes. Journal of Counseling Psychology, 51(4), 473–481. https://doi.org/10.1037/0022-0167.51.4.473
- Walden, M., Krosshaug, T., Bjorneboe, J., Andersen, T. E., Faul, O., & Hagglund, M. (2015). Three distinct mechanisms predominate in non-contact anterior cruciate ligament injuries in male professional football players: A systematic video analysis of 39 cases. British Journal of Sports Medicine, 49(22), 1452–1460. https://doi.org/10.1136/bjsports-2014-094573
- Wiggins, A. J., Grandhi, R. K., Schneider, D. K., Stanfield, D., Webster, K. E., & Myer, G. D. (2016). Risk of secondary injury in younger athletes after anterior cruciate ligament reconstruction: A systematic review and meta-analysis. The American Journal of Sports Medicine, 44(7), 1861–1876. https://doi.org/10.1177/0363546515621554
- Wikstrom, E. A., Tillman, M. D., Schenker, S. M., & Borsa, P. A. (2008). Jump-landing direction influences dynamic postural stability scores. Journal of Science and Medicine in Sport, 11(2), 106–111. https://doi.org/10.1016/j.jsams.2007.02.014
- Zaslow, T. L., Pace, J. L., Mueske, N. M., Chua, M. C., Katzel, M. J., Dennis, S. W., & Wren, T. A. L. (2016). Comparison of lateral shuffle and side-step cutting in young recreational athletes. Gait & Posture, 44, 189–193. https://doi.org/10.1016/j.gaitpost.2015.12.019