Abstract
Objective. Caspase-1 is a component of the NALP3 inflammasome, a cytosolic multiprotein complex that mediates the processing of pro-inflammatory caspases and cytokines. The inflammasome represents the first line of defense against cellular stress and is a crucial component of innate immunity. Caspase-1 is the enzyme responsible for the cleavage and activation of interleukin (IL)-1β, which is a potent pro-inflammatory cytokine, and plays a central role in the mechanisms leading to labor (preterm and term) particularly in the context of intrauterine infection/inflammation. In addition, caspase-1 cleaves IL-18 and IL-33. The objectives of this study were to determine whether there is a relationship between amniotic fluid concentrations of caspase-1 and gestational age, parturition (term and preterm), and intra-amniotic infection/inflammation (IAI).
Study design. A cross-sectional study was conducted including 143 pregnant women in the following groups: (1) mid-trimester of pregnancy (n = 18); (2) term not in labor (n = 25); (3) term in labor (n = 28); (4) preterm labor (PTL) who delivered at term (n = 23); (5) PTL without IAI who delivered preterm (n = 32); (6) PTL with IAI who delivered preterm neonates (n = 17). Caspase-1 concentrations in amniotic fluid were determined by a specific and sensitive immunoassay. Non-parametric statistics were used for analysis.
Results. (1) Caspase-1 was detected in amniotic fluid of women at term, but in none of the mid-trimester samples. (2) Patients in labor at term had a significantly higher median amniotic fluid concentration of caspase-1 than women at term not in labor (term in labor: 10.5 pg/mL, range 0.0–666.0 vs. term not in labor: 5.99 pg/mL, range 0.0–237.4; p < 0.05). (3) Among patients with spontaneous PTL, those with IAI (median 41.4 pg/mL, range 0.0–515.0) had a significantly higher median amniotic fluid caspase-1 concentration than those without IAI who delivered preterm (median 0.0 pg/mL, range 0.0–78.4) and than those who delivered at term (median 0.0 pg/mL, range 0.0–199.5); p < 0.001 for both comparisons.
Conclusions. (1) The presence and concentration of caspase-1 in the amniotic fluid varies as a function of gestational age. (2) Women with spontaneous labor at term had a higher median caspase-1 amniotic fluid concentration than women at term without labor. This suggests that the inflammasome may be activated in spontaneous parturition at term. Since most women with labor do not have intra-amniotic infection, we propose that cellular stress during labor accounts for activation of the inflammasome. (3) Preterm labor associated with infection/inflammation was also associated with a high concentration of caspase-1, suggesting that infection may induce caspase-1 production and activation of the inflammasome. (4) The sequential activation of the inflammasome and caspase-1, leading to interleukin-1β processing and secretion, is a candidate pathway leading to the activation of the common pathway of parturition.
Introduction
Caspase-1 Citation[1],Citation[2], also known as interleukin-1β converting enzyme (ICE) Citation[3],Citation[4], is a cysteine-aspartic proteaseCitation[5], promoting the processing, secretion, and release of pro-inflammatory cytokines, including interleukin (IL)-1β, IL-18, and IL-33 Citation[6-8]. Caspase-1 is the prototype of the inflammatory caspases, and a key component of the inflammasome Citation[8-10], which has been recently defined as a first line of the immune response to cell stress Citation[10], as well as a ‘master switch of inflammation’Citation[11]. The inflammasome, indeed, is an inducible multiprotein cytosolic complex Citation[8], assembling upon recognition of ‘danger signals’ by intracellular sensors, including the NOD-like receptors Citation[12], or pathogen-associated compounds Citation[10]. The inflammasome, in turn, activates inflammatory caspases Citation[8], of which caspase-1 is the prototype, and promotes the processing, secretion, and release of pro-inflammatory cytokines, including IL-1β, IL-18, and IL-33 Citation[6-8],Citation[10].
Term and preterm labor are inflammatory processes Citation[13-21], in which inflammatory mediators including cytokines such as IL-1 Citation[22-26], IL-6 Citation[22],Citation[27-30], tumor necrosis factor Citation[27],Citation[31-36], IL-18 Citation[37], IL-16 Citation[38], and chemokines Citation[39-45] play a central role. Of note, labor is associated with increased concentrations of IL-1β in both gestational tissues Citation[46-48] and biological fluids Citation[13],Citation[49], and intra-amniotic and/or systemic administration of IL-1β to mice and monkeys induces preterm labor Citation[24],Citation[50],Citation[51].
Considering the pivotal role of caspase-1 as a link between the activation of the inflammasome and the processing of IL-1β, we conducted this study to determine whether there is a relationship between amniotic fluid concentrations of caspase-1 and gestational age, parturition (term and preterm), and intra-amniotic infection/inflammation (IAI).
Materials and methods
Study design
This cross-sectional study was designed to examine the relationship among amniotic fluid concentrations of caspase-1 and gestational age, spontaneous labor at term, preterm parturition, and IAI.
Amniotic fluid was collected by transabdominal amniocentesis from 143 singleton pregnancies in the following groups: (1) Patients undergoing mid-trimester amniocentesis for clinical indications who delivered at term (n = 18); (2) women at term not in labor (n = 25); (3) patients at term in labor (n = 28); (4) women with preterm labor (PTL) and intact membranes who delivered at term (n = 23); (5) women in PTL without IAI who delivered preterm (n = 32); (6) women with PTL and IAI who delivered preterm (n = 17).
Clinical definitions
Patients were considered to have a normal pregnancy if they did not have an obstetrical, medical, or surgical complication of pregnancy and delivered a term (≥37 weeks) neonate. PTL was defined in the presence of regular uterine contractions occurring at a frequency of at least two every 10 minutes, combined with documented cervical changes prior to 37 weeks of gestation. Intra-amniotic infection was defined by a positive amniotic fluid culture for microorganisms. Intra-amniotic inflammation was defined in the presence of a white blood cell (WBC) count of > 100 cells/mm3.
Amniotic fluid sample collection and analysis
Amniotic fluid was collected by transabdominal amniocentesis. Fluid not required for clinical purposes was centrifuged to remove cellular and particulate matter and aliquots stored at −70°C until analysis. Amniotic fluid WBC count, Gram stain, and glucose concentrations were used in the management of patients with preterm labor. Amniotic fluid samples from the term and preterm groups were cultured for aerobic and anaerobic species and genital mycoplasmas (Ureaplasma urealyticum and Mycoplasma hominis). Pregnant patients were enrolled at Hutzel Hospital, Detroit, MI, USA, Pennsylvania Hospital, Philadelphia, Pennsylvania, USA, and Sotero del Rio Hospital, Puente Alto, Chile. The collection of samples for research was approved by the institutional review boards of Wayne State University, Pennsylvania Hospital, and Sotero del Rio Hospital, as well as the Eunice Kennedy Shriver National Institute of Child Health and Human Development, National Institutes of Health, Department of Health and Human Services (NIH/DHHS). All participants provided written informed consent for collection of clinical data and biological materials. Many of these samples have been previously employed to study the biology of inflammation, hemostasis, angiogenesis regulation, and growth factor concentrations in non-pregnant women, normal pregnant women, and those with complications.
Human caspase-1 immunoassays in amniotic fluid
A specific and sensitive immunoassay was used to determine the concentration of caspase-1 in amniotic fluid samples. Human caspase-1 immunoassay kits (Cistron Biotechnology, Pine Brook, NJ, USA) were validated for human amniotic fluid in our laboratory prior to the conduction of this study. Validation included spike and recovery experiments, which produced parallel curves indicating that amniotic fluid constituents did not interfere with antigen–antibody binding in this assay system. The inter-assay and intra-assay coefficients of variation for the caspase-1 immunoassay were 7.9% and 4.7%, respectively. The sensitivity was 3.54 pg/mL.
Statistical analysis
Data were tested for normality using the Kolmogorov–Smirnov test. Kruskal–Wallis was used for analysis of variance. Comparisons between two groups were performed using Mann–Whitney rank sum tests. A p value < 0.05 was considered statistically significant. We used SPSS v.14.0 (SPSS Inc., Chicago, IL, USA) for analysis.
Results
One hundred and forty-three patients were included in this study. The demographic and clinical characteristics of patients with PTL are shown in .
Table I. Demographic and clinical characteristics of patients with preterm labor.
Caspase-1 in amniotic fluid
Caspase-1 was detectable in 56.6% (81/143) of the amniotic fluid samples. Of interest, caspase-1 was detected in 76% (19/25) of women at term not in labor, but in none of the mid-trimester samples.
Spontaneous labor at term is associated with higher amniotic fluid concentrations of caspase-1
The median amniotic fluid caspase-1 concentration was significantly higher in women in spontaneous labor at term than in those not in labor at term (term in labor: median 10.5 pg/mL, range 0.0–666 vs. term not in labor: median 5.99 pg/mL, range 0.0–237.4; p < 0.05; ).
Figure 1. Amniotic fluid concentrations of caspase-1 in patients at term. The median amniotic fluid caspase-1 concentration was significantly higher in women in spontaneous labor at term than in those not in labor (term in labor: median 10.5 pg/mL, range 0.0–666.0 vs. term not in labor: median 5.99 pg/mL, range 0.0–237.4; p < 0.05).
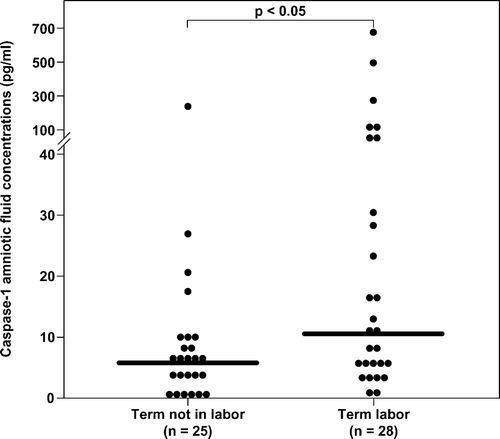
Caspase-1 is increased in the amniotic fluid of women with preterm labor in the presence of intra-amniotic infection/inflammation
Among patients with spontaneous PTL, those with IAI (median 41.4 pg/mL, range 0.0–515) had a significantly higher median amniotic fluid caspase-1 concentration than those without IAI who delivered preterm (median 0.0 pg/mL, range 0.0–78.4) and than those who delivered at term (median 0.0 pg/mL, range 0.0–199.5); p < 0.001 for both comparisons; ().
Figure 2. Amniotic fluid concentrations of caspase-1 in women with preterm labor. Among patients with spontaneous PTL, those with intra-amniotic infection/inflammation (median 41.4 pg/mL, range 0.0–515) had a significantly higher median amniotic fluid caspase-1 concentration than those without intra-amniotic infection/inflammation who delivered preterm (median 0.0 pg/mL, range 0.0–78.4) and than those who delivered at term (median 0.0 pg/mL, range 0.0–199.5); p < 0.001 for both comparisons.
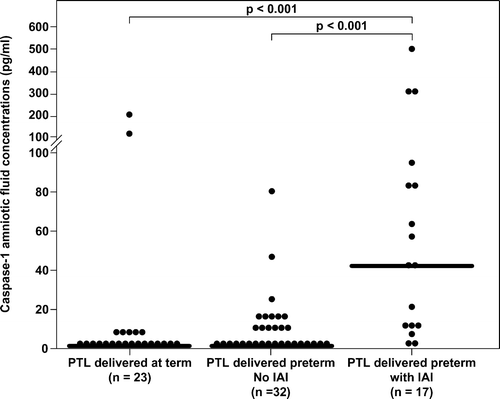
Discussion
Principal findings of the study
(1) Caspase-1 is detectable in amniotic fluid at term but not in the midtrimester. (2) Women in spontaneous labor at term have a higher median amniotic fluid concentration of caspase-1 than patients at term not in labor. (3) Among patients with preterm labor, those with IAI had a significantly higher median amniotic fluid caspase-1 concentration than those without IAI. These observations suggest that caspase-1 participates in the mechanisms leading to spontaneous term parturition and preterm labor associated with infection/inflammation.
Biology of caspase-1
Caspase-1 Citation[1],Citation[2], also known as interleukin-1β converting enzyme (ICE) Citation[3],Citation[4], is a member of the cysteine-aspartic proteases family (‘c-asp-ases’) Citation[5], enzymes involved in inflammation, apoptosis, cellular proliferation, and terminal differentiation Citation[52]. Caspases have been classified as ‘inflammatory’ (caspase-1, -4, and -5 and murine caspase-11 and -12) and ‘apoptotic’ (‘initiators’: caspase-2, -8, -9, and -10 and ‘executors’: caspase-3, -6, and -7) Citation[7],Citation[53], according to their predominant pathway of involvement. However, this distinction is not absolute Citation[54], and differences between the in vivo and in vitro activity of these enzymes have been proposed Citation[7]. Caspases process several proteins that are critical to major changes in cellular state, including nuclear proteins, transcription factors, cell cycle regulators, and kinases, as well as cytoskeletal proteins Citation[52]. Caspase-1 is the prototype of the inflammatory caspases, and a key component of the inflammasome Citation[8],Citation[11]. Its central role in inflammation and the potentially harmful consequences of an inappropriate and/or unbalanced activation, justify the existence of tight regulatory mechanisms operating both before and after its synthesis Citation[55]. Despite the existence of alternative pathways Citation[56],Citation[57], the major pathway leading to caspase-1 activation requires the assembly of the inflammasome Citation[8], and the active form of caspase-1 has only been detected in extracts of IL-1β producing cells Citation[58]. With high-resolution immunoelectron microscopy performed on lipopolysaccharide (LPS)-stimulated human monocytes, both the active and the precursor domains of caspase-1 have been localized in the cytoplasmatic matrix and on the cell surface Citation[59]. Of interest, only small amounts of the active form of caspase-1 are detectable in the intracellular milieu, even when IL-1β actively producing monocytes are tested Citation[60],Citation[61]. It has been proposed that the caspase-1 mediated processing of IL-1β occurs during the transport of this cytokine across the plasma membrane, followed by rapid secretion in the extracellular milieu Citation[59].
Caspase-1 is initially synthesized as a 45-kDa catalytically inactive zymogen (pro-caspase) Citation[62]. Meager amounts of this inactive precursor protein are constitutively produced by monocytic cells Citation[60], and mainly localized in the cytoplasmatic compartment Citation[59]. The active form of caspase-1 is heterodimeric in structure, and composed by the association of two polypeptides, p20 and p10 Citation[2],Citation[3],Citation[55],Citation[63],Citation[64].
Monocytes constitutively express very low levels of caspase-1 mRNA Citation[60], and its transcripts are also detected in other cells of the immune system, including T and B lymphocytes, and cells of monocytic origin, such as microglia Citation[65]. The transcription of caspase-1 gene, which maps on chromosome 11 Citation[66], is increased upon LPS and interferon (IFN)-γ stimulation; however, there is no evidence that higher intracellular mRNA levels result in increased pro-enzyme production Citation[67],Citation[68]. In addition, there is recent evidence that leptin induces mRNA expression of caspase-1 in monocytes/macrophages Citation[69], and that also the pro-inflammatory response mediated by statins in mitogen-activated peripheral blood mononuclear cells is mediated by caspase-1 activation Citation[70].
Caspase-1 processes and activates pro-IL-1β
IL-1β is a pro-inflammatory cytokine whose inactive precursor, pro-IL-1β, is localized in the cytoplasm of monocytes Citation[71]. Caspase-1 Citation[1],Citation[3-5],Citation[72] catalyzes the proteolytic cleavage of pro-IL-1β, between Asp116 and Ala117, which is necessary for functional activity Citation[73],Citation[74]. Maturation and secretion of IL-1β occur in a coordinated fashion, with a unique kinetic Citation[73]. Indeed, caspase-1 participates in both IL-1β processing and secretion Citation[58],Citation[59],Citation[75]. Evidence in support of a role of caspase-1 in IL-1β cleavage and activation includes: (1) production of IL-1β and IL-18 is concordantly blocked in caspase-1 null mice Citation[76],Citation[77] and (2) a selective caspase-1 inhibitor blocks the LPS-induced IL-1β production from human mononuclear cells Citation[78]. The critical role of IL-1β in the control of infections, and the harmful and potentially lethal consequences of an excessive cytokine production and release (i.e., endotoxic shock) Citation[79], deserves the existence of tight regulatory mechanisms controlling its transcription, processing, and secretion, as well as the presence of competitive inhibitors of its signaling Citation[80],Citation[81].
IL-1β is a potent pleiotropic pro-inflammatory cytokine, which participates in the activation of nuclear factor-kB (NF-kB) and mitogen-activated protein kinase (MAPK) pathways Citation[82], and is involved in the amplification of the inflammatory cascade Citation[53]. Evidence has been accumulating in the past years on the role of IL-1β in the mechanisms leading to implantation Citation[83],Citation[84] and term Citation[13],Citation[46],Citation[47],Citation[49] and preterm labor Citation[23],Citation[50].
Caspase-1 processes IL-18
IL-18, also known as IFN-γ-inducing factor, is a recently described cytokine sharing structural homology with the IL-1 family of proteins and functional properties with IL-12 Citation[85-87]. IL-18, like IL-1β, is synthesized as a 24-kDa inactive precursor lacking a signal peptide (pro-IL-18), which is cleaved after Asp35 by caspase-1 to yield an active 8-kD molecule Citation[78],Citation[88]. Evidence in support of a role of caspase-1 in IL-18 cleavage and activation includes: (1) caspase-1 deficient mice are defective in LPS-induced IL-18 production Citation[78]; (2) a selective caspase-1 inhibitor blocks LPS-induced IL-18 production from human peripheral blood mononuclear cells Citation[78]; (3) Kupffer cells deficient in caspase-1, upon LPS stimulation synthesize the IL-18 precursor but fail to process it into its active form Citation[88]; (4) caspase-1 inhibitors abolish the secretion of mature IL-18 by human granulocyte macrophages infected with influenza or Sendai virus Citation[89]. However, caspase-1 cleavage of pro-IL-18 is not exclusive and other proteases, such as proteinase-3, have been recently reported to generate biological activity from IL-18 Citation[90].
IL-18 is an activator of the T helper (Th)-1 response Citation[91], mainly through the induction of IFN-γ production in both T cells and natural killer (NK) cells Citation[86]. IL-18 enhances T and NK cells maturation and activation Citation[92], cytokine production Citation[93],Citation[94], and cytotoxicity Citation[85],Citation[95-99], as well as neutrophil activation, reactive oxygen intermediate synthesis, cytokine release, and degranulation Citation[100,101]. IL-18 is involved in the immune defense against bacteria (Chlamydias Citation[102,103], Mycoplasmas Citation[104-106], Mycobacterium tuberculosisCitation[107-109] and Mycobacterium lepraeCitation[110], Salmonellas Citation[111], Shigella Citation[112], and Yersinia Citation[113]), fungi Citation[114-116], protozoa Citation[117,118] and viruses Citation[89],Citation[119]. Of note, the roles of IL-18 in implantation Citation[120-123] and in the context of microbial invasion of the amniotic fluid Citation[124], intra-amniotic inflammation Citation[124], and preterm delivery Citation[124,125] have been subject of research.
Amniotic fluid caspase-1 in normal pregnancy
This is the first study reporting that caspase-1 is detectable in the amniotic fluid of normal pregnant women. The sources of the intra-amniotic caspase-1 are still unclear. Caspase-1 may participate in the processing and activation of IL-1β and IL-18, which take place at the maternal–fetal interface, where these cytokines play an autocrine–paracrine role for successful human implantation, pregnancy maintenance Citation[84],Citation[120-122],Citation[126], and labor Citation[13],Citation[46-49],Citation[127].
Sources of IL-1β during pregnancy include cyto-, syncytio-, and intermediate trophoblasts Citation[47],Citation[84],Citation[128], macrophages (Hofbauer cells) Citation[47], amniochorionic membranes Citation[129], and decidual stromal Citation[84],Citation[128] and NK cells Citation[128]. IL-1β regulates the activity of the matrix metalloproteinase (MMP)- 9, which has been linked to cytotrophoblast invasion Citation[83].
IL-18 (both gene and protein) is constitutively expressed in human chorion and decidua, but not in the amniotic epithelium Citation[130]. Evidence supports a role for IL-18 in modulating uterine NK cells, including their cytokine production and cytolytic activity, as well as in local vascular remodeling, through the induction of IFN-γ production Citation[123].
Our finding that caspase-1 was not detectable in any of the mid-trimester samples in contrast to 76% of amniotic fluid from women at term not in labor is unexpected. Indeed, previous studies have reported that placental IL-1β release, in which caspase-1 may play a role, is high in the first trimester and declines as term gestation approaches, reflecting the invasiveness of the cytotrophoblasts Citation[83]. There are several explanations for this result. First, recent gene array studies have elucidated that during decidualization there is an up-regulation of the gene, mRNA, and protein expression of IL-1β, but this is not accompanied by a coincidental increase in the secretion of the bioactive form of this cytokine Citation[131], a step requiring caspase-1. Second, it is possible that sources other than cytotrophoblast contribute to the amniotic fluid concentrations of caspase-1. Desquamated fetal epithelia (urinary tract, gastrointestinal, or other cells found in normal amniotic fluid) have been proposed to be the major contributors to the amniotic fluid IL-1 Citation[49]. If this is true, fetal epithelial cells may contribute to the pool of caspase-1 present in amniotic fluid in greater amounts as term approaches. Third, the detection of caspase-1 in the amniotic fluid at term, but not in mid-gestation, is in agreement with the previous report of cell-associated IL-1 activity in the amniotic fluid collected in the third but not in the second trimester Citation[49], as well as with the presence of higher IL-18 amniotic fluid concentrations as term approaches Citation[37].
Amniotic fluid caspase-1 in spontaneous labor at term
Women in spontaneous labor at term had a higher median amniotic fluid concentration of caspase-1 than patients at term not in labor. This finding is consistent with the view that labor at term is an event characterized by activation of the inflammatory cascade Citation[18],Citation[20],Citation[132]. The pathways leading to the activation of the inflammasome in the context of labor require further investigation. Of note, the higher concentrations of caspase-1 during labor at term mirror the increase in IL-1β concentrations reported in gestational tissues (placenta Citation[47], chorion Citation[48], amniotic membranes Citation[48], decidua Citation[46],Citation[48]) and biological fluids (amniotic fluid Citation[13],Citation[22],Citation[49]), as well as the higher maternal serum Citation[127] and amniotic fluid concentrations Citation[37] of IL-18, when compared to normal term controls. In turn, IL-1β stimulates myometrial contractility by several mechanisms, including: (1) induction of prostaglandin (PG) synthesis (PGE2 Citation[133-135], PGI2 Citation[134], and PGF2 Citation[136]) in myometrial cells Citation[133,134], amnion Citation[135,136], and choriodecidua Citation[136]; (2) activation of the transcription factor NF-kB, which is functionally linked to the expression of cyclo-oxygenase 2 messenger ribonucleic acid, protein, and PG synthesis Citation[137]; (3) potentiation of the forskolin-promoted cAMP (cyclic-adenosine monophosphate) production, resulting in cAMP accumulation Citation[134].
Amniotic fluid caspase-1 in preterm labor
Among patients with preterm labor, those with IAI had a significantly higher median amniotic fluid caspase-1 concentration than those without IAI. This finding is in agreement with previous reports supporting a role for two of the cytokines processed by caspase-1, IL-1β and IL-18, in the molecular mechanisms leading to the onset of preterm parturition Citation[23],Citation[37],Citation[49],Citation[50],Citation[124],Citation[125].
Evidence of the involvement of IL-1 in preterm labor
A solid body of evidence suggests that IL-1β is implicated in the mechanisms leading to preterm parturition, particularly in the context of IAI. There is, indeed, an epidemiologic/causal link between the presence of intra-amniotic infection, higher activity and/or concentrations of IL-1β in biological fluids, and higher concentrations of prostaglandins, as supported by the following observations: (1) Our group previously reported that the systemic administration (by subcutaneous injections) of recombinant human IL-1 to timed-pregnant C3H/HeJ inbred mice was followed by parturition within 24 hours in all of the treated mice, and that a premature delivery occurred in all IL-1-injected animals Citation[50]. (2) An immunoradiometric assay, validated for amniotic fluid and with a sensitivity of 50 pg/mL, was capable of detecting IL-1β in the amniotic fluid of all (15/15) pregnant women with preterm labor and intra-amniotic infection, versus 47.3% (10/21) of women in preterm labor, negative amniotic fluid cultures, and delivering prematurely, versus 47.2% (17/36) of samples from women with negative amniotic fluid cultures responding to tocolysis. All patients with histopathological signs of chorioamnionitis had detectable IL-1β in the amniotic fluid. Of interest, both IL-1α and IL-1β were significantly higher in women with microbial invasion of the amniotic cavity than in women who delivered prematurely but had negative amniotic fluid cultures (p = 0.0001 and p = 0.0006, respectively) Citation[23]. (3) Patients in preterm labor with intra-amniotic infection had a fluid phase IL-1β activity that strongly correlated with the PGE2 and PGF2α amniotic fluid concentrations Citation[49]. An increase in the fluid phase IL-1 activity is detectable also in amniotic fluid from women with spontaneous labor at term, but in this context it is predominantly attributable to IL-1α and not to IL-1βCitation[49], and only modest elevation in the amniotic fluid concentrations of prostaglandins and their metabolites Citation[138],Citation[139].
In summary, a microbial colonization of the amniotic cavity and the subsequent intrauterine inflammation may lead to an increase in local production of IL-1β, followed by PG-mediated myometrial contractility. The fetus, the mother, and the placenta may contribute to the higher amniotic fluid concentrations of IL-1β and caspase-1 in the context of preterm labor. A transmembranous passage of IL-1 has been demonstrated in our laboratory (unpublished observation). In addition, experimental evidence indicates that supernatants from endotoxin-stimulated human decidua contain a significantly higher IL-1 bioactivity (attributable both to IL-1α and IL-1β) than supernatants from unstimulated decidua Citation[140]. Furthermore, production of IL-1 from placentas of women in labor is 17-fold higher in the presence of chorioamnionitis Citation[47].
Evidence of the involvement of IL-18 in preterm labor
Previous investigations on the role of IL-18 in the context of preterm labor have yielded conflicting results. We have previously reported that: (1) both term and preterm parturition are associated with a modest increase in amniotic fluid IL-18 concentrations, although this trend did not reach statistical significance, and that (2) the microbial invasion of the amniotic cavity, in either preterm or term parturition, is associated with a significant increase in the amniotic fluid concentration of IL-18 Citation[37]. These findings are in agreement with another previous study in which it was reported that: (1) the median concentrations of IL-18 in cervical mucus and amniotic fluid are higher in women with preterm labor than in women not in labor at term Citation[124]. (2) Among women with preterm labor, the median amniotic fluid concentrations of IL-18 are significantly higher in the presence of microbial invasion of the amniotic cavity and correlate with the indices of intra-amniotic inflammation (defined as increased levels of IL-6 (≥1.5 ng/mL) and/or IL-8 (≥1.3 ng/mL)) Citation[124]. (3) Women with preterm labor and prompt delivery (delivery within seven days or <34 weeks of gestation) have significantly higher amniotic fluid concentrations of IL-18 than those delivering after several days or > 34 weeks Citation[124]. Of note, the IL-18 gene is constitutively expressed (RT-PCR, in situ hybridization, and immunohistochemistry) in human chorion and decidua, whereas the amniotic epithelium is devoid of IL-18 mRNA and protein Citation[130]. However, whether a higher expression of IL-18 is associated with preterm labor is not clear yet, as mice lacking IL-18 (by disruption of the IL-18 gene and/or IL-18 neutralization with intraperitoneal administration of a specific inhibitor of IL-18) have been shown to be more sensitive to LPS-induced preterm delivery and preterm fetal losses than the wild-type counterparts Citation[125]. The role of IL-18 as a negative regulator of TNF-α during sepsis (LPS challenge induces higher serum TNF-α concentrations in the IL-18 −/− mice than in the wild type) Citation[141], may contribute to this unexpected finding Citation[125].
Caspase-1 inhibitors: Implications for further research
Mice and rats deficient in caspase-1 (following administration of a caspase-1 inhibitor or gene deletion) display deficient maturation of pro-IL-1βCitation[77],Citation[142-144] and pro-IL-18 Citation[142,143]. Of interest, there is evidence that these animals, which are three- to four-fold more sensitive to bacterial infection than wild-type controls Citation[145], have significantly lower serum concentrations of TNF-αCitation[144], are resistant to the lethal effects of endotoxins Citation[77], have a longer survival than controls during experimental pancreatitis Citation[144], and are less likely to die from Escherichia coli intraperitoneal injection-induced sepsis Citation[146]. Thus, administration of caspase-1 inhibitors has been proposed as a therapeutic strategy in the treatment of local and systemic inflammatory processes, particularly in the context of sepsis Citation[142],Citation[147]. Caspase-1 inhibitors are the first orally active agents targeting cytokines, preventing the processing and release of the active forms of IL-1β and IL-18 Citation[142]. A blockage of lymphocyte apoptosis Citation[146], an event associated with the development of sepsis Citation[148-150], has been proposed as a further mechanism of action of caspase inhibitors, when tested in a model of cecal ligation and puncture Citation[147].
Of note, it has been proposed that caspase-1 inhibitors may be beneficial in the context of inflammation-related neurological damage. Administration of caspase-1 inhibitors to neonatally infected (E. coli) rats followed by subsequent LPS challenge prevented the expected LPS-induced memory impairment. These data suggest that IL-1β participates in the set of immune/inflammatory events resulting from neonatal infection and which likely contribute to cognitive alterations Citation[151]. In addition, caspase-1 inhibitors have been shown to reduce apoptosis, increase proliferation, and enhance survival of transplanted stem cells Citation[152]. This has considerable implications for the treatment of infection/inflammation-induced perinatal brain injury.
This study focuses on the immunoreactive caspase-1 concentrations in amniotic fluid. Further studies investigating the functional or biological activity of caspase-1 in the same milieu or in other maternal/fetal biological fluids, will contribute to clarifying the role of this enzyme in normal pregnancy, as well as in term and preterm parturition.
Acknowledgment
This research was supported by the Intramural Research Program of the Eunice Kennedy Shriver National Institute of Child Health and Human Development, NIH, DHHS.
Declaration of interest: The authors report no conflicts of interest. The authors alone are responsible for the content and writing of the paper.
References
- Cerretti D P, Kozlosky C J, Mosley B, Nelson N, Van Ness K, Greenstreet T A, March C J, Kronheim S R, Druck T, Cannizzaro L A, et al. Molecular cloning of the interleukin-1 beta converting enzyme. Science 1992; 256: 97–100
- Miller D K, Ayala J M, Egger L A, Raju S M, Yamin T T, Ding G J, Gaffney E P, Howard A D, Palyha O C, Rolando A M, et al. Purification and characterization of active human interleukin-1 beta-converting enzyme from THP.1 monocytic cells. J Biol Chem 1993; 268: 18062–18069
- Thornberry N A, Bull H G, Calaycay J R, Chapman K T, Howard A D, Kostura M J, Miller D K, Molineaux S M, Weidner J R, Aunins J, et al. A novel heterodimeric cysteine protease is required for interleukin-1 beta processing in monocytes. Nature 1992; 356: 768–774
- Kostura M J, Tocci M J, Limjuco G, Chin J, Cameron P, Hillman A G, Chartrain N A, Schmidt J A. Identification of a monocyte specific pre-interleukin 1 beta convertase activity. Proc Natl Acad Sci U S A 1989; 86: 5227–5231
- Alnemri E S, Livingston D J, Nicholson D W, Salvesen G, Thornberry N A, Wong W W, Yuan J. Human ICE/CED-3 protease nomenclature. Cell 1996; 87: 171
- Mariathasan S, Newton K, Monack D M, Vucic D, French D M, Lee W P, Roose-Girma M, Erickson S, Dixit V M. Differential activation of the inflammasome by caspase-1 adaptors ASC and Ipaf. Nature 2004; 430: 213–218
- Matute-Bello G. Targeting caspase-1 in sepsis: A novel approach to an old problem. Intensive Care Med 2007; 33: 755–757
- Martinon F, Burns K, Tschopp J. The inflammasome: A molecular platform triggering activation of inflammatory caspases and processing of proIL-beta. Mol Cell 2002; 10: 417–426
- Petrilli V, Dostert C, Muruve D A, Tschopp J. The inflammasome: A danger sensing complex triggering innate immunity. Curr Opin Immunol 2007; 19: 615–622
- Ogura Y, Sutterwala F S, Flavell R A. The inflammasome: First line of the immune response to cell stress. Cell 2006; 126: 659–662
- Martinon F, Tschopp J. Inflammatory caspases and inflammasomes: Master switches of inflammation. Cell Death Differ 2007; 14: 10–22
- Meylan E, Tschopp J, Karin M. Intracellular pattern recognition receptors in the host response. Nature 2006; 442: 39–44
- Romero R, Parvizi S T, Oyarzun E, Mazor M, Wu Y K, Avila C, Athanassiadis A P, Mitchell M D. Amniotic fluid interleukin-1 in spontaneous labor at term. J Reprod Med 1990; 35: 235–238
- Dudley D J. Pre-term labor: An intra-uterine inflammatory response syndrome?. J Reprod Immunol 1997; 36: 93–109
- Hagberg H, Mallard C, Jacobsson B. Role of cytokines in preterm labour and brain injury. BJOG 2005; 112(Suppl 1)16–18
- Chan E C, Fraser S, Yin S, Yeo G, Kwek K, Fairclough R J, Smith R. Human myometrial genes are differentially expressed in labor: A suppression subtractive hybridization study. J Clin Endocrinol Metab 2002; 87: 2435–2441
- Bisits A M, Smith R, Mesiano S, Yeo G, Kwek K, MacIntyre D, Chan E C. Inflammatory aetiology of human myometrial activation tested using directed graphs. PLoS Comput Biol 2005; 1: 132–136
- Romero R, Espinoza J, Goncalves L F, Kusanovic J P, Friel L A, Nien J K. Inflammation in preterm and term labour and delivery. Semin Fetal Neonatal Med 2006; 11: 317–326
- Haddad R, Tromp G, Kuivaniemi H, Chaiworapongsa T, Kim Y M, Mazor M, Romero R. Human spontaneous labor without histologic chorioamnionitis is characterized by an acute inflammation gene expression signature. Am J Obstet Gynecol 2006; 195: 394.e1–24
- Norman J E, Bollapragada S, Yuan M, Nelson S M. Inflammatory pathways in the mechanism of parturition. BMC Pregnancy Childbirth 2007; 7(Suppl 1)S7
- Romero R, Espinoza J, Goncalves L F, Kusanovic J P, Friel L, Hassan S. The role of inflammation and infection in preterm birth. Semin Reprod Med 2007; 25: 21–39
- Keelan J A, Marvin K W, Sato T A, Coleman M, McCowan L M, Mitchell M D. Cytokine abundance in placental tissues: Evidence of inflammatory activation in gestational membranes with term and preterm parturition. Am J Obstet Gynecol 1999; 181: 1530–1536
- Romero R, Mazor M, Brandt F, Sepulveda W, Avila C, Cotton D B, Dinarello C A. Interleukin-1 alpha and interleukin-1 beta in preterm and term human parturition. Am J Reprod Immunol 1992; 27: 117–123
- Romero R, Tartakovsky B. The natural interleukin-1 receptor antagonist prevents interleukin-1-induced preterm delivery in mice. Am J Obstet Gynecol 1992; 167: 1041–1045
- Romero R, Sepulveda W, Mazor M, Brandt F, Cotton D B, Dinarello C A, Mitchell M D. The natural interleukin-1 receptor antagonist in term and preterm parturition. Am J Obstet Gynecol 1992; 167: 863–872
- Winkler M, Kemp B, Fischer D C, Maul H, Hlubek M, Rath W. Tissue concentrations of cytokines in the lower uterine segment during preterm parturition. J Perinat Med 2001; 29: 519–527
- Fidel P L, Jr, Romero R, Wolf N, Cutright J, Ramirez M, Araneda H, Cotton D B. Systemic and local cytokine profiles in endotoxin-induced preterm parturition in mice. Am J Obstet Gynecol 1994; 170: 1467–1475
- Romero R, Avila C, Santhanam U, Sehgal P B. Amniotic fluid interleukin 6 in preterm labor. Association with infection. J Clin Invest 1990; 85: 1392–1400
- Romero R, Sepulveda W, Kenney J S, Archer L E, Allison A C, Sehgal P B. Interleukin 6 determination in the detection of microbial invasion of the amniotic cavity. Ciba Found Symp 1992; 167: 205–220
- Romero R, Yoon B H, Kenney J S, Gomez R, Allison A C, Sehgal P B. Amniotic fluid interleukin-6 determinations are of diagnostic and prognostic value in preterm labor. Am J Reprod Immunol 1993; 30: 167–183
- Romero R, Manogue K R, Mitchell M D, Wu Y K, Oyarzun E, Hobbins J C, Cerami A. Infection and labor. IV. Cachectin-tumor necrosis factor in the amniotic fluid of women with intraamniotic infection and preterm labor. Am J Obstet Gynecol 1989; 161: 336–341
- Romero R, Mazor M, Sepulveda W, Avila C, Copeland D, Williams J. Tumor necrosis factor in preterm and term labor. Am J Obstet Gynecol 1992; 166: 1576–1587
- Baumann P, Romero R, Berry S, Gomez R, McFarlin B, Araneda H, Cotton D B, Fidel P. Evidence of participation of the soluble tumor necrosis factor receptor I in the host response to intrauterine infection in preterm labor. Am J Reprod Immunol 1993; 30: 184–193
- Maymon E, Ghezzi F, Edwin S S, Mazor M, Yoon B H, Gomez R, Romero R. The tumor necrosis factor alpha and its soluble receptor profile in term and preterm parturition. Am J Obstet Gynecol 1999; 181: 1142–1148
- Fortunato S J, Menon R, Lombardi S J. Role of tumor necrosis factor-alpha in the premature rupture of membranes and preterm labor pathways. Am J Obstet Gynecol 2002; 187: 1159–1162
- Lonergan M, Aponso D, Marvin K W, Helliwell R J, Sato T A, Mitchell M D, Chaiwaropongsa T, Romero R, Keelan J A. Tumor necrosis factor-related apoptosis- inducing ligand (TRAIL), TRAIL receptors, and the soluble receptor osteoprotegerin in human gestational membranes and amniotic fluid during pregnancy and labor at term and preterm. J Clin Endocrinol Metab 2003; 88: 3835–3844
- Pacora P, Romero R, Maymon E, Gervasi M T, Gomez R, Edwin S S, Yoon B H. Participation of the novel cytokine interleukin 18 in the host response to intra-amniotic infection. Am J Obstet Gynecol 2000; 183: 1138–1143
- Athayde N, Romero R, Maymon E, Gomez R, Pacora P, Yoon B H, Edwin S S. Interleukin 16 in pregnancy, parturition, rupture of fetal membranes, and microbial invasion of the amniotic cavity. Am J Obstet Gynecol 2000; 182: 135–141
- Romero R, Ceska M, Avila C, Mazor M, Behnke E, Lindley I. Neutrophil attractant/activating peptide-1/interleukin-8 in term and preterm parturition. Am J Obstet Gynecol 1991; 165: 813–820
- Cherouny P H, Pankuch G A, Romero R, Botti J J, Kuhn D C, Demers L M, Appelbaum P C. Neutrophil attractant/activating peptide-1/interleukin-8: Association with histologic chorioamnionitis, preterm delivery, and bioactive amniotic fluid leukoattractants. Am J Obstet Gynecol 1993; 169: 1299–1303
- Romero R, Gomez R, Galasso M, Munoz H, Acosta L, Yoon B H, Svinarich D, Cotton D B. Macrophage inflammatory protein-1 alpha in term and preterm parturition: Effect of microbial invasion of the amniotic cavity. Am J Reprod Immunol 1994; 32: 108–113
- Athayde N, Romero R, Maymon E, Gomez R, Pacora P, Araneda H, Yoon B H. A role for the novel cytokine RANTES in pregnancy and parturition. Am J Obstet Gynecol 1999; 181: 989–994
- Gonzalez B E, Ferrer I, Valls C, Borras M, Lailla J M. The value of interleukin-8, interleukin-6 and interleukin-1beta in vaginal wash as predictors of preterm delivery. Gynecol Obstet Invest 2005; 59: 175–178
- Esplin M S, Romero R, Chaiworapongsa T, Kim Y M, Edwin S, Gomez R, Mazor M, Adashi E Y. Monocyte chemotactic protein-1 is increased in the amniotic fluid of women who deliver preterm in the presence or absence of intra-amniotic infection. J Matern Fetal Neonatal Med 2005; 17: 365–373
- Esplin M S, Peltier M R, Hamblin S, Smith S, Fausett M B, Dildy G A, Branch D W, Silver R M, Adashi E Y. Monocyte chemotactic protein-1 expression is increased in human gestational tissues during term and preterm labor. Placenta 2005; 26: 661–671
- Ammala M, Nyman T, Salmi A, Rutanen E M. The interleukin-1 system in gestational tissues at term: Effect of labour. Placenta 1997; 18: 717–723
- Taniguchi T, Matsuzaki N, Kameda T, Shimoya K, Jo T, Saji F, Tanizawa O. The enhanced production of placental interleukin-1 during labor and intrauterine infection. Am J Obstet Gynecol 1991; 165: 131–137
- Dudley D J, Collmer D, Mitchell M D, Trautman M S. Inflammatory cytokine mRNA in human gestational tissues: Implications for term and preterm labor. J Soc Gynecol Investig 1996; 3: 328–335
- Romero R, Brody D T, Oyarzun E, Mazor M, Wu Y K, Hobbins J C, Durum S K. Infection and labor. III. Interleukin-1: A signal for the onset of parturition. Am J Obstet Gynecol 1989; 160: 1117–1123
- Romero R, Mazor M, Tartakovsky B. Systemic administration of interleukin-1 induces preterm parturition in mice. Am J Obstet Gynecol 1991; 165: 969–971
- Sadowsky D W, Adams K M, Gravett M G, Witkin S S, Novy M J. Preterm labor is induced by intraamniotic infusions of interleukin-1beta and tumor necrosis factor-alpha but not by interleukin-6 or interleukin-8 in a nonhuman primate model. Am J Obstet Gynecol 2006; 195: 1578–1589
- Nhan T Q, Liles W C, Schwartz S M. Physiological functions of caspases beyond cell death. Am J Pathol 2006; 169: 729–737
- Creagh E M, Conroy H, Martin S J. Caspase-activation pathways in apoptosis and immunity. Immunol Rev 2003; 193: 10–21
- Joshi V D, Kalvakolanu D V, Cross A S. Simultaneous activation of apoptosis and inflammation in pathogenesis of septic shock: A hypothesis. FEBS Lett 2003; 555: 180–184
- Wilson K P, Black J A, Thomson J A, Kim E E, Griffith J P, Navia M A, Murcko, M A, Chambers S P, Aldape R A, Raybuck S A, et al. Structure and mechanism of interleukin-1 beta converting enzyme. Nature 1994; 370: 270–275
- Hersh D, Monack D M, Smith M R, Ghori N, Falkow S, Zychlinsky A. The Salmonella invasin SipB induces macrophage apoptosis by binding to caspase-1. Proc Natl Acad Sci U S A 1999; 96: 2396–2401
- Hilbi H, Moss J E, Hersh D, Chen Y, Arondel J, Banerjee S, Flavell R A, Yuan J, Sansonetti P J, Zychlinsky A. Shigella-induced apoptosis is dependent on caspase-1 which binds to IpaB. J Biol Chem 1998; 273: 32 895–32 900
- Tocci M J. Structure and function of interleukin-1 beta converting enzyme. Vitam Horm 1997; 53: 27–63
- Singer I I, Scott S, Chin J, Bayne E K, Limjuco G, Weidner J, Miller D K, Chapman K, Kostura M J. The interleukin-1 beta-converting enzyme (ICE) is localized on the external cell surface membranes and in the cytoplasmic ground substance of human monocytes by immuno-electron microscopy. J Exp Med 1995; 182: 1447–1459
- Ayala J M, Yamin T T, Egger L A, Chin J, Kostura M J, Miller D K. IL-1 beta-converting enzyme is present in monocytic cells as an inactive 45-kDa precursor. J Immunol 1994; 153: 2592–2599
- Miossec C, Decoen M C, Durand L, Fassy F, Diu-Hercend A. Use of monoclonal antibodies to study interleukin-1 beta-converting enzyme expression: Only precursor forms are detected in interleukin-1 beta-secreting cells. Eur J Immunol 1996; 26: 1032–1042
- Earnshaw W C, Martins L M, Kaufmann S H. Mammalian caspases: Structure, activation, substrates, and functions during apoptosis. Annu Rev Biochem 1999; 68: 383–424
- Nicholson D W, Ali A, Thornberry N A, Vaillancourt J P, Ding C K, Gallant M, Gareau Y, Griffin P R, Labelle M, Lazebnik Y A, et al. Identification and inhibition of the ICE/CED-3 protease necessary for mammalian apoptosis. Nature 1995; 376: 37–43
- Walker N P, Talanian R V, Brady K D, Dang L C, Bump N J, Ferenz C R, Franklin S, Ghayur T, Hackett M C, Hammill L D, et al. Crystal structure of the cysteine protease interleukin-1 beta-converting enzyme: A (p20/p10)2 homodimer. Cell 1994; 78: 343–352
- Moran L B, Duke D C, Graeber M B. The microglial gene regulatory network activated by interferon-gamma. J Neuroimmunol 2007; 183: 1–6
- Cerretti D P, Hollingsworth L T, Kozlosky C J, Valentine M B, Shapiro D N, Morris S W, Nelson N. Molecular characterization of the gene for human interleukin-1 beta converting enzyme (IL1BC). Genomics 1994; 20: 468–473
- Nett M A, Cerretti D P, Berson D R, Seavitt J, Gilbert D J, Jenkins N A, Copeland N G, Black R A, Chaplin D D. Molecular cloning of the murine IL-1 beta converting enzyme cDNA. J Immunol 1992; 149: 3254–3259
- Keane K M, Giegel D A, Lipinski W J, Callahan M J, Shivers B D. Cloning, tissue expression and regulation of rat interleukin 1 beta converting enzyme. Cytokine 1995; 7: 105–110
- Ahmed M, Shaban Z, Yamaji D, Okamatsu-Ogura Y, Soliman M, Abd E M, Ishioka K, Makondo K, Saito M, Kimura K. Induction of proinflammatory cytokines and caspase-1 by leptin in monocyte/macrophages from Holstein cows. J Vet Med Sci 2007; 69: 509–514
- Coward W R, Marei A, Yang A, Vasa-Nicotera M M, Chow S C. Statin-induced proinflammatory response in mitogen-activated peripheral blood mononuclear cells through the activation of caspase-1 and IL-18 secretion in monocytes. J Immunol 2006; 176: 5284–5292
- Singer I I, Scott S, Hall G L, Limjuco G, Chin J, Schmidt J A. Interleukin 1 beta is localized in the cytoplasmic ground substance but is largely absent from the Golgi apparatus and plasma membranes of stimulated human monocytes. J Exp Med 1988; 167: 389–407
- Black R A, Kronheim S R, Sleath P R. Activation of interleukin-1 beta by a co-induced protease. FEBS Lett 1989; 247: 386–390
- Hazuda D J, Lee J C, Young P R. The kinetics of interleukin 1 secretion from activated monocytes. Differences between interleukin 1 alpha and interleukin 1 beta. J Biol Chem 1988; 263: 8473–8479
- Black R A, Kronheim S R, Cantrell M, Deeley M C, March C J, Prickett K S, Wignall J, Conlon P J, Cosman D, Hopp T P, et al. Generation of biologically active interleukin-1 beta by proteolytic cleavage of the inactive precursor. J Biol Chem 1988; 263: 9437–9442
- Howard A D, Palyha O C, Griffin P R, Peterson E P, Lenny A B, Ding G J, Pickup D J, Thornberry N A, Schmidt J A, Tocci M J. Human IL-1 beta processing and secretion in recombinant baculovirus-infected Sf9 cells is blocked by the cowpox virus serpin crmA. J Immunol 1995; 154: 2321–2332
- Kuida K, Lippke J A, Ku G, Harding M W, Livingston D J, Su M S, Flavell R A. Altered cytokine export and apoptosis in mice deficient in interleukin-1 beta converting enzyme. Science 1995; 267: 2000–2003
- Li P, Allen H, Banerjee S, Franklin S, Herzog L, Johnston C, McDowell J, Paskind M, Rodman L, Salfeld J, et al. Mice deficient in IL-1 beta-converting enzyme are defective in production of mature IL-1 beta and resistant to endotoxic shock. Cell 1995; 80: 401–411
- Ghayur T, Banerjee S, Hugunin M, Butler D, Herzog L, Carter A, Quintal L, Sekut L, Talanian R, Paskind M, et al. Caspase-1 processes IFN-gamma-inducing factor and regulates LPS-induced IFN-gamma production. Nature 1997; 386: 619–623
- Beutler B, Cerami A. The endogenous mediator of endotoxic shock. Clin Res 1987; 35: 192–197
- Mantovani A, Muzio M, Ghezzi P, Colotta C, Introna M. Regulation of inhibitory pathways of the interleukin-1 system. Ann N Y Acad Sci 1998; 840: 338–351
- Burns K, Martinon F, Tschopp J. New insights into the mechanism of IL-1beta maturation. Curr Opin Immunol 2003; 15: 26–30
- Dinarello C A. Interleukin-1 beta, interleukin-18, and the interleukin-1 beta converting enzyme. Ann N Y Acad Sci 1998; 856: 1–11
- Librach C L, Feigenbaum S L, Bass K E, Cui T Y, Verastas N, Sadovsky Y, Quigley J P, French D L, Fisher S J. Interleukin-1 beta regulates human cytotrophoblast metalloproteinase activity and invasion in vitro. J Biol Chem 1994; 269: 17 125–17 131
- Simon C, Frances A, Piquette G, Hendrickson M, Milki A, Polan M L. Interleukin-1 system in the materno-trophoblast unit in human implantation: Immunohistochemical evidence for autocrine/paracrine function. J Clin Endocrinol Metab 1994; 78: 847–854
- Okamura H, Tsutsi H, Komatsu T, Yutsudo M, Hakura A, Tanimoto T, Torigoe K, Okura T, Nukada Y, Hattori K, et al. Cloning of a new cytokine that induces IFN-gamma production by T cells. Nature 1995; 378: 88–91
- Dinarello C A. IL-18: A TH1-inducing, proinflammatory cytokine and new member of the IL-1 family. J Allergy Clin Immunol 1999; 103: 11–24
- Ushio S, Namba M, Okura T, Hattori K, Nukada Y, Akita K, Tanabe F, Konishi K, Micallef M, Fujii M, et al. Cloning of the cDNA for human IFN-gamma-inducing factor, expression in Escherichia coli, and studies on the biologic activities of the protein. J Immunol 1996; 156: 4274–4279
- Gu Y, Kuida K, Tsutsui H, Ku G, Hsiao K, Fleming M A, Hayashi N, Higashino K, Okamura H, Nakanishi K, et al. Activation of interferon-gamma inducing factor mediated by interleukin-1beta converting enzyme. Science 1997; 275: 206–209
- Pirhonen J, Sareneva T, Kurimoto M, Julkunen I, Matikainen S. Virus infection activates IL-1 beta and IL-18 production in human macrophages by a caspase-1-dependent pathway. J Immunol 1999; 162: 7322–7329
- Sugawara S, Uehara A, Nochi T, Yamaguchi T, Ueda H, Sugiyama A, Hanzawa K, Kumagai K, Okamura H, Takada H. Neutrophil proteinase 3-mediated induction of bioactive IL-18 secretion by human oral epithelial cells. J Immunol 2001; 167: 6568–6575
- Takeda K, Tsutsui H, Yoshimoto T, Adachi O, Yoshida N, Kishimoto T, Okamura H, Nakanishi K, Akira S. Defective NK cell activity and Th1 response in IL-18-deficient mice. Immunity 1998; 8: 383–390
- Gracie J A, Robertson S E, McInnes I B. Interleukin-18. J Leukoc Biol 2003; 73: 213–224
- Hoshino T, Wiltrout R H, Young H A. IL-18 is a potent coinducer of IL-13 in NK and T cells: A new potential role for IL-18 in modulating the immune response. J Immunol 1999; 162: 5070–5077
- Hoshino T, Yagita H, Ortaldo J R, Wiltrout R H, Young H A. In vivo administration of IL-18 can induce IgE production through Th2 cytokine induction and up-regulation of CD40 ligand (CD154) expression on CD4+ T cells. Eur J Immunol 2000; 30: 1998–2006
- Tsutsui H, Nakanishi K, Matsui K, Higashino K, Okamura H, Miyazawa Y, Kaneda K. IFN-gamma-inducing factor up-regulates Fas ligand-mediated cytotoxic activity of murine natural killer cell clones. J Immunol 1996; 157: 3967–3973
- Dao T, Ohashi K, Kayano T, Kurimoto M, Okamura H. Interferon-gamma-inducing factor, a novel cytokine, enhances Fas ligand-mediated cytotoxicity of murine T helper 1 cells. Cell Immunol 1996; 173: 230–235
- Yoshimoto T, Takeda K, Tanaka T, Ohkusu K, Kashiwamura S, Okamura H, Akira S, Nakanishi K. IL-12 up-regulates IL-18 receptor expression on T cells, Th1 cells, and B cells: Synergism with IL-18 for IFN-gamma production. J Immunol 1998; 161: 3400–3407
- Micallef M J, Ohtsuki T, Kohno K, Tanabe F, Ushio S, Namba M, Tanimoto T, Torigoe K, Fujii M, Ikeda M, et al. Interferon-gamma-inducing factor enhances T helper 1 cytokine production by stimulated human T cells: Synergism with interleukin-12 for interferon-gamma production. Eur J Immunol 1996; 26: 1647–1651
- Dao T, Mehal W Z, Crispe I N. IL-18 augments perforin-dependent cytotoxicity of liver NK-T cells. J Immunol 1998; 161: 2217–2222
- Gracie J A, Forsey R J, Chan W L, Gilmour A, Leung B P, Greer M R, Kennedy K, Carter R, Wei X Q, Xu D, et al. A proinflammatory role for IL-18 in rheumatoid arthritis. J Clin Invest 1999; 104: 1393–1401
- Leung B P, Culshaw S, Gracie J A, Hunter D, Canetti C A, Campbell C, Cunha F, Liew F Y, McInnes I B. A role for IL-18 in neutrophil activation. J Immunol 2001; 167: 2879–2886
- Hook C E, Matyszak M K, Gaston J S. Infection of epithelial and dendritic cells by Chlamydia trachomatis results in IL-18 and IL-12 production, leading to interferon-gamma production by human natural killer cells. FEMS Immunol Med Microbiol 2005; 45: 113–120
- Lu H, Shen C, Brunham R C. Chlamydia trachomatis infection of epithelial cells induces the activation of caspase-1 and release of mature IL-18. J Immunol 2000; 165: 1463–1469
- Muneta Y, Minagawa Y, Shimoji Y, Nagata R, Markham P F, Browning G F, Mori Y. IL-18 expression in pigs following infection with Mycoplasma hyopneumoniae. J Interferon Cytokine Res 2006; 26: 637–644
- Tanaka H, Narita M, Teramoto S, Saikai T, Oashi K, Igarashi T, Abe S. Role of interleukin-18 and T-helper type 1 cytokines in the development of Mycoplasma pneumoniae pneumonia in adults. Chest 2002; 121: 1493–1497
- Narita M, Tanaka H, Abe S, Yamada S, Kubota M, Togashi T. Close association between pulmonary disease manifestation in Mycoplasma pneumoniae infection and enhanced local production of interleukin-18 in the lung, independent of gamma interferon. Clin Diagn Lab Immunol 2000; 7: 909–914
- Kinjo Y, Kawakami K, Uezu K, Yara S, Miyagi K, Koguchi Y, Hoshino T, Okamoto M, Kawase Y, Yokota K, et al. Contribution of IL-18 to Th1 response and host defense against infection by Mycobacterium tuberculosis: A comparative study with IL-12p40. J Immunol 2002; 169: 323–329
- Vankayalapati R, Wizel B, Weis S E, Samten B, Girard W M, Barnes P F. Production of interleukin-18 in human tuberculosis. J Infect Dis 2000; 182: 234–239
- Yamada G, Shijubo N, Shigehara K, Okamura H, Kurimoto M, Abe S. Increased levels of circulating interleukin-18 in patients with advanced tuberculosis. Am J Respir Crit Care Med 2000; 161: 1786–1789
- Garcia V E, Uyemura K, Sieling P A, Ochoa M T, Morita C T, Okamura H, Kurimoto M, Rea T H, Modlin R L. IL-18 promotes type 1 cytokine production from NK cells and T cells in human intracellular infection. J Immunol 1999; 162: 6114–6121
- Mastroeni P, Clare S, Khan S, Harrison J A, Hormaeche C E, Okamura H, Kurimoto M, Dougan G. Interleukin 18 contributes to host resistance and gamma interferon production in mice infected with virulent Salmonella typhimurium. Infect Immun 1999; 67: 478–483
- Sansonetti P J, Phalipon A, Arondel J, Thirumalai K, Banerjee S, Akira S. Caspase-1 activation of IL-1beta and IL-18 are essential for Shigella flexneri-induced inflammation. Immunity 2000; 12: 581–590
- Bohn E, Sing A, Zumbihl R, Bielfeldt C, Okamura H, Kurimoto M, Heesemann J, Autenrieth I B. IL-18 (IFN-gamma-inducing factor) regulates early cytokine production in, and promotes resolution of, bacterial infection in mice. J Immunol 1998; 160: 299–307
- Qureshi M H, Zhang T, Koguchi Y, Nakashima K, Okamura H, Kurimoto M, Kawakami K. Combined effects of IL-12 and IL-18 on the clinical course and local cytokine production in murine pulmonary infection with Cryptococcus neoformans. Eur J Immunol 1999; 29: 643–649
- Kawakami K, Qureshi M H, Zhang T, Okamura H, Kurimoto M, Saito A. IL-18 protects mice against pulmonary and disseminated infection with Cryptococcus neoformans by inducing IFN-gamma production. J Immunol 1997; 159: 5528–5534
- Mencacci A, Bacci A, Cenci E, Montagnoli C, Fiorucci S, Casagrande A, Flavell R A, Bistoni F, Romani L. Interleukin 18 restores defective Th1 immunity to Candida albicans in caspase 1-deficient mice. Infect Immun 2000; 68: 5126–5131
- Cai G, Kastelein R, Hunter C A. Interleukin-18 (IL-18) enhances innate IL-12-mediated resistance to Toxoplasma gondii. Infect Immun 2000; 68: 6932–6938
- Yap G S, Ortmann R, Shevach E, Sher A. A heritable defect in IL-12 signaling in B10.Q/J mice. II. Effect on acute resistance to Toxoplasma gondii and rescue by IL-18 treatment. J Immunol 2001; 166: 5720–5725
- Fujioka N, Akazawa R, Ohashi K, Fujii M, Ikeda M, Kurimoto M. Interleukin-18 protects mice against acute herpes simplex virus type 1 infection. J Virol 1999; 73: 2401–2409
- Tokmadzic V S, Tsuji Y, Bogovic T, Laskarin G, Cupurdija K, Strbo N, Koyama K, Okamura H, Podack E R, Rukavina D. IL-18 is present at the maternal–fetal interface and enhances cytotoxic activity of decidual lymphocytes. Am J Reprod Immunol 2002; 48: 191–200
- Ostojic S, Dubanchet S, Chaouat G, Abdelkarim M, Truyens C, Capron F. Demonstration of the presence of IL-16, IL-17 and IL-18 at the murine fetomaternal interface during murine pregnancy. Am J Reprod Immunol 2003; 49: 101–112
- Ledee-Bataille N, Dubanchet S, Coulomb-L'hermine A, Durand-Gasselin I, Frydman R, Chaouat G. A new role for natural killer cells, interleukin (IL)- 12, and IL-18 in repeated implantation failure after in vitro fertilization. Fertil Steril 2004; 81: 59–65
- Laskarin G, Strbo N, Bogovic C T, Juretic K, Ledee B N, Chaouat G, Rukavina D. Physiological role of IL-15 and IL-18 at the maternal–fetal interface. Chem Immunol Allergy 2005; 89: 10–25
- Jacobsson B, Holst R M, Mattsby-Baltzer I, Nikolaitchouk N, Wennerholm U B, Hagberg H. Interleukin-18 in cervical mucus and amniotic fluid: Relationship to microbial invasion of the amniotic fluid, intra-amniotic inflammation and preterm delivery. BJOG 2003; 110: 598–603
- Wang X, Hagberg H, Mallard C, Zhu C, Hedtjarn M, Tiger C F, Eriksson K, Rosen A, Jacobsson B. Disruption of interleukin-18, but not interleukin-1, increases vulnerability to preterm delivery and fetal mortality after intrauterine inflammation. Am J Pathol 2006; 169: 967–976
- Fazleabas A T, Kim J J, Strakova Z. Implantation: Embryonic signals and the modulation of the uterine environment—a review. Placenta 2004; 25(Suppl A)S26–31
- Ida A, Tsuji Y, Muranaka J, Kanazawa R, Nakata Y, Adachi S, Okamura H, Koyama K. IL-18 in pregnancy: The elevation of IL-18 in maternal peripheral blood during labour and complicated pregnancies. J Reprod Immunol 2000; 47: 65–74
- Jokhi P P, King A, Loke Y W. Cytokine production and cytokine receptor expression by cells of the human first trimester placental-uterine interface. Cytokine 1997; 9: 126–137
- Menon R, Swan K F, Lyden T W, Rote N S, Fortunato S J. Expression of inflammatory cytokines (interleukin-1 beta and interleukin-6) in amniochorionic membranes. Am J Obstet Gynecol 1995; 172: 493–500
- Menon R, Lombardi S J, Fortunato S J. IL-18, a product of choriodecidual cells, increases during premature rupture of membranes but fails to turn on the Fas-FasL-mediated apoptosis pathway. J Assist Reprod Genet 2001; 18: 276–284
- White C A, Dimitriadis E, Sharkey A M, Stoikos C J, Salamonsen L A. Interleukin 1 beta is induced by interleukin 11 during decidualization of human endometrial stromal cells, but is not released in a bioactive form. J Reprod Immunol 2007; 73: 28–38
- Lindstrom T M, Bennett P R. The role of nuclear factor kappa B in human labour. Reproduction 2005; 130: 569–581
- Hertelendy F, Rastogi P, Molnar M, Romero R. Interleukin-1beta-induced prostaglandin E2 production in human myometrial cells: Role of a pertussis toxin-sensitive component. Am J Reprod Immunol 2001; 45: 142–147
- Hertelendy F, Romero R, Molnar M, Todd H, Baldassare J J. Cytokine-initiated signal transduction in human myometrial cells. Am J Reprod Immunol 1993; 30: 49–57
- Romero R, Durum S, Dinarello C A, Oyarzun E, Hobbins J C, Mitchell M D. Interleukin-1 stimulates prostaglandin biosynthesis by human amnion. Prostaglandins 1989; 37: 13–22
- Mitchell M D, Chang M C, Chaiworapongsa T, Lan H Y, Helliwell R J, Romero R, Sato T A. Identification of 9alpha,11beta-prostaglandin F2 in human amniotic fluid and characterization of its production by human gestational tissues. J Clin Endocrinol Metab 2005; 90: 4244–4248
- Belt A R, Baldassare J J, Molnar M, Romero R, Hertelendy F. The nuclear transcription factor NF-kappaB mediates interleukin-1beta-induced expression of cyclooxygenase-2 in human myometrial cells. Am J Obstet Gynecol 1999; 181: 359–366
- Romero R, Emamian M, Wan M, Quintero R, Hobbins J C, Mitchell M D. Prostaglandin concentrations in amniotic fluid of women with intra-amniotic infection and preterm labor. Am J Obstet Gynecol 1987; 157: 1461–1467
- Romero R, Wu Y K, Sirtori M, Oyarzun E, Mazor M, Hobbins J C, Mitchell M D. Amniotic fluid concentrations of prostaglandin F2 alpha, 13,14-dihydro-15-keto-prostaglandin F2 alpha (PGFM) and 11-deoxy-13,14-dihydro-15-keto-11,16-cyclo-prostaglandin E2 (PGEM-LL) in preterm labor. Prostaglandins 1989; 37: 149–161
- Romero R, Wu Y K, Brody D T, Oyarzun E, Duff G W, Durum S K. Human decidua: A source of interleukin-1. Obstet Gynecol 1989; 73: 31–34
- Sakao Y, Takeda K, Tsutsui H, Kaisho T, Nomura F, Okamura H, Nakanishi K, Akira S. IL-18-deficient mice are resistant to endotoxin-induced liver injury but highly susceptible to endotoxin shock. Int Immunol 1999; 11: 471–480
- Dinarello C A. Therapeutic strategies to reduce IL-1 activity in treating local and systemic inflammation. Curr Opin Pharmacol 2004; 4: 378–385
- Boost K A, Hoegl S, Hofstetter C, Flondor M, Stegewerth K, Platacis I, Pfeilschifter J, Muhl H, Zwissler B. Targeting caspase-1 by inhalation-therapy: Effects of Ac-YVAD-CHO on IL-1 beta, IL-18 and downstream proinflammatory parameters as detected in rat endotoxaemia. Intensive Care Med 2007; 33: 863–871
- Norman J, Yang J, Fink G, Carter G, Ku G, Denham W, Livingston D. Severity and mortality of experimental pancreatitis are dependent on interleukin-1 converting enzyme (ICE). J Interferon Cytokine Res 1997; 17: 113–118
- Joshi V D, Kalvakolanu D V, Hebel J R, Hasday J D, Cross A S. Role of caspase 1 in murine antibacterial host defenses and lethal endotoxemia. Infect Immun 2002; 70: 6896–6903
- Sarkar A, Hall M W, Exline M, Hart J, Knatz N, Gatson N T, Wewers M D. Caspase-1 regulates Escherichia coli sepsis and splenic B cell apoptosis independently of interleukin-1beta and interleukin-18. Am J Respir Crit Care Med 2006; 174: 1003–1010
- Hotchkiss R S, Chang K C, Swanson P E, Tinsley K W, Hui J J, Klender P, Xanthoudakis S, Roy S, Black C, Grimm E, et al. Caspase inhibitors improve survival in sepsis: A critical role of the lymphocyte. Nat Immunol 2000; 1: 496–501
- Hotchkiss R S, Swanson P E, Cobb J P, Jacobson A, Buchman T G, Karl I E. Apoptosis in lymphoid and parenchymal cells during sepsis: Findings in normal and T- and B-cell-deficient mice. Crit Care Med 1997; 25: 1298–1307
- Wang S D, Huang K J, Lin Y S, Lei H Y. Sepsis-induced apoptosis of the thymocytes in mice. J Immunol 1994; 152: 5014–5021
- Ayala A, Herdon C D, Lehman D L, Ayala C A, Chaudry I H. Differential induction of apoptosis in lymphoid tissues during sepsis: Variation in onset, frequency, and the nature of the mediators. Blood 1996; 87: 4261–4275
- Bilbo S D, Biedenkapp J C, Der-Avakian A, Watkins L R, Rudy J W, Maier S F. Neonatal infection-induced memory impairment after lipopolysaccharide in adulthood is prevented via caspase-1 inhibition. J Neurosci 2005; 25: 8000–8009
- Micci M A, Pattillo M T, Kahrig K M, Pasricha P J. Caspase inhibition increases survival of neural stem cells in the gastrointestinal tract. Neurogastroenterol Motil 2005; 17: 557–564