Abstract
Infectious diseases caused by viruses like HIV and SARS-COV-2 (COVID-19) pose serious public health threats. In search for new antiviral small molecules from chemically underexplored Hypericum species, a previously undescribed atropisomeric C8-C8’ linked dimeric coumarin named bichromonol (1) was isolated from the stem bark of Hypericum roeperianum. The structure was elucidated by MS data and NMR spectroscopy. The absolute configuration at the biaryl axis was determined by comparing the experimental ECD spectrum with those calculated for the respective atropisomers. Bichromonol was tested in cell-based assays for cytotoxicity against MT-4 (CC50 = 54 µM) cells and anti-HIV activity in infected MT-4 cells. It exhibits significant activity at EC50 = 6.6–12.0 µM against HIV-1 wild type and its clinically relevant mutant strains. Especially, against the resistant variants A17 and EFVR, bichromonol is more effective than the commercial drug nevirapine and might thus have potential to serve as a new anti-HIV lead.
Graphical Abstract
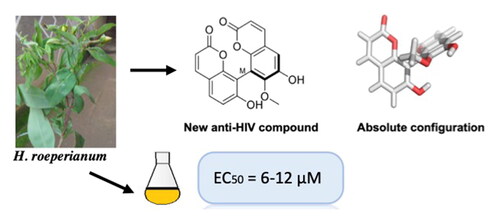
1. Introduction
Viral infections as those caused by the human immunodeficiency (HIV) or the new severe acute respiratory syndrome coronavirus 2 (SARS-COV-2) are among the major diseases enumerated by the World Health Organization (WHO) on its recent list of ten global health threats. There is a need for new antiviral drugs (UNAIDS Report Citation2020; Adamson et al. Citation2021).
Members of the Hypericum genus are well known for their biological activities against a range of diseases including depression, viral and microbial infections, skin wounds, gastrointestinal disorders, and intestinal worms (Toniolo et al. Citation2014; Mandrone et al. Citation2015; Caprioli et al. Citation2016; Fobofou et al. Citation2016; Venditti and Bianco Citation2018). During our collection of Cameroonian Hypericum species for bioactive compound discovery, we heard from the local population that the plants are traditionally used against mental disorders and by AIDS patients. This raised our curiosity, and we decided to evaluate their anti-HIV properties to determine whether Hypericum species can really help HIV-infected people and ultimately might hint toward a new anti-HIV lead compound from a medicinal plant (Fobofou et al. Citation2015). Hypericum roeperianum Schimp. ex A. Rich is one of the plant species that have recently been the focus of several investigations (Fobofou et al. Citation2014; Citation2015; Guefack et al. Citation2020; Damen et al. Citation2021; Demgne et al. Citation2021; Damen et al. Citation2022). Herein, the isolation, characterization, and anti-HIV activity of bichromonol (1) from the stem bark of H. roeperianum is reported. Previous studies on this plant (including our own investigations) reported the isolation of xanthones, polyketides, coumarins, and acylphloroglucinol derivatives as well as their cytotoxic, antibacterial, and anthelmintic activities (Fobofou et al. Citation2014; Citation2015; Guefack et al. Citation2020; Damen et al. Citation2021; Demgne et al. Citation2021; Damen et al. Citation2022). However, none of these compounds were found or described to exhibit anti-HIV activity. In our studies on the leaves of H. roeperianum, we could not find anti-HIV activity for the leaves extract and isolated constituents (prenylated acylphloroglucinol derivatives) at concentrations below cytotoxicity for exponentially growing MT-4 cells (Fobofou et al. Citation2015).
2. Results and discussions
The CHCl3 extract obtained from the stem bark of H. roeperianum exhibits significant anti-HIV-1IIIB activity (EC50= 0.4 µg/ml) associated with moderate cytotoxicity (CC50= 6 µg/ml) against MT-4 cells. MT-4 cells represent a HTLV-1-transformed human T-cell line sensitive to HIV infection. A portion of the CHCl3 extract (2.7 g) was subjected to repeated column chromatography to afford bichromonol (1) (1.4 mg, Rf = 0.34 in CH2Cl2-MeOH (90:10)).
Bichromonol (1) was isolated as an optically active, yellow, and amorphous substance, [α]25D −81.7 (c 6.0 mM, MeOH). The HR-ESI-FTMS indicates a deprotonated molecule at m/z 351.0525 ([M-H]-) consistent with the molecular formula C19H11O7-. The MS2 spectrum of the [M-H]- ion shows a base peak ion resulting from the loss of MeOH. Further fragmentations are mainly characterized by successive losses of CO2 and CO as previously described for MS/MS data of coumarin derivatives (Figure S4) (Heinke et al. Citation2012; Fobofou et al. Citation2014; Sun et al. Citation2020). The IR spectrum exhibits absorption bands of hydroxy (3353 cm−1) and carbonyl (1698 cm−1) groups. The 1H NMR spectrum (Table S1) shows the presence of eight proton signals including one methoxyl group (δH 3.69, 7-OMe). The observation of four distinct doublets at δH 6.31 (1H, d, J = 9.7 Hz, H-3), δH 7.90 (1H, d, J = 9.7 Hz, H-4), δH 6.17 (1H, d, J = 9.7 Hz, H-3′), δH 7.93 (1H, d, J = 9.7 Hz, H-4′) corresponding to two coumarin units (Liu et al. Citation2007), suggests that 1 is an unsymmetrical coumarin dimer. The 1H NMR spectrum (Table S1) also shows two signals at δH 6.95 (1H, d, J = 8.3 Hz, H-6′) and δH 7.57 (1H, d, J = 8.3 Hz, H-5′), characteristic of aromatic protons of a 1,2,3,4-tetrasubstituted benzene ring as well as one signal at δH 7.14 (1H, s, H-5) attributable to the proton of a 1,2,3,4,5-pentasubstituted benzene nucleus. The 1H and 13C NMR (Table S1) signals as well as the connectivity between the two coumarin units through a C-8/C-8′ biaryl axis were assigned unambiguously using DEPT, ROESY, COSY, HSQC, and HMBC spectroscopy. The 13C NMR spectrum reveals nineteen carbon signals including two carbonyl groups (δC 163.8, C-2; δC 163.7, C-2′), one methoxy group (δC 61.1, 7-OMe) as well as five oxygenated aromatic carbon signals (δC 148.8, C-6; δC 151.9, C-7; δC 147.6, C-8a; δC 161.6, C-7′; δC 155.0; C-8á). The COSY spectrum reveals correlations between H-3 and H-4, H-3′ and H-4′ as well as between H-5′ and H-6′. Key interactions are observed in the ROESY spectrum (Figure S15) between H-4 and H-3/H-5, H-4′ and H-3′/H-5′ as well as between H-5′ and H-6′. In addition, no ROESY correlation of the OMe group could be detected. These findings indicate the attachment of the methoxyl group to C-7 and the connection of the two coumarin units via C-8 and C-8′. This was further supported by the HMBC spectrum which reveals correlations from 7-OMe to C-7, from H-5 to C-4, C-8a, C-7, and C-6, from H-5′ to C-4′, C-8á, and C-7′ as well as from H-6′ to C-4á and C-8′ (Figure S15). Based on the above spectroscopic data, the structure of compound 1 () was characterized as 6,7′-dihydroxy-7-methoxy-8,8′-biscoumarin and trivially named bichromonol (derived from bis-2H-chromen-2-one alcohol, 1).
Figure 1. Structure of bichromonol (1) from Hypericum roeperianum and its most stable conformation with the Sa (or M) atropisomer configuration (dihedral angles 8a-8-8’-8á = −67.9°, 8-7-O-C = −71.4°) constructed using MOE 2013.0801 (see Supplemental Information).
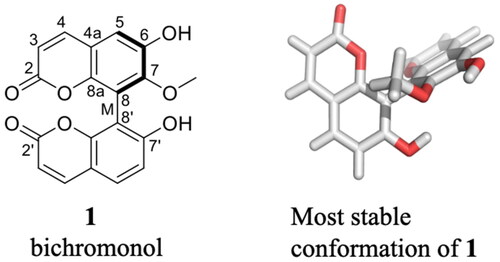
Table 1. Cytotoxicity and antiviral activity of bichromonol (1) against HIV-1 and its NNRTI-(N119, A17, EFVR) and NRTI-(AZTR, MDR) resistant variants.
Only a few simple coumarin derivatives were previously reported from the genus Hypericum (Ang’edu et al. Citation1999; Nedialkov et al. Citation2007). However, H. roeperianum is the sole producer of dimeric coumarins so far described from this genus (Fobofou et al. Citation2014). Furthermore, bichromonol (1) belongs to C8-C8’ linked dimeric coumarins and is reported herein as a new compound for the first time. The absolute configuration of bichromonol (1, ) was determined by comparing its experimental ECD spectrum with those calculated by quantum mechanical methods (see details in supplemental information) for both configurations Sa (or M) and Ra (or P) for 30 singlet and triplet states. The comparison of the calculated with the experimental ECD spectrum shows that the compound is preferentially populated in the Sa-configuration (Figure S3). In general, dimeric coumarins were reported as racemic mixtures or optically pure compounds with either M or P absolute configuration (Baba et al. Citation1990; Hussain et al. Citation2012).
The CHCl3 extract and bichromonol (1) from the stem bark of H. roeperianum were tested in a cell-based assay against the human immunodeficiency virus type-1 (HIV-1), using efavirenz as reference inhibitor. The cytotoxicity against the uninfected MT-4 cells was evaluated in parallel with the antiviral activity. The CHCl3 extract displays substantial anti-HIV-1IIIB (EC50 = 0.4 µg/ml) activity associated with a moderate cytotoxicity (CC50 = 6 µg/ml) against the uninfected MT-4 cells. As reported in , bichromonol (1) shows a relevant activity (EC50 = 8.7 μM) associated with a lower cytotoxicity (CC50 = 54 µM). In contrast, prenylated acylphloroglucinol derivatives obtained from the same species were not active against HIV-1 (Fobofou et al. Citation2015). Since a critical issue in the long-term clinical management of HIV diseases is the development of drug resistance, bichromonol (1) was evaluated against a panel of viruses possessing mutations that confer selective resistance either to nucleoside/nucleotide reverse transcriptase inhibitors (NRTIs) or non-nucleoside reverse transcriptase inhibitors (NNRTIs) that often appear during highly active antiretroviral therapy (HAART), reducing its effectiveness. Bichromonol (1) shows significant activity with EC50 values ranging from 6.6 to 12.0 μM on all the clinically relevant HIV-1 mutant strains tested, as depicted in . It is noteworthy to mention that its activity on some of the resistant strains is higher than that of the reference anti-HIV drug nevirapine (). The activity exhibited by the new compound (1) demonstrates its potential as anti-HIV drug and the role of natural products to provide unique chemical entities in drug discovery programs. Recently, also ethanolic extracts of Hypericum hircinium and its components betulinic acid and 5,7,3′,5′-tetrahydroxyflavanone 7-O-glucoside were reported as active against HIV (Esposito et al. Citation2013; Ornano et al. Citation2018).
The safety profile of Bichromonol was analyzed through transepithelial electrical resistance (TEER) assay (Figure S18). It was observed that the tested concentration (30 µM) did not affect the TEER over the time of the experiment (144 h), keeping values like those of the untreated cells. Furthermore, the mode of action of 1 was preliminarily assessed in an enzymatic assay aimed at evaluating its capability to inhibit HIV-1 reverse transcriptase (RT) activity in vitro. Interestingly, unlike Efavirenz, a known RT inhibitor (NNRTI), the compound was inactive against RT (Figure S19), suggesting a different mode of action.
Studies based on various coumarins from plant sources and their synthetic analogs indicate that some of them behave like potent non-nucleoside RT-inhibitors and others as inhibitors of HIV-integrase or HIV-protease (Kostova Citation2006). However, the major approved anti-HIV drugs fall into five categories: the nucleoside/nucleotide reverse transcriptase inhibitors (NRTIs), the non-nucleoside reverse transcriptase inhibitors (NNRTIs), the protease inhibitors (PIs), entry inhibitors, and integrase inhibitors (Zhuang et al. Citation2020). Studies to identify the mode of action for dimeric coumarins as new anti-HIV-leads are ongoing. In addition, bichromonol (1) was evaluated for antibacterial activity against representative human pathogenic Gram negative (Escherichia coli), and Gram positive (Staphylococcus aureus, and Enterococcus faecalis) bacteria. Ciprofloxacin was used as reference compound. The tested compound does not show significant inhibitory activity (MIC > 1 mg/L). The lack of antibacterial activities against a panel of microorganisms might support the specific inhibitory effect of bichromonol as antiviral agent.
3. Experimental (see supporting information)
Supporting Information. Plant material, extraction and isolation, bioassay and ECD calculation methods, 1 D and 2 D NMR spectra, MS/MS fragmentation behavior for compound 1. This material is available free of charge via the Internet.
Bichromonol (1), 6,7′-dihydroxy-7-methoxy-8,8′-biscoumarin: Yellow amorphous powder; [α]25D −81.7 (c 6 mM, MeOH); UV (MeOH), λmax (log ε): 287 (1.49), 321 (1.51) nm; IR (ATR) υmax (cm−1): 3353, 2940, 2937, 1698, 1599, 1503, 1452, 1426, 1398, 1316, 1240, 1144, 1121, 1018, 834, 759; 1H NMR (600 MHz, CD3OD) ppm: 7.93 (1H, d J = 9.65 Hz, H-4′), 7.90 (1H, d J = 9.65 Hz, H-4), 7.57 (1H, d J = 8.33 Hz, H-5′), 7.14 (1H, s, H-5), 6.95 (1H, d J = 8.33 Hz, H-6′), 6.31 (1H, d J = 9.65 Hz, H-3), 6.17 (1H, d J = 9.65 Hz, H-3′), 3.69 (3H, s, 7-OMe), see Table S1; 13C NMR (150 MHz, CD3OD) ppm: 163.7 (C-2′), 163.3 (C-2), 161.6 (C-7′), 155.0 (C-8a'), 151.9 (C-7), 148.8 (C-6), 147.6. (C-8a), 146.5 (C-4′), 145.9 (C-4), 130.5 (C-5′), 116.6 (C-4a), 116.2 (C-8), 115.1 (C-3), 114.6 (C-5), 114.4 (C-6′), 113.1 (C-4a'), 112.0 (C-3′), 108.9 (C-8′), 61.1 (7-OMe), see Table S1; negative ion ESI-FTMS [m/z (rel. int., %)]: [M-H]- at m/z 351.0525 (calcd. for C19H11O7-, 351.0510). MS2 [m/z 351 (48)]: m/z 319.0253 [M-MeOH]- (100, calcd for C18H7O6-, 319.0248). MS3 [m/z 319 (100)]: m/z 291.0305 [M-MeOH-CO]- (100, calcd for C17H7O5-, 291.0299), 275.0357 [M-MeOH-CO2]- (16, calcd for C17H7O4-, 275.0305). MS4 [m/z 291 (100)]: m/z263.0357 [M-MeOH-CO-CO]- (100, calcd for C16H7O4-, 263.0305), 247.0400 [M-MeOH-CO-CO2]- (14, calcd for C16H7O3-, 247.0401).MS5 [m/z 263 (100)]: m/z 235.0409 [M-MeOH-CO-CO-CO]- (100, calcd for C15H7O3-, 235.0401).
4. Conclusion
Finally, the significant anti-HIV-1 activity of H. roeperianum stem bark extract supports its use in Cameroonian traditional medicine by AIDS patients. The compound bichromonol (1) was isolated as active principle and might provide a new anti-HIV lead. However, further studies are still required to produce derivatives with improved therapeutic index, to evaluate its toxicity, pharmacodynamics, andelucidate its mode of action.
Supplemental Material
Download PDF (1.8 MB)Acknowledgments
The authors acknowledge financial support from the DAAD (A/10/98628), the project HyperSpEED by EFRE and the state Saxony-Anhalt (ZS/2019/07/99747), and the Italian Ministry for University and Research (MIUR) (grant PRIN 2017, Prot. 2017M8R7N9).
Disclosure statement
The authors declare that they have no conflict of interest.
Additional information
Funding
References
- Adamson CS, Chibale K, Goss RJM, Jaspars M, Newman DJ, Dorrington RA. 2021. Antiviral drug discovery: preparing for the next pandemic. Chem Soc Rev. 50(6):3647–3655.
- Ang’edu CA, Schmidt J, Porzel A, Gitu P, Midiwo JO, Adam G. 1999. Coumarins from Hypericum keniense (Guttiferae). Pharmazie. 54:235–236.
- Baba K, Taniguti M, Yoneda Y, Kozawa M. 1990. Coumarin glycosides from Edgeworthia chrysantha. Phytochemistry. 29(1):247–249.
- Caprioli G, Alunno A, Beghelli D, Bianco A, Bramucci M, Frezza C, Iannarelli R, Papa F, Quassinti L, Sagratini G, et al. 2016. Polar constituents and biological activity of the berry-like fruits from Hypericum androsaemum L. Front Plant Sci. 7:232.
- Damen F, Demgne OMF, Bitchagno GTM, Celik I, Mpetga JDS, Tankeo SB, Opatz T, Kuete V, Tane P. 2021. A new polyketide from the bark of Hypericum roeperianum Schimp. (Hypericaceae). Nat Prod Res. 35(14):2381–2387.
- Damen F, Simo MJD, Demgne OMF, Çelik İ, Wamba BEN, Tapondjou LA, Beng VP, Levent S, Kuete V, Tene M. 2022. Roeperone A, a new tetraoxygenated xanthone and other compounds from the leaves of Hypericum roeperianum Schimp. (Hypericaceae). Nat Prod Res. 36(8):2071–2077.
- Demgne OMF, Damen F, Fankam AG, Guefack MF, Wamba BEN, Nayim P, Mbaveng AT, Bitchagno GTM, Tapondjou LA, Penlap VB, et al. 2021. Botanicals and phytochemicals from the bark of Hypericum roeperianum (Hypericaceae) had strong antibacterial activity and showed synergistic effects with antibiotics against multidrug-resistant bacteria expressing active efflux pumps. J Ethnopharmacol. 277:114257.
- Esposito F, Sanna C, Del Vecchio C, Cannas V, Venditti A, Corona A, Bianco A, Serrilli AM, Guarcini L, Parolin C, et al. 2013. Hypericum hircinum L. components as new single-molecule inhibitors of both HIV-1 reverse transcriptase-associated DNA polymerase and ribonuclease H activities. Pathog Dis. 68(3):116–124.
- Fobofou SAT, Franke K, Arnold N, Schmidt J, Wabo HK, Tane P, Wessjohann LA. 2014. Rare biscoumarin derivatives and flavonoids from Hypericum riparium. Phytochemistry. 105:171–177.
- Fobofou SAT, Franke K, Porzel A, Brandt W, Wessjohann LA. 2016. Tricyclic acylphloroglucinols from Hypericum lanceolatum and regioselective synthesis of selancins A and B. J Nat Prod. 79(4):743–753.
- Fobofou SAT, Franke K, Sanna G, Porzel A, Bullita E, La Colla P, Wessjohann LA. 2015. Isolation and anticancer, anthelminthic, and antiviral (HIV) activity of acylphloroglucinols, and regioselective synthesis of empetrifranzinans from Hypericum roeperianum. Bioorg Med Chem. 23(19):6327–6334.
- Guefack MF, Damen F, Mbaveng AT, Tankeo SB, Bitchagno GTM, Çelik İ, Simo MJD, Kuete V. 2020. Cytotoxic constituents of the bark of Hypericum roeperianum towards multidrug-resistant cancer cells. Evid Based Complement Alternat Med. 2020:1–11.
- Heinke R, Franke K, Michels K, Ali NAA, Wessjohann L, Schmidt J. 2012. Analysis of furanocoumarins from Yemenite Dorstenia species by liquid chromatography/electrospray tandem mass spectrometry. J Mass Spectrom. 47(1):7–22.
- Hussain H, Hussain J, Al-Harrasi A, Krohn K. 2012. The chemistry and biology of bicoumarins. Tetrahedron. 68(12):2553–2578.
- Kostova L. 2006. Coumarins as inhibitors of HIV reverse transcriptase. Curr HIV Res. 4(3):347–363.
- Liu J, Feng Z, Xu J, Wang Y, Zhang P. 2007. Rare biscoumarins and a chlorogenic acid derivative from Erycibe obtusifolia. Phytochemistry. 68(13):1775–1780.
- Mandrone M, Lorenzi B, Venditti A, Guarcini L, Bianco A, Sanna C, Ballero M, Poli F, Antognoni F. 2015. Antioxidant and anti-collagenase activity of Hypericum hircinum L. Ind Crops Prod. 76:402–408.
- Nedialkov PT, Dimitrova D-Z, Girreser U, Kitanov GM. 2007. A new isocoumarin from Hypericum annulatum. Nat Prod Res. 21(12):1056–1060.
- Ornano L, Feroci M, Guarcini L, Venditti A, Bianco A. 2018. Anti-HIV agents from nature: natural compounds from Hypericum hircinum and carbocyclic nucleosides from iridoids. Stud Nat Prod Chem. 56:173–228.
- Sun C, Wang Y, Sun S, Chen X, Shi X, Fang H, Zhang Y, Fang Z. 2020. Fragmentation pathways of protonated coumarin by ESI-QE-Orbitrap-MS/MS coupled with DFT calculations. J Mass Spectrom. 55(5):e4496.
- Toniolo C, Nicoletti M, Maggi F, Venditti A. 2014. HPTLC determination of chemical composition variability in raw materials used in botanicals. Nat Prod Res. 28(2):119–126.
- UNAIDS Report. 2020. Accessed in July 2021. http://aidsinfo.unaids.org.
- Venditti A, Bianco A. 2018. Secondary metabolites of Hypericum richeri Vill. collected in Central Italy: chemotaxonomy and ethnomedicinal relevance. Trends Phytochem Res. 2:155–162.
- Zhuang C, Pannecouque C, De Clercq E, Chen F. 2020. Development of non-nucleoside reverse transcriptase inhibitors (NNRTIs): our past twenty years. Acta Pharm Sin B. 10(6):961–978.