ABSTRACT
The world energy requirement has skyrocketed radically in the past two decades raising concern over energy security and adequacy. Energy resource planning plays a substantial role in the global economy and geopolitics of clean energy to achieve global commitment towards sustainable development. It includes strategic management, control and storage of energy supply. Microgrids contribute another layer to the apparent advantages of renewables by boosting system performance and mitigating energy losses. The prospects of energy resource management with the benefits of a hybrid microgrid are discussed here with a brief review of past research works on challenges encountered in voltage and frequency regulations with low-inertia issues, demand response, renewable system integration with transition conditions, and tuning controllers with metaheuristic optimisation techniques.
Introduction
The current focus of the global power sector is towards renewable power generation from all possible sustainable sources. The Ministry of New and Renewable Energy (MNRE), India, also exercises in this regard to enhance the generation capacity of the nation by renewable power generation programs both in off-grid and grid-connected modes (MNRE India Citation2018; CEA India Citation2019). The net installed renewable power (except large Hydel power) connected to the grid shares 24.28% of the total installed capacity of India as of 31 December 2020 (MNRE India Citation2018) as presented in (a). The sharing of individual grid-interactive renewable power is presented in (b). As depicted in (CEA India Citation2019), the progress of renewable energy generation in India from the year 2014 (17.28%) to 2020 (21.25%) is not much convincing as India intends wind and solar power to achieve a share of 36% of the total installed capacity by 2030 (IEA Citation2020). Inflated demand for power across the globe due to the increasing population and detrimental consequences on the environment due to the proliferation of waste encourages to search for an alternative strategy for the generation of sustainable green energy using waste. Therefore, new and potentially renewable resources should be explored to address India’s energy security challenges and fulfil the commitments made under Paris Agreement i.e. reduction of CO2 emission and generation of 40% of India’s power demand from the non-fossil-based sources (Mathur and Singh Citation2019).
Figure 1. Grid-connected installed capacity in India: (a) Installed capacity, (b) Interactive renewable power as of December 2020 (MNRE India Citation2018).
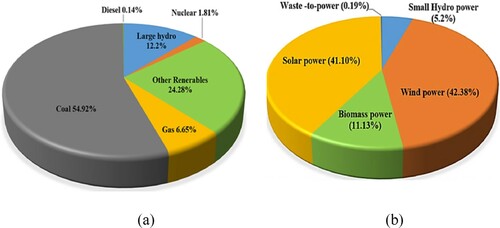
Table 1. Annual renewable power generation (GWh) (CEA India Citation2019).
Though worldwide electricity access has been increasing gradually (17% in 1990–87% in 2016), by 2016 around 940 million people did not have access to electricity to live a sustainable and decent living standard (Ritchie and Roser Citation2019).
As per International Energy Agency report a staged categorisation to record the evolving results that Variable renewable energy (VRE) integration may have on power systems prioritising different measures to support system flexibility is shown in . These stages also provide an assessment framework to depict the present and future trends in system integration of renewables in which India has been indexed in Phase 2. A coordinated strategy can dramatically reduce integration costs with ensured electricity security encountering three main areas: System-friendly deployment to maximise the exclusive benefit of wind and solar power, advanced operating strategies in order to ensure system security and Investment in additional flexible resources (IEA Citation2020).
In the present situation, most rural communities and coastal areas around the world are far from the main electricity supply. Community-based clean energy microgrids using renewable and bioenergy in such areas are developed to obtain sustainable clean energy from biodegradable waste and harvested power crops (Che et al. Citation2015; Tucho and Nonhebel Citation2017). The traditionally isolated renewable grids with Solar thermal power (STP) (Das, Roy, and Sinha Citation2012; Das, Sinha, and Roy Citation2014), Solar photovoltaic array (SPV) (Sundaray et al. Citation2014) as well as wind turbine generator (WTG) utilises Diesel engine generator (DEG) for optimised performance as solar irradiance and wind speed are extremely susceptible to climatic conditions (El-Fergany and El-Hameed Citation2017). Besides that, diesel is among the most replenishing fuels but also its effect on carbon emissions causes global warming. We therefore need to find some environmentally friendly renewable substitutes, such as bioenergy. MNRE has recently introduced programs to promote bioenergy cogeneration with SPV array (MNRE India Citation2017a). Even as power produced by bioenergy solely may not be adequate to supply the load of a specific region, groupings of small community-based hybrid microgrids (hμGs) that assimilate all potential renewable/bioenergy units could be a better alternative. Bioenergy harnessing strategies will reduce/reuse waste while generating green energy simultaneously. The rural societies can be encouraged by rendering services to yield one's electricity demand with the Hybrid Microgrid (hμG) based on Wind/Solar-bio-power cogeneration. Towards this, several schemes emulated by the Government of India to advance renewable/bioenergy coproduction (MNRE India Citation2017b).
With an objective to review the state of art in strategies of energy resource management to encounter the challenges of the hybrid microgrid in order to combat the inflated demand with sustainable energy targets, the paper also highlights some recent methods for demand/supply-side managements, reactive power control of hybrid microgrids. It also addresses the appropriate metaheuristic optimisation methods to harmonise controller parameters of traditional to modern controller for smooth regulation of voltage and frequency to obtain system stability in both isolated and interconnected microgrids.
The major concerns of the work are:
Classifying potential challenges in sustainable energy-based hybrid microgrids.
A thorough literature survey on recent works to address those challenges.
Ascertaining some potential research scope focusing on the challenges of hybrid microgrid both in isolated and interconnected modes.
Proposal for sustainable energy-based hybrid microgrids using local bioenergy resources with available renewable sources.
Planning suitable demand response support for demand-side management and virtual inertia support for supply-side management in microgrid.
Representing metaheuristic optimisation techniques and their hybrids for fine-tuning controller towards voltage and frequency regulation in both the modes.
The paper is organised as follows: Section 2 highlights some potential challenges of hybrid microgrids. A thorough literature survey on recent works to address those challenges is covered in Section 3. Section 4 points out some of the potential research scope focusing on the challenges of hybrid microgrid. Then the green energy-based hybrid microgrid employing waste to energy cycle and strategic energy resource planning as per recent trends are discussed in Section 5. Conclusions are intended in the last section of the paper.
2. Challenges of hybrid microgrid
The hμGs incorporate the advantages of renewable and traditional power generation while at the same time compensating for its weaknesses. Renewable energy generation capacity can contribute to any percent of the overall peak load at hμG. In general, the higher the participation from renewable power, the higher the potential savings in fuel and operating costs. In addition, hµG delivers reduced long-term operating cost for higher capital cost and reduced total ownership cost over conventional power production (Saury and Tomlinson Citation2016). Despite higher level of recognition of hµGs, the development is not much convincing or expected evaluation into new power generation is happening at a very slow rate.
The major issues related to these hµGs are addressed below that craft challenges in optimal regulation of the system voltage and frequency:
Unpredictable intermittency of renewable sources like Solar and Wind with climate-dependency, creates difficulty to stabilise the hµGs including SPV, STP, and WTG units.
Disturbances due to excessive penetration of variable renewable generations in microgrid also adds challenge to manage the system stability.
Most of the microgrids use Diesel generators to support the RES units, whereas the mineral diesel is non-sustainable and depleting day-by-day.
Low-inertia in microgrids creates difficulty for handling disturbances during frequent supply/demand variation.
There is a necessity for an extensive literature survey to discover the scopes for these challenges which is presented in the next section.
3. Literature survey
Brief literature of advancements in research over a decade is reviewed herein next five subsections, highlighting the strategies to encounter the major challenges in energy resource planning of a hybrid microgrid.
3.1. Renewable energy-based hybrid microgrids and frequency control issues
Numerous works have been reported so far, concerning low-inertia-based hybrid power systems for automatic load-frequency control (ALFC) of microgrids including So-lar/Wind/Diesel generator with energy storage support, operating both in isolated and interconnected modes. Gupta et al. designed A time series-dependent linearised model of a hybrid power generation system with SPV, biogas comprising of a photovoltaic system, biogas, biomass (fuel-wood), a fossil fuel, small/micro-hydro, and a storage battery, reducing cost-effectiveness based on demand and future economic constraints (Gupta, Saini, and Sharma Citation2011). Rasul, Ault, and Sajjad (Citation2015) examined the purpose of basic anaerobic digesters for both the production of biogas to power a simple turbine generator as well as their feasibility in rural communities’ usage. Basrawi, Ibrahim, and Yamada (Citation2015) explored the ideal scale of the micro-gas turbine cogeneration in the wastewater treatment plant was in relation to its economic efficiency.
Tamrakar, Pandey, and Dubey (Citation2015) proposed an effluent-based hydroelectric plant and power production utilising municipality sewage with a thorough discussion. Raza et al. (Citation2013) proposed the design of the micro-hydro-turbine generator (MHTG) system with Archimedes turbine and analysed the efficiency of interconnecting the power generation into the national grid.
Nabi, Akhter, and Shahadat (Citation2006) discussed the development in vehicle emissions of traditional diesel fuel including diesel–biodiesel blends. Agarwal (Citation2007) reported the applications of Biofuels (ethanol and biodiesel such as methyl) as fuels to internal combustion engines. Basha, Gopal, and Jebaraj (Citation2009) recorded the analysis of the generation, ignition, emissions, and efficiency of biodiesel. Arbon (Citation2002) discussed methods and applications of various biomass power generation and biomass-fueled combined heat and power (BCHP) units worldwide. Rajasekhar et al. (Citation2015) discussed various techniques for urban solid waste disposal techniques and technology for producing power in BCHP, together with a significant drop in biomass waste from landfill sites.
However, obstacles to a stable supply of power with effective load-frequency regulation (LFC) of renewable energy-based microgrids have not yet been adequately discussed. Das, Roy, and Sinha (Citation2012) investigated the efficiency of Genetic algorithm(GA) optimised integral (I), proportional–integral (PI), and proportional–integral–derivative (PID) controllers regulating the frequency deviation within the Proposed Dish-Stirling Solar Thermal-Based Decentralised Hybrid Energy System Combined with Aqua Electrolyze (AE) Battery, Energy Storage System (BESS), Diesel Engine Generators (DEG), and Fuel Cells (FC). Guerrero et al. (Citation2011) recorded hierarchical regulation in droop-controlled AC/DC microgrids.
The ORC related medium-sized parabolic trough collector (PTC) (Das, Sinha, and Roy Citation2014) and Linear Fresnel reflector (LFR) type STP units are compared in Cau and Cocco (Citation2014), Accounting 56 percent lesser land-area demanded by LFR mode STP than in the same production rated by PTC type STP modules. The efficiency of 1MWe rated LFR type STP unit is stated in Cocco and Serra (Citation2015) using heat transfer fluid such as thermal oil. It promotes the incorporation of LFR over PTC-based STP for massively populated countries such as India to harvest higher solar energy utilising the lesser area.
3.2. Combined reactive power and frequency control in hybrid microgrids
In real-world-paradigm reactive power control, it is often essentially dependent on the grid's voltage regulation. The principle of reactive power regulation with voltage control of the independent hybrid energy system is discussed by Bansal and Bhatti (Citation2008). The detailed mathematical derivation for combined ALFC and AVR are described by Anderson and Fouad (Citation2008) and examples discussed by Saadat (Citation2009). Few works of combined voltage and frequency control of isolated systems (Rakhshani, Rouzbehi, and Sadeh Citation2009; Gupta, Chauhan, and Khanna Citation2014) and interconnected multi-area systems (Othman and El-Fergany Citation2018) are reported recently for a nominal system frequency (f) of 60 Hz. Rakhshani, Rouzbehi, and Sadeh (Citation2009) described the coupling effects of both the AVR and LFC cycles by expanding the generalised linear AGC scheme to incorporate the excitation system and evaluated the suggested combined model on the single-area power grid. Gupta, Chauhan, and Khanna (Citation2014) addressed the integrated control for one area power system with automatic voltage regulation and damper winding, analysing the PI controller within AVR and the integral controller through the ALFC loop.
3.3. Low-inertia issues in microgrids and demand response supports
Recently some works have been reported several methods to handle low-inertia issues. The adjustable (Yang et al. Citation2019) and derivative technique (Fini and Golshan Citation2018; Kerdphol and Rahman Citation2019) based virtual inertia control units are considered with supercapacitors for frequency regulation of microgrids. The detailed overview of virtual synchronous generators (VSG) integrated to microgrids and power grids are discussed in Bevrani et al. (Citation2014) with their applications. A VSG model is reported in Nogami et al. (Citation2019) to improve transient stability/damping in large systems involving solar photovoltaic (SPV) units. A VSG-based inverter control approach is reported to improve the system dynamics in Khajehoddin, Karimi-Ghartemani, and Ebrahimi (Citation2018). A fast-acting inverter and storage-based VSG is developed to damp the oscillation of a DG in Shintai, Miura, and Ise (Citation2014).
Some recent works are being reported using DRS (Bao et al. Citation2015, Citation2017) for LFC of isolated/interconnected hybrid systems, whereas most of the works have been reported with ESS support (Das, Roy, and Sinha Citation2012; Das, Sinha, and Roy Citation2014; El-Fergany and El-Hameed Citation2017). Wang, Zhang, and Zhou (Citation2017) discussed the under-voltage along with under frequency load shedding approach focused on the actively engaging smart appliances. Dahraie et al. (Citation2017) reported coordination of electric vehicle contribution for LFC in islanded microgrids. Bao et al. introduced a hybrid stratified DR control strategy to support frequency regulation with precise specifications setting for each DR controller, and suggested a systematic design of parameters such as that of the frequency threshold and also the least off-time for particular DR controllers (Bao et al. Citation2015). They also implemented the DR frequency control technique within a multi-area power system and incorporated the tie-line power as that of an alternate DR input source in order to instantly maintain the frequency of various areas in the system (Bao et al. Citation2017). Pourmousavi et al. stated use of adaptive DR in the LFC system (Pourmousavi and Nehrir Citation2014) including real-time central DR for primary frequency regulation in microgrids (Pourmousavi and Nehrir Citation2012). Whereas, Zakariazadeh et al. (Citation2014) asserted a different strategy with real-time voltage regulation employing DR in an intelligent distribution system.
3.4. Controllers used in hybrid microgrids
Controllers play a vital role in the coordination of overall system responses where the tuning of the controller parameter is most sensitive in this regard. The hµGs are modelled using controllers for ALFC and AVR to regulate the overall performances. Most of the literature published on hμG LFCs either used the traditional proportional–integral–derivative (PID) (Das, Roy, and Sinha Citation2012; Das, Sinha, and Roy Citation2014; El-Fergany and El-Hameed Citation2017) controllers or comparing the behaviours of new controllers such as model predictive control scheme (Othman and El-Fergany Citation2018), fuzzy adaptive model predictive controllers (Kayalvizhi and Kumar Citation2017), and fractional-order controllers (Wang, Zeng, et al. Citation2017; Khooban et al. Citation2018) with PID responses to validate the proposed one. Also, the PID controller is very stable, cheaper, and effortlessly programmed to connect with tuning gain optimisation techniques as stated and described in many literatures.
3.5. Optimisation techniques for implementing control strategy in hybrid microgrids
There was a wide application of metaheuristic optimisation algorithms reported in most of the works in this area, to tune the classical as illustrated in . Mohanty, Panda, and Hota (Citation2014) reported the tuning of controller parameters using the differential evolutionary algorithm applied to LFC of the decentralised power system. Wang, Zeng, et al. (Citation2017) reported the design of a fractional-order frequency PID (FOPID) controller for an isolated hµG using a multi-objective extremal optimisation method. Pourmousavi et al. (Citation2010) discussed real-time energy management of a stand-alone hybrid wind-microturbine energy system using PSO. (c) S. Mirjalili reported some recent nature-inspired optimisation algorithms like Moth-flame optimisation (MFO) (Mirjalili Citation2015) and Salp-swarm algorithm (SSA) (Mirjalili Citation2017) individually, whereas Mirjalili, Mirjalili, and Lewis (Citation2014) reported Grey-wolf optimisation (GWO), Saremi, Mirjalili, and Lewis (Citation2017) reported Grasshopper optimisation algorithm (GOA). Tripathy et al. (Citation2019) applied GOA in LFC of multi-area power system successfully to tune fuzzy controllers. Guha, Roy, and Banerjee (Citation2016) reported the LFC of interlinked power systems with classical PI/PID controllers using GWO. Lal, Bhoi, and Barisal (Citation2016) reviewed the output of many classical controllers, such as I, PI, PID, IDD, and PID with the AGC second-order derivative filter for multiple area power systems employing MFO. A novel swarm-based algorithm called Selfish-Herd Optimiser (SHO) has recently been suggested by Fausto et al. (Citation2017) to solve the challenges of global optimisation. SHO is founded on a simulation of the generally recognised selfish-herd behaviour of an individual within each herd of animals exposed to certain predation risk. The framework of the tune controller benefits from SHO in microgrid applications that have not yet been used in the LFC analysis.
A few hybrid optimisation techniques have recently been demonstrated to boost the efficiency of simple algorithms such as Saha and Mukherjee (Citation2018), a quasi-oppositional chaotic antlion optimisation technique that hybridises quasi-counter-based investigation as well as chaotic linear search (CLS) via antlion optimisation technique, Shankar and Mukherjee (Citation2016) proposed Quasi-oppositional harmony search algorithm by hybridising Harmony search algorithm with Quasi-opposition based learning (QOBL) for LFC analysis. Those inspires to hybridise SHO in hµG applications. Sahoo, Routray, and Rout (Citation2020) discussed the microgrid control hierarchy in three segments as primary, secondary, and tertiary strategies for AC/DC/hµGs structures indicating the highpoints of state-of-art control methods such as intelligent control, synchronised and evolutionary approach of hµGs with specific benefits and demerits.
4. Current research scopes in hybrid microgrids
Many researchers recommended various robust control methods, inverter topologies, storage designs, load supervision and optimisation strategies to enhance the performance of microgrids underlining the scope of the future trends in the area of research applicable to energy resource planning are summarised here as in .
Some research gaps are identified from the above extensive literature reviews and the following research scopes are anticipated to be explored in this work.
The diesel generation units (DEG) are being used in most of the reported works on hµGs to support SPV/WTG type renewable units; however, the fuel used in DEG (i.e. Diesel) is a depleting resource itself. Hence, there is a great scope to explore some bio-renewable resource-based generating units that could provide sustainable power by waste-to-energy concept to be included in such hµGs.
The frequency regulations of such systems are mostly investigated using ALFC concept, whereas very few works based on combined voltage and frequency control concept are investigated using both ALFC and AVR so far. Hence, there is a wide scope to investigate the combined voltage and frequency control in the suggested hµGs.
The performances of such hµGs are highly affected due to intermittency of so-lar/wind based renewable resources and the uncertain load variations. Hence, there is an open scope for managing the power in such microgrids either from supply or demand side with the help of suitable support systems.
The controller gains are tuned with several metaheuristic optimisation algorithms to minimise the error function in similar works as mostly reported hitherto. There is abundant scope for developing hybrid optimisation algorithms to investigate the optimal control of voltage and frequency in the suggested hµGs.
5. Proposed green energy-based hybrid microgrid
5.1. Waste-to-energy cycle
Motivated by the above-discussed existing research, a bioenergy-based waste-to-energy cycle is conceptualised as presented in , associated with the recommendation of hμG, along with the Bioenergy Generation System (BEGS), the Renewable Energy System (RES) and the Virtual-Inertia Support (VIS) system/demand-response support (DRS) system. It is expected that all bio-waste including sewage from each house in community/locality house should be extracted then checked for the reduce–reuse–recycle procedure. Community waste should then be segregated, thoroughly pre-treatment to be consumed in the corresponding BEGS, as well as the by-products should therefore be treated to be used in organic farming or to aid either waste-processing or land filling. Crops from eco-agriculture are being used by the community and produce wastes thus completes the cycle as shown in .
The effluent of each household, together with the rainwater of a particular locality region, may be channeled to a typical sewage canal and deposited in a small repository (in the outskirts of the community) for the drainage of dissolved waste and the prior treatment of water. A low headed dam could also be installed upon this reservoir, and its power could well be produced by employing MHTG units from the wastewater treatment until it flows into the mainstream (Tamrakar, Pandey, and Dubey Citation2015). Such discharged-waters could be used for cultivation in agricultural land by irrigation. Non-recyclable waste can be used for generating power in the BCHP plant following proper processing and combining with generic biomass (like agricultural residues) (Arbon Citation2002). Residues are limited to a small percentage of the original waste and can be used for dumpsites, thus lowering the risk for local environmental pollution. Biogas is generated by the anaerobic digestion process or fermentation of bioenergy-crops as well as biodegradable waste, such as domestic sewage, kitchen scraps, agro-waste, dead plants, or animals’ residues (Rasul, Ault, and Sajjad Citation2015), that is used in the Biogas turbine generator (BGTG) system. Biodiesel is another Environment friendly, non-toxic, high flash point diesel fuel produced by the transesterification process of sugar, vegetable oils, or starch received from energy crops and livestock (Nabi, Akhter, and Shahadat Citation2006). Biodiesel is used in a Biodiesel engine generator (BDEG) unit. It has comparable characteristics to mineral diesel mostly with the benefit of having improved lubrication properties, lower sulfur level, and lower toxic emissions (Agarwal Citation2007). Residues of biofuels and biodiesel plants may be further refined for use as bio-fertilisers in organic farming.
5.2. Energy resource planning in hybrid microgrids
The aim of such hμG would be to have an economic energy generation in order to fulfil the needs of the consumer in a healthy, sustainable and resilient manner that requires energy resource planning. The comprehensive strategy for the deployment of microgrid energy planning is discussed in Kumar, Ali, and Thanki (Citation2018). However, the latest research pattern is more focused on Integrated Resource Planning (IRP) as outlined in .
The RES comprises the Solar Thermal Power (STP) unit, the Solar Photovoltaic Array (SPV) panel, and the Wind Turbine Generator (WTG) unit that uses accessible solar and wind energy, separately for electricity generation depending on specific climatic conditions (Das, Roy, and Sinha Citation2012; Das, Sinha, and Roy Citation2014). High penetration of renewable generations or relatively higher load fluctuations is the primary reason in autonomous hμGs of system frequency fluctuations due to limited inertia (Fini and Golshan Citation2018; Kerdphol and Rahman Citation2019; Shintai, Miura, and Ise Citation2014). Therefore, it is vital to provide inertia support to those microgrids virtually with a suitable energy storage system (ESS) and bidirectional power convertors. Recent works have been successfully used supercapacitors as ESS in VIS design (Yang et al. Citation2019; Bevrani et al. Citation2014) to provide active and reactive power support in large systems. This motivates to propose a virtual inertia support (VIS) system with suitable ESS for supply-side management in waste-to-energy-based hµG as illustrated in .
The ESS may include some combination of Battery energy storage (BES) unit (Das, Roy, and Sinha Citation2012), Flywheel energy storage (FES) unit (Das, Roy, and Sinha Citation2012), Super-magnetic energy storage (SES) unit (Sundaray et al. Citation2014), Super-capacitor based capacitive energy storage (CES) unit (Das, Roy, and Sinha Citation2012), Fuel-cell (FC) and Aqua electrolyzer (AE) units (Das, Roy, and Sinha Citation2012) aid for hμG throughout regular load fluctuations. In several cases, it is quite difficult to assign a sufficient landscape for both the deployment of all BEGS units and RES units next to one another to be attached to a single hμG for insulated/stand-alone service. In such case scenarios, the necessary units accessible at one location could build distributive hμGs, and then all these hμGs could be interlinked to serve the community's desired power demand with the assistance of ESS/DRC. Besides the critical loads within microgrids, there are certain electrical appliances such as freezers/refrigerators (FR), water heaters (WH), hybrid electric vehicles (HEV), and heat pumps (HP), etc., which are considered as inessential loads which could be used to contribute to the demand response (DR). The aggregation of any of these accessible DR devices that support microgrids in frequency regulation by temporarily raising or reducing their power consumption might be referred to as the DRS units (Bao et al. Citation2015, Citation2017).
The intermittence of renewable energy attributable to changes in climate is a significant obstacle for the microgrid to fulfil the changing demands with the reliable power supply to connected customers. Clean energy must be supplied to consumers under the acceptable voltage and frequency limits of their nominal values. The Deviation in load frequency and voltage due to fluctuations in demand and climatic anomalies tends to unstable and inefficient performance of the grid and impairs the efficiency of power generation. The reliability of the power grid may be controlled by preserving the system's voltage and frequency within the permitted operating limits. The voltage deviation (Δu) is regulated by the excitation of the generator whereas load-frequency deviation (Δf) is regulated by a governor. So, essentially, the generator controller has two loops of control. These are the automatic load-frequency control (ALFC) loop, which governs the frequency by controlling the active power, and another one is the automatic voltage control (AVR) loop, which governs the terminal voltage by trying to coordinate the system's reactive power (Kundur Citation2009). Although the time constants of the AVR loop are smaller than those of the ALFC loop whereas, the AVR loop is rapid than that of the ALFC loop. As a result, several researchers have neglected to link these loops. However, there is still a slight cross-coupling between the same two loops in a realistic situation (Anderson and Fouad Citation2008; Saadat Citation2009). Voltage and frequency variations are due to variance in load demand in actual power systems. In order to control both frequency and voltage, a hybrid ALFC and AVR model needs to be investigated for islanded and interconnected hμGs, to concurrently control the frequency and voltage of the system. The overall control block model of such hµG could be illustrated as in , for restoring power quality with coordinated voltage-frequency regulation including IRP as discussed earlier in .
5.3. State-of-the-art integrated resource planning for hybrid microgrids
Recently, the green energy-based hµGs are proposed considering all available BEGS and RES units for IRP as discussed above. A community-based hµG with SPV, BDEG, and BGTG units including BES is reported in Barik and Das (Citation2018b) for efficient frequency control using GOA in various real-world situations of renewable resource accessibility and load variance. Similarly, the utilisation of solid and liquid urban waste for power generation in BCHP and MHTG respectively, is, reported in Barik and Das (Citation2018a) to support the hµG with roof-top SPV units including AE and FC units for frequency regulation by SSA. The frequency oscillation of similar hµGs due to over-penetration of RES units are proficiently regulated in (Barik and Das Citation2019) with HEV charging station-based DRS unit both in isolated and interconnected modes by proposing a novel Quasi-oppositional selfish-herd optimisation (QSHO). Similarly, DRS-supported interconnected hµGs with PTC type STP units have successfully regulated the frequency in Barik and Das (Citation2018c) whereas, DRS-based 4-unequal interconnected hµGs have successfully regulated the system frequency in Barik, Das, and Muduli (Citation2019) considering the simultaneous source and variable load. Some recent works on frequency regulation of interconnected hµGs are reported using GOA (Bhuyan, Barik, and Das Citation2020), Sine-cosine algorithm (SCA) (Bhuyan, Das, and Barik Citation2019, Citation2020), SHO (Sahoo, Barik, and Das Citation2020), Water cycle optimisation (WCO) (Latif, Das, Ranjan, et al. Citation2019), Yellow saddle-fish goat optimisation (YSG) (Latif et al. "Illustration of Demand", Citation2019), and Butterfly optimisation algorithm (BOA) (Latif et al., "Maiden co-Ordinated Load Frequency", Citation2019).
Optimal modulation of voltage and frequency in green energy-based hμG has been thoroughly investigated in Barik and Das (Citation2020a) considering demand-side management DRS unit for QSHO tuned PID controllers. That hμG includes RES units such as LFR type SPV, STP, and WTG units for optimum use of locally available renewable resources, along with BEGS units such as BCHP, BGTG, MHTG, and BDEG units to support them. Whereas, a combined storage-based VIS unit is proposed for supply-side management of interconnected hµGs to overcome the low-inertia issues in Barik and Das (Citation2020b) by supporting the microgrid with virtual inertia during larger source/load disturbances. Recently, some modern controllers like fuzzy (Barik and Das Citation2020b; Barik et al. Citation2020), 2-degrees of freedom PID (Tripathy et al. Citation2020), fractional-order PID (Wang, Zeng, et al. Citation2017; Khooban et al. Citation2018), model predictive (Othman and El-Fergany Citation2018) and their blends (Kayalvizhi and Kumar Citation2017; Latif et al. "Illustration of Demand", Citation2019; Latif et al., "Maiden co-Ordinated Load Frequency", Citation2019) are reported for LFC, which paves a path towards designing some new/hybrid controllers for similar sustainable energy-based hµGs and investigate their responses. The power supplied to the consumer from these sustainable hµGs should be monitored continuously and preventive measures (Barik, Tripathy, and Mohanty Citation2013) to be adapted for reliable quality power supply.
6. Conclusion
The proposed hµG bio- energy model incorporating all BEGS, DRS, VIS, and RES units for IRP briefly highlights its prospects as an alternative strategy in energy resource planning aiding resource availability and combating the detrimental consequences of the proliferation of waste on the environment. The future scope of ongoing research in this area while facing challenges in operating isolated as well as interconnected modes of hµGs is discussed. The gap in research is addressed in operating system transition challenges in replacing DEG by waste -to energy concept with the obstacle in a stable supply of power with effective load-frequency regulation (LFC). The optimal regulation of the system voltage and frequency with appropriate metaheuristic optimisation to harmonise controller parameters of traditional to a modern controller and their hybrids, incorporation of suitable VIS and DRS units and VSG for low-inertia control, active and passive energy-based energy storage control strategies are necessary to boost system efficiency meanwhile integrating variable renewable energy in the power system. This work could be beneficial for all the researchers working towards sustainable energy development in the field of Energy Engineering, Environmental, and Social Engineering. Other renewable sources like geothermal, ocean-thermal, wave and tidal energy, etc. could be explored extending the work further including different flexible loads for DRS units and storage techniques for VIS units.
Acknowledgements
The authors would like to thank the Department of Electrical Engineering, NIT Silchar, for providing the required infrastructure and assistance for this research.
Disclosure statement
No potential conflict of interest was reported by the author(s).
References
- Agarwal, A. K. 2007. “Biofuels (Alcohols and Biodiesel) Applications as Fuels for Internal Combustion Engines.” Progress in Energy and Combustion Science 33 (3): 233–271.
- Anderson, P. M., and A. A. Fouad. 2008. Power System Control and Stability, 309–366. 2nd ed.. Piscataway, N.J.: John Wiley & Sons.
- Arbon, I. M. 2002. “Worldwide use of Biomass in Power Generation and Combined Heat and Power Schemes.” Journal of Power and Energy 216 (1): 41–57.
- Bansal, R. C., and T. S. Bhatti. 2008. Small signal analysis of isolated hybrid power systems: reactive power and frequency control analysis. Vol. 1, 20–179. Oxford United Kingdom: Alpha Science.
- Bao, Y. Q., Y. Li, B. Wang, and Y.-Y. Hong. 2015. “Design of a Hybrid Hierarchical Demand Response Control Scheme for the Frequency Control.” IET Generation, Transmission & Distribution 9 (15): 2303–2310.
- Bao, Y. Q., Y. Li, B. Wang, M. Hu, and P. Chen. 2017. “Demand Response for Frequency Control of Multi-Area Power System.” Journal of Modern Power System & Clean Energy 5 (1): 20–29.
- Barik, A. K., and D. C. Das. 2018a. “Active Power Management of Isolated Renewable Microgrid Generating Power from Rooftop Solar Arrays, Sewage Waters and Solid Urban Wastes of a Smart City Using Salp Swarm Algorithm.” In International Conference on Technologies for Smart-City Energy Security and Power (ICSESP). Bhubaneswar.
- Barik, A. K., and D. C. Das. 2018b. “Expeditious Frequency Control of Solar Photovoltaic/Biogas/Biodiesel Generator Based Isolated Renewable Microgrid Using Grasshopper Optimisation Algorithm.” IET Renewable Power Generation 1214: 1659–1667.
- Barik, A. K., and D. C. Das. 2018c. “Optimal Load-Frequency Regulation of Bio-Renewable Cogeneration Based Interconnected Hybrid Microgrids with Demand Response Support.” In 15th IEEE India Council International Conferences (INDICON). Coimbatore.
- Barik, A. K., and D. C. Das. 2019. “Proficient Load-Frequency Regulation of Demand Response Supported bio-Renewable Cogeneration Based Hybrid Microgrids with Quasi-Oppositional Selfish-Herd Optimisation.” IET Generation, Transmission & Distribution 13 (13): 2889–2898.
- Barik, A. K., and D. C. Das. 2020a. “Coordinated Regulation of Voltage and Load Frequency in Demand Response Supported bio Renewable Cogeneration-Based Isolated Hybrid Microgrid with Quasi-Oppositional Selfish Herd Optimisation.” International Transactions on Electrical Energy Systems 30 (1): e12176.
- Barik, A. K., and D. C. Das. 2020b. “A Storage Based Virtual Synchronous System for Load-Frequency Regulation of Interconnected Hybrid Microgrids Using Selfish-Herd Optimiser.” In IEEE International Conference on Renewable Energy Integration Into Smart Grids (ICREISG). Bhubaneswar.
- Barik, A. K., D. C. Das, and R. Muduli. 2019. “Demand Response Supported Optimal Load-Frequency Regulation of Sustainable Energy Based Four-Interconnected Unequal Hybrid Microgrids.” In IEEE International Conference on Sustainable Energy Technologies and Systems (ICSETS). Bhubaneswar.
- Barik, A. K., D. Tripathy, D. C. Das, and S. C. Sahoo. 2020. “Optimal Load-Frequency Regulation of Demand Response Supported Isolated Hybrid Microgrid Using Fuzzy PD + I Controller.” Intelligent Techniques and Applications in Science and Technology, Learning and Analytics in Intelligent Systems 12: 798–806. Springer International Publishing.
- Barik, A. K., D. Tripathy, and A. K. Mohanty. 2013. ““Detection & Mitigation of Power Quality Disturbances Using WPT & FACTS Technology.” International Journal of Science Engineering & Technology Research 2 (2): 336–343.
- Basha, S. A., K. R. Gopal, and S. Jebaraj. 2009. “A Review on Biodiesel Production, Combustion, Emissions and Performance.” Renewable and Sustainable Energy Reviews 13 (6): 1628–1634.
- Basrawi, F., H. Ibrahim, and T. Yamada. 2015. “Optimal Unit Sizing of Biogas-Fuelled Micro Gas Turbine Cogeneration Systems in a Sewage Treatment Plant.” Energy Procedia 75: 1052–1058.
- Bevrani, H., T. Ise, Y. Miura, and M. Watanabe. 2014. “Virtual Synchronous Generators: A Survey and new Perspectives.” International Journal of Electric Power & Energy Systems 54: 244–254.
- Bhuyan, M., A. K. Barik, and D. C. Das. 2020. “GOA Optimized Frequency Control of Solar Thermal/Sea-Wave/Biodiesel Generator Based Interconnected Hybrid Microgrids with DC Link.” International Journal of Sustainable Energy 39 (7): 615–633.
- Bhuyan, M., D. C. Das, and A. K. Barik. 2019. “A Comparative Analysis of DSM Based Autonomous Hybrid Micro Grid Using PSO and SCA.” In The IEEE Region 10 Symposium (TENSYMP-2019), 765–770. Kolkata.
- Bhuyan, M., D. C. Das, and A. K. Barik. 2020. “Sine-cosine Algorithm Based Automatic Load Frequency Control of Hybrid Microgrid with Demand Side Management.” Intelligent Techniques and Applications in Science and Technology, Learning and Analytics in Intelligent Systems 12: 505–515.
- Cau, G., and D. Cocco. 2014. “Comparison of Medium-Size Concentrating Solar Power Plants Based on Parabolic Trough and Linear Fresnel Collectors.” Energy Procedia 45: 101–110.
- CEA India. 2019. “Summary of All India Provisional Renewable Energy Generation.” [Online]. Accessed April 27, 2020. http://www.cea.nic.in/reports/monthly/renewable/2019/renewable-03.pdf.
- Che, L., X. Zhang, M. Shahidehpour, A. Alabdulwahab, and A. Abusorrah. 2015. “Optimal Interconnection Planning of Community Microgrids with Renewable Energy Sources.” IEEE Transactions on Smart Grid 8 (3): 1054–1063.
- Cocco, D., and F. Serra. 2015. “Performance Comparison of two-Tank Direct and Thermocline Thermal Energy Storage Systems for 1MWe Class Concentrating Solar Power Plants.” Energy 81: 526–536.
- Dahraie, M. V., H. R. Kermani, H. Najafi, A. A. Moghaddam, and J. Guerrero. 2017. “Coordination of EVs Participation for Load Frequency Control in Isolated Microgrids.” Applied Sciences 7 (6): 539.
- Das, D. C., A. K. Roy, and N. Sinha. 2012. “GA Based Frequency Controller for Solar Thermal– Diesel–Wind Hybrid Energy Generation/Energy Storage System.” International Journal of Electrical Power & Energy Systems 43 (1): 262–279.
- Das, D. C., N. Sinha, and A. K. Roy. 2014. “Automatic Generation Control of an Organic Rankine Cycle Solar–Thermal/Wind–Diesel Hybrid Energy System.” Energy Technology 2 (8): 721–731.
- El-Fergany, A. A., and M. A. El-Hameed. 2017. “Efficient Frequency Controllers for Autonomous Two-Area Hybrid Microgrid System Using Social-Spider Optimiser.” IET Generation, Transmission & Distribution 11 (3): 637–648.
- Fausto, F., E. Cuevas, A. Valdivia, and A. González. 2017. “A Global Optimization Algorithm Inspired in the Behavior of Selfish Herds.” Biosystems 160: 39–55.
- Fini, M. H., and M. E. H. Golshan. 2018. “Determining Optimal Virtual Inertia and Frequency Control Parameters to Preserve the Frequency Stability in Islanded Microgrids with High Penetration of Renewables.” Electric Power System Research 154: 13–22.
- Guerrero, J. M., J. C. Vasquez, J. Matas, L. G. DeVicuña, and M. Castilla. 2011. “Hierarchical Control of Droop-Controlled AC and DC Microgrids-A General Approach Toward Standardization.” IEEE Transactions on Industrial Electronics 58 (1): 158–172.
- Guha, D., P. K. Roy, and S. Banerjee. 2016. “Load Frequency Control of Interconnected Power System Using Grey Wolf Optimisation.” Swarm and Evolutionary Computing 27: 97–115.
- Gupta, A., A. Chauhan, and R. Khanna. 2014. “Design of AVR and ALFC for Single Area Power System Including Damping Control.” In IEEE Conference on Recent Advances in Engineering and Computer Sciences 2014, Chandigarh, India.
- Gupta, A., R. P. Saini, and M. P. Sharma. 2011. “Modelling of Hybrid Energy System – Part I: Problem Formulation and Model Development.” Renewable Energy 36 (2): 459–465.
- IEA. 2020. India 2020 Energy Policy Review. Accessed January 10, 2020. https://www.iea.org/reports/india-2020.
- Kayalvizhi, S., and D. V. Kumar. 2017. “Load Frequency Control of an Isolated Micro Grid Using Fuzzy Adaptive Model Predictive Control.” IEEE Access 5: 16241–16251.
- Kerdphol, T., and F. S. Rahman. 2019. “Robust Virtual Inertia Control of a low Inertia Microgrid Considering Frequency Measurement Effects.” IEEE Access 7: 57550–57560.
- Khajehoddin, S. A., M. Karimi-Ghartemani, and M. Ebrahimi. 2018. “Grid-supporting Inverters with Improved Dynamics.” IEEE Transactions on Industrial Electronics 66 (5): 3655–3667.
- Khooban, M. H., T. Niknam, M. Shasadeghi, T. Dragicevic, and F. Blaabjerg. 2018. “Load Frequency Control in Microgrids Based on a Stochastic Noninteger Controller.” IEEE Transactions on Sustainable Energy 9 (2): 853–861.
- Kumar, P., I. Ali, and D. V. Thanki. 2018. “Demand-Side Management: Energy Efficiency and Demand Response.” In Handbook of Research on Power and Energy System Optimization, edited by Pawan Kumar, Surjit Singh, Ikbal Ali, and Taha Selim Utsun, 453–479. USA: IGI Global.
- Kundur, P. 2009. In Power System Stability and Control, 2nd ed., edited by N. Balu, and M. G. Lauby, 309–366. New York: McGraw-Hill.
- Lal, D. K., K. K. Bhoi, and A. K. Barisal. 2016. “Performance Evaluation of MFO Algorithm for AGC of a Multi Area Power System.” In IEEE International Conference on Signal Processing, Communication, Power and Embeded Systems, Paralakhemundi.
- Latif, A., D. C. Das, A. K. Barik, and S. Ranjan. 2019. Illustration of Demand Response Supported Coordinated System Performance Evaluation of YSGA Optimized Dual Stage PIFOD-(1+ PI) Controller Employed with Wind-Tidal-Biodiesel Based Independent Two-Area Interconnected Microgrid System.” IET Renewable Power Generation 14 (6): 1074–1086.
- Latif, A., D. C. Das, A. K. Barik, and S. Ranjan. 2019. “Maiden co-Ordinated Load Frequency Control Strategy for ST-AWEC-GEC-BDDG Based Independent Three-Area Interconnected Microgrid System with Combined Effect of Diverse Energy Storage and DC Link Using BOA Optimized PFOID Controller.” IET Renewable Power Generation 13 (14): 1634–2646.
- Latif, A., D. C. Das, S. Ranjan, and A. K. Barik. 2019. “Comparative Performance Evaluation of WCA-Optimized Non-Integer Controller Employed with WPG-DSPG-PHEV Based Isolated 2-Area Interconnected Microgrid System.” IET Renewable Power Generation 13 (5): 725–736.
- Mathur, A. K., and S. Singh. 2019. “Status of India’s Renewable Energy Commitments for the Paris Agreement.” In 2019 International Conference on Power Generation Systems and Renewable Energy Technologies, Istanbul, Turkey.
- Mirjalili, S. 2015. “Moth-flame Optimization Algorithm: A Novel Nature-Inspired Heuristic Paradigm.” Knowledge-Based Systems 89: 228–249.
- Mirjalili, S. 2017. “Salp Swarm Algorithm: A Bio-Inspired Optimizer for Engineering Design Problems.” Advances in Engineering Software 114: 163–191.
- Mirjalili, S., S. M. Mirjalili, and A. Lewis. 2014. “Grey Wolf Optimizer.” Advances in Engineering Software 69: 46–61.
- MNRE India. 2017a. “Bio-Power India.” [Online]. Accessed October 27, 2017. http://mnre.gov.in/file-manager/biopower/BioPower-October-December-15-english.pdf.
- MNRE India. 2017b. “Biomass Power and Cogeneration Programme.” [Online]. Accessed October 27, 2017. http://mnre.gov.in/schemes/grid-connected/biomass-powercogen/.
- MNRE India. 2018. “Ministry of New and Renewable Energy, Physical Progress (Achievements).” [Online]. Accessed January 29, 2021. https://cea.nic.in/api/installed_capacity_allindia.php.
- Mohanty, B., S. Panda, and P. K. Hota. 2014. “Controller Parameters Tuning of Differential Evolution Algorithm and its Application to Load Frequency Control of Multi-Source Power System.” International Journal of Electrical Power & Energy Systems 54: 77–85.
- Nabi, M. N., M. S. Akhter, and M. M. Z. Shahadat. 2006. “Improvement of Engine Emissions with Conventional Diesel Fuel and Diesel-Biodiesel Blends.” Bioresource Technology 97 (3): 372–378.
- Nogami, S., A. Yokoyama, T. Daibu, and Y. Hono. 2019. “Virtual Synchronous Generator Model Control of PV for Improving Transient Stability and Damping in a Large-Scale Power System.” Electrical Engineering in Japan 208: 21–28.
- Othman, A. M., and A. A. El-Fergany. 2018. “Design of Robust Model Predictive Controllers for Frequency and Voltage Loops of Interconnected Power Systems Including Wind Farm and Energy Storage System.” IET Generation, Transmission & Distribution 12 (19): 4276–4283.
- Pourmousavi, S. A., and M. H. Nehrir. 2012. “Real-time Central Demand Response for Primary Frequency Regulation in Microgrids.” IEEE Transactions on Smart Grid 3 (4): 1988–1996.
- Pourmousavi, S. A., and M. H. Nehrir. 2014. “Introducing Dynamic Demand Response in the LFC Model.” IEEE Transactions on Power Systems 29 (4): 1562–1572.
- Pourmousavi, S. A., M. H. Nehrir, C. M. Colson, and C. Wang. 2010. “Real-time Energy Management of a Stand-Alone Hybrid Wind-Microturbine Energy System Using Particle Swarm Optimization.” IEEE Transactions on Sustainable Energy 1 (3): 193–201.
- Rajasekhar, M., N. V. Rao, G. C. Rao, G. Priyadarshini, and N. J. Kumar. 2015. “Energy Generation from Municipal Solid Waste by Innovative Technologies–Plasma Gasification.” Procedia Materials Science 10: 513–518.
- Rakhshani, E., K. Rouzbehi, and S. Sadeh. 2009. “A new Combined Model for Simulation of Mutual Effects Between LFC and AVR Loops.” In IEEE Asia-Pacific Power and Energy Engineering Conference 2009, Wuhan, China.
- Rasul, M. G., C. Ault, and M. Sajjad. 2015. “Bio-gas Mixed Fuel Micro gas Turbine co-Generation for Meeting Power Demand in Australian Remote Areas.” Energy Procedia 75 (1): 1065–1071.
- Raza, A., M. S. Main, D. Xu, and J. Ahmed. 2013. “A Micro Hydro Power Plant for Distributed Generation Using Municipal Water Waste with Archimedes Screw.” In 16th International Multi Topic Conference, Lahore, Pakistan.
- Ritchie, H., and M. Roser. 2019. “Access to Energy.” [Online]. https://ourworldindata.org/energy-access.
- Saadat, H. 2009. Power System Analysis, 527–576. 2nd ed. McGraw-Hill Higher Education.
- Saha, S., and V. Mukherjee. 2018. “A Novel Quasi-Oppositional Chaotic Antlion Optimizer for Global Optimization.” Applied Intelligence 48 (9): 2628–2660.
- Sahoo, S. C., A. K. Barik, and D. C. Das. 2020. “Selfish-herd Optimisation Based Frequency Regulation in Combined Solar-Thermal and Biogas Generator Based Hybrid Microgrid.” In IEEE International Conference on Contemporary Computing and Applications (ICCCA-2020), 1–6. Lucknow.
- Sahoo, B., S. K. Routray, and P. K. Rout. 2020. “AC, DC, and Hybrid Control Strategies for Smart Microgrid Application: A Review.” International Transactions on Electrical Energy System 31 (1). https://doi.org/https://doi.org/10.1002/2050-7038.12683.
- Saremi, S., S. Mirjalili, and A. Lewis. 2017. “Grasshopper Optimisation Algorithm: Theory and Application.” Advances in Engineering Software 105: 30–47.
- Saury, F. X., and C. Tomlinson. 2016. “Hybrid Microgrids: The Time is now.” Caterpillar Inc 1 (2): 1–12.
- Shankar, G., and V. Mukherjee. 2016. “Quasi Oppositional Harmony Search Algorithm Based Controller Tuning for Load Frequency Control of Multi-Source Multi-Area Power System.” International Journal of Electric Power & Energy Systems 75: 289–302.
- Shintai, T., Y. Miura, and T. Ise. 2014. “Oscillation Damping of a Distributed Generator Using a Virtual Synchronous Generator.” IEEE Transactions on Power Delivery 29 (2): 668–676.
- Sundaray, S., L. Mann, U. Bhattacharjee, S. Garud, and A. K. Tripathi. 2014. Reaching the Sun with Rooftop Solar. New Delhi: TERI.
- Tamrakar, A., S. K. Pandey, and S. C. Dubey. 2015. “Hydro Power Opportunity in the Sewage Waste Water. American International Journal of Research in Science, Technology, Engineering & Mathematics 10 (2): 179–183.
- Tripathy, D., A. K. Barik, N. B. Dev Choudhury, and B. K. Sahu. 2019. “Performance Comparison of SMO-Based Fuzzy PID Controller for Load Frequency Control.” In Soft Computing for Problem Solving, edited by Bansal J.C., Das K.N., Nagar A., Deep k., and Ojha A.K,879–892. Singapore: Springer.
- Tripathy, D., A. K. Barik, N. B. Dev Choudhury, and B. K. Sahu. 2020. “Comparative Analysis of FACTS Coordinated Hybrid Power System with RFB for AGC Using GOA Based F-2DOF-PID Controller.” Intelligent Techniques and Applications in Science and Technology, Learning and Analytics in Intelligent Systems 12: 209–218. Springer International Publishing.
- Tucho, G. T., and S. Nonhebel. 2017. “Alternative Energy Supply System to a Rural Village in Ethiopia.” Energy, Sustainability and Society 7 (1): 33.
- Wang, H., G. Zeng, Y. Dai, D. Bi, J. Sun, and X. Xie. 2017. “Design of a Fractional Order Frequency PID Controller for an Islanded Microgrid: A Multi-Objective Extremal Optimization Method.” Energies 10 (10): 1502.
- Wang, J., H. Zhang, and Y. Zhou. 2017. “Intelligent Under Frequency and Under Voltage Load Shedding Method Based on the Active Participation of Smart Appliances.” IEEE Transactions Smart Grid 8 (1): 353–361.
- Yang, L., Z. Hu, S. Xie, S. Kong, and W. Lin. 2019. “Adjustable Virtual Inertia Control of Supercapacitors in PV-Based AC Microgrid Cluster.” Electric Power System Research 73: 71–85.
- Zakariazadeh, A., O. Homaee, S. Jadid, and P. Siano. 2014. “A New Approach for Real Time Voltage Control Using Demand Response in an Automated Distribution System.” Applied Energy 117: 157–166.