ABSTRACT
Indonesia has abundant oil palm empty fruit bunches (OPEFBs). However, high lignin content in OPEFBs may hinder their valorisation to bioenergy resources, as its degradation by microorganisms is rate-limiting. This paper aims to review OPEFB as feedstock in anaerobic digestion (AD) systems and sustainable strategies to enhance methane yield. This paper provides a comprehensive review of the availability of OPEFB, the prospective of AD technology, and compares various biological pre-treatment and co-digestion strategies for superior AD process. Improving the value-added benefits from OPEFBs and simultaneously making pre-treatment processes more sustainable is critical to wider application. Several scenarios were proposed by combining multi-stage pre-treatment of physical and mushroom cultivation pre-treatment prior to AD. This paper presents an original approach to establishing a commercially viable and sustainable AD of OPEFBs in Indonesia by integrating multi-product biorefinery and a circular economy. Further investigation is required to ensure cost-effective and eco-friendly configurations.
Abbreviations: ABR: Anaerobic Baffled Reactor; AD: Anaerobic Digestion; AF: Anaerobic Filter; AFEX: Ammonia Fibre Expansion; AI: Artificial Intelligence; AnMBR: Anaerobic Membrane Bioreactor; ANN: Artificial Neural Network; ASP: Activated Sludge Process; CMM: Cattle Manure and Maize Silage; COD: Chemical Oxygen Demand; CPO: Crude Palm Oil; CPW: Cocoa Pods Waste; CSTR: Continuous Stirred-Tank Reactor; DH: Dry Husk; DM: Dry Matter; DMF: 2,5-dimethylfuran; EM: Effective Microorganisms; EGSB: Expanded Granular Sludge Bed; FBR: Fluidised Bed Reactors; FFB: Fresh Fruit Bunch; FTIR: Fourier-Transform Infrared Spectroscopy; GHG: Greenhouse Gasses; HRT: Hydraulic Retention Time; IC: Internal Circulation Reactor; IoT: Internet of Things; Lac: Laccase Enzyme; L-AD: Liquid-AD; LiP: Lignin Peroxidase; LRAD: Low-Rate AD; MARS: Multivariate Adaptive Regression Splines; MCC: Microcrystalline Cellulose; MDF: Medium-Density Fibreboard; MEMR: Ministry of Energy and Mineral Resources, Republic of Indonesia; ML: Machine Learning; MnP: Manganese Peroxidase; MPA: Marine Predators Algorithm; MSW: Municipal Solid Waste; OFMSW: Organic Fraction of Municipal Solid Waste; OLR: Organic Loading Rates; OPEFBs: Oil Palm Empty Fruit Bunches; OPF: Oil Palm Fibre; OPKS: Oil Palm Kernel Shell; OPMF: Oil Palm Mesocarp Fibre; OPT: Oil Palm Trunk; P(3HB): Poly(3-Hydroxybutyrate); PHA: Polyhydroxyalkanoate; PLTBg: Biogas Power Plants; POM: Palm Oil Mill; POME: Palm Oil Mill Effluent; SCG: Spent Coffee Grounds; SRF: Solid Refused Fuel; SRT: Solids Retention Time; SS-AD: Solid-State AD; TS: Total Solid; TSI: Torrefaction Severity Index; UASB: Up-flow Anaerobic Sludge Blanket; VFA: Volatile Fatty Acids; VP: Versatile Peroxide; VS: Volatile Solid; W2E: Waste-to-Energy.
1. Introduction
Globalisation of the oil palm industry for oil production has created significant forest losses and ecosystem degradation Thus, posing a new menace to biological diversity, particularly to the South East Asia countries such as Indonesia, Malaysia, and Papua New Guinea (Gatti et al. Citation2019). In Indonesia, every year, the oil palm industry produces a large quantity of residual biomass in the form of empty palm oil fruit bunches (OPEFBs). A large amount of OPEFBs has become a big challenge for oil palm industries in Indonesia (Nieves, Karimi, and Horváth Citation2011). Each OPEFB weighs around 3.5 kg with 130 mm thickness (Teh, Goh, and Kamarudin Citation2010); 17–-300 mm in length and 250–350 mm in width (Kerdsuwan and Laohalidanond Citation2011), thus making large dumping area a mandatory requirement within the industrial facilities. OPEFBs have now gained attention as a potential feedstock to produce bioenergy and bioproducts (Majesty and Herdiansyah Citation2019); as it is rich in organic materials with moisture content (60–70%) (Kim et al. Citation2013; Yacob et al. Citation2005). Different ranges of bioenergy (i.e. biogas, bioethanol, biohydrogen, biodiesel, solid refuse fuels, electricity, etc.) and high value-added products (i.e. xylitol, vanillin, activated carbon, etc.) can be produced from OPEFBs (Burhani and Triwahyuni Citation2019; Hambali and Rivai Citation2017; Harahap, Silveira, and Khatiwada Citation2019; Heryadi et al. Citation2019; Nuryadi, Pratomo, and Raksodewanto Citation2019; Parbowo, Ardy, and Susanto Citation2019; Rahmi et al. Citation2019; Yoo et al. Citation2019). In more detail, the potential valorisation of OPEFBs as bioenergy and high-value-added products, is shown in .
Figure 1. Potential valorisation of OPEFBs as bioenergy and high value products (The figure is adapted from Kasivisvanathan et al. (Citation2012); Vaskan, Pachón, and Gnansounou (Citation2018); Ahmad et al. (Citation2019); and Hafyan et al. (Citation2020)). MCC: microcrystalline cellulose, MDF: medium–density fibreboard, PHA: Polyhydroxyalkanoate, P(3HB): Poly(3–Hydroxybutyrate), SRF: solid refused fuel.
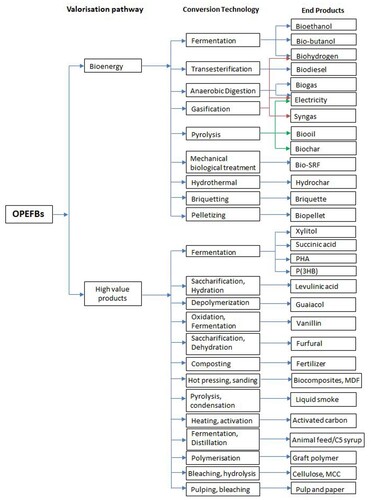
Anaerobic digestion (AD) is a commercial conversion technology resulting in renewable energy production in methane (Appels et al. Citation2011). AD provides a pathway for a closed balanced carbon cycle concerning atmospheric carbon dioxide and utilises wastes and biomass as a significant renewable energy resource (Chynoweth, Owens, and Legrand Citation2001). Therefore, AD is considered one of the best ways to achieve sustainable energy development goals in many developing countries, including Indonesia. AD technology was a well-established technology applicable for first- to fourth-generation biomass, as shown in . With most of the country's consumption coming from fossil-based fuels and its inability to local oil production since 2004, there is an urgent need to adopt sustainable and renewable energy production, such as producing biogas from OPEFBs (Khalil et al. Citation2019). Previous studies suggest that implementing AD technology for biogas production provides better economic and environmental benefits (Suhartini et al. Citation2022a; Citation2022b). Various studies have also emphasised the opportunities for transforming OPEFBs into biogas under various operational conditions, operated in mono- and co-digestion systems (Chaikitkaew, Kongjan, and O-Thong Citation2015; Hidayat et al. Citation2020; Nieves, Karimi, and Horváth Citation2011; O-Thong, Boe, and Angelidaki Citation2012; Suhartini, Hidayat, and Nurika Citation2020; Citation2021; Suksong et al. Citation2017a; Citation2016; Citation2017b; Citation2020a; Citation2020b). However, several problems were found to influence the biogas production from OPEFBs by AD. Several studies have emphasised that operating AD of OPEFBs in mono-digestion systems without pre-treatment has low efficacy (Suhartini, Hidayat, and Nurika Citation2020; Citation2021; Suksong et al. Citation2017b). In addition, several studies have highlighted that operating AD of untreated OPEFBs under thermophilic temperature has superior performance than mesophilic temperature (Suksong et al. Citation2017a; Walter et al. Citation2015). In practice, this could be associated with higher energy and operational costs if it cannot maximise the net energy production (Suryawanshi, Chaudhari, and Kothari Citation2010). Furthermore, the low methane production was mostly found in anaerobic mono-digestion of untreated OPEFBs due to its complex lignocellulosic structure (Rohma, Suhartini, and Nurika Citation2021). Many studies have highlighted that OPEFB has low biodegradation in the AD process mainly due to its high lignin content (20.4%–28.8%) (de Gonzalo et al. Citation2016; Hidayat et al. Citation2020). Nieves, Karimi, and Horváth (Citation2011) found that the higher the lignin content in biomass, the lower the biodegradability.
Table 1. Biomass resources and the conversion routes for biogas production.
Lignin functions as a protective component to maintaining a rigid structure in plants; thus, opening its compact structure increases the biodegradability (Nieves, Karimi, and Horváth Citation2011). Therefore, to increase the efficacy of AD of OPEFBs, the delignification is pivotal for releasing cellulose and hemicellulose. Furthermore, breaking the cellulose-hemicellulose-lignin bonds can also help to result in higher yields of reducing sugars (Prasad et al. Citation2019); and higher biogas or methane yields (Taherzadeh and Karimi Citation2008). Hence, pre-treatment of OPEFBs becomes essential to obtain maximum biogas/methane yield. Furthermore, the choice of pre-treatment is crucial for the overall success of the AD systems (Waluyo et al. Citation2018). Pre-treatment methods are generally divided into physical, physico-chemical, chemical, and biological pre-treatment, and their comparison is shown in .
Table 2. Summary and comparison of lignocellulosic biomass pre-treatment.
Physical pre-treatment tends to increase the accessible area and size of pores and decrease the cellulose crystallinity and polymerisation degrees. However, it can often be slow, energy-intensive, and prohibitively expensive (Kristiani et al. Citation2015). Chemical pre-treatment utilises chemical compounds to alter the lignocellulosic components in the OPEFBs, thus reducing their recalcitrance for further utilisation processes. A typical chemical pre-treatment performed on OPEFBs is acid and alkaline pre-treatment. In alkaline pre-treatment, a large amount of alkaline (e.g. NaOH) is used to alter the intermolecular bonds in the lignocellulosic materials (i.e. xylan, hemicelluloses). This method removes the structural and compositional obstacles for processes (i.e. hydrolysis), improves the digestibility rate, and increases the yield of fermentable sugars available for further conversion (Sudiyani et al. Citation2013; Triwahyuni et al. Citation2015). Recent studies on chemical pre-treatment of OPEFBs have also been investigated concerning bioenergy production. These methods include the use of aqueous ammonia, peracetic acid/alkaline peroxide, phosphoric acid, bisulphite, acid hydrolysis, and organosolv pre-treatments (Pangsang et al. Citation2019). Most of these methods use a range of chemicals or organic solvents at high temperatures such as 100–200°C for a short period, either in the presence or absence of catalysts (Chin et al. Citation2019). Chemical pre-treatments obtain high sugar concentration for most of the methods employed; however, the process's washing and neutralisation steps make it non-feasible for the overall process economics. Furthermore, consideration of the inhibitory compound formation, cost of chemicals, and potential chemical wastewater treatment, limited its application. Also, many studies have emphasised the potential inhibitory effect of chemical pre-treatment residues on AD microbial consortia which leads to reduce biogas and methane yield (Liew et al. Citation2021; Rohma, Suhartini, and Nurika Citation2021; Stanley et al. Citation2022; Zhang et al. Citation2018; Zheng et al. Citation2014). For example, our previous study by Rohma, Suhartini, and Nurika (Citation2021) found that the presence of excessive acid residues after acid pre-treatment may inhibit the AD process. Furthermore, Suhartini et al. (2022c) also reported that acid pre–treatment is not a feasible and sustainable option as it requires high operation costs for additional safety precautions, corrosion–resistant vessels, chemicals, safe disposal of waste chemical, and treatment of chemical wastewater.
Hence, the focus is more on chemical-free options such as hot-thermal water pre-treatment or biological pathways (Pangsang et al. Citation2019). Biological pre-treatment (alone or in combination with other pre-treatment) has been considered an economical pre-treatment method compared with other pre-treatment options (Saritpongteeraka et al. Citation2015). As shown in , despite some general weaknesses, biological pre-treatment may offer better economic and environmental benefits in terms of cost- and energy-saving, low use of chemicals, green operation, and no inhibitory compounds. Many studies have also highlighted that sustainable strategies for enhancing bioenergy production from lignocellulosic biomass should encompass appropriate green pre-treatment, intensification of conversion technology, and post-treatment operations (Ab Rasid et al. Citation2021; Bhutto et al. Citation2017; Kurian et al. Citation2013). The proper and cost-effective pre-treatment mays support the successful implementation of sustainable biorefinery of lignocellulosic biomass (Ab Rasid et al. Citation2021), including OPEFBs.
The utilisation of residues from the oil palm industry for biogas production using AD technology has been reported in Indonesia, Malaysia, and Thailand (as the top three oil palm producers). As already discussed, high concentrations of lignin and cellulose have negative synergy on biomass digestibility. These materials are inhibitory for biogas production as the biomass becomes less available for the microorganisms in the digester. Also, their further degradation leads to the accumulation of long-chain fatty acids, which are detrimental to AD (Temu et al. Citation2016). Therefore, green pre-treatments should be employed to achieve a cost-effective and sustainable biogas production via the AD process. Many studies have highlighted the various pre-treatments options for OPEFBs for bioenergy production. But, limited in-depth studies that focused on green pre-treatments (specifically biological methods) to support sustainable biorefinery of OPEFBs through biogas production in Indonesia. Therefore, this paper investigates the potential to implement biological methods for enhanced methane production from the AD of OPEFBs. This paper also investigates future perspectives on economically feasible and sustainable pre-treatment strategies for enhancing the efficacy of AD of OPEFBs. This paper presents a detailed analysis and comparison of key techniques in OPEFBs pre-treatments and AD, as well as the potential strategies proposed to support the established sustainable AD of OPEFBs in Indonesia.
2. OPEFB – availability and its potential for AD feedstock
2.1. Oil palm industry and the availability of OPEFBs
Oil palm grows mainly in Africa, Latin America, and Asia. Indonesia and Malaysia are the leading oil palm producers worldwide, followed by Thailand, Colombia, and Nigeria (Shahbandeh Citation2021; Citation2020). Indonesia has the largest certified palm planted area worldwide, accounting for 12.8 million hectares in 2018 (Hirschmann Citation2020). Oil palm plantation in Indonesia is divided into private plantations (49.81%), people's plantations (45.54%), and the country's estates plantations (4.65%). In 2018, the total production of oil palm in Indonesia was approximately 26.90 million tonnes, which was dominated by 5 (five) provinces, including Riau at 7.14 million tonnes (19.50%), Kalimantan Tengah at 5.76 million tonnes (15.74%), Sumatera Utara at 5.45 million tonnes (14.88%), Kalimantan Barat at 3.07 million tonnes (8.40%), and Sumatera Selatan at 3.04 million tonnes (8.31%), respectively (BPS-Statistics Indonesia Citation2018). These oil palms are mostly used for Crude Palm Oil (CPO) production, with a total value of 36.6 million tons in 2018 in Indonesia and predicted to increase annually (Hirschmann Citation2020). The global oil palm production was 56.38 million metric tons in 2012 and increased to 75.45 million metric tons in 2020 (Shahbandeh Citation2021; Statistica Research Department Citation2021). Similar trends were also observed in Indonesian oil palm production. The production increased from 26.02 million metric tons (in 2012) to 48.30 million metric tons (in 2020), giving an average increase of 53.08% annually. The global and Indonesian production of oil palm is shown in . The figure indicates that Indonesia has abundant oil palm production, hence a high potential for residual waste, including OPEFBs.
Figure 2. Production of palm oil worldwide and in Indonesia from 2012 to 2019 (in million tons) (The figure is adapted from Sharbandeh (Citation2021); (Citation2020) and Statistica Research Department (Citation2021)).
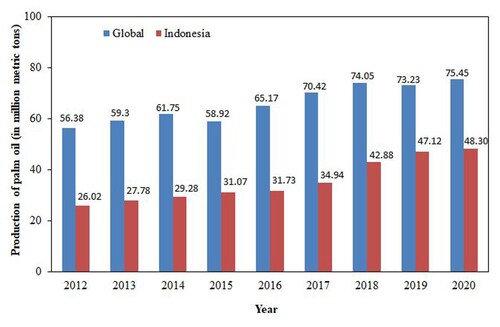
Furthermore, Suhartini et al. (Citation2022c) reported more than 700 palm oil mills (POMs) in Indonesia, which is predicted to grow each year. Three big companies are producing CPO in Indonesia, including Golden Agri-Resources Ltd. (GAR) (produces 2.44 million tonnes CPO), Wilmar International (produces 1.97 million metric tonnes CPO), and IOI Group (produces 756.6 thousand metric tonnes) (Hirschmann Citation2020). illustrates the oil palm value chain and material flow (i.e. material balance) in a POM. It can be seen that various stakeholders are playing a pivotal role in the oil palm industry, from material acquisition to CPO manufacturing ((a)). In Indonesia, tied/plasma, independent, and associated smallholders are critically important to ensure the oil palm's availability and sustainability supply to the industry, income generation for local farmers, and employment opportunities (Pacheco et al. Citation2017). Evidence also shows that the oil palm industry has contributed significantly to Indonesia's economy over the decades (Pramudya, Hospes, and Termeer Citation2017; Purnomo et al. Citation2020). Despite this, many have indicated a great environmental impact (Ayompe, Schaafsma, and Egoh Citation2021; Gatti et al. Citation2019; Meijaard et al. Citation2020; Teng, Khong, and Che Ha Citation2020). Considering the global commitment to bioeconomy and sustainability, the oil palm industries in Indonesia have made an effort to green and sustainable plantation and manufacturing processes (Ayompe, Schaafsma, and Egoh Citation2021; Boons and Mendoza Citation2010; Purnomo et al. Citation2020).
Figure 3. Palm oil value chain (a) (Adapted from Pacheco et al. (Citation2017)), and the material flow in POM (b) (Adapted from Rizal et al. (Citation2018)). CPO: crude palm oil, FFB: fresh fruit bunches, OPEFB: oil palm empty fruit bunch, OPF: oil palm fibre, OPKS: oil palm kernel shell, OPMF: oil palm mesocarp fibre, OPT: oil palm trunk, POM: palm oil mill, POME: palm oil mill effluent.
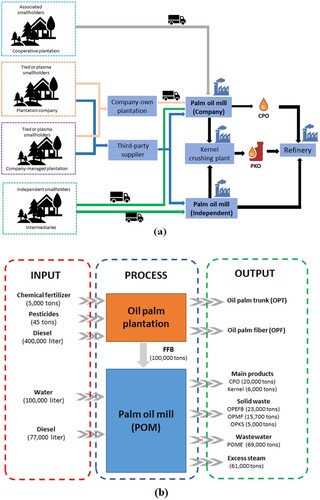
Indeed, an increase in CPO demand or production is followed by increasing the number of oil palm plantations and the waste resulting from POMs (Risdianto, Kardiansyah, and Sugiharto Citation2016). (b) shows the material balance at a POM, indicating potential waste residual generation. A study reported that 1 ton of fresh fruit bunch (FFB) can produce approximately 0.2–0.3 tons of OPEFBs (Suksong et al. Citation2020a). Other studies reported that POMs produce OPEFBs with the value of 25% (w/w) of FFB (Santi, Kalbuadi, and Goenadi Citation2019); or about 22% (w/w) of FFB (Abdullah and Sulaiman Citation2013). While according to Rizal et al. (Citation2018), as shown in (b), about 23% of OPEFBs have resulted from each ton of FFB used in CPO production, with a 20% yield of FFB. Similarly, our previous study also suggested that, on average, a POM produces 21% CPO and 22.5% OPEFBs from processing 1 ton FFB (Suhartini et al. Citation2022c). Therefore, based on the CPO production of 48.30 million metric tons in 2020 in Indonesia (as shown in ), this equates to ∼241.5 million tons of FFB (input) and potential OPEFBs of 55.54 million tons. This data shows a huge availability of OPEFBs in Indonesia. This biomass can be considered a highly potential biomass resource to be further valorised as bioenergy or high value-added products.
Considering the current potential data of OPEFBs in Indonesia, further measures are essential to prevent any detrimental environmental impact from the direct disposal of OPEFBs. Furthermore, higher cellulose, hemicellulose, and lignin content in OPEFBs make them attractive to bioenergy production, via thermal or biological routes. For instance, to date, about 700 POMs (with an average production capacity of 30–45 FFB/h) across the country have utilised OPEFBs for local bioenergy generation. This is done via on-site biomass power plants (up to 3500 MW electricity) and biogas power plants (PLTBg) operated in co-digestion with palm oil mill effluent (POME) (∼700 MW electricity) (Suhartini et al. Citation2022c). Another potential valorisation of OPEFBs is bioethanol production due to their higher cellulose content in OPEFBs. With the combination of various pre-treatments (for delignification) and fermentation, OPEFBs were found to have higher bioethanol yields (Harahap, Mardawati, and Nurliasari Citation2020a; Mardawati et al. Citation2019; Suhartini et al. Citation2022c). Yet, limited studies have reported the commercial bioethanol plant using OPEFBs as feedstock in Indonesia. In 2019, about 0.40 million L/year of bioethanol is produced in the country, but mostly using molasses as feedstock (Ministry of Energy and Mineral Resources (MEMR), Citation2019). In addition, a high hemicellulose content in OPEFBs also makes it viable for the production of xylitol, an artificial sweeter used in food, pharmaceuticals, beverages, health and personal care, and animal feed industries (Harahap, Mardawati, and Nurliasari Citation2020a; Mardawati et al. Citation2022; Citation2018; Suhartini et al. Citation2022c). By combining pre-treatment and biological routes (i.e. enzymatic and yeast fermentation), hemicellulose is converted into xylose and later into xylitol (Mardawati et al. Citation2022; Citation2018; Citation2017; Citation2014; Suhartini et al. Citation2022c). Currently, the top three leading countries as xylitol producers are China, the USA, and India (Ahuja et al. Citation2020); still no commercial xylitol manufacturing is available in Indonesia (Suhartini et al. Citation2022c). High lignin content in OPEFBs also makes it feasible for direct valorisation as briquettes (Cabrales, Arzola, and Araque Citation2020; Nyakuma, Johari, and Ahmad Citation2012); solid fuel pellets (Munawar and Subiyanto Citation2014; Nyakuma, Wong, and Oladokun Citation2021); fibre- and particle-board or reinforced polymer composites (Ramlee et al. Citation2021a; Citation2021b; Zuhri, Sapuan, and Ismail Citation2009). These studies demonstrated that the chemical composition of OPEFBs may influence the best conversion routes for bioenergy or bioproducts.
2.2. Potential use of OPEFBs as AD feedstock
OPEFBs are non-wood lignocellulosic biomass, known as a high organic material resource (O-Thong, Boe, and Angelidaki Citation2012). OPEFBs have moisture content in the range of 66–69%, volatile matter of 86.5–87.7%, fixed carbon of 10.8–14.5%, ash of 3.7–5.3%, and a calorific value of 18.88 MJ/kg (Sukiran et al. Citation2017). Generally, OPEFBs contain carbon (48.72%), hydrogen (7.86%), nitrogen (0.25%), and oxygen (48.18%) (on a dry basis), respectively (Loh Citation2017). As lignocellulosic biomass, OPEFBs primarily consist of cellulose, hemicellulose, and lignin, which create a highly resistant and recalcitrant biomass structure (Rizal et al. Citation2018). Cellulose is a linear homopolysaccharide that contains crystalline and amorphous structures (Sukiran et al. Citation2017). Hemicellulose is a highly branched heteropolysaccharide, consisting of a wide variety of sugars (such as glucose, mannose, galactose, xylose, arabinose, 4-O-methyl glucuronic acid, and galacturonic acid residues) (Sawatdeenarunat et al. Citation2015). Anaerobic microorganisms can utilise cellulose and hemicellulose for biogas production (Suksong et al. Citation2016). Meanwhile, lignin, a complex polymer of phenylpropane units, is the most factor limiting the biodegradability of lignocellulosic biomass (Xu et al. Citation2019). Lignin encapsulates cellulose and hemicellulose by crosslinking through hydrogen and covalent bonds, forming a hydrophobic three-dimensional structure. This complex structure prevents plant cell walls from rapid degradation by microorganisms, thereby rendering biomass hard to be efficiently converted during AD (Ma et al. Citation2019). Li et al. (Citation2019a) reported that the methane yield of cellulose was higher than hemicellulose, whereas lignin cannot be digested. However, the co-digestion of cellulose and hemicellulose increased methane yield and biodegradability. It suggested that biomass with high cellulose and low lignin content has higher biogas and methane production.
Thus, OPEFBs are potential renewable energy feedstock for biogas production via AD technology (Hidayat et al. Citation2020; O-Thong, Boe, and Angelidaki Citation2012; Suhartini, Hidayat, and Nurika Citation2020; Citation2021; Suksong et al. Citation2016; Citation2019b; Citation2020b). A study reported that AD of OPEFBs at a C/N ratio of 25–30 could have methane yields of 50–54 m3/ ton OPEFBs (Suksong et al. Citation2016). Another study suggests that methane yield from the AD of OPEFBs at operating conditions of TS (16% (w/w)), C/N ratio (30), and food to inoculum (F:I) ratio (2:1) was 0.358 m3 CH4 /kg VS (Chaikitkaew, Kongjan, and O-Thong Citation2015). OPEFB is rich in carbon but low in nitrogen. In the AD system, the C/N ratio indicates the nutrient balance that anaerobic microorganisms require for growth (Korres et al. Citation2013). Furthermore, a study reported that without pre-treatment, the AD process shows a low conversion efficiency (<60%) (Suksong et al. Citation2017a). Our previous study, reported in Suhartini et al. (Citation2021), that mono-digestion of untreated OPEFBs gave the lowest methane yield (0.185 m m3 CH4 /kg VS) compared to other organic wastes (i.e. rice straw, maize straw, vegetable waste, jackfruit straw, banana peel, orange peel, apple peel, and pineapple peel). This was due to the high lignin content in the OPEFBs. Therefore, specifically for OPEFBs, pre-treatment to remove lignin or to extract cellulose and hemicelluloses is necessary to enhance biogas and methane yields.
3. Anaerobic digestion of OPEFBs
3.1. AD process and influencing parameters
Biomass conversion into energy sources can use biological processes by converting complex organic components into value-added metabolites. This transformation process is carried out in an anaerobic digester that uses microorganisms to degrade organic components into methane and other products (Ohimain and Izah Citation2017). This process is also defined as anaerobic digestion (AD), a biochemical process where the breakdown of complex feedstock releases energy into simpler units in the absence of oxygen (Zhang, Hu, and Lee Citation2016).
AD is a complex process consisting of four phases of degradation named hydrolysis, acidogenesis, acetogenesis, and methanogenesis (Deublein and Stenhauser Citation2011). The dominant biogas containing methane gas is produced in the methanogenesis process (Nieves, Karimi, and Horváth Citation2011; Suksong et al. Citation2020b). The operations inside an anaerobic digester are complex and very sensitive to small changes in conditions, where each intermediate step is governed by strict requirements (Horan, Yaser, and Wid Citation2018). Therefore, the condition of each process significantly affects biogas production. There is a reshuffling process of complex organic matter in hydrolysis, where carbohydrates become glucose, proteins become amino acids, and fats become fatty acids. In the acidogenesis phase, the monomer sugars are broken down into volatile fatty acids (VFA). In the acetogenesis phase, the VFA is used as a substrate for methanogenic bacteria in the methanogenesis process. The methanogenesis phase is the most crucial in AD because this process is susceptible and influenced by certain factors (such as raw material composition, feedstock rate, temperature, and pH) (Li et al. Citation2020; Van et al. Citation2020). Methanogenic bacteria are susceptible to oxygen, and low oxygen concentrations can kill all methanogenic bacteria (Neves, Oliveira, and Alves Citation2004). In addition to the methanogenesis phase, a dark fermentation process can also be carried out to produce H2 gas. The stages in the AD process can be seen in . Furthermore, methane gas produced from AD ranges from 50 to 70%, where the higher the methane produced, the greater the potential energy that can be used (Ohimain and Izah Citation2017). In the study of Zhang et al. (Citation2007), biogas contains methane of 50–80% CH4 and CO2 of 20–50%, while in the study of Neves, Oliveira, and Alves (Citation2004), the biogas contains 60–70% CH4 and 30–40% CO2.
Figure 4. Pathways of anaerobic digestion for biogas production (Adapted from Rasapoor et al. (Citation2020); Li, Chen, and Wu (Citation2019b); and Rabii et al. (Citation2019)).
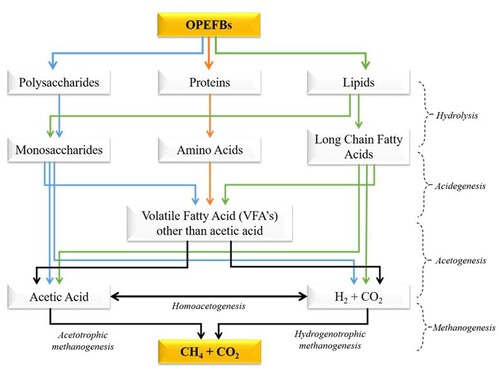
Biomass resources that contain lower (<40%) or higher water content are potential as feedstocks in the AD systems (Appels et al. Citation2011). Ideally, for higher efficacy performance of a dry (or high-solids) AD system, biomass should have a moisture content ranging from 20 to 40% (Rocamora et al. Citation2020); or any biomass with moisture content below 80% (or total solids/TS >20%) (Chiumenti, da Borso, and Limina Citation2018; Jansson et al. Citation2019). While biomass with moisture content above 85% (or TS < 15%) is better treated using a wet (or low-solids) AD system (Jansson et al. Citation2019). Compared with a wet-AD, operating a dry-AD system offers a lower water consumption, a lower water content in digestate (reducing digestate volume for storage and treatment), and a smaller reactor size (Chiumenti, da Borso, and Limina Citation2018; Jansson et al. Citation2019; Rocamora et al. Citation2020). However, a dry-AD needs a longer retention time, mixing for homogenisation, and often has a problem of the feedstock’ pumping than a wet-AD system (Jansson et al. Citation2019). Furthermore, Mata-Alvarez et al. (Citation2014) added that AD could be operated either using single substrates (mono-digestion) or with the addition of co-substrates (anaerobic co-digestion). However, there were various disadvantages to anaerobic mono-digestion, such as the variability of substrate properties, seasonality, or the presence of inhibiting/toxic materials. Therefore, the study further added that co-digestion of two or more substrates is more economically feasible and can produce higher methane production than mono-digestion. Khalid et al. (Citation2011) claimed that AD of organic solid waste might potentially be a better option to fulfil the future energy demand.
Various factors strongly influence biogas production, including nutrients, organic loading rates (OLR), hydraulic retention time (HRT), activity and population of microorganisms, presence of inhibitors/toxic substances, pressure, mixing conditions, substrates composition, alkalinity, pH, and temperature (Ohimain and Izah Citation2017). These operational parameters require constant monitoring and adjustment according to the biomass composition and the reactor conditions (Mao et al. Citation2015). Nutrients used in AD can be in the form of macro- and micro-molecules such as carbohydrates, proteins, fats, cellulose, nitrogen, sodium, magnesium, and calcium (Appels et al. Citation2011; Taricska et al. Citation2009). The growth and activity of microorganisms in AD reactors varies depending on each transformation process but the most crucial role in the formation of biogas/methane is methanogenic bacteria (Appels et al. Citation2011; Chen et al. Citation2014a; Citation2008). The development of these microorganisms must be avoided from any inhibitory substances such as light metal ions, heavy metals, and organic compounds (Chen et al. Citation2014a; Citation2008). The overall pressure inside the AD reactor is essential during methane production (Díaz et al. Citation2020; Lemmer et al. Citation2017). If the weight of the gas outside the reactor is greater than that inside the bioreactor, the pressure becomes negative, and the reactor explodes (Chen et al. Citation2014b; Suhartini, Heaven, and Banks Citation2014). The mixing and agitation processes may affect the even distribution of substrates and microorganisms, thus expanding the contact area of the substrate and microorganisms (Appels et al. Citation2011; Ohimain and Izah Citation2017). Chen et al. (Citation2014a) reviewed various toxic materials (i.e. ammonia, chlorophenols, sulphide, halogenated aliphatics, long-chain fatty acids, heavy metals, and the emerging nanomaterials) that present in the feedstock can inhibit the AD process to various degrees. Those toxic materials have several effects on disrupting the activities of acetogens and methanogenic, delaying the formation of methane, reducing the methane concentration of biogas, and deteriorating the methanogenesis process. The most influential factors on biogas yield are pH and temperature because these factors significantly affect the growth and metabolism of certain microorganisms in the AD reactor (Appels et al. Citation2011; Taricska et al. Citation2009). For example, in acidogenesis and acetogenesis phases, microorganisms can grow optimally at pH < 6, while in the methanogenesis phase, the pH must be > 6. While the temperature may affect the microbial density, gas transfer rate, depositional properties, and solid residue from the substrate (Ohimain and Izah Citation2017). For instance, in the AD of OPEFBs, a study found that operating at thermophilic has superior performance than in mesophilic conditions. This was due to the improved efficacy of methanogens (i.e. Methanoculleus and Methanosarcina) (Walter et al. Citation2015).
Therefore, it can be seen from the above discussion that AD is a complex process and the performance of a feedstock is governed by several factors. Continuous monitoring is essential to facilitate effective biogas production.
3.2. Current practice of AD in Indonesia
Indonesia is one of the most populated countries, with increasing population and energy demand problems. With the current dependence on fossil fuel-based energy, Indonesia faces a huge challenge in innovating and developing new and renewable energy technology. Khalil et al. (Citation2019) recommended transforming animal waste into biogas as a better option supported by an increase in the local livestock population, causing a large amount of animal organic waste available. Previously, Purwono et al. (Citation2013) also stated that implementing a biogas digester is suitable for providing alternative energy in Indonesia's remote and marginal villages.
Several studies and practices of AD have been carried out in Indonesia, using various biomass feedstocks, including dairy manure (Usack, Wiratni, and Angenent Citation2014); animal waste (Khalil et al. Citation2019); sawdust (Ardhiansyah and Budiyono Citation2018); paper sludge (Priadi et al. Citation2014); landfill leachate (Kawai et al. Citation2012); POMEs (Halim et al. Citation2017; Harsono, Grundmann, and Soebronto Citation2014); fruit waste (Marendra et al. Citation2019; Sitorus and Panjaitan Citation2013); food waste (Suhartini, Lestari, and Nurika Citation2019; Citation2022b); microalgae (Salafudin et al. Citation2015); and macroalgae (Suhartini et al. Citation2022a). A previous study by Adinurani et al. (Citation2013) found that Jatropha curcas Linn. capsule husk (JcL-CH) is a potential feedstock for producing biogas, either in a single- or two-stage AD system. Specifically, in the two-stage AD system, the methanogenesis reactor was equipped with a biofilm/bio-carrier made of palm fibre and glass wool. The study demonstrated that the two-stage AD system had superior performance to the single-stage AD, with 63.83% higher biogas production. It was also found that the methanogenesis reactor with glass wool biofilm was more effective than palm fibre. Their study also compared the quality and quantity of dry husk Jatropha curcas Linn (DH-JcL) in a single-stage AD system under batch and continuous conditions. The results showed that dry husk Jatropha curcas Linn in semi-continuous digester produced biogas with an average of 0.016 m3/kg DH-JcL and 0.01 m3/kg DH-JcL in the batch digester. The methane concentration in the semi-continuous digester was 83.15%, much higher than the batch digester of 68.61%. Another study by Priadi et al. (Citation2014) reported that anaerobic co-digestion of paper sludge with cow manure has higher methane production (0.269 m3/kg VS) than mono-digestion of paper sludge (0.015 m3/kg VS).
Besides biomass, various studies have also reported the AD of industrial wastewater in Indonesia. For example, Kawai et al. (Citation2012) demonstrated that AD of landfill leachate was an practical approach in transforming chemical oxygen demand (COD) into biogas, reducing COD concentration by up to 40%. Oktavitri et al. (Citation2019) found that slaughterhouse wastewater can also be used as feedstock in the AD system with Chlorella vulgaris addition for biogas purification. By using this integrated AD system, COD concentration in the slaughterhouse wastewater was reduced by up to 63% and enhanced CO2 removal in biogas by up to 7%. In addition, tofu-processing wastewater was also reported to have potential as an AD feedstock in Indonesia, either in continuous or batch AD systems (Darwin Citation2019). This study revealed that biogas produced from a continuous AD system was 0.123 m3/kg VS, which was much higher than the batch AD system (0.043 m3/kg VS). Such significant differences were possibly due to higher VS destruction found in continuous AD systems (76%) than in batch AD systems (57%). Additional benefits of implementing AD technology for treating tofu-processing wastewater were an increase in pH from 5 to neutral conditions (pH 6.6), following the industrial wastewater effluent discharge standard. A previous study by Syaichurrozi et al. (Citation2016) reported that adding yeast (Saccharomyces cerevisiae) to the AD of tofu wastewater can increase biogas and methane production. Therefore, it can be concluded that currently, there is no established AD route for OPEFBs in Indonesia. However, there is a potential for it to be used in an industrial-scale process having similar biogas potential to the feedstock compared above.
3.3. Development in AD technology prospective for treating OPEFBs
Biogas and methane production has been developed and implemented globally via AD technology. AD reactors are commonly divided based on the production rates: low-rate and high-rate systems (De La Rubia, Villamil, and Mohedano Citation2019). A low-rate AD (LRAD) system is characterised by the presence of suspended biomass, requiring a long HRT and solids retention time (SRT). This system is recommended for media with high solids concentrations (i.e. mixed sewage sludge and organic fraction of municipal solid waste (OFMSW)). In such a system, the anaerobic conversion capacity is completely related to the growth rate of the microorganism. Because the growth rate is very low, a high reactor volume and continuous stirring are required to ensure homogeneity. The most suitable reactor in this type is a continuous stirred-tank reactor (CSTR) (De La Rubia, Villamil, and Mohedano Citation2019; Gerardi Citation2003). A high-rate AD (HRAD) system involves the retention of biomass, characterised by a relatively short HRT but a long SRT allowing a reduction in reactor volume. This reactor is usually used for municipal and industrial wastewater treatment. Biomass retention can be achieved by immobilising anaerobic sludge through granule reactors (i.e. up-flow anaerobic sludge blanket/UASB, expanded granular sludge bed/EGSB, and internal circulation reactor/IC), and biofilm (anaerobic filter/AF and fluidised bed reactors/FBR). This way, the biomass concentration increases, and the reactor can be operated at a high OLR without cleaning the biomass. Another way to increase the biomass concentration is to return/feedback concentrated sludge in a secondary purifier, similar to the activated sludge process (ASP), or by using physical or membrane filtration (i.e. anaerobic baffled reactor/ABR, anaerobic membrane bioreactor/AnMBR) (De La Rubia, Villamil, and Mohedano Citation2019; Fagbohungbe et al. Citation2015; Gerardi Citation2003; Van et al. Citation2020). Furthermore, according to Stamatelatou, Antonopoulou, and Michailides (Citation2014), the selection of the AD reactor is mainly based on the total solid content (i.e. TS) and the organic content (i.e. volatile solid/VS) of the substrate feedstocks. A summary of the development in AD technology for biogas production is shown in . In the case of AD of OPEFBs, using a CSTR is recommended. In CSTR, agitation (or mixing) can homogenise nutrients, metabolites, and enzymes; thus, the contact surface area is faster and more expansive (Stamatelatou, Antonopoulou, and Michailides Citation2014). The CSTR also has a continuous and same rate of input feeding and effluent withdrawal to balance the microbial ability and population (Show, Yan, and Lee Citation2019). Other advantages of CSTR are uniformity in composition and temperature, the similar composition of inoculum and digestate (effluent), and stable operation (Foutch and Johannes Citation2003). The CSTR is also suitable for biomass with high solids concentration, such as OPEFBs. Therefore, this configuration is suitable for anaerobic mono- and co-digestion of OPEFBs with other organic waste (having TS > 20%). The system can also be integrated with pre-treatment facilities to enhance AD performance.
Table 3. Summary of prospective developed AD technology applied for biogas/methane production from OPEFBs.
Furthermore, based on the configuration system, AD is divided into single-, two- and three-stage systems (Speece Citation2008; Taricska et al. Citation2009; Van et al. Citation2020). In a single-stage AD, all stages of the AD process occur in one reactor. In a two-stage, hydrolysis to acidogenesis stages is occurred in the first reactor, while acetogenesis and methanogenesis stages are in the second reactor. The advantages of a single-stage AD system include simple operation, easier maintenance, and low installation and maintenance costs. While the disadvantages include a low input rate, a longer retention time, a higher chance of explosion, difficulty in controlling pH, low stability, and a higher possibility of inhibitors accumulation (Aziz et al. Citation2020; Speece Citation2008; Taricska et al. Citation2009; Van et al. Citation2020). A two-stage AD system is suitable for feedstock material with high solids or lipid concentrations, has a more stable digestion process, a faster retention time, a high OLR, and a high efficacy for VS/COD reduction. The drawbacks of a two-stage AD system include the accumulation of hydrogen (may inhibit acidogenesis bacteria), separation of the reactor (may reduce the nutrients needed by methanogenic bacteria), high installation and construction cost for manufacturing of two interconnected reactors, and requirement of skilled personnel for its complex operation requires (Aslanzadeh, Rajendran, and Taherzadeh Citation2014; Aziz et al. Citation2020; Speece Citation2008; Taricska et al. Citation2009; Van et al. Citation2020). Three-stage AD system is aimed to separate acetogenesis and methanogenesis stages, thus increasing the hydrolysis rate of biomass feedstock and improving biogas/methane yield. However, this system has a more complex configuration and high cost; therefore, selecting reactor type, mode of feeding, and temperature for each stage is critically important (Van et al. Citation2020).
4. Sustainable strategies for improving the AD of OPEFBs
Integrating a green and sustainable approach in AD is essential for enhancing the biogas and methane production from OPEFBs. Due to the high lignin content in OPEFBs, strategies are required to increase biodegradability and speed up the degradation process. With the current movement toward biorefining and bioeconomy concepts, sustainable strategies for improving the efficacy of the AD process are essential. Hence, this paper highlighted various sustainable strategies potential to be applied prior to or within the AD of OPEFBs, including biological pre-treatment and co-digestion, as shown in .
Figure 5. Sustainable strategies for improving the AD of OPEFBs, including the mechanism of each biological pre-treatment (Adapted from Abraham et al. (Citation2020); Mishra et al. (Citation2018); Mohd-Setapar, Abd-Talib, and Aziz (Citation2012); Villa et al. (Citation2020); Grimm and Wösten (Citation2018); Wan Mahari et al. (Citation2020); Pérez-Chávez, Mayer, and Albertó (Citation2019); and Zou et al. (Citation2020))
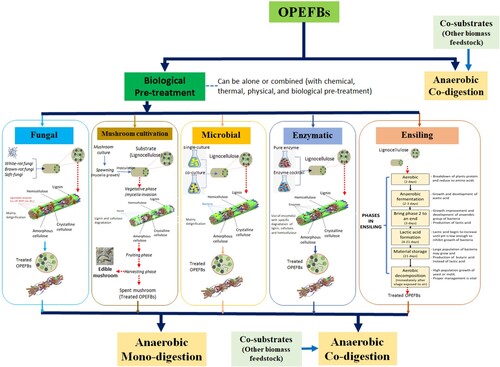
4.1. Biological pre-treatment of OPEFB for enhanced biogas/methane production
Various studies have emphasised the use of biological pre-treatment on OPEFBs as an alternative to chemical and thermal pre-treatment to increase further the efficacy of the AD process and the biogas and methane yields. These include fungi, microbes, enzymes, ensiling, and mushroom cultivation pre-treatment. shows a comparison of these biological pre-treatments, while details on the examples of each pre-treatment are shown in .
Table 4. The comparison of each biological pre-treatment.
Table 5. Other studies on biological pre-treatment on OPEFB prior AD process.
4.1.1 Fungal pre-treatment
In fungal pre-treatment, white-rot, brown-rot, and soft-fungi are mainly used to attack plant cell walls (i.e. lignin), composed of selective and non-selective lignin destruction, as explained by Kainthola et al. (Citation2021). They added that lignin and hemicellulose are specifically degraded in the selective lignin destruction process, and only a small fraction of cellulose is degraded. In the non-selective lignin destruction process, all lignocellulosic materials are degraded simultaneously. For this purpose, the fungi produce ligninolytic enzymes such as lignin peroxidase (LiP), polyphenol oxidase, manganese peroxidase (MnP), and laccase (Lac) to degrade lignin. Brown-rot fungi mainly attack cellulose, while white and soft-fungi attack lignin and cellulose (Agbor et al. Citation2011). Thus, the fungal pre-treatment can degrade lignocellulosic material to fermentable sugars using their produced lignocellulolytic enzymes (Intasit, Yeesang, and Cheirsilp Citation2019; Zou et al. Citation2020). Factors influencing the fungal pre-treatment include substrate composition, fungal strain, incubation period, moisture content, temperature, pH, nutrient, oxygen concentration, and cultivation condition (Kainthola et al. Citation2021). Longer incubation time needed in fungal pre-treatment becomes the major constraint for full-scale application (Montoya-Rosales et al. Citation2020). Furthermore, fungal pre-treatment can be carried out as a single- or co-culture fungal strain, as shown in .
Many studies have highlighted the successful application of single-culture fungal pre-treatment to alter the chemical composition and improve biodegradability. For example, Ishola, Isroi, and Taherzadeh (Citation2014) reported that treating OPEFBs with white-rot fungus Pleurotus floridanus LIPIMC996 for four-week improved the biodegradation rate of OPEFBs by 4.5-fold. They further stated that after fungal pre-treatment, an increase of hemicellulose (27.48%) and a decrease in cellulose (34.34%) were observed. This indicated that Pleurotus floridanus LIPIMC996 could degrade the lignin content in OPEFBs. The results of Fourier-transform infrared spectroscopy (FTIR) analysis also confirmed their findings, indicating a reduction in the cellulose hydrogen bond of the fungal-treated OPEFBs. After fungal pre-treatment, the degradation of lignin in OPEFBs showed a positive impact on increasing bioenergy production (i.e. bioethanol). Kamcharoen et al. (Citation2014) studied various white-rot fungi (i.e. Trametes versicolor TISTR 3224, Trametes sp. BCC 8729, Phanerochaete chrysosporium ATCC 24725, Marasmius sp. BCC 9542, and Xylaria sp. BCC 7749) for pre-treatment of OPEFBs. This study also indicated that fungal–treated OPEFBs have higher biodegradability than untreated OPEFBs, mainly due to lignin degradation. Suksong et al. (Citation2020b) also found that pre-treatment of OPEFBs using single-culture Trichoderma reesei TISTR 3080 and Pleurotus ostreatus DSM 11191 increased methane production by 52% and 44%, respectively. This was parallel to higher hydrolysis efficiency in cellulose, hemicellulose, and lignin by 10.9%, 22.2%, and 10.6% (for T. reesei) or by 9.35%, 8.08%, and 12.5% (for P. ostreatus). The fungal metabolism, i.e. enzyme activity, was beneficial for breakdown and reducing the sugar content ranging from 33.8–43.5 mg/g. Their study also confirmed the potential benefit of fungal pre-treatment: the energy recovery of pre-treated OPEFBs accounted for 200% higher than untreated OPEFBs.
A case study in Indonesia by Isroi (Citation2017) researched OPEFBs pre-treatment using white-rot fungi of Pleurotus floridanus and demonstrated that fungal pre-treatment altered the physical structure of OPEFBs to be more ‘breakable’. After pre-treatment, more lignin was degraded compared to cellulose or hemicellulose. This condition was mainly due to the ligninolytic enzyme production by Pleurotus floridanus, which contributes to the degradation of hemicellulose and lignin. The research also suggested that fungal pre-treatment of OPEFBs could enhance biodegradability, with the value of 95% over 28-day incubation, indicated by higher fermentable sugar production. Omar et al. (Citation2017) also reported that using white-rot fungi (i.e. Pycnoporus sanguineus) to pre-treat OPEFBs resulted in greater damage to the lignin structure and a decrease in lignin content. The ratio of OPEFBs:fungi (60:40) generated the best degradation performance within a 4-week incubation period, indicated by 90% lignin removal. In another research by Intasit, Yeesang, and Cheirsilp (Citation2019), oleaginous fungi Aspergillus tubingensis TSIP9 with cellulolytic enzymes were effective as a potent microorganism for solid-state fermentation with increased cellulose content after the treatment. Another recent study reported that pre-treatment of OPEFBs using an indigenous fungus (i.e. Schizophyllum commune ENN1) increased sugar yield (230 mg/g) and lignin removal (54%) (Arbaain et al. Citation2019). Our previous study also reported that fungal pre-treatment on OPEFBs using Phanerocheate chrysosporium improved organic matter and methane yield (Hidayat et al. Citation2020).
Besides single-culture fungi, co-culture fungi can also be used in biological pre-treatment. For instance, a previous study by Asiegbu, Paterson, and Smith (Citation1996) reported that using co-culture fungal strains (i.e. Pleurotus sajor-caju, Phanerochaete chrysosporium, and Trametes versicolor) reduce higher lignin content (8–16%) within shorter time (3-weeks incubation) than single-culture (4–14% lignin removal for 6-weeks incubation). van Heerden et al. (Citation2011) also found that P. sanguineus/A. flavipes co-culture could alter the chemical composition (i.e. cellulose, lignin, and hemicellulose) of woody biomass. Mohanan et al. (Citation2014) compared the use of single- and co-culture fungal strains (from genus Trichoderma, Aspergillus, Pycnoporus, and an unidentified strain of F113) for pre-treatment of kitchen waste. This study found that the co-culture of Trichoderma sp. with other fungal strains has higher degradation of lignocellulosic material than the respective single-culture. Furthermore, Ming et al. (Citation2019) studied the effect of co-culture fungal pre-treatment (using Aspergillus niger CBS 110.42 and CBS 554.65 and Phanerochaete chrysosporium CBS 246.84) on agricultural waste. The study reported that these co-culture fungal strains enhanced delignification by increasing the cellulose and xylan degrading activity (i.e. two-fold improvement). Saini and Sharma (Citation2021) also agreed that using fungal co-culture significantly reduced the pre-treatment time. Therefore, it can be highlighted that co-culture fungal pre-treatment offers higher efficiency of delignification and reduces the incubation time required. Hence, co-culture fungal pre-treatment has strong potential to be further implemented to support a more effective and efficient bioenergy production. Sperandio and Ferreira Filho (Citation2019) emphasised that implementing fungal co-culture may expand the biorefineries of lignocellulosic biomass for bioenergy and bio-based product generation.
Another potential route to enhance fungal pre-treatment is to combine it with other available pre-treatment. For example, Hidayatullah et al. (Citation2021) studied a combination pre-treatment on OPEFBs using a single-culture fungal strain (Marasmius sp and Phanerochaeta chrysosporium) with hydrothermal. The study suggests that using Marasmius sp. (30-day incubation time) followed by hydrothermal pre-treatment (160°C, 15 min) has better delignification results. This combination produces higher lignin degradation (26.67%) with higher xylose (166 mg/g OPEFB) and glucose (283 g/g OPEFB) production than using Phanerochaeta chrysosporium. Kannaiyan et al. (Citation2017) also found that a combination of hydrothermal and fungi pre-treatment increased deliginification rate, lignin degradation (i.e. by twofold), and reduced residence time. Castano, Crespo, and Torres (Citation2019) stated that there is an interaction effect between incubation time and temperature on the delignification process of lignocellulosic biomass. Higher temperatures may promote higher degradation of complex sugars (or polysaccharide) (Lu et al. Citation2018). Thereby, it explains the higher performance of combining biological with thermal pre-treatment. Another example is combining fungal with dilute acid pre-treatment, which can enhance biogas production (Saini and Sharma Citation2021). A combination of two biological pre-treatments, such as anaerobic ruminal fungi with fermentative bacteria, increased methane yield from the AD of wheat straw and mushroom spent raw by 3- and 3.5-fold, respectively (Ferraro et al. Citation2018). In addition, using white-rot fungi combined with chemical or physical pre-treatment was effective for delignification of straw biomass (Ghaffar, Fan, and McVicar Citation2015). Combining physical pre-treatment (i.e particle size reduction with grinding) with fungal pre-treatment may provide better lignin bond disruption, thus producing a higher hydrolyzed product yield (Wagle et al. Citation2022).
Tian, Fang, and Guo (Citation2012) stated that a combination of fungal pre-treatment with chemical pre-treatments (i.e. ionic liquids, supercritical CO2, organic solvents, and diluted acids) could enhance the transformation of lignocellulosic biomass into bioenergy and bioproducts. With this combination, problems occurred during fungal pre-treatment can be resolved. Thus, these findings confirmed that combination pre-treatments might offer effective ways prior to the AD route. Such an approach could completely breakdown the lignocellulosic materials in biomass feedstock, hence higher biogas and methane production.
4.1.2. Mushroom cultivation pre-treatment
In mushroom cultivation pre-treatment, the edible mushroom is grown in lignocellulosic biomass to promote degradation by mycelium penetration (to increase the pore size) and by disrupting functional linkages between polysaccharides, cellulose, and lignin (Pérez-Chávez, Mayer, and Albertó Citation2019; Zou et al. Citation2020). This breakdown is occurred due to the presence of lignocellulolytic enzymes produced by the edible mushrooms (Zou et al. Citation2020). The edible mushrooms (i.e. Agaricus bisporus, Pleurotus ostreatus/oyster mushroom, Lentinula edodes) are known to be effective in transforming lignin and cellulose into simple sugars and small compounds, further used as food or carbon source for the mushrooms (Albertó Citation2017; Pérez-Chávez, Mayer, and Albertó Citation2019; Zou et al. Citation2020). In detail, mushroom cultivation pre-treatment is shown in . Moreover, edible mushrooms can also be grown on compost or during the composting process, converting complex organic carbon into simpler forms and releasing many aliphatic compounds (O/N alkyl) (Triyono et al. Citation2019). One of the aliphatic compounds (i.e. humic acid) increases the ability to degrade lignin structure (Amir, Hophmayer-Tokich, and Kurnani Citation2016). Therefore, being fibrous in nature, a common practice found in the literature is using OPEFBs for mushroom cultivation. This practice is also seen as a different type of mushroom pre-treatment as it employs various mushroom species before utilising the biomass for further valorising routes, as shown in .
For instance, OPEFBs were potential as a substrate for oyster mushroom cultivation because they can stimulate the mushrooms’ growth (Sudirman et al. Citation2011). Mmanywa and Mshandete (Citation2017) reported that treating oil palm waste with Coprinus cinereus resulted in higher methane production (0.43–0.49 m3 CH4/kg VS) than untreated oil palm waste (0.33 −0.34 m3 CH4/kg VS). These findings indicate that such unique approaches can provide food (i.e. edible and medicinal mushrooms) and fuel (i.e. biogas), thus consequently reducing the environmental problems created by oil palm waste. Purnomo, Suprihatin, and Hasanudin (Citation2018) also adopted a similar approach to treating OPEFBs as mushroom cultivation media before feeding the biomass to the AD system. Their results also suggested that cultivating mushrooms can reduce lignin content by 2.67%, hemicellulose by 6.33%, and cellulose by 6.55%. Such an approach altered the lignocellulosic structure of OPEFBs, thus helping the microorganism consortia in the digester to easily degrade the organic matter into biogas or methane. After being treated as mushroom media, the study also reported a high biogas production from OPEFBs. This result agrees with a study by Triyono et al. (Citation2019), who also stated that using OPEFBs as a growing media for mushroom cultivation can be used as an alternative approach to enhance lignin degradation and add more economic benefits through mushroom production. A study by Saidu et al. (Citation2014) also demonstrated that oil palm mesocarp fibre could be used for oyster mushroom cultivation. Thereby, enhancing the degradation of complex material (i.e. lignin) and exposing more easily degradable organic material to microorganism consortia. Further digestion of treated oil palm mesocarp fibre produced higher biogas production than untreated biomass samples. Such finding also indicates that biological pre-treatment using mushrooms may increase the AD system's efficacy should the optimum conditions are achieved. Mamimin et al. (Citation2021) studied straw mushroom (Volvariella volvacea) cultivation as a strategy to enhance biodegradability and methane production from OPEFBs. Similar to other studies, their results showed that mushroom cultivation pre-treatment improved biodegradability by twofold, with an increase in methane yield by 4.6-fold compared to untreated OPEFBs. The visual morphology of pre-treated OPEFBs also demonstrated that the biomass surface was fractured by the mycelia, allowing accessibility for microorganisms or enzyme to break down cellulose and hemicellulose of OPEFBs.
Several studies have also reported the combination of mushroom cultivation pre-treatments for better delignification results. Chen et al. (Citation2022a) reported that Shiitake cultivation with an additional low concentration of nitrogen, following physical pre-treatment (i.e. particle size reduction), increased the delignification of wood biomass by 41.5%. Temu et al. (Citation2016) studied the combined pre-treatment of mixed oil palm waste prior AD process. After physical pre-treatment (i.e. particle size reduction), biological pre-treatment using Coprinus cinereus (also known as edible and medicinal mushrooms) was employed. The results showed a decrease lignin content of the waste by 23%. FTIR analysis results further indicated this by the loss of CH2 vibration, along with the removal of cellulose and hemicellulose. Their findings also showed that the methane yield increased to 0.287 m3 CH4/ kg VS. There has been very limited information available on this strategy; hence further in-depth studies are essential.
4.1.3 Microbial pre-treatment
The microbial pre-treatment can be carried out using a single- and co-culture (i.e. microbial consortia) to degrade lignocellulosic material. However, a study by Baghbanzadeh et al. (Citation2021) reported high efficacy of microbial pre-treatment using mixed culture than single species, as it can reduce metabolite repression and feedback regulation. Thus, various microbial species can simultaneously degrade lignin, cellulose, and hemicellulose under anaerobic or aerobic condition (Ravindran and Jaiswal Citation2016). The microbal pre-treatment is illustrated in . Furthermore, in the existence of oxygen, facultative anaerobic and aerobic microorganisms can degrade lignin polymers. Microbial pre-treatment is more economical than enzyme pre-treatment but slower (Baghbanzadeh et al. Citation2021); and needs a large sterile area (Abraham et al. Citation2020). Abraham et al. (Citation2020) stated the advantages of microbial pre-treatment, including low-energy consumption, no formation of inhibitory compounds, lignin, and hemicellulose hydrolysis, and cellulose structure alteration. Various studies also reported different microbes for pre-treating the OPEFB biomass, as shown in .
Several studies reported using a single-culture of microorganism as pre-treatment option to enhance the biodegradation of OPEFBs. For instance, Jumali and Ismail (Citation2017) studied the microbial pre-treatment of OPEFBs biomass using Ganoderma boninense spores under a solid-state fermentation system. The study revealed that OPEFBs have higher lignin degradation after being treated with Ganoderma boninense spores for 7-day incubation, with ∼62% lignin removal. While only ∼5.1% lignin degradation was observed in untreated OPEFBs. This finding confirmed that increasing the number of microbes used and the incubation period can increase lignin removal. Thus, the microbial pre-treatment using Ganoderma boninense spores can be used as an alternative to enhance the bioconversion of OPEFBs biomass into fermentable sugars, resulting in more routes for valorisation. While Salihu and Alam (Citation2016) reported that pre-treatment of OPEFBs using Bacillus sp. enhanced biogas production by 95%. A study by Nurika et al. (Citation2022) also found that using a single culture of lignin-degrading bacteria (i.e. Comamonas testosteroni, Agrobacterium sp., Lysinibacillus sphaericus, and Paenibacillus sp) increase delignification in the range of 12.24% - 25.84%. This is also followed by improved methane production from 0.005 m3/kgVS (untreated OPEFBs) to 0.042 m3/kgVS (C. test), 0.016 m3/kgVS (Agrobacterium sp.), 0.021 m3/kgVS (L. sphaericus), and 0.029 m3/kgVS (Paenibacillus sp), respectively.
The biological pre-treatment was also researched towards adding co-culture microorganisms to improve biodegradation and biogas or methane production. Shahriarinour et al. (Citation2011) stated that biological pre-treatment of OPEFBs with effective microorganisms (EM) significantly enhanced biodegradation due to increase in cellulose production by 13%. Another study found that pre-treatment using a bio-augmentation of Bacillus subtilis and mixed methanogens in varying ratios was highly effective and increased methane yield from the anaerobic mono-digestion of OPEFBs (Chin et al. Citation2020). They reported that Bacillus subtilis, a major industrial microorganism, can effectively degrade cellulose and adapt to aerobic and anaerobic conditions with a low nutrient requirement, fast-growth rate and industrially safe. Walter et al. (Citation2015) found that pre-treatment of OPEFB using a combination of microorganisms from a genus of hydrogenotrophic (i.e. Methanoculleus and Methanobrevibacter) and genus of acetoclastic (i.e. Methanosarcina) can produce much higher biomethane. Shah et al. (Citation2019) showed that using mixed cultures of Bacillus sp. to pre-treat rice straw increased the delignification rate (indicated by a reduced lignin content), further increasing methane production by 76%. Yan et al. (Citation2012) reported that using mixed cultures of lignocellulolytic microorganisms as pre-treatment prior to the AD process improved methane production by 20%. This was possibly due to the higher efficacy of delignification, resulting in a higher breakdown of lignin, hemicellulose, and cellulose content. Ali, Abomohra, and Sun (Citation2017) also found that co-culture microorganism pre-treatment improved the delignification of sawdust, resulting in more production of biogas (24.5%) and methane (72.6%), respectively. These findings highlighted the application of co-culture microorganisms as a pre-treatment option that offer a more efficient and greener route for better valorisation of lignocellulosic biomass. Another study of co-culture microbial pretreatment was also done by Suksong et al. (Citation2019a), which resulted in the biodegradation efficiency of 62% (i.e. Clostridiaceae rich consortium) and 82% (i.e. Lachnospiraceae rich consortium), and produced maximum methane yields of 0.252 and 0.349 m3 CH4/kg VS, respectively.
A combination of microbial pre-treatment with other green pre-treatment options has also been investigated. Md Razali et al. (Citation2018) studied pre-treatment of OPEFBs using Clostridium acetobutylicum ATCC 824 with the addition of cellulase enzyme. The study revealed that OPEFBs were slowly degraded by Clostridium acetobutylicum ATCC 824. The addition of cellulase enzyme enhanced the delignification, indicated by increased production of fermentable sugars. Shahriarinour et al. (Citation2011) stated that pre-treatment of OPEFBs with effective microorganisms (EM), in combination with chemical and hydrothermal pre-treatment, significantly reduced lignin content from ∼10% to 4.2%, thus improving the efficacy of biodegradation. Zhong et al. (Citation2016) also found that combining co-culture microbial with fungi pre-treatment trigger a greater synergistic effect, resulting in higher biodegradation of lignocellulosic materials in wheat straw. This is parallel to an increase in biogas and methane production by 39.24% and 80.34%, respectively. They suggest that microbial-fungi pre-treatment offered a more complete degradation of lignocellulosic materials, resulting in a greater accumulation of easily degraded organic compounds. They claimed that combining biological pre-treatment could shorten the initial digestion period in the AD system and fasten the biogas and methane production. These findings suggest that a combination of microbial pre-treatment with other alternative pre-treatment may offer better delignification of OPEFBs, leading to higher efficacy in the AD process.
4.1.4. Enzymatic pre-treatment
Enzymatic pre-treatment has been highlighted as an effective pre-treatment to enhance the biodegradability of lignocellulosic biomass (Agabo-García et al. Citation2019); which employ a pure (or single) enzyme (Ravindran and Jaiswal Citation2016); and enzyme cocktail (Lopes, Ferreira Filho, and Moreira Citation2018). In detail, the enzymatic pre-treatment is illustrated in . Various enzymes (i.e. lipases, glucanases, and proteases) are widely used in the pre-treatment of lignocellulosic biomass. However, other enzymes (i.e. Lac, MnP, and versatile peroxide/VP) oxidise specific components of the lignin structure in lignocellulosic biomass (Ravindran and Jaiswal Citation2016). For instance, MnP oxidises the phenolic structure of lignin, LiP targets non-phenolic components, and VP can oxidise phenolic and non-phenolic structures (Falade et al. Citation2017). MnP requires the presence of a chelating agent to stabilise Mn (III) and oxidise more reactive phenolic structures (Hofrichter Citation2002). Furthermore, removing phenol could enhance microbial growth, improving the fermentation process's efficacy and reducing time in the lag phase. The factor influencing enzymatic pre-treatment include biomass feedstock's source and characteristics (Agabo-García et al. Citation2019). Enzymatic pre-treatment also depends on the enzyme ratio, reaction time, temperature, and pH of the solution. The lignin degradation depends on these parameters, which must be optimised for desirable products (Amin et al. Citation2010). The main advantages of the enzymatic pre-treatment include high pure monomer sugar yields (mainly glucose), lower environmental impact, and mild reaction conditions compared to the chemical acid hydrolysis routes (Hamzah, Idris, and Shuan Citation2011); low-energy demand fast process, cellulose structure alteration, and partial hydrolysis of hemicellulose (Abraham et al. Citation2020). Furthermore, enzyme is selective to delignify the lignin content much faster than microbial pre-treatment (Rizal et al. Citation2018). However, the techno-economic feasibility of the process still needs to be assessed and verified if intended for the scale-up (Chiesa and Gnansounou Citation2014); due to the high cost of enzymes (Abraham et al. Citation2020).
Many studies have reported the positive impact of enzymatic pre-treatment (using a single enzyme) to enhance the biodegradability of OPEFBs. The reported enzymes on OPEFBs include a combination of Accellerase, Multifect Xylanase, Multifect Pectinase, cellulase, β 1–4 glucosidase, etc. (Ariffin et al. Citation2008; Hamzah, Idris, and Shuan Citation2011; Lau et al. Citation2010). A previous study by Amin et al. (Citation2010) demonstrated that pre-treatment of OPEFBs using LiP and MnP enzymes reduced lignin by 71.69% and 67.94%, respectively. Another study comparing enzymatic hydrolysis and ammonia fibre expansion methods on OPEFBs found that, at the optimum conditions, the enzymatic pre-treatment could achieve 90% of the total cellulosic ethanol yield within the 72-hours of the pre-treatment (Lau et al. Citation2010). Ishmael et al. (Citation2016) studied enzymatic pre-treatment on OPEFBs using the laccase enzyme. Their study reported that increasing laccase enzyme concentration from 5 to 20 IU/g has increased the delignification, indicated by an increase in total sugar from 2.4 to 3.5 mg/mL and in weight loss from 6.3% to 20.1%, respectively. This study also demonstrated that various factors might influence the efficacy of enzymatic pre-treatment of OPEFBs, including substrate's concentration, substrate's particle size, pH, incubation time, and temperature. In this study, for example, at a pH of 5, the laccase enzyme achieved the optimum lignin removal. Therefore, it can be said that using the laccase enzyme can be a safe option for pre-treatment of OPEFBs prior to AD or other potential bioenergy utilisation. Another study by Deb et al. (Citation2019) reported that using cellulase enzyme in treating oil palm mixed waste and POME increased the hydrolysis rate and damaged the POME structure. Thus, it is easily accessible for degradation, leading to an increase in the overall production of sugars. A similar study by Weide et al. (Citation2020) has also reported that adding cellulase enzyme to the AD process improved methane yields of agricultural wastes (i.e. silage, straw, and cattle manure), ranging from 0.3% to 21.1%.
The positive impact of cocktail enzymatic pre-treatment on improving biogas and methane yield from lignocellulosic biomass has also been highlighted. For instance, Weide et al. (Citation2020) studied enzymatic pre-treatment on a mixture of cattle manure and maize silage (CMM) using two cocktail enzymes, including EM4 (cellulase + xylanase + beta-glucanase) and EM5 (xylanase + glucosidase + endo-pectinase). Their study found that using these cocktails enzyme improve methane yield from 0.559 m3/kgTS to 0.582 m3/kgTS (CMM + EM4) and 0.599 m3/kgTS (CMM + EM5), or by 11.0% and 6.8%, respectively. Another study reported that applying cocktail enzyme (i.e. endoglucanase + xylanase + pectinase) pre-treatment on sugar beet pulp silage produced 27.9% higher biogas production (Ziemiński and Kowalska-Wentel Citation2015). Treating spent hops with cocktail enzyme (i.e. endoglucanase + xylanase + pectinase) pre-treatment increased biogas production by 13% (Ziemiński, Romanowska, and Kowalska Citation2012). A previous study also showed that enzymatic pre-treatment on sorghum forage using a cocktail enzyme (i.e. endoglucanase + exoglucanase + xylanase) resulted in 15% higher methane yield (Rollini et al. Citation2014). Using cocktail enzyme (i.e. MnP + versatile peroxidase) could improve methane production from corn stover by 17% (Schroyen et al. Citation2014); while using a cocktail enzyme (i.e. cellulase + cellobiase) increase methane yield from switch grass by 38.9% (El-Mashad Citation2015). Improvement in biogas and methane production was because cocktail enzyme completely degraded the lignocellulosic materials, as their component requires different enzymes to degrade (Lopes, Ferreira Filho, and Moreira Citation2018). For example, cellulose compound in the cell wall of lignocellulosic materials requires cellulase enzyme to degrade and often need endoglucanases to hydrolyze into cellobiose, which further hydrolyzes with β-glucosidase to release glucose. Lignin needs enzymes (such as laccase) to be oxidised into phenolic and aromatic compounds. Furthermore, to improve the performance of enzymatic pre-treatment, applying a cocktail enzyme could be proposed to enhance the AD of OPEFBs. Yet, limited information is available, further studies need to be investigated for pre-treating OPEFBs since.
Improving the performance of the AD system using a combination of enzymatic with other pre-treatments has been highlighted in various studies. Ahmad Rizal et al. (Citation2018), for example, studied the combination of physical (i.e. particle size reduction), thermal (i.e. superheated steam), and enzymatic (i.e. addition of laccase enzyme) pre-treatment significantly reduced lignin content of OPEFBs by 38.7%. This combination also resulted in a higher glucose yields (increase by 4.6-fold), which may enhance biogas and methane yield if used as feedstock in AD. Lau et al. (Citation2010) studied ammonia fibre expansion (AFEX) and physical pre-treatment (i.e. particle size reduction) combined with enzymatic pre-treatment (i.e. cocktail enzyme of Accellerase, Multifect xylanase, and Multifect pectinase). Their study showed that, the combination pre-treatment, the hydrolysis yield increased by 90% over 7 h and the recalcitrance compounds could be reduced without damaging the fermentable materials. Rollini et al. (Citation2014) also found that combining alkaline and cocktail enzymatic pre-treatment on sorghum forage improved methane yield by 37%, much higher than using cocktail enzymatic pre-treatment alone (15%). Combination enzymatic with other green pre-treatments needs to be explored further for OPEFBs.
4.1.5. Ensilaging pre-treatment
The concept of ensiling is not new but has been practiced by farmers and experimented with by the academic community on crop digestibility since the late 1970s (Coblentz and Akins Citation2018). Ensilaging technology is a conventional technology widely used to store animal feed (Ravindran and Jaiswal Citation2016; Tanjore, Richard, and Marshall Citation2012). The ensiling process is essentially simple yet can become complex due to numerous interactions among the biomass and the microbial species involved. The primary desired product from ensiling is the conversion of the biomass sugars into lactic acid in an anaerobic fermentation setup. Nevertheless, complications arise due to the aerobic conditions at the start and end of the process. Furthermore, simple sugars are not the only substrates available in the biomass that either get converted or become inhibitory to the ensiling bacteria (Mohd-Setapar, Abd-Talib, and Aziz Citation2012; Villa et al. Citation2020). They stated that the different phases of ensiling are classified mainly into six: phase 1 – initial aerobic phase, phase 2 – anaerobic fermentation phase, phase 3 – bring phase 2 to an end, phase 4 – lactic acid formation, phase 5 – material storage, and phase 6 – aerobic decomposition. In details, the ensiling process is illustrated in . Typically used as a method for forage conservation, the investigation is proceeding on ensiling by researchers/academics or industry on diversified crops around the world. Ensiling offers the best means for preserving the nutritive value of given biomass from the point of harvest to storage until further valorisation (Muck Citation2012). Ravindran and Jaiswal (Citation2016) reported that naturally occurring bacteria generate enzymes that degrade the lignocellulosic material into fermentable sugar suitable for animal feedstock during ensiling. Furthermore, they found that Lactobacillaceae is a dominant species in the microbial consortium during ensiling. Factors influencing ensiling pre-treatment include substrate composition, harvest time, additives, solid content, ensiling duration, moisture content, pH, and loss of dry matter (DM) (Sieborg et al. Citation2020). The study reported that the amount of butyric acid present during the incubation period required much attention, as indicated by the metabolic activity and significant losses to DM.
As discussed earlier, OPEFBs have high water retaining capacity, therefore, they can be degraded naturally using ensiling. Carrere et al. (Citation2016) claimed that ensiling had been widely used to store energy crops; however, as AD pre-treatment, the ensiling advantages include low energy demand and scalability. Several studies, as shown in , have also reported the positive effects of ensiling on improving the digestability and enhancing the biogas or methane potential from various biomass feedstocks such as sorghum (Sambusiti et al. Citation2013a; Citation2013b); wheat straw (Rincón, Banks, and Heaven Citation2010; Citation2012); corn straw (Zhong et al. Citation2011); corn stalk (Menardo et al. Citation2015; Zhang et al. Citation2018; Zhao et al. Citation2016); grass (Abu-Dahrieh et al. Citation2011; Quiñones et al. Citation2011); maize (Evranos and Demirel Citation2015; Oliva-Merencio et al. Citation2015); tall fescue (Feng et al. Citation2018); and sugar beet (Ahmed and Kazda Citation2017). For instance, Mayulu (Citation2014) studied that ensiling the oil palm industry residues increased their digestibility due to a reduction in crude fibre, as well as an increase in crude protein and nutrient content.
Several studies have emphasised the minimal effect of ensiling pre-treatment alone in improving AD performance. Therefore, combining different options is advisable for practical and commercial applications. A study by Vervaeren et al. (Citation2010), for instance, highlighted that ensiling of maize substrate with the addition of biological additives (i.e. homo- and hetero-fermentative strains, yeasts, and enzymes) significantly improved the ensilage quality and slightly enhanced the biogas and methane production in the range of 10.1% to 14.7%. They further added that adding biological additives during ensiling may also improve the hydrolysis stage in the AD process (i.e. degradation of complex organic materials). Additional research has been carried out to ensure feasible application of ensiling pre-treatment. Yunqin, Dehan, and Lishang (Citation2010) investigated that pulp and paper sludge pre-treated with combining mushroom cultivated combined enzymatic pre-treatment (e.g. laccase enzyme) increased delignification by 34.2%. While (Li et al. Citation2019a) reported that combining ensiling with enzymatic pre-treatment (i.e. using Acremonium cellulase) or with microbial pre-treatment (i.e. using L. plantarum A1) alone improved the delignification of corn stalk. However, a combination of ensiling with Acremonium cellulase and L. plantarum A1 performed has superior performance in the degradation of lignocellulose. Hence, this combination can be proposed as a suitable pre-treatment option for bioenergy production. Yet, there are limited studies and data available for OPEFBs. Thus, further investigation in this area is essential.
4.2. Co-digestion of OPEFBs
Co-digestion combines two or more substrates during the AD process as an alternative to increasing biogas production when mono-digestion is challenging to achieve maximum methane yield (Aziz et al. Citation2020). Various studies have emphasised that co-digestion is one of the sustainable approaches to enhancing biogas/methane yield and tackling waste problems. The addition of co-digestion substrates in the AD system could enhance the microbial’ presence and activity, add nutrient supply (that the main biomass is lacking), improve nutrient balance, improve potential detoxification from toxic substances, and provide additional carbon sources (i.e. readily biodegradable substances) (Chen et al. Citation2016; Jaman et al. Citation2022; Jin et al. Citation2018; Panichnumsin et al. Citation2010; Pastor et al. Citation2013; Suhartini et al. Citation2022a). Studies reported that co-digestion promotes co-metabolism between co-substrates with anaerobic consortia through the supply of carbon/energy sources or electron donors, thus enhancing the ability of the microorganism to degrade the organic matter present in the feedstock mixture (Gu Citation2021; Li et al. Citation2021a; Citation2021b; Suhartini et al. Citation2022a). Furthermore, co-digestion of OPEFBs could efficiently stabilise the carbon and nitrogen content in the feedstock to balance the C/N ratio to start up the digestion (Nurliyana et al. Citation2015).
Various studies have also emphasised co-digestion of OPEFBs with other biomass substrates as green strategies for improving biogas and methane yields, as shown in . For instance, in a study conducted by Suksong et al. (Citation2019b), co-digestion of OPEFBs with sludge from liquid-AD (L-AD) was carried out using solid-state AD (SS-AD) under mesophilic and thermophilic conditions. Their study demonstrated that co-digestion operated at thermophilic temperature has superior performance (i.e. process stability and biogas/methane production) than at mesophilic temperature, giving the average methane production of 120 m3 CH4/ton of OPEFBs. Liew et al. (Citation2021) added that anaerobic co-digestion of OPEFBs with POME at a ratio of 0.6:1 under mesophilic and thermophilic conditions resulted in the highest methane production (i.e. more than 2-fold higher) compared to anaerobic mono-digestion of OPEFBs or POME. Their study suggests that POME (as substrate rich in nitrogen) provide additional nutrient to the microorganism consortia and the synergistic effect between the two substrates, thus enhancing the AD process stability. However, they reported that co-digestion of alkaline pre-treated OPEFBs (at 8% w/v of NaOH solution and a ratio of 1:19 (OPEFBs:NaOH)) with POME at a ratio of 0.31:1 and 0.45:1 did not significantly improved the methane yield. Their finding suggested that the mild concentration of NaOH during alkaline pre-treatment has no effective impact on enhancing delignification and biodegradation.
Table 6. Performance on anaerobic co-digestion of OPEFBs with biomass substrates from several studies.
Pre-treatment prior to anaerobic co-digestion is often required for higher biogas and methane yield. Mamimin et al. (Citation2021) reported that anaerobic co-digestion of mushroom pre-treated OPEFBs with POME significantly enhanced biodegradability by 90.8% and increased methane yield from 0.281 m3CH4/kgVS (mushroom pre-treated OPEFBs alone) to 0.405 m3CH4/kgVS. In addition, a previous study by O-Thong, Boe, and Angelidaki (Citation2012) compared the methane production of mono- and co-digestion of OPEFBs with POME, with and without hydrothermal pre-treatment. OPEFBs were treated with hydrothermal (at 230°C for 15 min.) and NaOH (1% w/w), following mixing with POME at various ratios with an initial loading of 46 g VS/L. Their study reported that co-digestion of untreated OPEFBs with POME under thermophilic conditions enhanced biodegradability and increased methane production in the range of 25–32% (or 0.276–0.340 m3 CH4/kg VSadded) at mixing ratios of 0.4:1–2.3:1. While co-digestion of treated OPEFBs with POME at a ratio of 6.8:1, the highest methane production was achieved at a value of 0.392 m3 CH4/kg VSadded (or 82.7 m3 CH4/ton) with an electrical potential of 330 kWh. A study reported by Kim et al. (Citation2013) also shows a better co-digestion performance of treated OPEFBs (i.e. drying and grinding) with POME over mono-digestion, resulting in a methane yield of 0.4 L/kg of the substrate used. Moreover, Nurliyana et al. (Citation2015) also highlighted that co-digestion of treated OPEFBs (i.e. drying and particle size reduction) with POME at a ratio of 24%:76% produced the highest methane (0.203 m3 CH4/kg VSadded) with an electrical potential of 196 kWh.
The above findings confirmed that OPEFBs are highly potential as feedstock for AD. The biological pre-treatments, using co-culture microorganisms and cocktails enzyme, combined with other pre-treatment resulted in a higher reduction of lignin content and increased biogas and methane yields. These pre-treatments are needed to completely break down the complex structure of lignin bonds, allowing easier and faster access for microorganisms or enzymes to degrade cellulose and hemicellulose (Yang and Wyman Citation2008). Also, co-digestion of OPEFBs with other potential substrates may offer higher efficacy of the AD process due to additional nutrient and co-metabolism effect in the AD reactor. Thus, it can improve the microorganism's ability to effectively degrade and consume the organic materials in the mixed substrates, as highlighted in several studies (Gu Citation2016; Suhartini et al. Citation2022a; Zhang et al. Citation2021). Therefore, biological pre-treatments and co-digestion can be highlighted as alternative strategies to increase the biogas or methane yields of OPEFBs. Yet, these options still need in-depth investigation to find cost-effective, energy-saving, and eco-friendly commercial operations.
5. Potential future opportunities
The current status of OPEFBs for commercial application in the bioenergy sector is still ongoing research (Maryana et al. Citation2021; Purwandari et al. Citation2013; Risdianto, Kardiansyah, and Sugiharto Citation2016; Sudiyani and Hermiati Citation2010; Sudiyani et al. Citation2013). The oil palm oil mostly treated OPEFBs for private use, i.e. fertilizer via natural decomposition, steam for boilers, fuels for combustion machines, or burned (Nurdiawati et al. Citation2015; Srasri et al. Citation2022). But, OPEFBs have the potential to be used for bioenergy production, biofertilizer, and other bio-products. The findings for exploring the potential of OPEFBs are urgent due to the potential adverse impacts of underutilised or directly disposed of OPEFBs (Harahap et al. Citation2020b; Khangkhachit et al. Citation2021). One of the main sectors which are very close to human activity is energy and power utilisation; thus, using OPEFBs as feedstock on bioenergy production and collaborating with the well build technology such as the AD is advised (Liew et al. Citation2021; Mamimin et al. Citation2021; Tepsour et al. Citation2019). The use of biological pre-treatment is proposed, as the enzyme activity contribution and solid content decrement played key roles in degrading the lignocellulose layer. Hence, the feasible and sustainable pre-treatment selected should be based on the following consideration (Cai et al. Citation2021; Ravindran and Jaiswal Citation2016; Taherzadeh and Karimi Citation2008): (1) aid a high process efficiency; (2) avoid hemicellulose or cellulose destruction; (3) less or no formation of inhibitory compounds; (4) energy-saving process; (5) little or no chemical/additives addition; (6) shorter operation time; (7) no release of pollutants and toxic by-products; (8) cost-effective process (i.e. less cost for particle size reduction, for pre-treatment reactor construction or relevant equipment, for microbial culture or enzyme, for storage, etc.); (9) lower operational and capital cost; and (10) eco-friendly process.
In Indonesia, therefore, based on the aforementioned findings, it is suggested that AD of OPEFBs can potentially be applied using four scenarios. Prior to AD in all scenarios, pre-treatment of OPEFBs were essential to enhance delignification, which enhanced biogas and methane production. Considering various technical benefits and challenges stated in , thus mushroom cultivation pre-treatment is advised in all proposed scenarios. Compared with other biological pre-treatment, cultivation mushroom pre-treatment has recently been highlighted to have low capital cost, no reliance on chemicals or additives, less energy consumption, no inhibitory or toxic compound formation, mild operating condition, and scalability. Before mushroom cultivation pre-treatment, OPEFBs are subjected to physical treatment of particle size reduction. Previous studies have reported that particle size reduction of OPEFBs prior to any biological pre-treatment could advocate better delignification and biodegradation (Ahmad Rizal et al. Citation2018; Chen et al. Citation2022a; Temu et al. Citation2016).
Therefore, in all scenarios, the AD system was combined with physical pre-treatment (i.e. particle size reduction) and mushroom cultivated pre-treatment (i.e. edible mushroom). Then, it is followed by the biogas utilisation unit, digestate storage, digestate treatment, and digestate utilisation. Scenario 1 is anaerobic mono-digestion of OPEFBs with a single-stage configuration using a CSTR. Scenario 2 is anaerobic co-digestion of OPEFBs with other biomass substrates (with TS > 20%) using a single-stage CSTR configuration. Scenario 3 is anaerobic co-digestion of OPEFBs with biomass substrate (with TS < 20%) using a single-stage CSTR system. This scenario aimed to facilitate anaerobic co-digestion of OPEFBs with wastewater containing high organic pollutants (such as POME). For better homogenisation, mixing is essential before the feedstock entering the AD reactor after the combined pre-treatment. Furthermore, the moisture content in the feedstock mixture should also be controlled for better biogas and methane yields. Scenarios 2 and 3 could be applied if other local biomass substrates or wastewater can provide better synergistic effects, thus improving biogas and methane production. Scenario 4 is anaerobic co-digestion of OPEFBs using two-stage system of CSTR and UASB. In the first stage, using a CSTR bioreactor, with similar aims as stated in Scenarios 1 and 2. In the second stage, the UASB bioreactor is used because it is suitable for liquid substrates with high amounts of dissolved organic compounds and lipids. Therefore, the suitable feedstock used for this configuration is co-digestion of OPEFBs with POME. Using a two-stage configuration can produce biogas with the highest CH4 yield. Furthermore, the stability of the AD process is easier to be achieved and efficiently reduces COD concentration in POME. Thus, with this scenario, it can tackle two waste problems, and at the same time, aiming to produce higher biogas or methane yields. The scheme of the proposed the sustainable AD of OPEFBs system is shown in .
Figure 6. Proposed scenario for the sustainable AD of OPEFBs with integrated multi-product biorefinery concept in Indonesia.
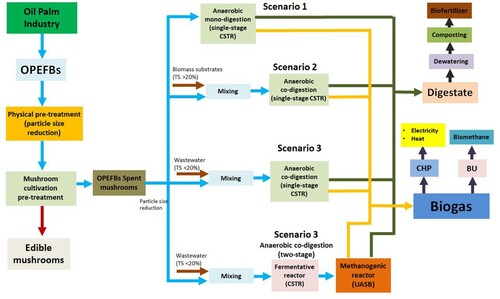
Despite multi-stage pre-treatment, the proposed scenarios may offer feasible and sustainable of using OPEFBs for multi-product production. This is also consistent with the integrated multi-product biorefinery and circular economy concept. OPEFBs are utilised as feedstock for edible mushroom cultivation. Then, after mushroom harvesting, the spent OPEFBs substrates are used for feeding the AD digester to produce biogas. The resulting biogas can be converted into electricity and heat (using a combined and heat power unit) or into biomethane (using a biogas upgrading system). The residual digestate can be valorised as biofertiliser in oil palm plantations or nearby agricultural farm area. Thus, a closed-cycle of OPEFBs valorisation can be achieved with minimal or no waste generation. Previous studies have also emphasised that combining mushroom cultivation pre-treatment with AD technology may support sustainable integrated biorefinery and a circular economy of agro-industrial waste and agricultural residues through the production of multi-products (i.e. edible mushroom, biogas, biofertiliser, and vegetable production) (Kumar et al. Citation2022; Pérez-Chávez, Mayer, and Albertó Citation2019; Richard et al. Citation2020). Wagle et al. (Citation2022) stated that multi-product biorefinery provide optimal valorisation of lignocellulosic biomass with less waste generation and carbon emission. They suggested that multi-product biorefinery could promote the sustainable biofuels production, trigger a more sustainable agricultural practice, strengthen energy and food security, and provide additional income to the local residents. However, emerging research on improving the process's efficacy, developing the plant's design, investigating the techno-economy and sustainability analysis, and examining the commercialisation prospects is critical. Hence, the sustainable and multi-product biorefinery of these proposed scenarios could maximise the value generation and the economic prospect of OPEFBs.
Furthermore, waste biorefineries offer sustainable solutions to limit the final disposal of waste by promoting their valorisation through bio-based production chains (i.e. bioenergy and value-added products) to foster long-term economic, social, and environmental benefits (Atabani et al. Citation2022a; Citation2022b; Hoang et al. Citation2022a; Citation2022b). Similarly, Hoang et al. (Citation2022c) stated that waste-to-energy (W2E) facilitates a promising solution to global problems in energy supply and environmental pollution. They stated that integrated energy systems, efficient design, lean manufacturing system, sale of by-products, and the high-capacity plant should be incorporated with W2E technology to obtain maximum economic and environmental benefits. Moreover, various studies have also emphasised different solutions for the sustainable development of bioenergy and circular economy from waste and biomass. For instance, Yetri et al. (Citation2020) found that cocoa pod waste (CPW) is highly abundant in Indonesia. Their study revealed that activated carbon monoliths from CPW have superior performance as a supercapacitor electrode. They claimed that this strategy could offer environmental benefits (i.e. reducing environmental pollution) and economic benefits (i.e. reducing imported carbon electrodes). Lee et al. (Citation2022) reported that combining spent coffee grounds (SCG) with water chestnut shell biochar could offer energy-saving drying, thus optimising the valorisation of SCG as a coal co-firing fuel. Atabani et al. (Citation2022a) evaluated the biorefining of SCG in combination with other biomass (i.e. coffee silverskin, pine sawdust, crude glycerol, coal fine, and saw) for fuel pellet production offers a higher combustion efficiency and a lower emission of CO2 and NOx. They suggested that this strategy may reduce the volume of organic wastes via the production of bioenergy and high-value-added products, thus enhancing the circular economy in the global world through waste biorefinery. Hoang et al. (Citation2022b) also reported that carbohydrates extracted from various biomass/waste feedstock (i.e. agricultural residues, food crops, forest residues, municipal solid waste/MSW, and algae) are potential for the production of furan-based biofuels (i.e. 2,5-dimethylfuran (DMF)). This approach offers a green and prospective solution to produce bioenergy, reduce fossil fuel dependency, and preserve sustainable development globally.
Some management solutions for energy are considered essential to support sustainable bioenergy production. Taking a case study in Vietnam, sustainable bioenergy production via biomass gasification could be achieved if there are supportive Government policies in technology, infrastructure, investment, education, and finances (Hoang et al. Citation2022a). Furthermore, various approaches to improve biomass-based bioenergy production and management could be applied by incorporating digitalisation, system information, and intelligent infrastructure (Nguyen et al. Citation2021a; Citation2021b). For instance, the Internet of Things (IoT) application may offer a solution to improve energy efficiency, thus reducing the operational costs and the environmental impact (Nguyen et al. Citation2021a). Furthermore, Chen et al. (Citation2022b) studied a type of artificial intelligence (AI), specifically machine learning (ML) approaches using multivariate adaptive regression splines (MARS) and artificial neural network (ANN) to predict the torrefaction severity index (TSI) of various torrefied biomass feedstock. Their study revealed that this method could potentially be applied to estimate the scaling-up of bioenergy production via torrefaction pathways. Another study by Bakır et al. (Citation2022) also reported that the marine predators algorithm (MPA) could be implemented in bioenergy and fossil fuel-based energy generation. This approach offers a solution to forecast the greenhouse gasses (GHG) emissions, hence may assist the decision- and policy-maker in establishing an energy-efficient clean power plant in India. These findings suggest that using the framework of IoT or AI has emerged as an attractive concept to save energy while improving energy production at the same time.
However, some limitations exist to establishing sustainable bioenergy and circular economy via the AD of waste or biomass. These include uncertainties and competition of biomass feedstock, cost of technology, low level of social awareness, high capital and investment cost, and lack of supporting policies for bioenergy production (Hoang et al. Citation2022a). Other studies also pointed out some technological challenges over the implementation of AD technology, such as scalability, high energy and water requirement, inadequate control and monitoring of the AD process, performance instability, and contamination (Lin et al. Citation2018; Norrahim et al. Citation2022; Suhartini et al. Citation2022b). Other challenges in implementing of AD are post-treatment of digestate, relatively slow digestion, large investment, and lack of legislation for digestate utilisation (Lin et al. Citation2018). Moreover, other issues that may arise from the AD of palm oil biomass include potential odour, water or soil pollution, and GHG emission problems from digestate if not properly handled (Norrahim et al. Citation2022). Specific to OPEFBs, our previous study also found that the AD of treated OPEFBs (i.e. fungally, microbially, or chemically pre-treated) (Hidayat et al. Citation2020; Nurika et al. Citation2022; Rohma, Suhartini, and Nurika Citation2021) has higher biogas and methane potential compared with AD of untreated OPEFBs (Suhartini, Hidayat, and Nurika Citation2020; Citation2021). The findings suggested that pre-treatment is essential to enhance bioenergy production but may increase the operational cost, technology infrastructure, and energy requirement (Norrahim et al. Citation2022; Rizal et al. Citation2018). A further in-depth evaluation is increasingly critical to improve the conversion efficacy and commercialisation of OPEFBs via AD technology.
The future direction of valorising OPEFBs to biogas should focus on the integrated biorefinery and bioeconomy concept to achieve maximum valorisation with minimal or zero waste production. Fermoso et al. (Citation2018) suggested that AD-based biorefinery, whether partially or integrated, offers great potential for effectively converting various low-value biomass or waste substrates into valuable products. Several strategies are proposed in various studies, for instance, the implementation of advanced control and monitoring system, the recovery of other valuable products (i.e. digestate pellet or biochars, organic acids) from AD process (Anukam et al. Citation2019); and the adoption of low-cost anaerobic digester technology (Mendieta et al. Citation2021). Furthermore, it is recommended to seek a more cost-effective AD process and technology through technology modification, operating parameters optimisation, and effective microorganism selection for pre-treatment and AD process (Anukam et al. Citation2019; Shrestha et al. Citation2017). Shrestha et al. (Citation2017) added that, with respect to biological pre-treatment prior AD process, providing a similar natural ecosystem of the microorganism used during the pre-treatment is suggested for better lignocellulose degradation and higher biogas or methane yield. Future research should also focus on implementing multi-product biorefinery via AD as a central process. This approach offers many benefits, including the generation of eco-friendly energy, achievement of energy efficiency, and comprehensive waste management via production of bioenergy and bio-based products (Rekleitis et al. Citation2020); creation of the green downstream industry, improvement of local economies, creation of employment opportunities, and creation of new lucrative revenue (Abdullah and Hussein Citation2021; Ali et al. Citation2015). Hence, integrated biorefinery with zero-waste and circular economy consideration create a win-win solution to meet the three pillars of sustainable development goals (i.e. economic/profit, environment/planet, and social/people). Moreover, a recent study by Goswami et al. (Citation2022) reported that applying big data analytics (BDA) with combination of IoT and ML promotes the creation of smart and intelligent bio-refineries. Such approaches offer solution to remotely supervise the operation, improve the operation's efficiency, and enhance savings in energy or operational cost. Yet, further research on comprehensive analysis, evaluation, and potential commercialisation of adopting smart and intelligent bio-refineries is critically required.
6. Conclusion
This review paper shows that OPEFBs can be a potential feedstock for the AD system in Indonesia, considering additional pre-treatment for improving delignification and enhancing biodegradation efficiency. In contrast to other pre-treatment, biological pre-treatment offers a green option for handling OPEFBs because it is low cost, less energy consumption, no release of toxic compounds, less pollutants generation, and environmentally safe. Co-digestion strategies may improve biomass digestibility, hence biogas/methane yield Among the discussed biological pre-treatment, the mushroom cultivation pre-treatment provides additional economic benefits from producing and selling of edible mushrooms. Based on the reviewed pre-treatment options, combining biological pre-treatment with other influential, low cost and eco-friendly pre-treatments may result in superior performance on delignification and promote higher biogas or methane production. Combining AD technology, either in mono- or co-digestion system, with multi-stage pre-treatment (i.e. physical followed by mushroom cultivation pre-treatment) could maximise the value of OPEFBs with multi-product generation (i.e. biogas, edible mushroom, and biofertiliser). Therefore, in the Indonesia context, this scenario is proposed for integrating multi-product biorefinery and circular economy with a zero-waste concept. Integrating multi-product biorefinery through AD of OPEFBs may offer feasible and sustainable valorisation routes with lower detrimental environmental impact. Further studies on optimising pre-treatment and AD process efficacy, as well as on the potential of full-scale to commercialisation are highly advised. Further work is also recommended to address key issues around technical, environmental, economic, legislation, and sustainable supply chains.
Acknowledgements
Authors would like to thanks the Ministry of Research, Technology, and Higher Education for the research funding provided through Penelitian Dasar Multi Tahun (Multi-Year Basic Research) Scheme (Grant Number: 108/E4.1/AK.04.PT/2021 and Contract Number: 1271.34/UN10.C10/TU/2021). The authors would also like to thank the Faculty of Agricultural Technology, Universitas Brawijaya, for supporting the manuscript's writing and publication.
Disclosure statement
No potential conflict of interest was reported by the author(s).
Additional information
Funding
References
- Ab Rasid, N. S., A. Shamjuddin, A. Z. Abdul Rahman, and N. A. S. Amin. 2021. “Recent Advances in Green Pre-Treatment Methods of Lignocellulosic Biomass for Enhanced Biofuel Production.” Journal of Cleaner Production 321: 1–23. doi:10.1016/j.jclepro.2021.129038.
- Abdullah, M. A., and H. A. Hussein. 2021. “Integrated Algal and Oil Palm Biorefinery as a Model System for Bioenergy Co-Generation with Bioproducts and Biopharmaceuticals.” Bioresources and Bioprocessing 8 (40): 1–29. doi:10.1186/s40643-021-00396-0.
- Abdullah, N., and F. Sulaiman. 2013. “Chapter 3 - The Oil Palm Wastes in Malaysia.” In Biomass Now-Sustainable Growth and Use, edited by M. D. Matovic, Vol. 1, 75–93. Rijeka: IntechOpen.
- Abdullah, B., S. A. F. A. Syed Muhammad, Z. Shokravi, S. Ismail, K. A. Kassim, A. N. Mahmood, and M. M. A. Aziz. 2019. “Fourth Generation Biofuel: A Review on Risks and Mitigation Strategies.” Renewable and Sustainable Energy Reviews 107: 37–50. doi:10.1016/j.rser.2019.02.018.
- Abraham, A., A. K. Mathew, H. Park, O. Choi, R. Sindhu, B. Parameswaran, A. Pandey, J. H. Park, and B.-I. Sang. 2020. “Pretreatment Strategies for Enhanced Biogas Production from Lignocellulosic Biomass.” Bioresource Technology 301: 122725. doi:10.1016/j.biortech.2019.122725.
- Abu-Dahrieh, J., A. Orozco, E. Groom, and D. Rooney. 2011. “Batch and Continuous Biogas Production from Grass Silage Liquor.” Bioresource Technology 102 (23): 10922–10928. doi:10.1016/j.biortech.2011.09.072.
- Adinurani, P. G., T. Liwang, Salafudin, L. O. Nelwan, Y. Sakri, S. K. Wahono, and R. Hendroko. 2013. “The Study of Two Stages Anaerobic Digestion Application and Suitable Bio-Film as an Effort to Improve bio-gas Productivity from Jatropha curcas Linn Capsule Husk.” Energy Procedia 32: 84–89. doi:10.1016/j.egypro.2013.05.011.
- Agabo-García, C., M. Pérez, B. Rodríguez-Morgado, J. Parrado, and R. Solera. 2019. “Biomethane Production Improvement by Enzymatic pre-Treatments and Enhancers of Sewage Sludge Anaerobic Digestion.” Fuel 255: 1–6. doi:10.1016/j.fuel.2019.115713.
- Agbor, V. B., N. Cicek, R. Sparling, A. Berlin, and D. B. Levin. 2011. “Biomass Pretreatment: Fundamentals Toward Application.” Biotechnology Advances 29 (6): 675–685. doi:10.1016/j.biotechadv.2011.05.005.
- Aguilar-Rivera, N., R. C. Llarena-Hernández, C. Michel-Cuello, M. R. Gámez-Pastrana, and T. de Jesús Debernardi-Vazquez. 2017. “Chapter 1. Competitive Edible Mushroom Production from Nonconventional Waste Biomass.” In Future Foods, edited by H. Mikkola, 1–26. Croatia: IntechOpen.
- Ahmad Rizal, N. F., M. F. Ibrahim, M. R. Zakaria, E. Kamal Bahrin, S. Abd-Aziz, and M. A. Hassan. 2018. “Combination of Superheated Steam with Laccase Pretreatment Together with Size Reduction to Enhance Enzymatic Hydrolysis of Oil Palm Biomass.” Molecules 23 (4): 1–14. doi:10.3390/molecules23040811.
- Ahmad, A., S. M. U. Shah, M. F. Othman, and M. A. Abdullah. 2014. “Enhanced Palm oil Mill Effluent Treatment and Biomethane Production by Co-Digestion of Oil Palm Empty Fruit Bunches with Chlorella Sp.” The Canadian Journal of Chemical Engineering 92 (9): 1636–1642. doi:10.1002/cjce.22029.
- Ahmad, F. B., Z. Zhang, W. O. S. Doherty, and I. M. O’Hara. 2019. “The Outlook of the Production of Advanced Fuels and Chemicals from Integrated Oil Palm Biomass Biorefinery.” Renewable and Sustainable Energy Reviews 109: 386–411. doi:10.1016/j.rser.2019.04.009.
- Ahmed, S., and M. Kazda. 2017. “Characteristics of On-demand Biogas Production by Using Sugar Beet Silage.” Anaerobe 46: 114–121. doi:10.1016/j.anaerobe.2017.04.016.
- Ahuja, V., M. Macho, D. Ewe, M. Singh, S. Saha, and K. Saurav. 2020. “Biological and Pharmacological Potential of Xylitol: A Molecular Insight of Unique Metabolism.” Foods (basel, Switzerland) 9 (11): 1–24. doi:10.3390/foods9111592.
- Albertó, E. 2017. “Chapter 19 - Naturally Occurring Strains of Edible Mushrooms: A Source to Improve the Mushroom Industry.” In Edible and Medicinal Mushrooms: Technology and Applications, edited by C. Z. Diego and A. Pardo-Giménez, 415–425. West Sussex: John Wiley & Sons Ltd.
- Ali, S. S., A. E.-F. Abomohra, and J. Sun. 2017. “Effective Bio-pretreatment of Sawdust Waste with a Novel Microbial Consortium for Enhanced Biomethanation.” Bioresource Technology 238: 425–432. doi:10.1016/j.biortech.2017.03.187.
- Ali, S. S., A. M. Mustafa, M. Kornaros, A. Manni, J. Sun, and M. A. Khalil. 2020. “Construction of Novel Microbial Consortia CS-5 and BC-4 Valued for the Degradation of Catalpa Sawdust and Chlorophenols Simultaneously with Enhancing Methane Production.” Bioresource Technology 301: 1–15. doi:10.1016/j.biortech.2019.122720.
- Ali, A. A. M., M. R. Othman, Y. Shirai, and M. A. Hassan. 2015. “Sustainable and Integrated Palm oil Biorefinery Concept with Value-Addition of Biomass and Zero Emission System.” Journal of Cleaner Production 91: 96–99. doi:10.1016/j.jclepro.2014.12.030.
- Amin, N., N. Ya’aini, M. Misson, R. Haron, and M. Mohamed. 2010. “Enzymed Pretreated Empty Palm Fruit Bunch for Biofuel Production.” Journal of Applied Sciences 10 (12): 1181–1186. doi:10.3923/jas.2010.1181.1186.
- Amir, E., S. Hophmayer-Tokich, and T. B. Kurnani. 2016. “Socio-economic Considerations of Converting Food Waste Into Biogas on a Household Level in Indonesia: The Case of the City of Bandung.” Recycling 1 (1): 61–88. doi:10.3390/recycling1010061.
- Anukam, A., A. Mohammadi, M. Naqvi, and K. Granström. 2019. “A Review of the Chemistry of Anaerobic Digestion: Methods of Accelerating and Optimizing Process Efficiency.” Processes 7 (8): 1–19. doi:10.3390/w13182566.
- Appels, L., J. Lauwers, J. Degrève, L. Helsen, B. Lievens, K. Willems, J. Van Impe, and R. Dewil. 2011. “Anaerobic Digestion in Global bio-Energy Production: Potential and Research Challenges.” Renewable and Sustainable Energy Reviews 15 (9): 4295–4301. doi:10.1016/j.rser.2011.07.121.
- Arbaain, E. N. N., E. K. Bahrin, M. F. Ibrahim, Y. Ando, and S. Abd-Aziz. 2019. “Biological Pretreatment of Oil Palm Empty Fruit Bunch by Schizophyllum commune ENN1 Without Washing and Nutrient Addition.” Processes 7 (402): 1–8. doi:10.3390/pr7070402.
- Ardhiansyah, H., and B. Budiyono. 2018. “Enhanced Biogas Production from Sawdust with Various Pretreatments During Solid-State Anaerobic Digestion: An Overview.” Advanced Science Letters 24 (12): 9645–9647. doi:10.1166/asl.2018.13101.
- Ariffin, H., M. Hassan, M. Umi Kalsom, N. Abdullah, and Y. Shirai. 2008. “Effect of Physical, Chemical and Thermal Pretreatments on the Enzymatic Hydrolysis of Oil Palm Empty Fruit Bunch (OPEFB).” Journal of Tropical Agriculture and Food Science 36 (2): 259–268.
- Aro, E.-M. 2016. “From First Generation Biofuels to Advanced Solar Biofuels.” Ambio 45 (1): 24–31. doi:10.1007/s13280-015-0730-0.
- Asiegbu, F. O., A. Paterson, and J. E. Smith. 1996. “The Effects of Co-fungal Cultures and Supplementation with Carbohydrate Adjuncts on Lignin Biodegradation and Substrate Digestibility.” World Journal of Microbiology and Biotechnology 12 (3): 273–279. doi:10.1007/BF00360927.
- Aslanzadeh, S., K. Rajendran, and M. J. Taherzadeh. 2014. “A Comparative Study Between Single- and Two-Stage Anaerobic Digestion Processes: Effects of Organic Loading Rate and Hydraulic Retention Time.” International Biodeterioration & Biodegradation 95: 181–188. doi:10.1016/j.ibiod.2014.06.008.
- Atabani, A. E., E. Mahmoud, M. Aslam, S. R. Naqvi, D. Juchelková, S. K. Bhatia, I. A. Badruddin, T. M. Y. Khan, A. T. Hoang, and P. Palacky. 2022a. “Emerging Potential of Spent Coffee Ground Valorization for Fuel Pellet Production in a Biorefinery.” Environment, Development and Sustainability 2022: 1–39. doi:10.1007/s10668-022-02361-z.
- Atabani, A. E., V. K. Tyagi, G. Fongaro, H. Treichel, A. Pugazhendhi, and A. T. Hoang. 2022b. “Integrated Biorefineries, Circular Bio-Economy, and Valorization of Organic Waste Streams with Respect to Bio-Products.” Biomass Conversion and Biorefinery 12 (3): 565–565. doi:10.1007/s13399-021-02017-4.
- Ayompe, L. M., M. Schaafsma, and B. N. Egoh. 2021. “Towards Sustainable Palm oil Production: The Positive and Negative Impacts on Ecosystem Services and Human Wellbeing.” Journal of Cleaner Production 278: 1–11. doi:10.1016/j.jclepro.2020.123914.
- Aziz, M. M. A., K. A. Kassim, M. ElSergany, S. Anuar, M. E. Jorat, H. Yaacob, A. Ahsan, M. A. Imteaz, and Arifuzzaman. 2020. “Recent Advances on Palm Oil Mill Effluent (POME) Pretreatment and Anaerobic Reactor for Sustainable Biogas Production.” Renewable and Sustainable Energy Reviews 119: 1–14. doi:10.1016/j.rser.2019.109603.
- Baghbanzadeh, M., J. Savage, H. Balde, M. Sartaj, A. C. VanderZaag, N. Abdehagh, and B. Strehler. 2021. “Enhancing Hydrolysis and Bio-Methane Generation of Extruded Lignocellulosic Wood Waste Using Microbial Pre-Treatment.” Renewable Energy 170: 438–448. doi:10.1016/j.renene.2021.01.131.
- Bajpai, P. 2017. Anaerobic Technology in Pulp and Paper Industry. Singapore: Springer.
- Bakır, H., Ü Ağbulut, A. E. Gürel, G. Yıldız, U. Güvenç, M. E. M. Soudagar, A. T. Hoang, B. Deepanraj, G. Saini, and A. Afzal. 2022. “Forecasting of Future Greenhouse gas Emission Trajectory for India Using Energy and Economic Indexes with Various Metaheuristic Algorithms.” Journal of Cleaner Production 360 (131946): 1–24. doi:10.1016/j.jclepro.2022.131946.
- Banu, J. R., S. Sugitha, S. Kavitha, R. Y. Kannah, J. Merrylin, and G. Kumar. 2021. “Lignocellulosic Biomass Pretreatment for Enhanced Bioenergy Recovery: Effect of Lignocelluloses Recalcitrance and Enhancement Strategies.” Frontiers in Energy Research (679): 1–17. doi:10.3389/fenrg.2021.646057.
- Bateni, H., K. Karimi, A. Zamani, and F. Benakashani. 2014. “Castor Plant for Biodiesel, Biogas, and Ethanol Production with a Biorefinery Processing Perspective.” Applied Energy 136: 14–22. doi:10.1016/j.apenergy.2014.09.005.
- Bhutto, A. W., K. Qureshi, K. Harijan, R. Abro, T. Abbas, A. A. Bazmi, S. Karim, and G. Yu. 2017. “Insight Into Progress in Pre-treatment of Lignocellulosic Biomass.” Energy 122: 724–745. doi:10.1016/j.energy.2017.01.005.
- Boons, F., and A. Mendoza. 2010. “Constructing Sustainable Palm Oil: How Actors Define Sustainability.” Journal of Cleaner Production 18 (16): 1686–1695. doi:10.1016/j.jclepro.2010.07.003.
- Borreani, G., E. Tabacco, R. J. Schmidt, B. J. Holmes, and R. E. Muck. 2018. “Silage Review: Factors Affecting Dry Matter and Quality Losses in Silages.” Journal of Dairy Science 101 (5): 3952–3979. doi:10.3168/jds.2017-13837.
- BPS-Statistics Indonesia. 2018. Indonesian Oil Palm Statistics 2018. Jakarta: BPS-Statistics Indonesia.
- Brémond, U., R. de Buyer, J.-P. Steyer, N. Bernet, and H. Carrere. 2018. “Biological Pretreatments of Biomass for Improving Biogas Production: An Overview from Lab Scale to Full-Scale.” Renewable and Sustainable Energy Reviews 90: 583–604. doi:10.1016/j.rser.2018.03.103.
- Burhani, D., and E. Triwahyuni. 2019. An Integrated Biorefinery Process of oil Palm Empty Fruit Bunch for Bioethanol and Flame Retardant. 020034–020034. New York: AIP Publishing.
- Cabrales, H., N. Arzola, and O. Araque. 2020. “The Effects of Moisture Content, Fiber Length and Compaction Time on African Oil Palm Empty Fruit Bunches Briquette Quality Parameters.” Heliyon 6 (12): 1–11. doi:10.1016/j.heliyon.2020.e05607.
- Cai, Y., Z. Zheng, F. Schäfer, W. Stinner, X. Yuan, H. Wang, Z. Cui, and X. Wang. 2021. “A Review About Pretreatment of Lignocellulosic Biomass in Anaerobic Digestion: Achievement and Challenge in Germany and China.” Journal of Cleaner Production 299: 1–14. doi:10.1016/j.jclepro.2021.126885.
- Carrere, H., G. Antonopoulou, R. Affes, F. Passos, A. Battimelli, G. Lyberatos, and I. Ferrer. 2016. “Review of Feedstock Pretreatment Strategies for Improved Anaerobic Digestion: From Lab-Scale Research to Full-Scale Application.” Bioresource Technology 199: 386–397. doi:10.1016/j.biortech.2015.09.007.
- Castano, J. D., C. C. Crespo, and E. Torres. 2019. “Evaluation of Chemical and Biological Treatments to Degrade oil Palm Empty Fruit Bunches (Elaeis guineensis Jacq.) and Their Potential Use.” Journal of Oil Palm Research 31 (2): 271–280. doi:10.21894/jopr.2019.0016.
- Chaikitkaew, S., P. Kongjan, and S. O-Thong. 2015. “Biogas Production from Biomass Residues of Palm Oil Mill by Solid State Anaerobic Digestion.” Energy Procedia 79: 838–844. doi:10.1016/j.egypro.2015.11.575.
- Chen, W.-H., R. Aniza, A. A. Arpia, H.-J. Lo, A. T. Hoang, V. Goodarzi, and J. Gao. 2022b. “A Comparative Analysis of Biomass Torrefaction Severity Index Prediction from Machine Learning.” Applied Energy 324 (119689): 1–12. doi:10.1016/j.apenergy.2022.119689.
- Chen, Y., J. J. Cheng, and K. S. Creamer. 2008. “Inhibition of Anaerobic Digestion Process: A Review.” Bioresource Technology 99 (10): 4044–4064. doi:10.1016/j.biortech.2007.01.057.
- Chen, Y., J. He, Y.-Q. Wang, T. A. Kotsopoulos, P. Kaparaju, and R. J. Zeng. 2016. “Development of an Anaerobic Co-Metabolic Model for Degradation of Phenol, m-Cresol and Easily Degradable Substrate.” Biochemical Engineering Journal 106: 19–25. doi:10.1016/j.bej.2015.11.003.
- Chen, F., C. Martín, T. A. Lestander, A. Grimm, and S. Xiong. 2022a. “Shiitake Cultivation as Biological Preprocessing of Lignocellulosic Feedstocks – Substrate Changes in Crystallinity, Syringyl/Guaiacyl Lignin and Degradation-Derived by-Products.” Bioresource Technology 344: 1–10. doi:10.1016/j.biortech.2021.126256.
- Chen, J. L., R. Ortiz, T. W. Steele, and D. C. Stuckey. 2014a. “Toxicants Inhibiting Anaerobic Digestion: A Review.” Biotechnology Advances 32 (8): 1523–1534. doi:10.1016/j.biotechadv.2014.10.005.
- Chen, Y., B. Rößler, S. Zielonka, A. Lemmer, A.-M. Wonneberger, and T. Jungbluth. 2014b. “The Pressure Effects on Two-Phase Anaerobic Digestion.” Applied Energy 116: 409–415. doi:10.1016/j.apenergy.2013.11.012.
- Chiesa, S., and E. Gnansounou. 2014. “Use of Empty Fruit Bunches from the Oil Palm for Bioethanol Production: A Thorough Comparison Between Dilute Acid and Dilute Alkali Pretreatment.” Bioresource Technology 159: 355–364. doi:10.1016/j.biortech.2014.02.122.
- Chin, D. W. K., S. Lim, Y. L. Pang, L. K. Leong, and C. H. Lim. 2019. “Investigation of Organosolv Pretreatment to Natural Microbial-Degraded Empty Fruit Bunch for Sugar Based Substrate Recovery.” Energy Procedia 158: 1065–1071. doi:10.1016/j.egypro.2019.01.258.
- Chin, K. L., M. Y. Nurliyana, P. San H’ng, C. L. Lee, W. Z. Go, P. San Khoo, R. A. R. Nazrin, and S. N. Ashikin. 2020. “Effects of Bacterial Bio-Augmentation on the Methane Potential from Facultative Digestion of Palm oil Mill Effluent and Empty Fruit Bunch.” Waste and Biomass Valorization 11: 3407–3418. doi:10.1007/s12649-019-00680-3.
- Chiumenti, A., F. da Borso, and S. Limina. 2018. “Dry Anaerobic Digestion of cow Manure and Agricultural Products in a Full-Scale Plant: Efficiency and Comparison with wet Fermentation.” Waste Management 71: 704–710. doi:10.1016/j.wasman.2017.03.046.
- Chynoweth, D. P., J. M. Owens, and R. Legrand. 2001. “Renewable Methane from Anaerobic Digestion of Biomass.” Renewable Energy 22 (1): 1–8. doi:10.1016/S0960-1481(00)00019-7.
- Coblentz, W. K., and M. S. Akins. 2018. “Silage Review: Recent Advances and Future Technologies for Baled Silages.” Journal of Dairy Science 101 (5): 4075–4092. doi:10.3168/jds.2017-13708.
- Darwin, D. D. 2019. “Removal of Organic Pollutants from Tofu-Processing Wastewater Through Anaerobic Treatment Process with Short Hydraulic Retention Time.” Environmental Research, Engineering and Management 75 (1): 34–42. doi:10.5755/j01.erem.75.1.21532.
- de Gonzalo, G., D. I. Colpa, M. H. M. Habib, and M. W. Fraaije. 2016. “Bacterial Enzymes Involved in Lignin Degradation.” Journal of Biotechnology 236: 110–119. doi:10.1016/j.jbiotec.2016.08.011.
- De La Rubia, M., J. Villamil, and A. Mohedano. 2019. “Anaerobic Digestion for Methane and Hydrogen Production.” In Wastewater Treatment Residues as Resources for Biorefinery Products and Biofuels, edited by J. A. Olivares, D. Puyol, J. A. Melero, and J. Dufour, 67–83. Amsterdam: Elsevier.
- Deb, N., M. Z. Alam, M. F. R. Al-khatib, and A. Elgharbawy. 2019. “Development of Acid-Base-Enzyme Pretreatment and Hydrolysis of Palm Oil Mill Effluent for Bioethanol Production.” In Liquid Biofuel Production, edited by L. K. Singh and G. Chaudhary, 197–221. New Jersey: Scrivener Publishing LLC.
- Deublein, D., and A. Stenhauser. 2011. Biogas from Waste and Renewable Resources: An Introduction. Weinheim: Wiley-VCH.
- Dębowski, M., M. Zieliński, A. Grala, and M. Dudek. 2013. “Algae Biomass as an Alternative Substrate in Biogas Production Technologies—Review.” Renewable and Sustainable Energy Reviews 27: 596–604. doi:10.1016/j.rser.2013.07.029.
- Dhouib, A., F. Aloui, N. Hamad, and S. Sayadi. 2006. “Pilot-plant Treatment of Olive Mill Wastewaters by Phanerochaete chrysosporium Coupled to Anaerobic Digestion and Ultrafiltration.” Process Biochemistry 41 (1): 159–167. doi:10.1016/j.procbio.2005.06.008.
- Dimawarnita, F., U. Perwitasari, S. Marsudi, Y. Faramitha, and S. Suharyanto. 2022. “The Utilization of oil Palm Empty Fruit Bunches for Growth of Oyster Mushroom (Pleurotus ostreatus) and Biodelignification Process During Planting Cycle.” Agrivita 44 (1): 165. doi:10.17503/agrivita.v44i1.2311.
- Díaz, I., F. Fdz-Polanco, B. Mutsvene, and M. Fdz-Polanco. 2020. “Effect of Operating Pressure on Direct Biomethane Production from Carbon Dioxide and Exogenous Hydrogen in the Anaerobic Digestion of Sewage Sludge.” Applied Energy 280: 1–9. doi:10.1016/j.apenergy.2020.115915.
- dos Santos, L. A., T. H. L. Silva, C. R. D. M. Oliveira, J. F. T. Jucá, and A. F. D. M. S. Santos. 2022. “Silage as a pre-Treatment of Orange Bagasse Waste to Increase the Potential for Methane Generation.” Science of The Total Environment 823: 1–10. doi:10.1016/j.scitotenv.2022.153613.
- Dutta, K., A. Daverey, and J.-G. Lin. 2014. “Evolution Retrospective for Alternative Fuels: First to Fourth Generation.” Renewable Energy 69: 114–122. doi:10.1016/j.renene.2014.02.044.
- El-Mashad, H. M. 2015. “Biomethane and Ethanol Production Potential of Spirulina platensis Algae and Enzymatically Saccharified Switchgrass.” Biochemical Engineering Journal 93: 119–127. doi:10.1016/j.bej.2014.09.009.
- Evranos, B., and B. Demirel. 2015. “The Impact of Ni, Co and Mo Supplementation on Methane Yield from Anaerobic Mono-Digestion of Maize Silage.” Environmental Technology 36 (12): 1556–1562. doi:10.1080/09593330.2014.997297.
- Fagbohungbe, M. O., I. C. Dodd, B. M. J. Herbert, H. Li, L. Ricketts, and K. T. Semple. 2015. “High Solid Anaerobic Digestion: Operational Challenges and Possibilities.” Environmental Technology & Innovation 4: 268–284. doi:10.1016/j.eti.2015.09.003.
- Falade, A. O., U. U. Nwodo, B. C. Iweriebor, E. Green, L. V. Mabinya, and A. I. Okoh. 2017. “Lignin Peroxidase Functionalities and Prospective Applications.” MicrobiologyOpen 6 (1): 1–14. doi:10.1002/mbo3.394.
- Fan, L., A. Pandey, R. Mohan, and C. Soccol. 2000. “Use of Various Coffee Industry Residues for the Cultivation of Pleurotus ostreatus in Solid State Fermentation.” Acta Biotechnologica 20 (1): 41–52. doi:10.1002/abio.370200108.
- Feng, L., E. F. Kristensen, V. Moset, A. J. Ward, and H. B. Møller. 2018. “Ensiling of Tall Fescue for Biogas Production: Effect of Storage Time, Additives and Mechanical Pretreatment.” Energy for Sustainable Development 47: 143–148.
- Fermoso, F. G., A. Serrano, B. Alonso-Fariñas, J. Fernández-Bolaños, R. Borja, and G. Rodríguez-Gutiérrez. 2018. “Valuable Compound Extraction, Anaerobic Digestion, and Composting: A Leading Biorefinery Approach for Agricultural Wastes.” Journal of Agricultural and Food Chemistry 66 (32): 8451–8468. doi:10.1021/acs.jafc.8b02667.
- Ferraro, A., G. Dottorini, G. Massini, V. Mazzurco Miritana, A. Signorini, G. Lembo, and M. Fabbricino. 2018. “Combined Bioaugmentation with Anaerobic Ruminal Fungi and Fermentative Bacteria to Enhance Biogas Production from Wheat Straw and Mushroom Spent Straw.” Bioresource Technology 260: 364–373. doi:10.1016/j.biortech.2018.03.128.
- Foutch, G. L., and A. H. Johannes. 2003. “Reactors in Process Engineering.” In Encyclopedia of Physical Science and Technology, edited by R. A. Meyers, 3rd ed., Vol. 1, 23–43. San Diego: Academic Press.
- Gatti, R. C., J. Liang, A. Velichevskaya, and M. Zhou. 2019. “Sustainable Palm oil may not be so Sustainable.” Science of the Total Environment 652: 48–51.
- Gerardi, M. H. 2003. The Microbiology of Anaerobic Digesters. New Jersey: John Wiley & Sons.
- Ghaffar, S. H., M. Fan, and B. McVicar. 2015. “Bioengineering for Utilisation and Bioconversion of Straw Biomass Into bio-Products.” Industrial Crops and Products 77: 262–274. doi:10.1016/j.indcrop.2015.08.060.
- Ghosh, P., G. Shah, S. Sahota, L. Singh, and V. K. Vijay. 2020. “Chapter 7 - Biogas Production from Waste: Technical Overview, Progress, and Challenges.” In Bioreactors: Sustainable Design and Industrial Applications in Mitigation of GHG Emissions, edited by L. Singh, A. Yousuf, and D. M. Mahapatra, 89–104. Oxford: Elsevier.
- Goswami, L., R. Kayalvizhi, P. K. Dikshit, K. C. Sherpa, S. Roy, A. Kushwaha, B. S. Kim, R. Banerjee, S. Jacob, and R. C. Rajak. 2022. “A Critical Review on Prospects of Bio-Refinery Products from Second and Third Generation Biomasses.” Chemical Engineering Journal 448 (137677): 1–19. doi:10.1016/j.cej.2022.137677.
- Gould, M. C. 2015. “Chapter 18 - Bioenergy and Anaerobic Digestion.” In Bioenergy: Biomass to Biofuels, edited by A. Dahiya, 297–317. Boston: Academic Press.
- Gómez, D., J. L. Ramos-Suárez, B. Fernández, E. Muñoz, L. Tey, M. Romero-Güiza, and F. Hansen. 2019. “Development of a Modified Plug-Flow Anaerobic Digester for Biogas Production from Animal Manures.” Energies 12 (13): 1–17. doi:10.3390/en12132628.
- Grangeiro, L. C., S. G. C. de Almeida, B. S. D. Mello, L. T. Fuess, A. Sarti, and K. J. Dussán. 2019. “Chapter 7 - New Trends in Biogas Production and Utilization.” In Sustainable Bioenergy: Advances and Impacts, edited by M. Rai and A. P. Ingle, 199–223. Oxford: Elsevier.
- Grimm, D., and H. A. B. Wösten. 2018. “Mushroom Cultivation in the Circular Economy.” Applied Microbiology and Biotechnology 102 (18): 7795–7803. doi:10.1007/s00253-018-9226-8.
- Gu, J.-D. 2016. “Biodegradation Testing: So Many Tests but Very Little new Innovation.” Applied Environmental Biotechnology (Transferred) 1 (1): 92–95. doi:10.26789/AEB.2016.01.007.
- Gu, J.-D. 2021. “On Environmental Biotechnology of Bioremediation.” Applied Environmental Biotechnology 5 (2): 28–33. doi:10.26789/AEB.2020.02.002.
- Hafyan, R. H., L. K. Bhullar, S. Mahadzir, M. R. Bilad, N. A. H. Nordin, M. D. H. Wirzal, Z. A. Putra, G. P. Rangaiah, and B. Abdullah. 2020. “Integrated Biorefinery of Empty Fruit Bunch from Palm oil Industries to Produce Valuable Biochemicals.” Processes 8 (7): 1–24. doi:10.3390/pr8070868.
- Halim, L., M. Mellyanawaty, R. B. Cahyono, H. Sudibyo, and W. Budhijanto. 2017. Anaerobic Digestion of Palm oil Mill Effluent with Lampung Natural Zeolite as Microbe Immobilization Medium and Digested cow Manure as Starter, 10003. New York: AIP Publishing.
- Hambali, E., and M. Rivai. 2017. The Potential of Palm oil Waste Biomass in Indonesia in 2020 and 2030, 012050–012050. Bristol: IOP Publishing.
- Hamzah, F., A. Idris, and T. K. Shuan. 2011. “Preliminary Study on Enzymatic Hydrolysis of Treated Oil Palm (Elaeis) Empty Fruit Bunches Fibre (EFB) by Using Combination of Cellulase and β 1-4 Glucosidase.” Biomass and Bioenergy 35: 1055–1059. doi:10.1016/j.biombioe.2010.11.020.
- Harahap, F., S. Leduc, S. Mesfun, D. Khatiwada, F. Kraxner, and S. Silveira. 2020b. “Meeting the Bioenergy Targets from Palm oil Based Biorefineries: An Optimal Configuration in Indonesia.” Applied Energy 278: 1–15. doi:10.1016/j.apenergy.2020.115749.
- Harahap, B. M., E. Mardawati, and D. Nurliasari. 2020a. “A Comprehensive Review: Integrated Microbial Xylitol, Bioethanol, and Cellulase Production from oil Palm Empty Fruit Bunches.” Jurnal Industri Pertanian 2 (1): 142–157.
- Harahap, F., S. Silveira, and D. Khatiwada. 2019. “Cost Competitiveness of Palm oil Biodiesel Production in Indonesia.” Energy 170: 62–72.
- Harmini, S., M. D. Hutomo, L. Bahruddin, R. Millati, M. N. Cahyanto, C. Niklasson, and M. J. Taherzadeh. 2013. “Fungal Pretreatment of oil Palm Empty Fruit Bunch: Effect of Manganese and Nitrogen.” Cellulose Chemistry and Technology. 47 (9-10): 751–757.
- Harsono, S. S., P. Grundmann, and S. Soebronto. 2014. “Anaerobic Treatment of Palm oil Mill Effluents: Potential Contribution to net Energy Yield and Reduction of Greenhouse gas Emissions from Biodiesel Production.” Journal of Cleaner Production 64: 619–627. doi:10.1016/j.jclepro.2013.07.056.
- Hendriks, A. T. W. M., and G. Zeeman. 2009. “Pretreatments to Enhance the Digestibility of Lignocellulosic Biomass.” Bioresource Technology 100 (1): 10–18. doi:10.1016/j.biortech.2008.05.027.
- Herrmann, C., M. Heiermann, and C. Idler. 2011. “Effects of Ensiling, Silage Additives and Storage Period on Methane Formation of Biogas Crops.” Bioresource Technology 102 (8): 5153–5161. doi:10.1016/j.biortech.2011.01.012.
- Heryadi, R., A. Uyun, E. Yandri, S. Nur, K. Abdullah, and O. Anne. 2019. Biomass to Methanol Plant Based on Gasification of Palm Empty Fruit Bunch, 012036–012036. Bristol: IOP Publishing.
- Hidayat, N., S. Suhartini, R. N. Utami, and M. B. Pangestuti. 2020. “Anaerobic Digestion of Fungally Pre-Treated oil Palm Empty Fruit Bunches: Energy and Carbon Emission Footprint.” IOP Conference Series: Earth and Environmental Science 524 (012019): 1–9. doi:10.1088/1755-1315/524/1/012019.
- Hidayatullah, I. M., M. D. Al Husna, H. Radiyan, M. T. A. P. Kresnowati, S. H. Suhardi, T. Setiadi, and R. Boopathy. 2021. “Combining Biodelignification and Hydrothermal Pretreatment of oil Palm Empty Fruit Bunches (OPEFB) for Monomeric Sugar Production.” Bioresource Technology Reports 15: 1–6. doi:10.1016/j.biteb.2021.100808.
- Hirschmann, R. 2020. Palm Oil Industry in Indonesia- Statistics & Facts.
- Hoang, A. T., Z. Huang, S. Nižetić, A. Pandey, X. P. Nguyen, R. Luque, H. C. Ong, Z. Said, T. H. Le, and V. V. Pham. 2022a. “Characteristics of Hydrogen Production from Steam Gasification of Plant-Originated Lignocellulosic Biomass and its Prospects in Vietnam.” International Journal of Hydrogen Energy 47 (7): 4394–4425. doi:10.1016/j.ijhydene.2021.11.091.
- Hoang, A. T., A. Pandey, Z. Huang, R. Luque, K. H. Ng, A. M. Papadopoulos, W.-H. Chen, et al. 2022b. “Catalyst-Based Synthesis of 2,5-Dimethylfuran from Carbohydrates as a Sustainable Biofuel Production Route.” ACS Sustainable Chemistry & Engineering 10 (10): 3079–3115. doi:10.1021/acssuschemeng.1c06363.
- Hoang, A. T., P. S. Varbanov, S. Nižetić, R. Sirohi, A. Pandey, R. Luque, K. H. Ng, and V. V. Pham. 2022c. “Perspective Review on Municipal Solid Waste-to-Energy Route: Characteristics, Management Strategy, and Role in Circular Economy.” Journal of Cleaner Production 359 (131897): 1–29. doi:10.1016/j.jclepro.2022.131897.
- Hofrichter, M. 2002. “Review: Lignin Conversion by Manganese Peroxidase (MnP).” Enzyme and Microbial Technology 30 (4): 454–466. doi:10.1016/S0141-0229(01)00528-2.
- Horan, N., A. Z. Yaser, and N. Wid. 2018. Anaerobic Digestion Preocesses: Application and Effluent Treatment. Singapore: Springer Nature Singapore Pte Ltd.
- Intasit, R., J. Yeesang, and B. Cheirsilp. 2019. “Biological Pretreatment of Empty Fruit Bunch (EFB) Using Oleaginous Aspergillus tubingensis TSIP9.” Journal of Water and Environment Technology 17: 244–250. doi:10.2965/jwet.18-054.
- Ishmael, U. C., R. S. Shah, J. V. Palliah, M. F. F. Asras, S. S. Ahmad, and V. B. Ayodele. 2016. “Statistical Modeling and Optimization of Enzymatic Pretreatment of Empty Fruit Bunches with Laccase Enzyme.” BioResources 11 (2): 5013–5032. doi:10.1016/j.biombioe.2019.105442.
- Ishola, M. M., I. Isroi, and M. J. Taherzadeh. 2014. “Effect of Fungal and Phosphoric Acid Pretreatment on Ethanol Production from oil Palm Empty Fruit Bunches (OPEFB).” Bioresource Technology 165: 9–12. doi:10.1016/j.biortech.2014.02.053.
- Ishola, M. M., R. Millati, S. Syamsiah, M. N. Cahyanto, C. Niklasson, and M. J. Taherzadeh. 2012. “Structural Changes of oil Palm Empty Fruit Bunch (OPEFB) After Fungal and Phosphoric Acid Pretreatment.” Molecule 17 (12): 4995–15012.
- Isroi, I. 2017. “Characteristic of oil Palm Empty Fruit Bunch Pretreated with Pleurotus floridanus.” Menara Perkebunan 85 (2): 67–76. doi:10.22302/iribb.jur.mp.8v5i2.234.
- Jaman, K., N. Amir, M. A. Musa, A. Zainal, L. Yahya, A. M. Abdul Wahab, S. Suhartini, T. N. Tuan Mohd Marzuki, R. Harun, and S. Idrus. 2022. “Anaerobic Digestion, Codigestion of Food Waste, and Chicken Dung: Correlation of Kinetic Parameters with Digester Performance and on-Farm Electrical Energy Generation Potential.” Fermentation 8 (28): 1–19. doi:10.3390/fermentation8010028.
- Janke, L., B. K. McCabe, P. Harris, A. Hill, S. Lee, S. Weinrich, S. Marchuk, and C. Baillie. 2019. “Ensiling Fermentation Reveals Pre-Treatment Effects for Anaerobic Digestion of Sugarcane Biomass: An Assessment of Ensiling Additives on Methane Potential.” Bioresource Technology 279: 398–403. doi:10.1016/j.biortech.2019.01.143.
- Jansson, A. T., R. J. Patinvoh, I. Sárvári Horváth, and M. J. Taherzadeh. 2019. “Dry Anaerobic Digestion of Food and Paper Industry Wastes at Different Solid Contents.” Fermentation 5 (2): 1–10. doi:10.3390/fermentation5020040.
- Jin, W., X. Xu, F. Yang, C. Li, and M. Zhou. 2018. “Performance Enhancement by Rumen Cultures in Anaerobic Co-Digestion of Corn Straw with pig Manure.” Biomass and Bioenergy 115: 120–129. doi:10.1016/j.biombioe.2018.05.001.
- Jumali, S. S., and S. Ismail. 2017. “Ganoderma Boninense Efficacy in Delignifying oil Palm Empty Fruit Bunches.” Pertanika Journal of Science & Technology 25: 1–8.
- Kainthola, J., A. Podder, M. Fechner, and R. Goel. 2021. “An Overview of Fungal Pretreatment Processes for Anaerobic Digestion: Applications, Bottlenecks and Future Needs.” Bioresource Technology 321: 1–11. doi:10.1016/j.biortech.2020.124397.
- Kamcharoen, A., V. Champreda, L. Eurwilaichitr, and P. Boonsawang. 2014. “Screening and Optimization of Parameters Affecting Fungal Pretreatment of oil Palm Empty Fruit Bunch (EFB) by Experimental Design.” International Journal of Energy and Environmental Engineering 5 (4): 303–312. doi:10.1007/s40095-014-0136-y.
- Kannaiyan, R., N. Mahinpey, V. Kostenko, and R. J. Martinuzzi. 2017. “Enhanced Delignification of Wheat Straw by the Combined Effect of Hydrothermal and Fungal Treatments.” Chemical Engineering Communications 204 (7): 803–812. doi:10.1080/00986445.2017.1322961.
- Kasivisvanathan, H., R. T. L. Ng, D. H. S. Tay, and D. K. S. Ng. 2012. “Fuzzy Optimisation for Retrofitting a Palm oil Mill Into a Sustainable Palm oil-Based Integrated Biorefinery.” Chemical Engineering Journal 200-202: 694–709. doi:10.1016/j.cej.2012.05.113.
- Kawai, M., I. F. Purwanti, N. Nagao, A. Slamet, J. Hermana, and T. Toda. 2012. “Seasonal Variation in Chemical Properties and Degradability by Anaerobic Digestion of Landfill Leachate at Benowo in Surabaya, Indonesia.” Journal of Environmental Management 110: 267–275. doi:10.1016/j.jenvman.2012.06.022.
- Kerdsuwan, S., and K. Laohalidanond. 2011. Renewable Energy from Palm oil Empty Fruit Bunch, edited by M. Nayeripour, 123–150. Shanghai: InTech.
- Khalid, A., M. Arshad, M. Anjum, T. Mahmood, and L. Dawson. 2011. “The Anaerobic Digestion of Solid Organic Waste.” Waste Management 31 (8): 1737–1744.
- Khalil, M., M. A. Berawi, R. Heryanto, and A. Rizalie. 2019. “Waste to Energy Technology: The Potential of Sustainable Biogas Production from Animal Waste in Indonesia.” Renewable and Sustainable Energy Reviews 105: 323–331. doi:10.1016/j.rser.2019.02.011.
- Khangkhachit, W., W. Suyotha, C. Leamdum, and P. Prasertsan. 2021. “Production of Thermostable Xylanase Using Streptomyces Thermocarboxydus ME742 and Application in Enzymatic Conversion of Xylan from oil Palm Empty Fruit Bunch to Xylooligosaccharides.” Biocatalysis and Agricultural Biotechnology 37: 1–13. doi:10.1016/j.bcab.2021.102180.
- Kim, S. H., S. M. Choi, H. J. Ju, and J. Y. Jung. 2013. “Mesophilic Co-Digestion of Palm oil Mill Effluent and Empty Fruit Bunches.” Environmental Technology 34 (13-14): 2163–2170. doi:10.1080/09593330.2013.826253.
- Korres, N. E., P. O’Kiely, J. A. Benzie, and J. S. West. 2013. Bioenergy Production by Anearobic Digestion. Abingdon: Routledge.
- Koupaie, E. H., S. Dahadha, A. A. Bazyar Lakeh, A. Azizi, and E. Elbeshbishy. 2019. “Enzymatic Pretreatment of Lignocellulosic Biomass for Enhanced Biomethane Production-A Review.” Journal of Environmental Management 233: 774–784. doi:10.1016/j.jenvman.2018.09.106.
- Kristiani, A., N. Effendi, Y. Aristiawan, F. Aulia, and Y. Sudiyani. 2015. “Effect of Combining Chemical and Irradiation Pretreatment Process to Characteristic of oil Palm’s Empty Fruit Bunches as raw Material for Second Generation Bioethanol.” Energy Procedia 68: 195–204. doi:10.1016/j.egypro.2015.03.248.
- Kumar, B., N. Bhardwaj, K. Agrawal, V. Chaturvedi, and P. Verma. 2020. “Current Perspective on Pretreatment Technologies Using Lignocellulosic Biomass: An Emerging Biorefinery Concept.” Fuel Processing Technology 199: 106244. doi:10.1016/j.fuproc.2019.106244.
- Kumar, P., E. M. Eid, M. A. Taher, M. H. E. El-Morsy, H. E. M. Osman, D. A. Al-Bakre, B. Adelodun, et al. 2022. “Biotransforming the Spent Substrate of Shiitake Mushroom (Lentinula edodes Berk.).” A Synergistic Approach to Biogas Production and Tomato (Solanum Lycopersicum L.). Horticulturae 8 (479): 1–15. doi:10.3390/horticulturae8060479.
- Kurian, J. K., G. R. Nair, A. Hussain, and G. S. V. Raghavan. 2013. “Feedstocks, Logistics and Pre-Treatment Processes for Sustainable Lignocellulosic Biorefineries: A Comprehensive Review.” Renewable and Sustainable Energy Reviews 25: 205–219. doi:10.1016/j.rser.2013.04.019.
- Lau, M. J., M. W. Lau, C. Gunawan, and B. E. Dale. 2010. “Ammonia Fiber Expansion (AFEX) Pretreatment, Enzymatic Hydrolysis, and Fermentation on Empty Palm Fruit Bunch Fiber (EPFBF) for Cellulosic Ethanol Production.” Applied Biochemistry and Biotechnology 162: 1847–1857. doi:10.1007/s12010-010-8962-8.
- Lee, K.-T., J.-Y. Tsai, A. T. Hoang, W.-H. Chen, D. S. Gunarathne, K.-Q. Tran, A. Selvarajoo, and V. Goodarzi. 2022. “Energy-Saving Drying Strategy of Spent Coffee Grounds for Co-Firing Fuel by Adding Biochar for Carbon Sequestration to Approach net Zero.” Fuel 326 (124984): 1–13. doi:10.1016/j.fuel.2022.124984.
- Lemmer, A., W. Merkle, K. Baer, and F. Graf. 2017. “Effects of High-Pressure Anaerobic Digestion up to 30 bar on pH-Value, Production Kinetics and Specific Methane Yield.” Energy 138: 659–667. doi:10.1016/j.energy.2017.07.095.
- Li, Y., Y. Chen, and J. Wu. 2019b. “Enhancement of Methane Production in Anaerobic Digestion Process: A Review.” Applied Energy 240: 120–137. doi:10.1016/j.apenergy.2019.01.243.
- Li, F., Z. Ding, W. Ke, D. Xu, P. Zhang, J. Bai, S. Mudassar, I. Muhammad, and X. Guo. 2019a. “Ferulic Acid Esterase-Producing Lactic Acid Bacteria and Cellulase Pretreatments of Corn Stalk Silage at two Different Temperatures: Ensiling Characteristics, Carbohydrates Composition and Enzymatic Saccharification.” Bioresource Technology 282: 211–221.
- Li, Y., L. Liu, Q. Wang, J. Wu, T. Liu, H. Liu, Y. Hong, and T. Huang. 2021a. “Enhanced Anaerobic co-Metabolism of Coal Gasification Wastewater via the Assistance of Zero-Valent Iron.” Journal of Water Process Engineering 40: 101817. doi:10.1016/j.jwpe.2020.101817.
- Li, Y., Z. Wang, T. Li, S. Jiang, Z. Sun, H. Jiang, M. Qian, H. Zhou, and Q. Xu. 2020. “Changes in Microbial Community and Methanogenesis During High-Solid Anaerobic Digestion of Ensiled Corn Stover.” Journal of Cleaner Production 242: 118479. doi:10.1016/j.jclepro.2019.118479.
- Li, Y., Q. Wang, L. Liu, S. Tabassum, J. Sun, and Y. Hong. 2021b. “Enhanced Phenols Removal and Methane Production with the Assistance of Graphene Under Anaerobic co-Digestion Conditions.” Science of The Total Environment 759: 143523. doi:10.1016/j.scitotenv.2020.143523.
- Liew, Z. K., Y. J. Chan, Z. T. Ho, Y. H. Yip, M. C. Teng, A. I. T. Ameer Abbas bin, S. Chong, P. L. Show, and C. L. Chew. 2021. “Biogas Production Enhancement by Co-Digestion of Empty Fruit Bunch (EFB) with Palm oil Mill Effluent (POME): Performance and Kinetic Evaluation.” Renewable Energy 179: 766–777. doi:10.1016/j.renene.2021.07.073.
- Lim, S. H., and D. Ibrahim. 2013. “Bioconversion of oil Palm Frond by Aspergillus niger to Enhances It’s Fermentable Sugar Production.” Pakistan Journal of Biological Sciences 16 (18): 920–926.
- Lin, L., F. Xu, X. Ge, and Y. Li. 2018. “Improving the Sustainability of Organic Waste Management Practices in the Food-Energy-Water Nexus: A Comparative Review of Anaerobic Digestion and Composting.” Renewable and Sustainable Energy Reviews 89: 151–167. doi:10.1016/j.rser.2018.03.025.
- Loh, S. K. 2017. “The Potential of the Malaysian oil Palm Biomass as a Renewable Energy Source.” Energy Conversion and Management 141: 285–298. doi:10.1016/j.enconman.2016.08.081.
- Lopes, A. D. M., E. Ferreira Filho, and L. Moreira. 2018. “An Update on Enzymatic Cocktails for Lignocellulose Breakdown.” Journal of Applied Microbiology 125 (3): 632–645. doi:10.1111/jam.13923.
- Lu, X., N. Li, X. Qiao, Z. Qiu, and P. Liu. 2018. “Effects of Thermal Treatment on Polysaccharide Degradation During Black Garlic Processing.” LWT 95: 223–229. doi:10.1016/j.lwt.2018.04.059.
- Lü, J., C. Sheahan, and P. Fu. 2011. “Metabolic Engineering of Algae for Fourth Generation Biofuels Production.” Energy & Environmental Science 4 (7): 2451–2466. doi:10.1039/C0EE00593B.
- Ma, Z., J. Wang, C. Li, Y. Yang, X. Liu, C. Zhao, and D. Chen. 2019. “New Sight on the Lignin Torrefaction Pretreatment: Relevance Between the Evolution of Chemical Structure and the Properties of Torrefied Gaseous, Liquid, and Solid Products.” Bioresource Technology 288: 1–9. doi:10.1016/j.biortech.2019.121528.
- Mahdy, A., L. Mendez, M. Ballesteros, and C. González-Fernández. 2015. “Protease Pretreated Chlorella vulgaris Biomass Bioconversion to Methane via Semi-Continuous Anaerobic Digestion.” Fuel 158: 35–41. doi:10.1016/j.fuel.2015.04.052.
- Majesty, K., and H. Herdiansyah. 2019. The Empty Palm oil Fruit Bunch as the Potential Source of Biomass in Furfural Production in Indonesia: Preliminary Process Design and Environmental Perspective, 012096–012096. Bristol: IOP Publishing.
- Mamimin, C., S. Chanthong, C. Leamdum, S. O-Thong, and P. Prasertsan. 2021. “Improvement of Empty Palm Fruit Bunches Biodegradability and Biogas Production by Integrating the Straw Mushroom Cultivation as a Pretreatment in the Solid-State Anaerobic Digestion.” Bioresource Technology 319: 1–10. doi:10.1016/j.biortech.2020.124227.
- Mao, C., Y. Feng, X. Wang, and G. Ren. 2015. “Review on Research Achievements of Biogas from Anaerobic Digestion.” Renewable and Sustainable Energy Reviews 45: 540–555. doi:10.1016/j.rser.2015.02.032.
- Mardawati, E., B. M. Harahap, E. A. Febrianti, A. T. Hartono, N. P. Siahaan, A. Wulandari, S. Yudiastuti, S. Suhartini, and K. Kasbawati. 2022. “Integrated and Partial Process of Xylitol and Bioethanol Production from oil Palm Empty Fruit Bunches.” Advances in Food Science, Sustainable Agriculture and Agroindustrial Engineering (AFSSAAE) 5 (1): 68–76. doi:10.21776/ub.afssaae.2022.005.01.5.
- Mardawati, E., N. Maharani, D. W. Wira, B. M. Harahap, T. Yuliana, and E. Sukarminah. 2018. “Xylitol Production from oil Palm Empty Fruit Bunches (OPEFB) via Simultaneous Enzymatic Hydrolysis and Fermentation Process.” Journal of Industrial and Information Technology in Agriculture 2 (1): 29–34. doi:10.24198/jiita.v2i1.
- Mardawati, E., A. V. Putri, T. Yuliana, S. Rahimah, S. Nurjanah, and I. Hanidah. 2019. “Effects of Substrate Concentration on Bioethanol Production from oil Palm Empty Fruit Bunches with Simultaneous Saccharification and Fermentation (SSF).” IOP Conference Series: Earth and Environmental Science 230: 1–9. doi:10.1088/1755-1315/230/1/012079.
- Mardawati, E., A. Trirakhmadi, M. Kresnowati, and T. Setiadi. 2017. “Kinetic Study on Fermentation of Xylose for the Xylitol Production.” Journal of Industrial and Information Technology in Agriculture 1 (1): 1–8. doi:10.24198/jiita.v1i1.
- Mardawati, E., A. Werner, T. Bley, M. T. A. P. Kresnowati, and T. Setiadi. 2014. “The Enzymatic Hydrolysis of oil Palm Empty Fruit Bunches to Xylose.” Journal of the Japan Institute of Energy 93 (10): 973–978. doi:10.3775/jie.93.973.
- Marendra, F., A. Prasetya, R. B. Cahyono, and T. Ariyanto. 2019. “A Sustainability Assessment of Biogas Plant Based on Fruit Waste in Indonesia: Case Study of Biogas Plant Gamping, Yogyakarta.” IOP Conference Series: Materials Science and Engineering, 543, 1–8. doi:10.1088/1757-899x/543/1/012059.
- Martínez-Gutiérrez, E. 2018. “Biogas Production from Different Lignocellulosic Biomass Sources: Advances and Perspectives.” 3 Biotech 8 (5): 1–8. doi:10.1007/s13205-018-1257-4.
- Maryana, R., E. Triwahyuni, T. B. Bardant, Y. Irawan, and Y. Sudiyani. 2021. Potency and Challenges in the Commercialization of Bioethanol First and Second Generation in Indonesia. Proceedings The SATREPS Conference, 79–84.
- Mata-Alvarez, J., J. Dosta, M. S. Romero-Güiza, X. Fonoll, M. Peces, and S. Astals. 2014. “A Critical Review on Anaerobic co-Digestion Achievements Between 2010 and 2013.” Renewable Sustainable Energy Review 36: 412–427.
- Mayulu, H. 2014. “The Nutrient Digestibility of Locally Sheep fed with Amofer Palm oil Byproduct-Based Complete Feed.” International Journal of Science and Engineering 7 (2): 106–112. doi:10.12777/ijse.7.1.106-111.
- Md Razali, N., M. Ibrahim, E. Kamal Bahrin, and S. Abd-Aziz. 2018. “Optimisation of Simultaneous Saccharification and Fermentation (SSF) for Biobutanol Production Using Pretreated oil Palm Empty Fruit Bunch.” Molecules 23 (1944): 1–17. doi:10.3390/molecules23081944.
- Mehta, V., J. K. Gupta, and S. C. Kaushal. 1990. “Cultivation of Pleurotus Florida Mushroom on Rice Straw and Biogas Production from the Spent Straw.” World Journal of Microbiology and Biotechnology 6 (4): 366–370. doi:10.1007/bf01202116.
- Meijaard, E., T. M. Brooks, K. M. Carlson, E. M. Slade, J. Garcia-Ulloa, D. L. A. Gaveau, J. S. H. Lee, et al. 2020. “The Environmental Impacts of Palm oil in Context.” Nature Plants 6 (12): 1418–1426. doi:10.1038/s41477-020-00813-w.
- Menardo, S., P. Balsari, E. Tabacco, and G. Borreani. 2015. “Effect of Conservation Time and the Addition of Lactic Acid Bacteria on the Biogas and Methane Production of Corn Stalk Silage.” BioEnergy Research 8 (4): 1810–1823. doi:10.1007/s12155-015-9637-7.
- Mendieta, O., L. Castro, E. Vera, J. Rodríguez, and H. Escalante. 2021. “Toward the Adoption of Anaerobic Digestion Technology Through low-Cost Biodigesters: A Case Study of Non-Centrifugal Cane Sugar Producers in Colombia.” Water 13 (18): 1–16. doi:10.3390/w13182566.
- Milledge, J. J., and P. J. Harvey. 2016. “Ensilage and Anaerobic Digestion of Sargassum muticum.” Journal of Applied Phycology 28 (5): 3021–3030. doi:10.1007/s10811-016-0804-9.
- Ming, C., A. Dilokpimol, C. Zou, W. Liao, L. Zhao, M. Wang, R. P. de Vries, and Y. Kang. 2019. “The Quest for Fungal Strains and Their Co-Culture Potential to Improve Enzymatic Degradation of Chinese Distillers’ Grain and Other Agricultural Wastes.” International Biodeterioration & Biodegradation 144: 1–12. doi:10.1016/j.ibiod.2019.104765.
- MEMR (Ministry of Energy and Mineral Resources). 2019. Handbook of Energy and Economic Statistics of Indonesia. Jakarta: Ministry of Energy and Mineral Resources.
- Mishra, S., P. K. Singh, S. Dash, and R. Pattnaik. 2018. “Microbial Pretreatment of Lignocellulosic Biomass for Enhanced Biomethanation and Waste Management.” 3 Biotech 8 (458): 1–12. doi:10.1007/s13205-018-1480-z.
- Mmanywa, M. S., and A. M. Mshandete. 2017. “Co-production of Coprinus cinereus (Schaeff.) S. Gray. s. Lato Mushrooms and Biogas from Palm oil Wastes in Tanzania.” Biotechnology Journal International 17 (3): 1–13. doi:10.9734/BJI/2017/30479.
- Mohanan, K., R. R. Ratnayake, K. Mathaniga, C. L. Abayasekara, and N. Gnanavelrajah. 2014. “Effect of Co-Culturing of Cellulolytic Fungal Isolates for Degradation of Lignocellulosic Material.” Journal of Yeast and Fungal Research 5 (3): 31–38. doi:10.5897/JYFR2014.0134.
- Mohd-Setapar, S. H., N. Abd-Talib, and R. Aziz. 2012. “Review on Crucial Parameters of Silage Quality.” APCBEE Procedia 3: 99–103. doi:10.1016/j.apcbee.2012.06.053.
- Montoya-Rosales, J. J., M. Peces, L. M. González-Rodríguez, F. Alatriste-Mondragón, and D. K. Villa-Gómez. 2020. “A Broad Overview Comparing a Fungal, Thermal and Acid pre-Treatment of Bean Straw in Terms of Substrate and Anaerobic Digestion Effect.” Biomass and Bioenergy 142: 1–8. doi:10.1016/j.biombioe.2020.105775.
- Moravvej, Z., M. A. Makarem, and M. R. Rahimpour. 2019. “Chapter 20 - The Fourth Generation of Biofuel.” In Second and Third Generation of Feedstocks: The Evolution of Biofuels, edited by A. Basile and F. Dalena, 557–597. Oxford: Elsevier.
- Muck, R. E. 2012. Microbiology of Ensiling. 16th International Silage Conference Proceeding, 2012, Finland. Hameenlinna, 75–86.
- Munawar, S. S., and B. Subiyanto. 2014. “Characterization of Biomass Pellet Made from Solid Waste oil Palm Industry.” Procedia Environmental Sciences 20: 336–341. doi:10.1016/j.proenv.2014.03.042.
- Mustafa, A. M., T. G. Poulsen, and K. Sheng. 2016. “Fungal Pretreatment of Rice Straw with Pleurotus ostreatus and Trichoderma reesei to Enhance Methane Production Under Solid-State Anaerobic Digestion.” Applied Energy 180: 661–671. doi:10.1016/j.apenergy.2016.07.135.
- Muthangya, M., A. Manoni Mshandete, and A. Kajumulo Kivaisi. 2009. “Two-stage Fungal Pre-Treatment for Improved Biogas Production from Sisal Leaf Decortication Residues.” International Journal of Molecular Sciences 10 (11): 4805–4815. doi:10.3390/ijms10114805.
- Neves, L., R. Oliveira, and M. M. Alves. 2004. “Influence of Inoculum Activity on the Bio-Methanization of a Kitchen Waste Under Different Waste/Inoculum Ratios.” Process Biochemistry 39 (12): 2019–2024. doi:10.1016/j.procbio.2003.10.002.
- Nguyen, X. P., N. D. Le, V. V. Pham, T. T. Huynh, V. H. Dong, and A. T. Hoang. 2021b. “Mission, Challenges, and Prospects of Renewable Energy Development in Vietnam.” Energy Sources, Part A: Recovery, Utilization, and Environmental Effects, 1–13. doi:10.1080/15567036.2021.1965264.
- Nguyen, H. P., P. Q. H. Le, V. V. Pham, X. P. Nguyen, D. Balasubramaniam, and A.-T. Hoang. 2021a. “Application of the Internet of Things in 3E (Efficiency, Economy, and Environment) Factor-Based Energy Management as Smart and Sustainable Strategy.” Energy Sources, Part A: Recovery, Utilization, and Environmental Effects, 1–23. doi:10.1080/15567036.2021.1954110.
- Nieves, D. C., K. Karimi, and I. S. Horváth. 2011. “Improvement of Biogas Production from oil Palm Empty Fruit Bunches (OPEFB).” Industrial Crops and Products 34: 1097–1101. doi:10.1016/j.indcrop.2011.03.022.
- Nolan, P., E. M. Doyle, J. Grant, and P. O’Kiely. 2018. “Upgrading Grass Biomass During Ensiling with Contrasting Fibrolytic Enzyme Additives for Enhanced Methane Production.” Renewable Energy 115: 462–473. doi:10.1016/j.renene.2017.07.039.
- Norrahim, M. N. F., M. A. A. Farid, A. A. Lawal, T. A. T. Yasim-Anuar, M. H. Samsudin, and A. A. Zulkifli. 2022. “Emerging Technologies for Value-Added use of oil Palm Biomass.” Environmental Sciences Advances 1: 259–275. doi:10.1039/d2va00029f.
- Nurdiawati, A., S. Novianti, I. N. Zaini, B. Nakhshinieva, H. Sumida, F. Takahashi, and K. Yoshikawa. 2015. “Evaluation of Hydrothermal Treatment of Empty Fruit Bunch for Solid Fuel and Liquid Organic Fertilizer Co-Production.” Energy Procedia 79: 226–232. doi:10.1016/j.egypro.2015.11.469.
- Nurika, I., E. N. Shabrina, N. Azizah, S. Suhartini, T. D. H. Bugg, and G. C. Barker. 2022. “Application of Ligninolytic Bacteria to the Enhancement of Lignocellulose Breakdown and Methane Production from oil Palm Empty Fruit Bunches (OPEFB).” Bioresource Technology Reports 17 (100951): 1–10. doi:10.1016/j.biteb.2022.100951.
- Nurliyana, M. Y., P. S. H’ng, H. Rasmina, M. S. U. Kalsom, K. L. Chin, S. H. Lee, W. C. Lum, and G. D. Khoo. 2015. “Effect of C/N Ratio in Methane Productivity and Biodegradability During Facultative Co-Digestion of Palm oil Mill Effluent and Empty Fruit Bunch.” Industrial Crops and Products 76: 409–415. doi:10.1016/j.indcrop.2015.04.047.
- Nuryadi, A. P., T. B. Pratomo, and A. A. Raksodewanto. 2019. Analysis of the Feasibility of Small-Biomass Power Generation from the Palm oil Mill–Study Case: Palm oil Mill in Riau-Indonesia, 012018–012018. Bristol: IOP Publishing.
- Nutongkaew, T., P. Prasertsan, C. Leamdum, S. Sattayasamitsathit, and P. Noparat. 2019. “Bioconversion of oil Palm Trunk Residues Hydrolyzed by Enzymes from Newly Isolated Fungi and use for Ethanol and Acetic Acid Production Under two-Stage and Simultaneous Fermentation.” Waste and Biomass Valorization, 1–15. doi:10.1007/s12649-019-00678-x.
- Nyakuma, B., A. Johari, and A. Ahmad. 2012. “Analysis of the Pyrolytic Fuel Properties of Empty Fruit Bunch Briquettes.” Journal of Applied Sciences 12 (24): 2527–2533.
- Nyakuma, B. B., S. Wong, and O. Oladokun. 2021. “Non-oxidative Thermal Decomposition of oil Palm Empty Fruit Bunch Pellets: Fuel Characterisation, Thermogravimetric, Kinetic, and Thermodynamic Analyses.” Biomass Conversion and Biorefinery 11 (4): 1273–1292. doi:10.1007/s13399-019-00568-1.
- O-Thong, S., K. Boe, and I. Angelidaki. 2012. “Thermophilic Anaerobic co-Digestion of oil Palm Empty Fruit Bunches with Palm oil Mill Effluent for Efficient Biogas Production.” Applied Energy 93: 648–654.
- Ohimain, E. I., and S. C. Izah. 2017. “A Review of Biogas Production from Palm oil Mill Effluents Using Different Configurations of Bioreactors.” Renewable and Sustainable Energy Reviews 70: 242–253. doi:10.1016/j.rser.2016.11.221.
- Oktavitri, N. I., W. B. Pratiwi, I. Purnamasari, M. Hayati, M. R. Fitrianingtyas, and S. Hadinnata. 2019. “Anaerobic Digestion of Slaughterhouse Wastewater: CO2 capture of Biogas Using Chlorella vulgaris.” Indonesian Journal of Chemistry 19 (1): 1–8. doi:10.22146/ijc.25129.
- Olatunji, K. O., N. A. Ahmed, and O. Ogunkunle. 2021. “Optimization of Biogas Yield from Lignocellulosic Materials with Different Pretreatment Methods: A Review.” Biotechnology for Biofuels 14 (159): 1–34. doi:10.1186/s13068-021-02012-x.
- Oliva-Merencio, D., I. Pereda-Reyes, U. Schimpf, S. Koehler, and A. J. D. Silva. 2015. “Cellulase Effect on Anaerobic Digestion of Maize Silage Under Discontinuous Operation.” Engenharia Agrícola 35 (5): 951–958. doi:10.1590/1809-4430-Eng.Agric.v35n5p951-958/2015.
- Omar, F. N., H. S. Hafid, A. S. Baharuddin, M. A. P. Mohammed, and J. Abdullah. 2017. “Oil Palm Fiber Biodegradation: Physico-Chemical and Structural Relationships.” Planta 246: 567–577. doi:10.1007/s00425-017-2717-5.
- Pacheco, P., S. Gynch, A. Dermawan, H. Komarudin, and B. Okarda. 2017. The Palm oil Global Value Chain: Implication for Economic Growth and Social and Environmental Sustainability. Bogor: Center for International Forestry Research (CIFOR).
- Pangsang, N., U. Rattanapan, A. Thanapimmetha, P. Srinopphakhun, C. G. Liu, X. Q. Zhao, F. W. Bai, and C. Sakdaronnarong. 2019. “Chemical-free Fractionation of Palm Empty Fruit Bunch and Palm Fiber by hot-Compressed Water Technique for Ethanol Production.” Energy Reports 93: 337–348. doi:10.1016/j.egyr.2019.02.008.
- Panichnumsin, P., A. Nopharatana, B. Ahring, and P. Chaiprasert. 2010. “Production of Methane by co-Digestion of Cassava Pulp with Various Concentrations of pig Manure.” Biomass and Bioenergy 34 (8): 1117–1124. doi:10.1016/j.biombioe.2010.02.018.
- Parbowo, H. S., A. Ardy, and H. Susanto. 2019. Techno-economic Analysis of Dimethyl Ether Production Using oil Palm Empty Fruit Bunches as Feedstock–a Case Study for Riau, 012060–012060. Bristol: IOP Publishing.
- Pastor, L., L. Ruiz, A. Pascual, and B. Ruiz. 2013. “Co-digestion of Used Oils and Urban Landfill Leachates with Sewage Sludge and the Effect on the Biogas Production.” Applied Energy 107: 438–445. doi:10.1016/j.apenergy.2013.02.055.
- Pérez-Chávez, A. M., L. Mayer, and E. Albertó. 2019. “Mushroom Cultivation and Biogas Production: A Sustainable Reuse of Organic Resources.” Energy for Sustainable Development 50: 50–60. doi:10.1016/j.esd.2019.03.002.
- Piñeros-Castro, Y., and M. Velásquez-Lozano. 2014. “Biodegradation Kinetics of oil Palm Empty Fruit Bunches by White rot Fungi.” International Biodeterioration & Biodegradation 91: 24–28.
- Poszytek, K., M. Ciezkowska, A. Sklodowska, and L. Drewniak. 2016. “Microbial Consortium with High Cellulolytic Activity (MCHCA) for Enhanced Biogas Production.” Frontiers in Microbiology 7 (324): 1–11. doi:10.3389/fmicb.2016.00324.
- Pramudya, E. P., O. Hospes, and C. J. A. M. Termeer. 2017. “Governing the Palm-Oil Sector Through Finance: The Changing Roles of the Indonesian State.” Bulletin of Indonesian Economic Studies 53 (1): 57–82. doi:10.1080/00074918.2016.1228829.
- Prasad, R. K., S. Chatterjee, P. B. Mazumder, S. K. Gupta, S. Sharma, M. G. Vairale, S. Datta, S. K. Dwivedi, and D. K. Gupta. 2019. “Bioethanol Production from Waste Lignocelluloses: A Review on Microbial Degradation Potential.” Chemosphere 231: 588–606. doi:10.1016/j.chemosphere.2019.05.142.
- Priadi, C., D. Wulandari, I. Rahmatika, and S. S. Moersidik. 2014. “Biogas Production in the Anaerobic Digestion of Paper Sludge.” APCBEE Procedia 9: 65–69. doi:10.1016/j.apcbee.2014.01.012.
- Purnomo, H., B. Okarda, A. Dermawan, Q. P. Ilham, P. Pacheco, F. Nurfatriani, and E. Suhendang. 2020. “Reconciling oil Palm Economic Development and Environmental Conservation in Indonesia: A Value Chain Dynamic Approach.” Forest Policy and Economics 111: 1-12. doi:10.1016/j.forpol.2020.102089.
- Purnomo, A., M. Suprihatin, and U. Hasanudin. 2018. “Comparison of Biogas Production from oil Palm Empty Fruit Bunches of Post-Mushroom Cultivation Media (EFBMM) from Semi wet and dry Fermentation.” Journal of Environmental & Earth Science. 8 (6): 88–96.
- Purwandari, F. A., A. P. Sanjaya, R. Millati, M. N. Cahyanto, I. S. Horváth, C. Niklasson, and M. J. Taherzadeh. 2013. “Pretreatment of oil Palm Empty Fruit Bunch (OPEFB) by N-Methylmorpholine-N-Oxide (NMMO) for Biogas Production: Structural Changes and Digestion Improvement.” Bioresource Technology 128: 461–466. doi:10.1016/j.biortech.2012.10.088.
- Purwono, B. S. A., and Rahbini Suyanta. 2013. “Biogas Digester as an Alternative Energy Strategy in the Marginal Villages in Indonesia.” Energy Procedia 32: 136–144.
- Quiñones, T. S., M. Plöchl, J. Budde, and M. Heiermann. 2011. “Enhanced Methane Formation Through Application of Enzymes: Results from Continuous Digestion Tests.” Energy & Fuels 25 (11): 5378–5386.
- Rabii, A., S. Aldin, Y. Dahman, and E. Elbeshbishy. 2019. “A Rreview on Anaerobic co-Digestion with a Focus on the Microbial Populations and the Effect of Multi-Stage Digester Configuration.” Energies 12: 6. doi:10.3390/en12061106.
- Rahmi, M., B. Taufiq, R. Indah, K. Syahputri, M. Mangara, and A. Wandika. 2019. Economic Feasibility Analysis of Hydrogen Production from raw Materials of oil Palm Empty Fruit Bunches, 012052–012052. Bristol: IOP Publishing.
- Ramlee, N. A., M. Jawaid, S. A. K. Yamani, E. S. Zainudin, and S. Alamery. 2021a. “Effect of Surface Treatment on Mechanical, Physical and Morphological Properties of oil Palm/Bagasse Fiber Reinforced Phenolic Hybrid Composites for Wall Thermal Insulation Application.” Construction and Building Materials 276: 1–10. doi:10.1016/j.conbuildmat.2020.122239.
- Ramlee, N. A., J. Naveen, and M. Jawaid. 2021b. “Potential of oil Palm Empty Fruit Bunch (OPEFB) and Sugarcane Bagasse Fibers for Thermal Insulation Application – A Review.” Construction and Building Materials 271: 1–16. doi:10.1016/j.conbuildmat.2020.121519.
- Rasapoor, M., B. Young, R. Brar, A. Sarmah, W. Q. Zhuang, and S. Baroutian. 2020. “Recognizing the Challenges of Anaerobic Digestion: Critical Steps Toward Improving Biogas Generation.” Fuel 261: 1–12. doi:10.1016/j.fuel.2019.116497.
- Ravindran, R., and A. K. Jaiswal. 2016. “A Comprehensive Review on Pre-Treatment Strategy for Lignocellulosic Food Industry Waste: Challenges and Opportunities.” Bioresource Technology 199: 92–102. doi:10.1016/j.biortech.2015.07.106.
- Rekleitis, G., K.-J. Haralambous, M. Loizidou, and K. Aravossis. 2020. “Utilization of Agricultural and Livestock Waste in Anaerobic Digestion (A.D): Applying the Biorefinery Concept in a Circular Economy.” Energies 13 (17): 1–14. doi:10.3390/en13174428.
- Richard, E. N., A. Hilonga, R. L. Machunda, and K. N. Njau. 2020. “Two-Stage Banana Leaves Wastes Utilization Towards Mushroom Growth and Biogas Production.” 3 Biotech 10 (542): 1–12. doi:10.1007/s13205-020-02525-6.
- Rincón, B., C. J. Banks, and S. Heaven. 2010. “Biochemical Methane Potential of Winter Wheat (Triticum aestivum L.): Influence of Growth Stage and Storage Practice.” Bioresource Technology 101 (21): 8179–8184. doi:10.1016/j.biortech.2010.06.039.
- Rincón, B., S. Heaven, C. J. Banks, and Y. Zhang. 2012. “Anaerobic Digestion of Whole-Crop Winter Wheat Silage for Renewable Energy Production.” Energy & Fuels 26 (4): 2357–2364. doi:10.1021/ef201985x.
- Risdianto, H., T. Kardiansyah, and A. Sugiharto. 2016. “Empty Fruit Bunches for Pulp and Paper Production: The Current State in Indonesia.” Journal of Korea TAPPI 48 (6): 25–31. doi:10.7584/JKTAPPI.2016.12.48.6.25.
- Rizal, N. F. A. A., M. F. Ibrahim, M. R. Zakaria, S. Abd-Aziz, P. L. Yee, and M. A. Hassan. 2018. “Pre-Treatment of oil Palm Biomass for Fermentable Sugars Production.” Molecules 23 (1381): 1–14. doi:10.3390/molecules23061381.
- Rocamora, I., S. T. Wagland, R. Villa, E. W. Simpson, O. Fernández, and Y. Bajón-Fernández. 2020. “Dry Anaerobic Digestion of Organic Waste: A Review of Operational Parameters and Their Impact on Process Performance.” Bioresource Technology 299: 1–11. doi:10.1016/j.biortech.2019.122681.
- Rohma, N. A., S. Suhartini, and I. Nurika. 2021. “Chemical Pre-Treatments on oil Palm Empty Fruit Bunches: Impacts on Characteristics and Methane Potential.” IOP Conference Series: Earth and Environmental Science 924 (012071): 1–11. doi:10.1088/1755-1315/924/1/012071.
- Rollini, M., C. Sambusiti, A. Musatti, E. Ficara, I. Retinò, and F. Malpei. 2014. “Comparative Performance of Enzymatic and Combined Alkaline-Enzymatic Pretreatments on Methane Production from Ensiled Sorghum Forage.” Bioprocess and Biosystems Engineering 37 (12): 2587–2595. doi:10.1007/s00449-014-1235-0.
- Rosado, F. R., C. Kemmelmeier, and S. M. Da Costa. 2002. “Alternative Method of Inoculum and Spawn Production for the Cultivation of the Edible Brazilian Mushroom Pleurotus ostreatoroseus SING.” Journal of Basic Microbiology 42 (1): 37–44. doi:10.1002/1521-4028(200203)42:137::Aid-jobm37>3.0.Co;2-s.
- Rouches, E., R. Escudié, E. Latrille, and H. Carrère. 2019. “Solid-State Anaerobic Digestion of Wheat Straw: Impact of S/I Ratio and Pilot-Scale Fungal Pretreatment.” Waste Management 85: 464–476. doi:10.1016/j.wasman.2019.01.006.
- Rouches, E., I. Herpoël-Gimbert, J. P. Steyer, and H. Carrere. 2016. “Improvement of Anaerobic Degradation by White-rot Fungi Pretreatment of Lignocellulosic Biomass: A Review.” Renewable and Sustainable Energy Reviews 59: 179–198. doi:10.1016/j.rser.2015.12.317.
- Saelor, S., P. Kongjan, and S. O-Thong. 2017. “Biogas Production from Anaerobic co-Digestion of Palm oil Mill Effluent and Empty Fruit Bunches.” Energy Procedia 138: 717–722. doi:10.1016/j.egypro.2017.10.206.
- Saha, B. C., N. Qureshi, G. J. Kennedy, and M. A. Cotta. 2016. “Biological Pretreatment of Corn Stover with White-Rot Fungus for Improved Enzymatic Hydrolysis.” International Biodeterioration & Biodegradation 109: 29–35. doi:10.1016/j.ibiod.2015.12.020.
- Saidu, M., A. Yuzir, M. R. Salim, Salmiati, S. Azman, and N. Abdullah. 2014. “Biological pre-Treated oil Palm Mesocarp Fibre with Cattle Manure for Biogas Production by Anaerobic Digestion During Acclimatization Phase.” International Biodeterioration & Biodegradation 95: 189–194. doi:10.1016/j.ibiod.2014.06.014.
- Saini, S., and K. K. Sharma. 2021. “Fungal Lignocellulolytic Enzymes and Lignocellulose: A Critical Review on Their Contribution to Multiproduct Biorefinery and Global Biofuel Research.” International Journal of Biological Macromolecules 193: 2304–2319. doi:10.1016/j.ijbiomac.2021.11.063.
- Salafudin, R. H. Setyobudi, S. K. Wahono, A. Nindita, P. G. Adinurani, Y. A. Nugroho, A. Sasmito, and T. Liwang. 2015. “Biological Purification System: Integrated Biogas from Small Anaerobic Digestion and Natural Microalgae.” Procedia Chemistry 14: 387–393. doi:10.1016/j.proche.2015.03.069.
- Salihu, A., and M. Z. Alam. 2016. “Pretreatment Methods of Organic Wastes for Biogas Production.” Journal of Applied Sciences 16 (3): 124–137. doi:10.3923/jas.2016.124.137.
- Sambusiti, C., E. Ficara, F. Malpei, J. P. Steyer, and H. Carrère. 2013a. “Benefit of Sodium Hydroxide Pretreatment of Ensiled Sorghum Forage on the Anaerobic Reactor Stability and Methane Production.” Bioresource Technology 144: 149–155. doi:10.1016/j.biortech.2013.06.095.
- Sambusiti, C., F. Monlau, E. Ficara, H. Carrère, and F. Malpei. 2013b. “A Comparison of Different pre-Treatments to Increase Methane Production from two Agricultural Substrates.” Applied Energy 104: 62–70. doi:10.1016/j.apenergy.2012.10.060.
- Santi, L. P., D. N. Kalbuadi, and D. H. Goenadi. 2019. “Empty Fruit Bunches as Potential Source for Biosilica Fertilizer for oil Palm.” Journal of Tropical Biodiversity and Biotechnology 4 (3): 90–96. doi:10.22146/jtbb.38749.
- Saritpongteeraka, K., S. Chaiprapat, P. Boonsawang, and S. Sung. 2015. “Solid State co-Fermentation as Pretreatment of Lignocellulosic Palm Empty Fruit Bunch for Organic Acid Recovery and Fiber Property Improvement.” International Biodeterioration & Biodegradation 100: 172–180. doi:10.1016/j.ibiod.2015.03.001.
- Sawatdeenarunat, C., K. C. Surendra, D. Takara, H. Oechsner, and S. K. Khanal. 2015. “Anaerobic Digestion of Lignocellulosic Biomass: Challenges and Opportunities.” Bioresource Technology 178: 178–186. doi:10.1016/j.biortech.2014.09.103.
- Sayara, T., and A. Sánchez. 2019. “A Review on Anaerobic Digestion of Lignocellulosic Wastes: Pretreatments and Operational Conditions.” Applied Sciences 9 (21): 1–23. doi:10.3390/app9214655.
- Schroyen, M., H. Vervaeren, S. W. H. Van Hulle, and K. Raes. 2014. “Impact of Enzymatic Pretreatment on Corn Stover Degradation and Biogas Production.” Bioresource Technology 173: 59–66. doi:10.1016/j.biortech.2014.09.030.
- Schroyen, M., H. Vervaeren, H. Vandepitte, S. W. H. Van Hulle, and K. Raes. 2015. “Effect of Enzymatic Pretreatment of Various Lignocellulosic Substrates on Production of Phenolic Compounds and Biomethane Potential.” Bioresource Technology 192: 696–702. doi:10.1016/j.biortech.2015.06.051.
- Shah, T. A., C. C. Lee, W. J. Orts, and R. Tabassum. 2019. “Biological Pretreatment of Rice Straw by Ligninolytic Bacillus sp. Strains for Enhancing Biogas Production.” Environmental Progress & Sustainable Energy 38 (3): e13036. doi:10.1002/ep.13036.
- Shahbandeh, M. 2020. Production Volume of Palm oil Worldwide from 2012/2013 to 2019/2020.
- Shahbandeh, M. 2021. Palm oil: Global Production Volume 2012/13-2020/21.
- Shahriarinour, M., M. N. A. Wahab, A. B. Ariff, S. Mustafa, and R. Mohamad. 2011. “Effect of Various Pretreatments of oil Palm Empty Fruit Bunch Fibres for Subsequent use as Substrate on the Performance of Cellulase Production by Aspergillus terreus.” BioResources 6 (1): 291–307.
- Show, K.-Y., Y. Yan, and D.-J. Lee. 2019. “Chapter 16 - Bioreactor and Bioprocess Design for Biohydrogen Production.” In Biohydrogen - Biomass, Biofuels, Biochemicals, edited by A. Pandey, S. V. Mohan, J.-S. Chang, P. C. Hallenbecka, and C. Larroche, 2nd ed, 391–411. Amsterdam: Elsevier.
- Shrestha, S., X. Fonoll, S. K. Khanal, and L. Raskin. 2017. “Biological Strategies for Enhanced Hydrolysis of Lignocellulosic Biomass During Anaerobic Digestion: Current Status and Future Perspectives.” Bioresource Technology 245: 1245–1257. doi:10.1016/j.biortech.2017.08.089.
- Sieborg, M. U., B. D. Jønson, S. U. Larsen, A. H. Vazifehkhoran, and J. M. Triolo. 2020. “Co-ensiling of Wheat Straw as an Alternative Pre-Treatment to Chemical, Hydrothermal and Mechanical Methods for Methane Production.” Energies 13 (4047): 1–19. doi:10.3390/en13164047.
- Sindhu, R., P. Binod, and A. Pandey. 2016. “Biological Pretreatment of Lignocellulosic Biomass – An Overview.” Bioresource Technology 199: 76–82. doi:10.1016/j.biortech.2015.08.030.
- Sitorus, B., and S. D. Panjaitan. 2013. “Biogas Recovery from Anaerobic Digestion Process of Mixed Fruit-Vegetable Wastes.” Energy Procedia 32: 176–182. doi:10.1016/j.egypro.2013.05.023.
- Speece, R. E. 2008. Anaerobic Biotechnology and Odor/Corrosion Control for Municipalities and Industries. Nashville: Archae Press.
- Sperandio, G. B., and E. X. Ferreira Filho. 2019. “Fungal Co-Cultures in the Lignocellulosic Biorefinery Context: A Review.” International Biodeterioration & Biodegradation 142: 109–123. doi:10.1016/j.ibiod.2019.05.014.
- Srasri, S., N. Bhudsarakam, P. Limsutthiphong, T. Ratanapitag, and A. Julsereewong. 2022. “Design of Step Grate Firing by Utilizing Palm Empty-Fruit-Bunch Fuel for Industrial Steam Boiler Construction.” Energy Reports 8: 275–282. doi:10.1016/j.egyr.2021.11.142.
- Stamatelatou, K., G. Antonopoulou, and P. Michailides. 2014. “15 - Biomethane and Biohydrogen Production via Anaerobic Digestion/Fermentation.” In Advances in Biorefineries: Biomass and Waste Supply Chain Exploitation,, edited by K. Waldron, 476–524. London: Woodhead Publishing.
- Stanley, J. T., A. Thanarasu, P. S. Kumar, K. Periyasamy, S. Raghunandhakumar, P. Periyaraman, K. Devaraj, A. Dhanasekaran, and S. Subramanian. 2022. “Potential Pre-Treatment of Lignocellulosic Biomass for the Enhancement of Biomethane Production Through Anaerobic Digestion- A Review.” Fuel 318: 1–19. doi:10.1016/j.fuel.2022.123593.
- Statistica Research Department. 2021. Production Volume of Palm Oil in Indonesia 2012-2020.
- Sudirman, L. I., A. Sutrisna, S. Listiyowati, L. Fadli, and B. Tarigan. 2011. The Potency of oil Palm Plantation Wastes for Mushroom Production. Proceedings of the 7th International Conference on Mushroom Biology and Mushroom products. Citeseer, 383–389.
- Sudiyani, Y., and E. Hermiati. 2010. “Utilization of oil Palm Empty Fruit Bunch (OPEFB) for Bioethanol Production Through Alkali and Dilute Acid Pretreatment and Simultaneous Saccharification and Fermentation.” Indonesian Journal of Chemistry 10 (2): 261–267. doi:10.22146/ijc.21471.
- Sudiyani, Y., D. Styarini, E. Triwahyuni, Sudiyarmanto, K. C. Sembiring, Y. Aristiawan, H. Abimanyu, and M. H. Han. 2013. “Utilization of Biomass Waste Empty Fruit Bunch Fiber of Palm oil for Bioethanol Production Using Pilot–Scale Unit.” Energy Procedia 32: 31–38. doi:10.1016/j.egypro.2013.05.005.
- Suhartini, S., S. Heaven, and C. J. Banks. 2014. “Comparison of Mesophilic and Thermophilic Anaerobic Digestion of Sugar Beet Pulp: Performance, Dewaterability and Foam Control.” Bioresource Technology 152: 202–211. doi:10.1016/j.biortech.2013.11.010.
- Suhartini, S., N. Hidayat, and I. Nurika. 2020. “Evaluation of Biogas Potential from Empty Fruit oil Palm Bunches.” IOP Conference Series: Earth and Environmental Science 443 (012013): 1–10. doi:10.1088/1755-1315/443/1/012013.
- Suhartini, S., S. H. Indah, F. A. Rahman, N. A. Rohma, N. L. Rahmah, I. Nurika, N. Hidayat, and L. Melville. 2022a. “Enhancing Anaerobic Digestion of Wild Seaweed Gracilaria verrucosa by Co-Digestion with Tofu Dregs and Washing Pre-Treatment.” Biomass Conversion and Biorefinery, 1–23. doi:10.1007/s13399-022-02507-z.
- Suhartini, S., Y. P. Lestari, and I. Nurika. 2019. “Estimation of Methane and Electricity Potential from Canteen Food Waste.” IOP Conference Series: Earth and Environmental Science 230: 1–6.
- Suhartini, S., I. Nurika, R. Paul, and L. Melville. 2021. “Estimation of Biogas Production and the Emission Savings from Anaerobic Digestion of Fruit-Based Agro-Industrial Waste and Agricultural Crops Residues.” BioEnergy Research 14 (3): 844–859. doi:10.1007/s12155-020-10209-5.
- Suhartini, S., N. A. Rohma, Elviliana, I. Santoso, R. Paul, P. Listiningrum, and L. Melville. 2022b. “Food Waste to Bioenergy: Current Status and Role in Future Circular Economies in Indonesia.” Energy, Ecology and Environment 7 (4): 297–339. doi:10.1007/s40974-022-00248-3.
- Suhartini, S., N. A. Rohma, E. Mardawati, Kasbawati, N. Hidayat, and L. Melville. 2022c. “Biorefining of oil Palm Empty Fruit Bunches for Bioethanol and Xylitol Production in Indonesia: A Review.” Renewable and Sustainable Energy Reviews 154: 1–25. doi:10.1016/j.rser.2021.111817.
- Sukiran, M. A., F. Abnisa, W. M. A. Wan Daud, N. Abu Bakar, and S. K. Loh. 2017. “A Review of Torrefaction of oil Palm Solid Wastes for Biofuel Production.” Energy Conversion and Management 149: 101–120. doi:10.1016/j.enconman.2017.07.011.
- Suksong, W., A. Jehlee, A. Singkhala, P. Kongjan, P. Prasertsan, T. Imai, and S. O-Thong. 2017a. “Thermophilic Solid-State Anaerobic Digestion of Solid Waste Residues from Palm oil Mill Industry for Biogas Production.” Industrial Crops and Products 95: 502–511. doi:10.1016/j.indcrop.2016.11.002.
- Suksong, W., P. Kongjan, P. Prasertsan, T. Imai, and S. O-Thong. 2016. “Optimization and Microbial Community Analysis for Production of Biogas from Solid Waste Residues of Palm oil Mill Industry by Solid-State Anaerobic Digestion.” Bioresource Technology 214: 166–174. doi:10.1016/j.biortech.2016.04.077.
- Suksong, W., P. Kongjan, P. Prasertsan, and S. O-Thong. 2019a. “Thermotolerant Cellulolytic Clostridiaceae and Lachnospiraceae Rich Consortium Enhanced Biogas Production from oil Palm Empty Fruit Bunches by Solid-State Anaerobic Digestion.” Bioresource Technology 291: 1–12. doi:10.1016/j.biortech.2019.121851.
- Suksong, W., C. Mamimin, P. Prasertsan, P. Kongjan, and S. O-Thong. 2019b. “Effect of Inoculum Types and Microbial Community on Thermophilic and Mesophilic Solid-State Anaerobic Digestion of Empty Fruit Bunches for Biogas Production.” Industrial Crops and Products 133: 193–202. doi:10.1016/j.indcrop.2019.03.005.
- Suksong, W., K. Promnuan, J. Seengenyoung, and S. O-Thong. 2017b. “Anaerobic co-Digestion of Palm oil Mill Waste Residues with Sewage Sludge for Biogas Production.” Energy Procedia 138: 789–794. doi:10.1016/j.egypro.2017.10.068.
- Suksong, W., W. Tukanghan, K. Promnuan, P. Kongjan, A. Reungsang, H. Insam, and S. O-Thong. 2020a. “Biogas Production from Palm oil Mill Effluent and Empty Fruit Bunches by Coupled Liquid and Solid-State Anaerobic Digestion.” Bioresource Technology 296: 122304. doi:10.1016/j.biortech.2019.122304.
- Suksong, W., N. Wongfaed, B. Sangsri, P. Kongjan, P. Prasertsan, S. M. Podmirseg, H. Insam, and S. O-Thong. 2020b. “Enhanced Solid-State Biomethanisation of oil Palm Empty Fruit Bunches Following Fungal Pretreatment.” Industrial Crops and Products 145: 1–9. doi:10.1016/j.indcrop.2020.112099.
- Sulfahri, Mushlihah, S., D. R. Husain, A. Langford, and A. C. M. A. R. Tassakka. 2020. “Fungal Pretreatment as a Sustainable and low Cost Option for Bioethanol Production from Marine Algae.” Journal of Cleaner Production 265: 1–8. doi:10.1016/j.jclepro.2020.121763.
- Suryawanshi, P. C., A. B. Chaudhari, and R. M. Kothari. 2010. “Thermophilic Anaerobic Digestion: The Best Option for Waste Treatment.” Critical Reviews in Biotechnology 30 (1): 31–40. doi:10.3109/07388550903330505.
- Syaichurrozi, I., R. Rusdi, T. Hidayat, and A. Bustomi. 2016. “Kinetics Studies Impact of Initial pH and Addition of Yeast Saccharomyces Cerevisiae on Biogas Production from Tofu Wastewater in Indonesia.” International Journal of Engineering 29 (8): 1037–1046. doi:10.5829/idosi.ije.2016.29.08b.02.
- Taherzadeh, M. J., and K. Karimi. 2008. “Pretreatment of Lignocellulosic Wastes to Improve Ethanol and Biogas Production: A Review.” International Journal of Molecular Sciences 9 (9): 1621–1651. doi:10.3390/ijms9091621.
- Tanjore, D., T. L. Richard, and M. N. Marshall. 2012. “Experimental Methods for Laboratory-Scale Ensilage of Lignocellulosic Biomass.” Biomass and Bioenergy 47: 125–133. doi:10.1016/j.biombioe.2012.09.050.
- Taricska, J. R., D. A. Long, J. P. Chen, Y. T. Hung, and S. W. Zou. 2009. “. Anaerobic Digestion.” In Biological Treatment Processes, Handbook of Environmental Engineering, 8th ed, 589–634, edited by L. K. Wang, N. C. Pereira, and Y. T. Hung. New York: Humana Press.
- Teh, C., G. Goh, and K. Kamarudin. 2010. “Physical Changes to oil Palm Empty Fruit Bunches (EFB) and EFB mat (Ecomat) During Their Decomposition in the Field.” Pertanika Journal of Tropical Agricultural Science 33 (1): 39–44.
- Temu, S. G., A. P. Moshi, I. A. Nges, A. M. Mshandete, A. K. Kivaisi, and B. Mattiasson. 2016. “Mixed Palm oil Waste Utilization Through Integrated Mushroom and Biogas Production.” British Biotechnology Journal 11 (4): 1–12. doi:10.9734/BBJ/2016/23385.
- Teng, S., K. W. Khong, and N. Che Ha. 2020. “Palm oil and its Environmental Impacts: A big Data Analytics Study.” Journal of Cleaner Production 274: 1–13. doi:10.1016/j.jclepro.2020.122901.
- Tepsour, M., N. Usmanbaha, T. Rattanaya, R. Jariyaboon, S. O-Thong, P. Prasertsan, and P. Kongjan. 2019. “Biogas Production from oil Palm Empty Fruit Bunches and Palm oil Decanter Cake Using Solid-State Anaerobic co-Digestion.” Energies 12 (22): 1–14. doi:10.3390/en12224368.
- Tian, X. F., Z. Fang, and F. Guo. 2012. “Impact and Prospective of Fungal pre-Treatment of Lignocellulosic Biomass for Enzymatic Hydrolysis.” Biofuels, Bioproducts and Biorefining 6 (3): 335–350. doi:10.1002/bbb.346.
- Triwahyuni, E., H. Abimayu, A. Cahyono, E. T. Cahyono, and Y. Sudiyani. 2015. “Alkaline Delignification of oil Palm Empty Fruit Bunch Using Black Liquor from Pretreatment.” Procedia Chemistry 16: 99–105. doi:10.1016/j.proche.2015.12.032.
- Triyono, S., A. Haryanto, M. Telaumbanua, J. Lumbanraja, and F. To. 2019. “Cultivation of Straw Mushroom (Volvariella volvacea) on oil Palm Empty Fruit Bunch Growth Medium.” International Journal of Recycling of Organic Waste in Agriculture 8: 381–392. doi:10.1007/s40093-019-0259-5.
- Usack, J. G., W. Wiratni, and L. T. Angenent. 2014. “Improved Design of Anaerobic Digesters for Household Biogas Production in Indonesia: One cow, one Digester, and one Hour of Cooking per day.” The Scientific World Journal 2014: 1–9. doi:10.1155/2014/318054.
- van Beek, T. A., B. Kuster, F. W. Claassen, T. Tienvieri, F. Bertaud, G. Lenon, M. Petit-Conil, and R. Sierra-Alvarez. 2007. “Fungal Bio-Treatment of Spruce Wood with Trametes versicolor for Pitch Control: Influence on Extractive Contents, Pulping Process Parameters, Paper Quality and Effluent Toxicity.” Bioresource Technology 98 (2): 302–311. doi:10.1016/j.biortech.2006.01.008.
- Van, D. P., T. Fujiwara, B. L. Tho, P. P. S. Toan, and G. H. Minh. 2020. “A Review of Anaerobic Digestion Systems for Biodegradable Waste: Configurations, Operating Parameters, and Current Trends.” Environmental Engineering Research 25 (1): 1–17. doi:10.4491/eer.2018.334.
- van Heerden, A., N. J. le Roux, J. Swart, T. Rypstra, S. Gardner-Lubbe, and A. Botha. 2011. “Chemical Alterations Induced by Pycnoporus sanguineus/Aspergillus flavipes Co-Cultures in Wood from Different Tree Species.” Wood Science and Technology 45 (2): 237–248. doi:10.1007/s00226-010-0318-6.
- Vasco-Correa, J., and A. Shah. 2019. “Techno-Economic Bottlenecks of the Fungal Pretreatment of Lignocellulosic Biomass.” Fermentation 5 (2): 1–23. doi:10.3390/fermentation5020030.
- Vaskan, P., E. R. Pachón, and E. Gnansounou. 2018. “Techno-Economic and Life-Cycle Assessments of Biorefineries Based on Palm Empty Fruit Bunches in Brazil.” Journal of Cleaner Production 172: 3655–3668. doi:10.1016/j.jclepro.2017.07.218.
- Vervaeren, H., K. Hostyn, G. Ghekiere, and B. Willems. 2010. “Biological Ensilage Additives as Pretreatment for Maize to Increase the Biogas Production.” Renewable Energy 35 (9): 2089–2093. doi:10.1016/j.renene.2010.02.010.
- Villa, R., L. Ortega Rodriguez, C. Fenech, and O. C. Anika. 2020. “Ensiling for Anaerobic Digestion: A Review of key Considerations to Maximise Methane Yields.” Renewable and Sustainable Energy Reviews 134: 110401. doi:10.1016/j.rser.2020.110401.
- Wagle, A., M. J. Angove, A. Mahara, A. Wagle, B. Mainali, M. Martins, R. Goldbeck, and S. Raj Paudel. 2022. “Multi-Stage Pre-Treatment of Lignocellulosic Biomass for Multi-Product Biorefinery: A Review.” Sustainable Energy Technologies and Assessments 49: 1–20. doi:10.1016/j.seta.2021.101702.
- Walter, A., I. H. Franke-Whittle, A. O. Wagner, and H. Insam. 2015. “Methane Yields and Methanogenic Community Changes During Co-Fermentation of Cattle Slurry with Empty Fruit Bunches of oil Palm.” Bioresource Technology 175: 619–623. doi:10.1016/j.biortech.2014.10.085.
- Waluyo, J., R. I. F. Anggraini, H. H. Kurniawan, and Y. Sudiyani. 2018. Physical Chemical Characterization of Alkali Pretreatment for oil Palm Empty Fruit Bunch, 020072. New York: AIP Publishing.
- Wan, C., and Y. Li. 2011. “Effectiveness of Microbial Pretreatment by Ceriporiopsis subvermispora on Different Biomass Feedstocks.” Bioresource Technology 102 (16): 7507–7512. doi:10.1016/j.biortech.2011.05.026.
- Wan, C., and Y. Li. 2012. “Fungal Pretreatment of Lignocellulosic Biomass.” Biotechnology Advances 30 (6): 1447–1457. doi:10.1016/j.biotechadv.2012.03.003.
- Wan Mahari, W. A., W. Peng, W. L. Nam, H. Yang, X. Y. Lee, Y. K. Lee, R. K. Liew, et al. 2020. “A Review on Valorization of Oyster Mushroom and Waste Generated in the Mushroom Cultivation Industry.” Journal of Hazardous Materials 400: 123156. doi:10.1016/j.jhazmat.2020.123156.
- Weide, T., C. D. Baquero, M. Schomaker, E. Brügging, and C. Wetter. 2020. “Effects of Enzyme Addition on Biogas and Methane Yields in the Batch Anaerobic Digestion of Agricultural Waste (Silage, Straw, and Animal Manure).” Biomass and Bioenergy 132 (105442): 1–10. doi:10.1016/j.biombioe.2019.105442.
- Wu, P., L. Li, J. Jiang, Y. Sun, Z. Yuan, X. Feng, and Y. Guo. 2020. “Effects of Fermentative and Non-Fermentative Additives on Silage Quality and Anaerobic Digestion Performance of Pennisetum purpureum.” Bioresource Technology 297: 1–13. doi:10.1016/j.biortech.2019.122425.
- Xu, Z., P. Lei, R. Zhai, Z. Wen, and M. Jin. 2019. “Recent Advances in Lignin Valorization with Bacterial Cultures: Microorganisms, Metabolic Pathways, and bio-Products.” Biotechnology for Biofuels 12 (32): 1–19. doi:10.1186/s13068-019-1376-0.
- Yacob, S., Y. Shirai, M. A. Hassan, M. Wakisaka, and S. Subash. 2005. “Baseline Study of Methane Emission from Open Digesting Tank of Palm oil Mill Effluent.” Chemosphere 59: 1575–1781.
- Yan, L., Y. Gao, Y. Wang, Q. Liu, Z. Sun, B. Fu, X. Wen, Z. Cui, and W. Wang. 2012. “Diversity of a Mesophilic Lignocellulolytic Microbial Consortium Which is Useful for Enhancement of Biogas Production.” Bioresource Technology 111: 49–54. doi:10.1016/j.biortech.2012.01.173.
- Yang, B., and C. E. Wyman. 2008. “Pretreatment: The key to Unlocking low-Cost Cellulosic Ethanol.” Biofpr- Biofuels, Bioproducts and Biorefining 2 (1): 26–40. doi:10.1002/bbb.49.
- Yetri, Y., A. T. Hoang, Mursida, Muldarisnur, E. Taer, and M. Q. Chau. 2020. “Synthesis of Activated Carbon Monolith Derived from Cocoa Pods for Supercapacitor Electrodes Application.” Energy Sources, Part A: Recovery, Utilization, and Environmental Effects, 1–15. doi:10.1080/15567036.2020.1811433.
- Yoo, H. M., S. W. Park, Y. C. Seo, and K. H. Kim. 2019. “Applicability Assessment of Empty Fruit Bunches from Palm oil Mills for use as Bio-Solid Refuse Fuels.” Journal of Environmental Management 234: 1–7.
- Yunqin, L., W. Dehan, and W. Lishang. 2010. “Biological Pretreatment Enhances Biogas Production in the Anaerobic Digestion of Pulp and Paper Sludge.” Waste Management & Research 28 (9): 800–810. doi:10.1177/0734242X09358734.
- Zabaniotou, A., and P. Kamaterou. 2019. “Food Waste Valorization Advocating Circular Bioeconomy - A Critical Review of Potentialities and Perspectives of Spent Coffee Grounds Biorefinery.” Journal of Cleaner Production 211: 1553–1566. doi:10.1016/j.jclepro.2018.11.230.
- Zadrazil, F. 1997. “Changes in in Vitro Digestibility of Wheat Straw During Fungal Growth and After Harvest of Oyster Mushrooms (Pleurotus spp.) on Laboratory and Industrial Scale.” Journal of Applied Animal Research 11 (1): 37–48. doi:10.1080/09712119.1997.9706159.
- Zhang, R., H. M. El-Mashad, K. Hartman, F. Wang, G. Liu, C. Choate, and P. Gamble. 2007. “Characterization of Food Waste as Feedstock for Anaerobic Digestion.” Bioresource Technology 98 (4): 929–935. doi:10.1016/j.biortech.2006.02.039.
- Zhang, Q., J. He, M. Tian, Z. Mao, L. Tang, J. Zhang, and H. Zhang. 2011. “Enhancement of Methane Production from Cassava Residues by Biological Pretreatment Using a Constructed Microbial Consortium.” Bioresource Technology 102 (19): 8899–8906. doi:10.1016/j.biortech.2011.06.061.
- Zhang, Q., J. Hu, and D. J. Lee. 2016. “Biogas from Anaerobic Digestion Processes: Research Updates.” Renewable Energy 98: 108–119. doi:10.1016/j.renene.2016.02.029.
- Zhang, H., Z. Ning, H. Khalid, R. Zhang, G. Liu, and C. Chen. 2018. “Enhancement of Methane Production from Cotton Stalk Using Different Pretreatment Techniques.” Scientific Reports 8 (3463): 1–9. doi:10.1038/s41598-018-21413-x.
- Zhang, W., X. Wang, W. Xing, R. Li, T. Yang, N. Yao, and D. Lv. 2021. “Links Between Synergistic Effects and Microbial Community Characteristics of Anaerobic co-Digestion of Food Waste, Cattle Manure and Corn Straw.” Bioresource Technology 329: 1–10. doi:10.1016/j.biortech.2021.124919.
- Zhao, Y., J. Yu, J. Liu, H. Yang, L. Gao, X. Yuan, Z.-J. Cui, and X. Wang. 2016. “Material and Microbial Changes During Corn Stalk Silage and Their Effects on Methane Fermentation.” Bioresource Technology 222: 89–99. doi:10.1016/j.biortech.2016.09.113.
- Zheng, Y., J. Zhao, F. Xu, and Y. Li. 2014. “Pretreatment of Lignocellulosic Biomass for Enhanced Biogas Production.” Progress in Energy and Combustion Science 42: 35–53. doi:10.1016/j.pecs.2014.01.001.
- Zhong, C., C. Wang, F. Wang, H. Jia, P. Wei, and Y. Zhao. 2016. “Enhanced Biogas Production from Wheat Straw with the Application of Synergistic Microbial Consortium Pretreatment.” RSC Advances 6 (65): 60187–60195. doi:10.1039/C5RA27393E.
- Zhong, W., Z. Zhang, Y. Luo, S. Sun, W. Qiao, and M. Xiao. 2011. “Effect of Biological Pretreatments in Enhancing Corn Straw Biogas Production.” Bioresource Technology 102 (24): 11177–11182. doi:10.1016/j.biortech.2011.09.077.
- Ziemiński, K., and M. Kowalska-Wentel. 2015. “Effect of Enzymatic Pretreatment on Anaerobic Co-Digestion of Sugar Beet Pulp Silage and Vinasse.” Bioresource Technology 180: 274–280. doi:10.1016/j.biortech.2014.12.035.
- Ziemiński, K., I. Romanowska, and M. Kowalska. 2012. “Enzymatic Pretreatment of Lignocellulosic Wastes to Improve Biogas Production.” Waste Management 32 (6): 1131–1137. doi:10.1016/j.wasman.2012.01.016.
- Zou, Y., F. Du, Q. Hu, X. Yuan, D. Dai, and M. Zhu. 2020. “Integration of Pleurotus tuoliensis Cultivation and Biogas Production for Utilization of Lignocellulosic Biomass as Well as its Benefit Evaluation.” Bioresource Technology 317: 124042. doi:10.1016/j.biortech.2020.124042.
- Zuhri, M., S. Sapuan, and N. Ismail. 2009. “Oil Palm Fibre Reinforced Polymer Composites: A Review.” Progress in Rubber Plastics and Recycling Technology 25 (4): 233–246. doi:10.1177/147776060902500403.