ABSTRACT
Introduction
Carbapenem-resistant (CR) Pseudomonas aeruginosa infections constitute a serious clinical threat globally. Patients are often critically ill and/or immunocompromised. Antibiotic options are limited and are currently centered on beta-lactam–beta-lactamase inhibitor (BL–BLI) combinations and the siderophore cephalosporin cefiderocol.
Areas covered
This article reviews the mechanisms of P. aeruginosa resistance and their potential impact on the activity of current treatment options, along with evidence for the clinical efficacy of BL–BLI combinations in P. aeruginosa infections, some of which specifically target infections due to CR organisms. The preclinical and clinical evidence supporting cefiderocol as a treatment option for P. aeruginosa involving infections is also reviewed.
Expert opinion
Cefiderocol is active against most known P. aeruginosa mechanisms mediating carbapenem resistance. It is stable against different serine- and metallo-beta-lactamases, and, due to its iron channel-dependent uptake mechanism, is not impacted by porin channel loss. Furthermore, the periplasmic level of cefiderocol is not affected by upregulated efflux pumps. The potential for on-treatment resistance development currently appears to be low, although more clinical data are required. Information from surveillance programs, real-world compassionate use, and clinical studies demonstrate that cefiderocol is an important treatment option for CR P. aeruginosa infections.
1. Introduction
Antibiotic-resistant Pseudomonas aeruginosa presents a challenging clinical scenario. P. aeruginosa is able to readily develop resistance to a number of commonly used antibiotics [Citation1,Citation2], including first-line antipseudomonal agents [Citation1,Citation3,Citation4]. As such, P. aeruginosa is one of the most commonly isolated carbapenem-resistant (CR) Gram-negative bacteria encountered in the hospital [Citation5]. Isolates that are multidrug-resistant (MDR), extensively drug-resistant (XDR) [Citation1,Citation5–8] and/or with difficult-to-treat resistance (DTR; exhibiting non-susceptibility to piperacillin–tazobactam, ceftazidime, cefepime, aztreonam, meropenem, imipenem-cilastatin, ciprofloxacin, and levofloxacin) [Citation9] have become a fairly common clinical occurrence. The extent of the problem has been recognized by both the US Centers for Disease Control and Prevention, which considers MDR/CR P. aeruginosa to be a serious threat [Citation10], and the World Health Organization, which listed CR P. aeruginosa among the critical pathogens urgently requiring new antibiotics [Citation11].
The implications of resistance are particularly important given the patient population involved [Citation12,Citation13]. P. aeruginosa infections are frequent in immunocompromised patients, such as those with cancer, and hospitalized patients [Citation10]; therefore, appropriate and timely treatment are essential for avoiding excess morbidity and mortality. Of note, risk factors related to infection severity, especially intensive care unit (ICU) admission, intubation, or invasive devices, are often linked to risk of acquiring MDR P. aeruginosa [Citation2,Citation3,Citation10,Citation14]. Prior carbapenem treatment has also been shown to be a risk factor for the development of CR P. aeruginosa infections [Citation15]. The most frequent infections caused by CR or MDR P. aeruginosa are respiratory tract infections (RTI), nosocomial pneumonia (NP), complicated urinary tract infection (cUTI), and bloodstream infection (BSI) [Citation16–18]. All these infection types caused by CR P. aeruginosa are associated with an approximate 10–35% all-cause mortality rates [Citation16,Citation18,Citation19] as well as with high rates of treatment failure and recurrence [Citation17,Citation18,Citation20].
Despite recent approvals of new antibiotics, there remain relatively few active treatment options for CR P. aeruginosa infections (). Choices center on the beta-lactam–beta-lactamase inhibitor (BL–BLI) combinations, such as ceftolozane-tazobactam (C/T), ceftazidime-avibactam (CZA), and imipenem-relebactam (I/R), and the siderophore cephalosporin, cefiderocol. Meropenem–vaborbactam (MVB) may also have a somewhat limited role, with evidence for activity against some strains of MDR/CR P. aeruginosa [Citation21–24]; however, MVB, unlike the other new approved antibiotics, is not included as an option for DTR P. aeruginosa in guidelines from the Infectious Diseases Society of America [Citation9].
Figure 1. Antipseudomonal cephalosporins and their characteristics.
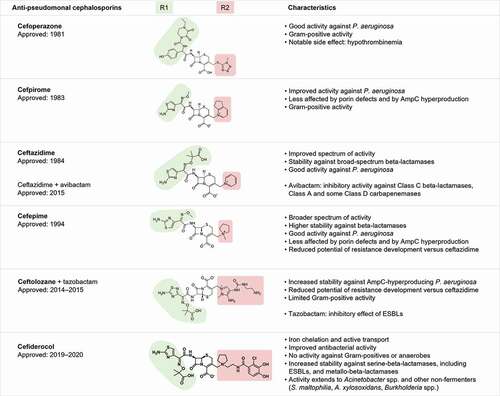
While current data show generally high susceptibility rates of CR P. aeruginosa to newer antibiotics, there is considerable geographical variation () [Citation6,Citation7,Citation25–52]. In addition, cross-resistance between C/T and CZA has been noted for carbapenem-non-susceptible strains [Citation28,Citation34,Citation42]. Furthermore, host-dependent risk factors are an important consideration in guiding susceptibility testing, as there have been reports of variable susceptibility rates to C/T and CZA of meropenem-non-susceptible P. aeruginosa isolated from patients with cystic fibrosis (CF) [Citation53].
Table 1. Summary of in vitro susceptibility of ceftazidime–avibactam, ceftolozane–tazobactam, cefiderocol, imipenem–relebactam, and meropenem–vaborbactam against carbapenem-resistant Pseudomonas aeruginosa in different regions’ surveillance programs
2. Mechanisms of resistance in carbapenem-resistant P. aeruginosa with impact on susceptibility to beta-lactam–beta-lactamase inhibitor combinations and cefiderocol
Both intrinsic and acquired (through horizontal gene transfer or mutations) antibiotic resistance mechanisms () described in P. aeruginosa present a challenge for the selection of antibiotic treatment [Citation3,Citation54–70]. Restriction of the outer membrane permeability is a key factor in intrinsic resistance [Citation54,Citation71]. Constitutive efflux pumps (e.g. MexAB-OprM) contribute to resistance to meropenem and several other classes of antibiotics, including fluoroquinolones and aminoglycosides [Citation54]. As described below, mutation-associated loss or decreased functionality of the key outer membrane porin channel protein OprD [Citation72,Citation73] and overproduction of AmpC, a chromosomal cephalosporinase (or Pseudomonas-derived cephalosporinase [PDC] in P. aeruginosa) [Citation73], can work in concert with the efflux pumps to cause further antibiotic resistance.
Table 2. Mechanisms of resistance contributing to reduced activity or resistance to beta-lactam–beta-lactamase inhibitors and cefiderocol in carbapenem-resistant Pseudomonas aeruginosa.
OprD provides entry to P. aeruginosa isolates for several antibiotic classes, including imipenem and meropenem [Citation54,Citation71] but not ertapenem. While oprD mutation in the absence of other resistance mechanisms does not affect the activity of C/T, CZA, and I/R, loss of permeability leads to reduced susceptibility for MVB in CR isolates, although data are scarce [Citation57,Citation70] (). Porin mutation also restricts imipenem entry into P. aeruginosa, leading to resistance. However, the combination of imipenem with relebactam has been shown to reduce imipenem minimum inhibitory concentrations (MICs) to ≤1 μg/mL in isolates with AmpC hyperproduction, OprD inactivation, and/or efflux pump overexpression [Citation55,Citation67]. Further studies also have shown that relebactam reduced imipenem MICs in isolates producing extended-spectrum beta-lactamases (ESBLs), including Vietnamese extended-spectrum beta-lactamase (VEB), Pseudomonas extended resistance (PER) ESBL, and Guiana extended-spectrum beta-lactamase (GES), but only to levels that remained largely above the susceptibility breakpoint [Citation59]. Due to the distinctive mechanism used by cefiderocol to gain entry into bacterial cells, an action mediated via iron-transport channels, neither porin loss nor upregulated efflux pump have been found to impact on P. aeruginosa susceptibility to this antibiotic [Citation74,Citation75].
Chromosomal constitutive (low expression) AmpC can be induced by a number of antibiotics, including aminopenicillins, some cephalosporins, and carbapenems. In the presence of other resistance mechanisms, AmpC (or PDC) induction and de-repression may also result in resistance to beta-lactam antibiotics other than C/T, CZA, and carbapenems. C/T and CZA are stable against induced or de-repressed AmpC by virtue of ceftolozane and avibactam, respectively (). During treatment, however, both C/T and CZA can induce resistance through emerging mutations in AmpC/PDC, with the result that CR P. aeruginosa may become cross-resistant to other BL–BLI combinations [Citation64,Citation76,Citation77]. A recent study demonstrated that specific mutations in the AmpC enzyme omega loop can lead to resistance to C/T and CZA, and slightly reduced susceptibility to cefiderocol in the absence of cefiderocol exposure, and increased susceptibility to I/R, a phenomenon known as ‘collateral sensitivity’ [Citation64]. In the study, in contrast to what was observed with C/T and CZA, cefiderocol MIC values rarely changed to non-susceptibility (i.e. >4 µg/mL) [Citation64].
Another form of acquired carbapenem resistance in P. aeruginosa involves the horizontal transfer of genes encoding beta-lactamases, including carbapenemases [Citation14,Citation54]. The relative expression by CR P. aeruginosa of the horizontally acquired ESBLs and carbapenemases shows regional variation [Citation1]. For example, in Colombia, Klebsiella pneumoniae carbapenemase (KPC)-producing isolates occur as frequently as Verona integron-encoded metallo-beta-lactamase (VIM) producers [Citation78]. In Asia, imipenemase-1 carbapenemase (IMP-1) was the most common metallo-beta-lactamase (MBL) in Japan [Citation79], while in India, both VIM and New Delhi MBL (NDM) are frequent carbapenemases in CR P. aeruginosa [Citation80]. In addition, constitutive expression of horizontally transferred genes for non-inducible AmpC enzymes (e.g. FOX [cefoxitin-hydrolyzing class C beta-lactamase] or CMY [cephamycinase]) increases the baseline rate of AmpC-mediated hydrolysis [Citation55].
The use of beta-lactamase inhibitors to restore beta-lactam susceptibility can be impeded by additional P. aeruginosa resistance mechanisms. Relebactam, an irreversible inhibitor of AmpC [Citation55], is not removed by upregulated efflux pumps and so can restore susceptibility to imipenem in AmpC hyperproducers and in those with certain class A enzymes (e.g. KPC). However, susceptibility rates are variable and depend on the expression and/or type of class A enzyme [Citation55,Citation59]. For example, expression by CR P. aeruginosa of either an ESBL or a carbapenemase variant of the class A GES enzyme can reduce susceptibility to I/R [Citation55]. In addition, in vivo evolution of GES beta-lactamases can be driven by CZA treatment and may lead to a switch in the phenotype from carbapenem resistant to susceptible [Citation81]. Vaborbactam is an irreversible, non-beta-lactam, cyclic boronic acid-based inhibitor of serine-beta-lactamases, in particular class A and class C enzymes, mainly in Enterobacterales [Citation82]. Preclinical experiments and surveillance studies with MVB in P. aeruginosa yielded apparently conflicting results. While addition of vaborbactam led to no increase in the in vitro activity, the in vivo bactericidal effects of MVB against certain strains of P. aeruginosa were greater than those of meropenem alone [Citation83]. Based on evidence from Enterobacterales, the failure of vaborbactam to reduce meropenem MICs in P. aeruginosa may be the result of concomitant porin channel mutations, efflux pump upregulation, and/or production of class B carbapenemases (i.e. MBLs) [Citation57,Citation82,Citation84].
Carbapenem resistance in P. aeruginosa is commonly due to the production of MBLs, the prevalence of which varies by hospital and by region. Rates are concerningly high in Singapore [Citation34], India [Citation80,Citation85], Japan [Citation86], and Europe [Citation87,Citation88] but appear to be lower in the USA [Citation89]. MBL-mediated CR P. aeruginosa isolates are generally resistant to treatment with C/T, CZA, I/R, and MVB [Citation34,Citation82,Citation90,Citation91]. Cefiderocol is mostly stable against hydrolysis by MBLs [Citation62,Citation92].
The production of carbapenemases by CR P. aeruginosa, and the type of carbapenemases produced, have considerable therapeutic implications. Rapid diagnostic tests could help to identify patients harboring carbapenemase-producing isolates [Citation93] and so guide appropriate treatment. The need for testing should be guided by local epidemiology [Citation94]. An algorithm to predict P. aeruginosa-associated carbapenemase production has been developed, which incorporates non-susceptibility to carbapenems and cefepime, ceftazidime, and piperacillin-tazobactam (with or without non-susceptibility to C/T or CZA) [Citation95] and also phenotype testing with modified carbapenem inactivation method to confirm the presence of an active carbapenemase [Citation95]. Accurate detection of various enzymes in carbapenemase-positive CR P. aeruginosa has been successful by subsequent rapid diagnostic testing; however, an expanded spectrum of detectable enzymes was needed to uncover the true prevalence of carbapenemases in isolates collected from different regions [Citation94].
In addition, the development of biofilms by P. aeruginosa is an adaptive response mechanism that impedes antibiotic penetration into bacterial cells [Citation2,Citation54,Citation71,Citation96]. Through biofilm formation, P. aeruginosa undergoes phenotypic changes and may become less susceptible or resistant to antibiotics in patients with cystic fibrosis or other chronic lung infections, who therefore often require prolonged and repeated antibiotic treatment [Citation71]. In biofilms, the so-called rugose small colony variants of P. aeruginosa cells are responsible for the excessive production of extracellular matrix elements, which, by creating a thick layer, very efficiently protect these cells from antibiotics allowing them to adapt to the environment and to persist for several weeks [Citation97,Citation98].
3. Treatment of carbapenem-resistant P. aeruginosa infections
The presence of documented clinical risk factors for serious CR/MDR P. aeruginosa infections in hospitalized patients and a knowledge of local susceptibility data corresponding to mechanisms mediating carbapenem resistance and/or history of travel to endemic regions are critical for guiding selection of the most appropriate antibiotic therapy [Citation14,Citation80,Citation94]. Prior hospitalization, ICU stay, tracheal intubation, Acute Physiology and Chronic Health Evaluation II score, diabetes mellitus, and urinary catheter are all associated with MDR P. aeruginosa and/or an increased risk of mortality in MDR P. aeruginosa infections [Citation2,Citation14]. Infection- and treatment-associated risk factors for MDR P. aeruginosa include colonization, prior broad-spectrum antibiotic use, and prior carbapenem use [Citation2,Citation14]. Management decisions should also include consideration of a patient’s travel history, given that there is geographical diversity in the extent and type of carbapenemase production by P. aeruginosa [Citation94].
P. aeruginosa is also a primary causative pathogen of infections in patients with CF and leads to considerable morbidity and mortality [Citation71]. The duration of antibiotic treatment is based on the type of infection and activity of the initial empiric treatment [Citation9,Citation99].
Resistance to aminoglycosides and fluoroquinolones, once the mainstay of treatment and sometimes still active against CR P. aeruginosa, has led to the search for new approaches for the front-line setting. Antibiotic combinations may provide a solution, based on findings of synergy between some antibiotics in in vitro studies [Citation54]. However, this concept is not well established [Citation100–102], and clinical evidence supporting combination use is still scarce despite being widely used in real life [Citation101,Citation103]. It is reasonable to consider empiric antibiotic therapy, based on local susceptibility pattern, to increase the likelihood that at least one agent is active. The approved BL–BLI combinations, CZA, C/T, and I/R, have demonstrated effectiveness in P. aeruginosa infections, including CR forms, in the clinical setting () [Citation69,Citation70,Citation104–124]. These agents are recommended as monotherapy for DTR P. aeruginosa once their in vitro activity is confirmed [Citation9]. Because dosing recommendations for these antibiotics are available primarily for adult patients, including elderly patients, management of infections due to CR P. aeruginosa is still highly challenging for certain patient populations such as pediatric patients or during pregnancy.
Table 3. Comparison of recently approved antibiotics with in vitro activity against carbapenem–resistant Pseudomonas aeruginosa.
CZA was active against 72% of CR P. aeruginosa isolates from the ERACE-PA Global Surveillance Program (2019–2021), and against 91% of carbapenemase-negative isolates [Citation94]. In the INFORM Global Surveillance Program (2012–2015) involving isolates from Asia-Pacific countries, susceptibility of MBL-negative meropenem-non-susceptible and MDR P. aeruginosa isolates was 83.6% and 68.2%, respectively [Citation33]. The clinical efficacy of CZA has been demonstrated in cUTI, complicated intra-abdominal infection (cIAI), and NP in the multicenter, Phase III RECAPTURE [Citation106], REPRISE [Citation107], REPROVE [Citation108], and RECLAIM [Citation109] trials, including patients with P. aeruginosa infections, some with CR forms (). In a pooled analysis of the Phase III trial program, among 95 patients with ≥1 MDR P. aeruginosa isolate, the favorable microbiological response rate with CZA was 57.1% [Citation125].
For C/T, susceptibility rates in CR P. aeruginosa overall and carbapenemase-negative CR P. aeruginosa isolates were 63% and 88%, respectively, in the ERACE-PA Global Surveillance Program (2019–2021) [Citation94]. The multinational SIDERO surveillance program recently reported that the average susceptibility rate for C/T in P. aeruginosa isolates was 76%, reaching 90% among US isolates, thereby indicating a higher proportion of resistance in Europe [Citation42]. The Phase III ASPECT trials investigating C/T in cIAI, cUTI and NP included 5.3%, 3%, and 25% of patients, respectively, with P. aeruginosa infections [Citation113–115]; efficacy of C/T was similar to that of comparator agents. In a retrospective study of 21 patients with MDR P. aeruginosa infections (86% RTIs), the success rate of C/T was 71%, but resistance emerged in 3 patients (14%) [Citation116].
For I/R, data from the SMART Surveillance Europe database (2015–2017) reported that among P. aeruginosa isolates from IAI and UTI sources, 94.4% and 93.0%, respectively, were susceptible to I/R; the rate was 79.8% for MDR isolates from IAI and UTI combined [Citation51]. In the RESTORE-IMI 1 trial, 77% of imipenem-non-susceptible infections were due to P. aeruginosa [Citation120]; a favorable response against P. aeruginosa was observed in 13/16 patients (81%) receiving I/R. In the RESTORE-IMI 2 trial in hospital-acquired pneumonia/ventilator-associated pneumonia, 18.9% of 531 patients had P. aeruginosa as a baseline pathogen; 28-day all-cause mortality was 33.3% among a small number of patients with P. aeruginosa treated with I/R versus 12.0% among those who received piperacillin/tazobactam [Citation121].
For MVB, limited clinical data are available; none of the patients involved in the Phase III TANGO-I and TANGO-II trials in cUTI/pyelonephritis had P. aeruginosa infections at baseline [Citation122,Citation123]. In a real-world cohort of patients receiving MVB for a variety of Gram-negative infections, none of the eight patients in whom MVB was used to primarily target P. aeruginosa died by Day 30 [Citation124].
4. Cefiderocol for the treatment of carbapenem-resistant P. aeruginosa infections
4.1. In vitro activity
Cefiderocol is a siderophore cephalosporin (), a beta-lactam conjugated with a catechol group, and therefore acts as an iron chelator [Citation74]. It binds penicillin-binding proteins and inhibits cell wall synthesis in Gram-negative bacteria [Citation74]. Cefiderocol demonstrates bactericidal activity against Gram-negative bacteria but lacks activity against anaerobic bacteria and Gram-positive bacteria [Citation62,Citation74,Citation75].
Cefiderocol enters bacterial cells through iron-transport channels via an active uptake mechanism [Citation74,Citation75]. Bacterial iron uptake is facilitated by specific transport systems including siderophore receptors in the outer membrane, TonB-ExbBD energy transducing proteins, and inner membrane iron transport channels [Citation126]. Two types of natural siderophores, pyoverdines and pyochelin, are produced by P. aeruginosa that facilitate iron uptake through iron transporters [Citation127]. Cefiderocol exploits two different routes: PiuA and PiuD are utilized in the absence of pyochelin, and FptA in the presence of pyochelin [Citation128]. A deficiency of PiuA or overproduction of pyoverdine due to pvdS mutation has been shown to lead to an 8- to 16-fold increase in the cefiderocol MIC [Citation128,Citation129]. However, resistance has not been observed to emerge under human pharmacokinetic exposure in in vitro chemostat models and in vivo murine thigh infection models [Citation130].
Cefiderocol is able to counter all mechanisms of carbapenem resistance. Cefiderocol is a single molecule and not a BL–BLI fixed combination antibiotic [Citation74]. Its stability against hydrolysis by beta-lactamases, including carbapenemases, is intrinsic to the molecular structure and is linked to the various side chains, including the catechol moiety responsible for iron binding [Citation74,Citation131]. It is mostly stable against serine-beta-lactamases (classes A, C, and D) and against MBLs [Citation62,Citation63,Citation92]. An exception to this is class A PER or VEB ESBL, which can be expressed by P. aeruginosa [Citation65]. PER-producing P. aeruginosa has a higher cefiderocol MIC than P. aeruginosa strains expressing other types of beta-lactamases (e.g. MBL, AmpC) [Citation62,Citation65]. According to a UK study, at ≤4 μg/mL, 73.3% of 15 PER-producing isolates and 90% of 10 VEB-producing isolates were susceptible to cefiderocol [Citation65]. Cefiderocol also maintains activity against P. aeruginosa strains that overproduce AmpC [Citation63]. In addition, because of the iron-transport channel-related mode of bacterial cell entry used by cefiderocol, porin channel loss and/or efflux pump upregulation have minimal impact on its antibacterial activity [Citation62].
Cefiderocol has also been reported to reduce P. aeruginosa-associated biofilm formation and its mass, an effect that tends to show concentration and time dependency [Citation132]. This effect may be partially explained by increased synthesis of natural siderophores and possible upregulation of siderophore transporters by P. aeruginosa during biofilm formation in order to meet increased demand for iron [Citation132,Citation133]. However, further investigation is needed to confirm the activity of cefiderocol in suppressing the growth of small colony variant forms of P. aeruginosa.
Cefiderocol has demonstrated excellent in vitro activity against clinical isolates of P. aeruginosa in the SIDERO-WT and SIDERO-CR surveillance studies, including against DTR strains and strains resistant/non-susceptible to C/T, CZA, and I/R [Citation42,Citation134–138]. Activity has been shown against respiratory, bloodstream, and urinary tract infection isolates [Citation27,Citation139]. Cefiderocol has also demonstrated in vitro activity against MDR P. aeruginosa strains isolated from cancer patients (i.e. 97.5% were inhibited at ≤4 μg/mL) [Citation140] and from ICU patients (i.e. 100% were inhibited at ≤1 μg/mL) [Citation30], and against a pandrug-resistant P. aeruginosa clinical isolate producing MBLs [Citation141].
Susceptibility testing is conducted using either broth microdilution with iron-depleted cation-adjusted Mueller–Hinton broth or using 30 µg disks on standard Mueller–Hinton agar plates without the need to adjust iron concentrations in the agar; these methods are endorsed by both Clinical and Laboratory Standards Institute (CLSI) and European Committee on Antimicrobial Susceptibility Testing committees [Citation142]. Gradient diffusion strips, currently only validated for P. aeruginosa, are also commercially available. For disk diffusion and gradient diffusion testing, unlike broth microdilution, iron depletion of media is not required, presumably because iron is bound in the medium, mimicking an iron-depleted status.
4.2. Preclinical models
The in vivo effectiveness and pharmacodynamic target of cefiderocol have been investigated in several animal models of P. aeruginosa infection [Citation143–146]. In neutropenic murine models of P. aeruginosa thigh and lung infection, including CR strains, use of cefiderocol resulted in stasis with ~63% fraction of time over the MIC (fT>MIC) and a 1-log reduction with ~70–72% fT>MIC [Citation144]. The in vivo effectiveness was independent of carbapenemase production [Citation144]. In addition, among 20 P. aeruginosa strains with MICs of ≤4 µg/mL, 85% showed bacterial stasis or ≥1-log growth reduction compared with baseline inoculum in a murine thigh infection model using humanized drug exposure [Citation145]. The in vivo effectiveness was also shown against CR P. aeruginosa using humanized dosing of cefiderocol in a rat model of pneumonia [Citation143]. Notably, unlike the observations with previous investigational siderophore antibiotics (i.e. MB-1, SMC-3176), the in vivo efficacy of cefiderocol has been shown to correlate with its in vitro activity [Citation146].
4.3. Clinical data in carbapenem-resistant Pseudomonas infections
Cefiderocol currently has regulatory approval for the treatment of cUTI and NP in the USA [Citation147], and for infections caused by susceptible pathogens with limited treatment options in Europe [Citation148] (). Of note, cefiderocol dose adjustment is required for patients with moderate or severe renal impairment; and for patients with augmented renal clearance, the recommendation is to increase the frequency of the infusions (i.e. 2 g, once every 6 h) [Citation147,Citation148]. Approval for cUTI and NP was based on the Phase II APEKS-cUTI study [Citation149] and the Phase III APEKS-NP study, respectively [Citation150]. These two randomized, double-blind, non-inferiority studies enrolled patients with P. aeruginosa infections, which were primarily carbapenem-susceptible isolates, and only a low proportion were CR isolates. However, patients in both studies were at a high risk of being infected with MDR pathogens and dosing of the comparator agent was selected to address MDR Gram-negative pathogens [Citation149,Citation150].
In both studies, comparable clinical and microbiological outcomes were reported between treatment arms for P. aeruginosa infections [Citation149,Citation150]. In patients with P. aeruginosa infections in the APEKS-cUTI study, the rates for the composite of clinical and microbiological outcomes at test of cure (TOC) (which was the primary endpoint in the main population) were 46.7% (7/15) for cefiderocol and 50% (2/4) for imipenem-cilastatin (treatment difference: –3.33), and clinical cure at TOC was around 75% in both arms (cefiderocol: 73.3 [11/15], imipenem-cilastatin: 75.0% [3/4], treatment difference: –1.67) [Citation149]. Cefiderocol MIC90 was 0.25 µg/mL in both treatment arms. In the APEKS-NP study, Day 14 all-cause mortality rates (the primary endpoint in the main population) of 8% (2/24) and 13% (3/23; treatment difference: –4.7; 95% confidence interval [CI]: –22.4; 12.9) were reported with cefiderocol and high-dose, extended-infusion meropenem, respectively, in patients with NP caused by P. aeruginosa [Citation150]. At Day 28, the corresponding all-cause mortality rates were 8% (2/24) and 26% (6/23; treatment difference: –17.8; 95% CI: –38.8; 3.3), respectively. At TOC, the clinical cure rates were 67% (16/24) for cefiderocol and 71% (17/24) for meropenem (treatment difference: –4.2; 95% CI: –30.4; 22.0), and the microbiological eradication rates were 38% (9/24) and 46% (11/24; treatment difference: –8.3; 95% CI: –36.1; 19.5), respectively. Follow-up clinical cure rates were high for both cefiderocol (58%) and meropenem (54%). Cefiderocol MIC90 was 0.5 µg/mL at baseline [Citation150]. The strength of these two clinical studies is that cefiderocol treatment was administered as monotherapy; thus, efficacy of this new agent is not confounded by the activity of another antibiotic.
Probably the most relevant clinical trial data were generated in the CREDIBLE-CR study, which was conducted in patients with serious CR infections and formed the basis of the regulatory approval of cefiderocol for the treatment of patients with infections caused by susceptible pathogens with limited treatment options [Citation151]. The study enrolled patients with a variety of infections, including NP, BSI/sepsis, and cUTI. There was only a very limited number of general exclusion criteria, but diagnosis-specific inclusion and exclusion criteria were also applicable [Citation151]. The primary endpoint was clinical cure rate in patients with NP and BSI/sepsis, and microbiological eradication rate in patients with cUTI. In CR P. aeruginosa infections, the clinical cure rate at TOC was 58% in the cefiderocol arm and 50% in the best-available therapy (BAT) arm. The microbiological eradication rate against CR Pseudomonas was low (1/12 patients), and 7/12 of the isolates had indeterminate responses (mainly due to missing samples) [Citation151]. The cefiderocol MIC90 was 2 µg/mL in the cefiderocol arm, and the highest MIC was 4 µg/mL. A post-hoc analysis of the CREDIBLE-CR study reported that clinical cure at TOC was achieved in all four patients with CR P. aeruginosa isolates producing an MBL enzyme and in seven of 10 patients with OprD deficiency; however, the microbiological findings were confounded by the high indeterminate microbiological response rate (e.g. missing samples, additional antibiotics) [Citation151,Citation152]. All-cause mortality rates at the end of study in the safety population of CREDIBLE-CR were similar between treatment arms in primarily CR P. aeruginosa infections without Acinetobacter spp. co-infection (18% and 18%) and were increased with cefiderocol treatment in polymicrobial infections with Acinetobacter spp. (35% and 17%). Patients who had Acinetobacter spp. at baseline were sicker, older, more frequently in the ICU, and more frequently had prior/ongoing shock, all of which might have contributed to the increased mortality in the cefiderocol arm, including the patients with CR P. aeruginosa co-infections [Citation151].
Clinical evidence is also available for patients who received cefiderocol in a compassionate use setting (). Patients had a variety of DTR/CR P. aeruginosa infections, including aortic valve endocarditis, osteomyelitis, surgical site infection (wound infection), transplant patient (portal prosthesis), IAI, RTIs, BSI, esophageal-pleural fistula empyema, and meningitis, and had no alternative treatment options. Many of the infections were polymicrobial or patients were colonized with another pathogen () [Citation153–167]. Among 29 patients with P. aeruginosa isolates that had cefiderocol MICs up to 4 µg/mL or susceptibility confirmed by disk zone diameter, 24 receiving cefiderocol responded to treatment. Among these 24 responders, 14 patients received cefiderocol in combination therapy and 10 patients received monotherapy. Six patients had isolates with cefiderocol MICs ≥8 µg/mL, some of which belonged to the hypervirulent ST type ST357, and did not respond to treatment [Citation158,Citation161]; cefiderocol was discontinued in one patient due to non-susceptibility [Citation165]. In a series of 46 patients in the compassionate use program, which included a few patients with CF, clinical response to cefiderocol was seen in 67% of patients with cefiderocol MIC values of ≤4 µg/mL (CLSI susceptibility breakpoint) [Citation168]. Response to treatment was observed for >80% of patients with CR P. aeruginosa isolates with cefiderocol MIC >1 µg/mL (i.e. US Food and Drug Administration susceptibility breakpoint). The response rate was similar for the CR P. aeruginosa isolates with cefiderocol MIC 2–4 and ≥8 µg/mL [Citation168]. Among the 95 CR P. aeruginosa isolates, of which 77% were not susceptible to C/T and 69% were not susceptible to CZA, five isolates had cefiderocol MIC >4 µg/mL [Citation168]. These data highlight the cross-resistance between C/T and CZA and maintained cefiderocol susceptibility in a large proportion of CR P. aeruginosa, for which cefiderocol had been an effective choice for treatment [Citation168].
Table 4. Compassionate use of cefiderocol in extensively drug-resistant Pseudomonas aeruginosa infections
There are as yet few data detailing development by P. aeruginosa of resistance to cefiderocol. Among 41 P. aeruginosa clinical isolates derived from patients in the APEKS-NP (n = 24) and CREDIBLE-CR (n = 17) studies, three demonstrated a ≥4-fold increase in cefiderocol MIC with treatment [Citation169]. One of these isolates emerged as resistant and two remained susceptible according to CLSI susceptibility breakpoints [Citation169]. In a real-world clinical-use setting, cefiderocol resistance was reported in two patients following cefiderocol treatment [Citation158,Citation159].
5. Conclusions
Among the limited treatment options for CR P. aeruginosa, the most recently approved BL–BLI inhibitor combinations display a high rate of in vitro activity and favorable efficacy and safety profile in clinical studies; however, resistance to all agents has been reported. As a single agent without a beta-lactamase inhibitor, cefiderocol was developed to overcome all mechanisms of carbapenem resistance, including those that have been seen occurring in CR P. aeruginosa in surveillance studies. Thus, cefiderocol, with its potent activity, is a promising treatment choice against CR P. aeruginosa, with proven efficacy in clinical studies and case reports in patients with challenging infections, such as NP or BSI.
6. Expert opinion
Selection of an appropriate antibiotic for the treatment of CR P. aeruginosa from the limited treatment options is a challenging task for the physician, the microbiologist, or the pharmacist given the variety of resistance mechanisms. Risk factors for being infected by P. aeruginosa are well known [Citation14]; however, host factors, prior treatment failure, local antibiogram, travel history, diversity of carbapenem resistance mechanisms, and susceptibility are equally important factors to consider when CR strains are confirmed. Delayed and/or inappropriate antibiotic treatment for septic shock and/or ICU patients with CR infections increases the risk of mortality [Citation11,Citation170,Citation171]; every hour delay in receiving appropriate antibiotic treatment increases the patient’s mortality risk by 7.6% [Citation170]. Outcomes may depend on the infection site, too; UTIs require a shorter treatment period and are associated with a lower mortality risk, whereas BSI and NP carry a greater risk of mortality [Citation99,Citation108,Citation114,Citation115,Citation149–151].
The species and its antibiotic susceptibility (resistance) profile are not known when empiric antibiotic treatment is initiated, although for suspected P. aeruginosa infection, coverage with two antipseudomonal agents is reasonable when resistance risk according to epidemiological situation and patients’ characteristics is high. Many first-line agents can induce resistance even to some of the newer antibiotics, suggesting that careful consideration of available empiric antibiotics is required prior to treatment. Prevention of further resistance development is one of the primary objectives of combination therapy for P. aeruginosa infections [Citation101,Citation102]; however, only a limited number of agents from different classes have proved to act synergistically in vitro, hampering their use in combination. Current treatment trends, at least in Europe, show that polymyxin, either as monotherapy or in combination therapy, is frequently prescribed for CR P. aeruginosa infections [Citation101], despite limited data on clinical evidence supporting combination [Citation101]. Currently, there is no evidence that cefiderocol and other antibiotics have synergistic effects against CR P. aeruginosa because isolates with high cefiderocol MIC values are rare [Citation42,Citation137]. Despite lack of evidence, cefiderocol was administered in combination with other antimicrobials for many patients in the compassionate use program, who received cefiderocol as a last resort treatment because no alternative treatment option was available [Citation168].
Importantly, CR P. aeruginosa has limited treatment options, and cross resistance is already known for the recently approved BL–BLI combinations (e.g. C/T, CZA, I/R, MVB) due to their similar target mechanisms of resistance [Citation29,Citation42,Citation135]. In contrast, cefiderocol has advantages over these antibiotics in its stability against hydrolysis by various beta-lactamases, including many of the ESBLs and metallo-carbapenemases, as well as against resistance due to porin channel loss or upregulated efflux pumps, covering all categories of CR mechanisms in P. aeruginosa. It is less prone to resistance development during treatment, although further relevant clinical data are still required to confirm this observation.
Cefiderocol showed the highest susceptibility rates among the newer treatment options against CR P. aeruginosa in the SIDERO multinational surveillance program, which collected isolates over a 5-year period [Citation24,Citation29,Citation42,Citation137]. In SIDERO, less than 0.1% of P. aeruginosa isolates had cefiderocol MIC >4 µg/mL, and susceptibility rates among P. aeruginosa isolates which were non-susceptible to C/T and CZA were 99.8% and 100%, respectively [Citation42,Citation137]. Among these C/T-non-susceptible and CZA-non-susceptible isolates 85–88% were meropenem non-susceptible, suggesting a very limited number of treatment options [Citation137]. For such cases, cefiderocol could be considered as an active treatment option [Citation9]. In the study by Mushtaq et al. [Citation65], 13.5% of P. aeruginosa isolates demonstrated cefiderocol MIC >4 µg/mL in a special collection of unusual resistant isolates enriched for PER and MBL producers gathered over a 10-year period by the Public Health England Antimicrobial Resistance and HealthCare Associated Infections Reference Unit. To date, all surveillance reports suggest high-level and sustained susceptibility to cefiderocol, strengthening its potential recommendation for routine clinical use against CR P. aeruginosa. Susceptibility testing available for use in clinical microbiology laboratories (recommended) or through reference laboratories could guide recommendations for treatment of individual patients.
One relevant threat among carbapenemase-producing CR P. aeruginosa is the expression of an MBL enzyme, against which there are only two active antibiotics: cefiderocol in monotherapy and aztreonam in combination with a beta-lactamase inhibitor of the diazabicyclooctane group, such as avibactam. MBL-producing CR P. aeruginosa is spreading in some regions [Citation94], and new MBL enzymes may emerge in CR P. aeruginosa. It has been recognized that MBL-producing CR P. aeruginosa infections can have a faster onset of illness and faster progression to death than non-MBL-producing CR P. aeruginosa infections [Citation14,Citation172]. Thus, cefiderocol is a reasonable treatment choice in regions where MBL-producing CR P. aeruginosa is endemic, as none of the other treatment options have coverage against such strains as single agents.
Clinical data with cefiderocol treatment, which was utilized as monotherapy in clinical trials [Citation149–151] and often in combination therapy in the compassionate use program [Citation168] and in case reports (), have been promising. Cefiderocol was well tolerated in clinical studies. Adverse events reported included events previously associated with the cephalosporin class, such as Clostridioides difficile colitis, diarrhea, rash, or increased liver enzymes (). Cefiderocol showed similar efficacy to active comparator agents in clinical studies. The APEKS-cUTI and APEKS-NP studies enrolled patients with high risk of being infected by MDR Gram-negative bacteria, including P. aeruginosa. The non-inferiority of cefiderocol to high-dose, extended-infusion meropenem treatment in patients with NP suggests that cefiderocol has sufficient lung penetration to be effective against this infection. All-cause mortality in carbapenem-susceptible P. aeruginosa infections treated with cefiderocol was similar or lower than that of high-dose, extended-infusion meropenem in APEKS-NP, and similar between cefiderocol and best available therapy in CREDIBLE-CR for patients with CR P. aeruginosa without Acinetobacter spp. co-infection. In the subset of patients with Acinetobacter spp. co-infection, an imbalance in the underlying risk factors at randomization was observed, increasing the overall mortality risk. Therefore, more clinical data specific to CR P. aeruginosa infections are warranted to confirm the utility of cefiderocol against this challenging pathogen.
Overall, surveillance programs, real-world compassionate use data, and the CREDIBLE-CR study data demonstrated that cefiderocol is a valuable treatment option, without being restricted by the mechanism of carbapenem resistance, for CR P. aeruginosa infections. These considerations have been reflected in recent clinical practice guidelines or expert recommendations for the treatment of challenging, drug-resistant pathogens, including CR P. aeruginosa [Citation9,Citation99,Citation173].
Article highlights
Carbapenem-resistant (CR) Pseudomonas aeruginosa continues to be a challenging problem for infectious disease specialists and other physicians due to limited treatment options because of a variety of resistance mechanisms, which often co-exist in this species.
CR P. aeruginosa has developed multiple mechanisms that confer resistance to different beta-lactam antibiotics, including first-line treatment extended-spectrum, antipseudomonal penicillins, cephalosporins, and carbapenems, and resistance often extends to other classes as well, such as fluoroquinolones, aminoglycosides, and occasionally polymyxins.
The recent approvals of beta-lactam–beta-lactamase inhibitor (BL–BLI) combinations (e.g. ceftolozane–tazobactam, ceftazidime–avibactam, imipenem–relebactam, and to a lesser extent, meropenem–vaborbactam) offer solutions in the management of CR P. aeruginosa infections with clear evidence of their clinical efficacy; however, emerging resistance and cross-resistance have been reported.
Cefiderocol, a siderophore cephalosporin, with a distinct mechanism of cell entry via iron transport channels, can bypass many of the known beta-lactam resistance mechanisms. Moreover, among isolates resistant to the abovementioned BL–BLI combinations, cefiderocol has displayed a high level of in vitro activity.
Cefiderocol is stable against nearly all beta-lactamases, including class A Klebsiella pneumoniae carbapenemase, class B metallo-beta-lactamases, class C AmpC, and class D oxacillinase enzymes. Furthermore, loss of outer membrane porin channels and upregulated efflux pumps do not impact the cellular uptake of cefiderocol.
The multinational, 5-year SIDERO surveillance program reported sustained cefiderocol susceptibility rates in each year for meropenem-non-susceptible P. aeruginosa, with isolates rarely demonstrating cefiderocol minimum inhibitory concentrations >4 µg/mL.
In two prospective, double-blind, non-inferiority clinical studies and one pathogen-focused, descriptive, open-label, clinical study cefiderocol demonstrated clinical and microbiological efficacy against P. aeruginosa in patients with complicated urinary tract infections, nosocomial pneumonia, and among critically ill patients with serious CR infections.
Additional clinical evidence from the compassionate use program suggests that a large proportion of patients with CR P. aeruginosa, who had no alternative treatment options due to resistance, responded to cefiderocol treatment.
Cefiderocol appears to be an appropriate choice for the treatment of CR P. aeruginosa among the current treatment options, particularly in regions where resistance to BL–BLIs has been increasingly reported.
Declaration of interest
R Canton has participated in educational programs organized by Chiesi, MSD, Pfizer and Shionogi, received research support from MSD, Shionogi, and Venatorx Pharmaceuticals, and participated in evaluation of clinical trials for MSD and Pfizer. Y Doi has received honoraria for participation in scientific advisory boards for GSK, MSD, Gilead, Shionogi, MeijiSeika, Chugai, and bioMérieux, and received speaking fees from MSD, bioMérieux, and Shionogi. P J Simner has received research grants and consulting fees from OpGen Inc. and BD Diagnostics, research grants from Affinity Biosensors and Qiagen, and consulting fees from Shionogi Inc. and GeneCapture. The authors have no other relevant affiliations or financial involvement with any organization or entity with a financial interest in or financial conflict with the subject matter or materials discussed in the manuscript apart from those disclosed.
Reviewer disclosures
Peer reviewers on this manuscript have no relevant financial or other relationships to disclose.
Author contributions
All authors contributed substantially to the conception and structure of the review article, interpretation of the relevant literature, drafting, reviewing, and editing of the article, and all authors approved the final version for submission.
Acknowledgments
Editorial and medical writing support was provided by Adrienn Kis and Joanne Shrewsbury-Gee, Highfield, Oxford, United Kingdom. This support was sponsored by Shionogi & Co., Ltd., Osaka, Japan.
Additional information
Funding
References
- O’Donnell JN, Bidell MR, Lodise TP. Approach to the treatment of patients with serious multidrug-resistant Pseudomonas aeruginosa infections. Pharmacotherapy. 2020;40:952–969.
- Ibrahim D, Jabbour JF, Kanj SS. Current choices of antibiotic treatment for Pseudomonas aeruginosa infections. Curr Opin Infect Dis. 2020;33:464–473.
- Blomquist KC, Nix DE. A critical evaluation of newer β-lactam antibiotics for treatment of Pseudomonas aeruginosa infections. Ann Pharmacother. 2021;55:1010–1024. https://doi.org/https://doi.org/10.1177/1060028020974003.
- Nguyen L, Garcia J, Gruenberg K, et al. Multidrug-resistant Pseudomonas infections: hard to treat, but hope on the horizon? Curr Infect Dis Rep. 2018;20:23.
- Babiker A, Clarke LG, Saul M, et al. Changing epidemiology and decreased mortality associated with carbapenem-resistant Gram-negative bacteria from 2000 – 2017. Clin Infect Dis. 2021;73:e4521–e4530.
- Sader HS, Huband MD, Carvalhaes CG, et al. Frequency and antimicrobial susceptibility of bacteria isolated from patients hospitalized with pneumonia in US medical centers during 2018. Open Forum Infect Dis. 2019;6(Suppl 2):S756.
- Shortridge D, Arends SJR, Duncan LR, et al. Ceftolozane–tazobactam activity against difficult-to-treat resistance in Pseudomonas aeruginosa from bloodstream infections in US hospitals. Open Forum Infect Dis. 2019;6(Suppl 2):S580.
- Gajdács M, Bátori Z, Burián K. Interplay between phenotypic resistance to relevant antibiotics in Gram-negative urinary pathogens a data-driven analysis of 10 years’ worth of antibiogram data. Life (Basel). 2021;11:1059.
- Tamma PD, Aitken SL, Bonomo RA, et al. Infectious Diseases Society of America guidance on the treatment of extended-spectrum β-lactamase producing Enterobacterales (ESBL-E), carbapenem-resistant Enterobacterales (CRE), and Pseudomonas aeruginosa with difficult-to-treat resistance (DTR-P. aeruginosa). Clin Infect Dis. 2021;72:1109–1116.
- Centers for Disease Control and Prevention. Antibiotic resistance threats in the United States. 2019. [cited 2021 Jul 19]. Available from 2021 Jul 19: https://www.cdc.gov/drugresistance/pdf/threats-report/2019-ar-threats-report-508.pdf
- World Health Organization. Global priority list of antibiotic-resistant bacteria to guide research, discovery, and development of new antibiotics. 2017. [cited 2021 Dec 10]. Available from 2021 Dec 10: https://www.who.int/medicines/publications/WHO-PPL-Short_Summary_25Feb-ET_NM_WHO.pdf?ua=1 .
- Lodise TP Jr, Patel N, Kwa A, et al. Predictors of 30-day mortality among patients with Pseudomonas aeruginosa bloodstream infections: impact of delayed appropriate antibiotic selection. Antimicrob Agents Chemother. 2007;51:3510–3515.
- Micek ST, Wunderink RG, Kollef MH, et al. An international multicenter retrospective study of Pseudomonas aeruginosa nosocomial pneumonia: impact of multidrug resistance. Crit Care. 2015;19:219.
- Bassetti M, Vena A, Croxatto A, et al. How to manage Pseudomonas aeruginosa infections. Drugs Context. 2018;7:212527.
- Raman G, Avendano EE, Chan J, et al. Risk factors for hospitalized patients with resistant or multidrug-resistant Pseudomonas aeruginosa infections: a systematic review and meta-analysis. Antimicrob Resist Infect Control. 2018;7:79.
- Cai B, Echols R, Magee G, et al. Prevalence of carbapenem-resistant Gram-negative infections in the United States predominated by Acinetobacter baumannii and Pseudomonas aeruginosa. Open Forum Infect Dis. 2017;4:ofx176.
- Tartof SY, Kuntz JL, Chen LH, et al. Development and assessment of risk scores for carbapenem and extensive β-lactam resistance among adult hospitalized patients with Pseudomonas aeruginosa infection. JAMA Network Open. 2018;1:e183927.
- Rebold N, Morrisette T, Lagnf AM, et al. Early multicenter experience with imipenem-cilastatin-relebactam for multidrug-resistant Gram-negative infections. Open Forum Infect Dis. 2021;8:ofab554.
- Peña C, Suarez C, Gozalo M, et al. Spanish Network for Research in Infectious Diseases REIPI. Prospective multicenter study of the impact of carbapenem resistance on mortality in Pseudomonas aeruginosa bloodstream infections. Antimicrob Agents Chemother. 2012;56:1265–1272.
- Luyt CE, Sahnoun T, Gautier M, et al. Ventilator-associated pneumonia in patients with SARS-CoV-2-associated acute respiratory distress syndrome requiring ECMO: a retrospective cohort study. Ann Intensive Care. 2020;10:158.
- Carvalhaes CG, Shortridge D, Sader HS, et al. Activity of meropenem-vaborbactam against bacterial isolates causing pneumonia in patients in U.S. hospitals during 2014 to 2018. Antimicrob Agents Chemother. 2020;64:e02177–19.
- Patel TS, Kaye KS, Krishnan J, et al. Comparative in vitro activity of meropenem/vaborbactam and meropenem against a collection of real-world clinical isolates of Pseudomonas aeruginosa. Open Forum Infect Dis. 2019;6(Suppl 2):S251.
- Shortridge D, Streit JM, Carvalhaes C, et al. Activity of meropenem-vaborbactam and comparators against Pseudomonas aeruginosa isolates from patients with pneumonia in European hospitals. Presented at 31st ECCMID 2021; 2021 July 9–12; Abstract 01048.
- Klatt M, Dillman N, Eschenauer G, et al. Comparative in vitro activity of ceftolozane/tazobactam, ceftazidime/avibactam, meropenem/vaborbactam, imipenem/relebactam, and cefiderocol against P. aeruginosa, including drug-resistant isolates, at an academic medical center. Presented at 31st ECCMID 2021; 2021 July 9–12; Abstract 01228.
- Lob S, Hackel M, Young K, et al. In vitro activity of imipenem/relebactam against Gram-negative pathogens from patients with bloodstream infections in the United States and Canada – SMART 2018. Open Forum Infect Dis. 2020;7(Suppl 1):S789–790.
- Carvalhaes CG, Mendes RE, Flamm RK, et al. Comparative antimicrobial susceptibility of Gram-negative bacteria isolated from patients with bloodstream infections and pneumonia when tested against tazobactam combinations. Open Forum Infect Dis. 2019;6(Suppl 2):S746.
- Nguyen S, Hackel M, Hayes J, et al. In vitro antibacterial activity of cefiderocol against carbapenem-non-susceptible Gram-negative bacteria from hospitalized patients in the United States: SIDERO-WT-2014–2017. Presented at ASM Microbe 2019; 2019 Jun 20–24; San Francisco, CA, USA; Poster AAR-764.
- Sader HS, Carvalhaes CG, Streit JM, et al. Antimicrobial activity of ceftazidime-avibactam, ceftolozane-tazobactam and comparators tested against Pseudomonas aeruginosa and Klebsiella pneumoniae isolates from United States Medical Centers in 2016–2018. Microb Drug Resist. 2021;27:342–349.
- Shortridge D, Streit JM, Mendes R, et al. Activity of cefiderocol and comparators against US isolates of Pseudomonas aeruginosa, Acinetobacter baumannii-calcoaceticus complex, and Stenotrophomonas maltophilia, including carbapenem-resistant isolates. Presented at 31st ECCMID 2021; 2021 Jul 9–12; Abstract 01606.
- Golden AR, Adam HJ, Baxter M, et al. In vitro activity of cefiderocol, a novel siderophore cephalosporin, against Gram-negative bacilli isolated from patients in Canadian intensive care units. Diagn Microbiol Infect Dis. 2020;97:115012.
- Kazmierzak KM, de Figueiredo MLN, Lemos Luengas EV, et al. In vitro activity of ceftazidime-avibactam against enterobacterales and Pseudomonas aeruginosa collected in Latin America as part of the ATLAS global surveillance program, 2017–2019. Open Forum Infect Dis. 2020;7 (Suppl 1):S788.
- Kresken M, Korte-Berwanger M, Gatermann SG, et al. In vitro activity of cefiderocol against aerobic Gram-negative bacterial pathogens from Germany. Int J Antimicrob Agents. 2020;56:106128.
- Karlowsky JA, Kazmierczak KM, Bouchillon SK, et al. In vitro activity of ceftazidime-avibactam against clinical isolates of Enterobacteriaceae and Pseudomonas aeruginosa collected in Asia-Pacific countries: results from the INFORM Global Surveillance Program, 2012 to 2015. Antimicrob Agents Chemother. 2018;62:e02569–17.
- Teo JQ, Lim JC, Tang CY, et al. Ceftolozane/tazobactam resistance and mechanisms in carbapenem-nonsusceptible Pseudomonas aeruginosa. mSphere. 2021;6:e01026–20.
- Hsueh SC, Lee YJ, Huang YT, et al. In vitro activities of cefiderocol, ceftolozane/tazobactam, ceftazidime/avibactam and other comparative drugs against imipenem-resistant Pseudomonas aeruginosa and Acinetobacter baumannii, and Stenotrophomonas maltophilia, all associated with bloodstream infections in Taiwan. J Antimicrob Chemother. 2019;74:380–386.
- Shortridge D, Pfaller MA, Duncan LR, et al. Antimicrobial activity of ceftolozane-tazobactam tested against contemporary (2015–2017) P. aeruginosa isolates from a global surveillance program. ASM Microbe 2018; 2018 Jun 7–11; Atlanta, Georgia, USA.; Atlanta, Georgia, USA.
- Karlowsky JA, Lob SH, Young K, et al. Activity of ceftolozane/tazobactam against Gram-negative isolates from patients with lower respiratory tract infections – SMART United States 2018-2019. BMC Microbiol. 2021;21:74.
- Pfaller MA, Shortridge D, Sader HS, et al. Ceftolozane-tazobactam activity against drug-resistant Enterobacteriaceae and Pseudomonas aeruginosa causing healthcare-associated infections in Latin America: report from an antimicrobial surveillance program (2013–2015). Braz J Infect Dis. 2017;21:627–637.
- Sader HS, Carvalhaes CG, Duncan LR, et al. Susceptibility trends of ceftolozane/tazobactam and comparators when tested against European Gram-negative bacterial surveillance isolates collected during 2012–18. J Antimicrob Chemother. 2020;75:2907–2913.
- Lob S, Kazmierczak K, Chen W, et al. In vitro activity of ceftolozane/tazobactam against Pseudomonas aeruginosa from ICU and non-ICU patients with respiratory tract infections in the Asia-Pacific region – SMART 2016–2018. Presented at ICID 2020; 2020 February 20–23; Kuala Lumpur, Malaysia; Poster 1361.
- McKenzie T, Nguyen S, Hayes J, et al. Cefiderocol activity against North American clinical isolates SIDERO-WT-2014–2017. Presented at ASM Microbe 2019; 2019 June 20–24; San Francisco, CA, USA; Poster AAR-762.
- Nguyen S, Fam D, Hackel MS, et al. Susceptibility of phenotypic subsets of Pseudomonas aeruginosa isolates to cefiderocol and comparator agents from SIDERO-WT 2014–2019. Open Forum Infect Dis. 2021;8(Suppl 1):S491.
- Iregui A, Khan Z, Landman D, et al. Activity of cefiderocol against Enterobacterales, Pseudomonas aeruginosa, and Acinetobacter baumannii endemic to medical centers in New York City. Microb Drug Resist. 2020;26:722–726.
- Falagas ME, Skalidis T, Vardakas KZ, et al. Activity of cefiderocol (S-649266) against carbapenem-resistant Gram-negative bacteria collected from inpatients in Greek hospitals. J Antimicrob Chemother. 2017;72:1704–1708.
- Naas T, Lina G, Santerre Henriksen A, et al. In vitro activity of cefiderocol and comparators against isolates of Gram-negative pathogens from a range of infection sources: SIDERO-WT-2014-2018 studies in France. JAC Antimicrob Resist. 2021;3:dlab081.
- Stracquadanio S, Torti E, Longshaw C, et al. In vitro activity of cefiderocol and comparators against isolates of Gram-negative pathogens from a range of infection sources: SIDERO-WT-2014-2018 studies in Italy. J Glob Antimicrob Resist. 2021;25:390–398.
- Cercenado E, Cardenoso L, Penin R, et al. In vitro activity of cefiderocol and comparators against isolates of Gram-negative bacterial pathogens from a range of infection sources: SIDERO‑WT‑2014-2018 studies in Spain. J Glob Antimicrob Resist. 2021;26:292–300.
- Liu PY, Ko WC, Lee WS, et al. In vitro activity of cefiderocol, cefepime/enmetazobactam, cefepime/zidebactam, eravacycline, omadacycline, and other comparative agents against carbapenem-non-susceptible Pseudomonas aeruginosa and Acinetobacter baumannii isolates associated from bloodstream infection in Taiwan between 2018–2020. J Microbiol Immunol Infect. 2021;S1684-1182(21)00186–9 doi:https://doi.org/10.1016/j.jmii.2021.08.012. Online ahead of print .
- Karlowsky JA, Lob SH, Kazmierczak KM, et al. In vitro activity of imipenem/relebactam against Enterobacteriaceae and Pseudomonas aeruginosa isolated from intraabdominal and urinary tract infection samples: SMART Surveillance United States 2015–2017. J Glob Antimicrob Resist. 2020;21:223–228.
- Lob S, Hackel M, Badal R, et al. In vitro activity of imipenem-relebactam (mk-7655) against Enterobacteriaceae and Pseudomonas aeruginosa from Latin America – SMART 2015. Presented at ASM Microbe 2017; 2017 June 1–5; New Orleans, LA, USA; Poster 46.
- Lob SH, Karlowsky JA, Young K, et al. In vitro activity of imipenem-relebactam against resistant phenotypes of Enterobacteriaceae and Pseudomonas aeruginosa isolated from intraabdominal and urinary tract infection samples – SMART surveillance Europe 2015–2017. J Med Microbiol. 2020;69:207–217.
- Lob S, Kazmierczak K, Chen W, et al. Activity of imipenem/relebactam against clinical isolates of P. aeruginosa and K. pneumoniae collected in Asia/Pacific Countries – SMART 2016-2018. Presented at ICID 2020; 2020 February 20–23; Kuala Lumpur, Malaysia; Poster 2418.
- Sader HS, Duncan LR, Doyle TB, et al. Antimicrobial activity of ceftazidime/avibactam, ceftolozane/tazobactam and comparator agents against Pseudomonas aeruginosa from cystic fibrosis patients. JAC Antimicrob Resist. 2021;3:dlab126.
- Horcajada JP, Montero M, Oliver A, et al. Epidemiology and treatment of multidrug-resistant and extensively drug-resistant Pseudomonas aeruginosa infections. Clin Microbiol Rev. 2019;32:e00031–19.
- Young K, Painter RE, Raghoobar SL, et al. In vitro studies evaluating the activity of imipenem in combination with relebactam against Pseudomonas aeruginosa. BMC Microbiol. 2019;19:150.
- Wi YM, Greenwood-Quaintance KE, Schuetz AN, et al. Activity of ceftolozane-tazobactam against carbapenem-resistant, non-carbapenemase-producing Pseudomonas aeruginosa and associated resistance mechanisms. Antimicrob Agents Chemother. 2017;62:e01970–17.
- Papp-Wallace KM, Mack AR, Taracila MA, et al. Resistance to novel β-lactam-β-lactamase inhibitor combinations. The price of progress. Infect Dis Clinics North Am. 2020; 34:773–819.
- Ortiz de la Rosa JM, Nordmann P, Poirel L. ESBLs and resistance to ceftazidime/avibactam and ceftolozane/tazobactam combinations in Escherichia coli and Pseudomonas aeruginosa. J Antimicrob Chemother. 2019;74:1934–1939.
- Mushtaq S, Meunier D, Vickers A, et al. Activity of imipenem/relebactam against Pseudomonas aeruginosa producing ESBLs and carbapenemases. J Antimicrob Chemother. 2021;76:434–442.
- Zincke D, Balasubramanian D, Silver LL, et al. Characterization of a carbapenem-hydrolyzing enzyme, PoxB, in Pseudomonas aeruginosa PAO1. Antimicrob Agents Chemother. 2015;60:936–945.
- Arca-Suárez J, Lasarte-Monterrubio C, Rodiño-Janeiro BK, et al. Molecular mechanisms driving the in vivo development of OXA-10-mediated resistance to ceftolozane/tazobactam and ceftazidime/avibactam during treatment of XDR Pseudomonas aeruginosa infections. J Antimicrob Chemother. 2021;76:91–100.
- Ito A, Sato T, Ota M, et al. In vitro antibacterial properties of cefiderocol, a novel siderophore cephalosporin, against Gram-negative bacteria. Antimicrob Agents Chemother. 2017;62:e01454–17.
- Ito A, Nishikawa T, Ota M, et al. Stability and low induction propensity of cefiderocol against chromosomal AmpC β-lactamases of Pseudomonas aeruginosa and Enterobacter cloacae. J Antimicrob Chemother. 2018;73:3049–3052.
- Simner PJ, Beisken S, Bergman Y, et al. Cefiderocol activity against clinical Pseudomonas aeruginosa isolates exhibiting ceftolozane-tazobactam resistance. Open Forum Infect Dis. 2021;8:ofab311.
- Mushtaq S, Sadouki Z, Vickers A, et al. In vitro activity of cefiderocol, a siderophore cephalosporin, against multidrug-resistant Gram-negative bacteria. Antimicrob Agents Chemother. 2020;64:e01582–20.
- Ito-Horiyama T, Ishii Y, Ito A, et al. Stability of novel siderophore cephalosporin S-649266 against clinically relevant carbapenemases. Antimicrob Agents Chemother. 2016;60:4384–4386.
- Fraile-Ribot PA, Zamorano L, Orellana R, et al. Activity of imipenem-relebactam against a large collection of Pseudomonas aeruginosa clinical isolates and isogenic β-lactam-resistant mutants. Antimicrob Agents Chemother. 2020;64:e02165–19.
- Papp-Wallace KM, Endimiani A, Taracila MA, et al. Carbapenems: past, present, and future. Antimicrob Agents Chemother. 2011;55:4943–4960.
- Vabomere (meropenem and vaborbactam for injection, for intravenous use). Prescribing Information. Melinta Therapeutics, LLC, Lincolnshire IL 60069 USA; 2020.
- Vaborem (meropenem/vaborbactam 1 g/1 g powder for concentrate for solution for infusion). Summary of Product Characteristics. Menarini International Operations Luxembourg S.A.; 1 Avenue de la Gare, L-1611; Luxembourg; 2021.
- Pang Z, Raudonis R, Glick BR, et al. Antibiotic resistance in Pseudomonas aeruginosa: mechanisms and alternative therapeutic strategies. Biotechnol Adv. 2019;37(1):177–192.
- Rodriguez-Martinez J-M, Poirel L, Nordmann P. Molecular epidemiology and mechanisms of carbapenem resistance in Pseudomonas aeruginosa. Antimicrob Agents Chemother. 2009;53(11):4783–4788.
- Yin S, Chen P, You B, et al. Molecular typing and carbapenem resistance mechanisms of Pseudomonas aeruginosa isolated from a Chinese burn center from 2011 to 2016. Front Microbiol. 2018;9:1135.
- Aoki T, Yoshizawa H, Yamawaki K, et al. Cefiderocol (S-649266), a new siderophore cephalosporin exhibiting potent activities against Pseudomonas aeruginosa and other Gram-negative pathogens including multi-drug resistant bacteria: structure activity relationship. Eur J Med Chem. 2018;155:847–868.
- Ito A, Nishikawa T, Matsumoto S, et al. Siderophore cephalosporin cefiderocol utilizes ferric iron transporter systems for antibacterial activity against Pseudomonas aeruginosa. Antimicrob Agents Chemother. 2016;60:7396–7401.
- Barnes MD, Taracila MA, Rutter JD, et al. Deciphering the evolution of cephalosporin resistance to ceftolozane-tazobactam in Pseudomonas aeruginosa. mBio. 2018;9:e02085–18.
- Tamma PD, Beisken S, Bergman Y, et al. Modifiable risk factors for the emergence of ceftolozane-tazobactam resistance. Clin Infect Dis. 2021;73:e4599–e4606.
- Vanegas JM, Cienfuegos AV, Ocampo AM, et al. Similar frequencies of Pseudomonas aeruginosa isolates producing KPC and VIM carbapenemases in diverse genetic clones at tertiary-care hospitals in Medellin, Colombia. J Clin Microbiol. 2014;52:3978–3986.
- Osawa K, Shigemura K, Kitagawa K, et al. Molecular characteristics of carbapenem-resistant Pseudomonas aeruginosa isolated from urine in Hyogo, Japan. Int J Urol. 2019;26:127–133.
- Verma N, Prahraj AK, Mishra B, et al. Detection of carbapenemase-producing Pseudomonas aeruginosa by phenotypic and genotypic methods in a tertiary care hospital of East India. J Lab Physicians. 2019;11:287–291.
- Fraile-Ribot PA, Fernández J, Gomis-Font MA, et al. In vivo evolution of GES β-lactamases driven by ceftazidime/avibactam treatment of Pseudomonas aeruginosa infections. Antimicrob Agents Chemother. 2021;65:e0098621.
- Jorgensen SCJ, Rybak MJ. Meropenem and vaborbactam: stepping up the battle against carbapenem-resistant Enterobacteriaceae. Pharmacotherapy. 2018;38:444–461.
- Sabet M, Tarazi Z, Griffith DC. Activity of meropenem-vaborbactam against Pseudomonas aeruginosa and Acinetobacter baumannii in a neutropenic mouse thigh infection model. Antimicrob Agents Chemother. 2018;63:e01665–18.
- Petty LA, Henig O, Patel TS, et al. Overview of meropenem-vaborbactam and newer antimicrobial agents for the treatment of carbapenem-resistant Enterobacteriaceae. Infect Drug Resist. 2018;11:1461–1472.
- Rahman M, Prasad KN, Gupta S, et al. Prevalence and molecular characterization of New Delhi metallo-beta-lactamases in multidrug-resistant Pseudomonas aeruginosa and Acinetobacter baumannii from India. Microb Drug Resist. 2018;24:792–798.
- Hishinuma T, Uchida H, Tohya M, et al. Emergence and spread of VIM-type metallo-beta-lactamase-producing Pseudomonas aeruginosa clinical isolates in Japan. J Glob Antimicrob Resist. 2020;23:265–268.
- Croughs PD, Klaassen CHW, van Rosmalen J, et al. Unexpected mechanisms of resistance in Dutch Pseudomonas aeruginosa isolates collected during 14 years of surveillance. Int J Antimicrob Agents. 2018;52:407–410.
- Carugati M, Piazza A, Peri AM, et al. Fatal respiratory infection due to ST308 VIM-1-producing Pseudomonas aeruginosa in a lung transplant recipient: case report and review of the literature. BMC Infect Dis. 2020;20:635.
- Tamma PD, Smith TT, Adebayo A, et al. Prevalence of bla CTX-M genes in Gram-negative bloodstream isolates across 66 hospitals in the United States. J Clin Microbiol. 2021;59:e00127–21.
- Poulakou G, Lagou S, Karageorgopoulos DE, et al. New treatments of multidrug-resistant Gram-negative ventilator-associated pneumonia. Ann Transl Med. 2018;6:423.
- Karvouniaris M, Pontikis K, Nitsotolis T, et al. New perspectives in the antibiotic treatment of mechanically ventilated patients with infections from Gram-negatives. Expert Rev Anti Infect Ther. 2021;19:825–844.
- Yamano Y. In vitro activity of cefiderocol against a broad range of clinically important Gram-negative bacteria. Clin Infect Dis. 2019;69(Suppl 7):S544–S551.
- Sadek M, Poirel L, Nordmann P. Rapid detection of carbapenemase-producing Pseudomonas spp. using the NitroSpeed-Carba NP test. Diagn Microbiol Infect Dis. 2021;99:115280.
- Gill CM, Aktaþ E, Alfouzan W, et al. ERACE-PA Global Study Group. The ERACE-PA global surveillance program: ceftolozane/tazobactam and ceftazidime/avibactam in vitro activity against a global collection of carbapenem-resistant Pseudomonas aeruginosa. Eur J Clin Microbiol Infect Dis. 2021;40(12):2533–2541.
- Gill CM, Asempa TE, Nicolau DP. Development and application of a pragmatic algorithm to guide definitive carbapenemase testing to identify carbapenemase-producing Pseudomonas aeruginosa. Antibiotics (Basel). 2020;9(11):738.
- Behzadi P, Baráth Z, Gajdács M. It’s not easy being green: a narrative review on the microbiology, virulence and therapeutic prospects of multidrug-resistant Pseudomonas aeruginosa. Antibiotics. 2021;10:42.
- Diaz Iglesias Y, Van Bambeke F. Activity of antibiotics against Pseudomonas aeruginosa in an in vitro model of biofilms in the context of cystic fibrosis: influence of the culture medium. Antimicrob Agents Chemother. 2020;64:e02204–19.
- Sindeldecker D, Stoodley P. The many antibiotic resistance and tolerance strategies of Pseudomonas aeruginosa. Biofilm. 2021;3:100056.
- Timsit JF, Ruppé E, Barbier F, et al. Bloodstream infections in critically ill patients: an expert statement. Intensive Care Med. 2020;46:266–284.
- Chen IH, Nicolau DP, Kuti JL. Imipenem/cilastatin/relebactam alone and in combination against Pseudomonas aeruginosa in the in vitro pharmacodynamic model. Antimicrob Agents Chemother. 2020;64:e01764–20.
- Carrara E, Savoldi A, Piddock LJV, et al. Clinical management of severe infections caused by carbapenem-resistant Gram-negative bacteria: a worldwide cross-sectional survey addressing the use of antibiotic combinations. Clin Microbiol Infect. 2022;28:66–72.
- Scudeller L, Righi E, Chiamenti M, et al. Systematic review and meta-analysis of in vitro efficacy of antibiotic combination therapy against carbapenem-resistant Gram-negative bacilli. Int J Antimicrob Agents. 2021;57:106344.
- Jorgensen SCJ, Trinh TD, Zasowski EJ, et al. Real-world experience with ceftazidime-avibactam for multidrug-resistant Gram-negative bacterial infections. Open Forum Infect Dis. 2019;6:ofz522.
- Avycaz. Ceftazidime-avibactam for injection for intravenous use. Prescribing Information. Allergan USA, Inc; Madison NJ 07940 USA; 2020.
- Zavicefta. Ceftazidime-avibactam (2 g/0.5 g powder for concentrate for solution for infusion). Summary of product characteristics. Pfizer Ireland Pharmaceuticals Operations Support Group; Ringaskiddy, County Cork, Ireland; 2021.
- Wagenlehner FM, Sobel JD, Newell P, et al. Ceftazidime-avibactam versus doripenem for the treatment of complicated urinary tract infections, including acute pyelonephritis: RECAPTURE, a phase 3 randomized trial program. Clin Infect Dis. 2016;63:754–762.
- Carmeli Y, Armstrong J, Laud PJ, et al. Ceftazidime-avibactam or best available therapy in patients with ceftazidime-resistant Enterobacteriaceae and Pseudomonas aeruginosa complicated urinary tract infections or complicated intra-abdominal infections (REPRISE): a randomised, pathogen-directed, phase 3 study. Lancet Infect Dis. 2016;16:661–673.
- Torres A, Zhong N, Pachl J, et al. Ceftazidime-avibactam versus meropenem in nosocomial pneumonia, including ventilator-associated pneumonia (REPROVE): a randomised, double-blind, phase 3 non-inferiority trial. Lancet Infect Dis. 2018;18:285–295.
- Mazuski JE, Gasink LB, Armstrong J, et al. Efficacy and safety of ceftazidime-avibactam plus metronidazole versus meropenem in the treatment of complicated intra-abdominal infection: results from a randomized, controlled, double-blind, phase 3 program. Clin Infect Dis. 2016;62:1380–1389.
- Zerbaxa. Ceftolozane-tazobactam for injection for intravenous use. Prescribing Information. Merck & Co., Inc.; Whitehouse Stations NJ 08889 USA; 2020.
- Zerbaxa. Ceftolozane-tazobactam (1 g/0.5 g powder for solution for infusion). Summary of product characteristics. Merck Sharp & Dohme B.V. Waarderweg 39, 2031 BN Haarlem, The Netherlands; 2020.
- Lucasti C, Hershberger E, Miller B, et al. Multicenter, double-blind, randomized, phase II trial to assess the safety and efficacy of ceftolozane-tazobactam plus metronidazole compared with meropenem in adult patients with complicated intra-abdominal infections. Antimicrob Agents Chemother. 2014;58:5350–5357.
- Solomkin J, Hershberger E, Miller B, et al. Ceftolozane/tazobactam plus metronidazole for complicated intra-abdominal infections in an era of multidrug resistance: results from a randomized, double-blind, Phase 3 trial (ASPECT-cIAI). Clin Infect Dis. 2015;60:1462–1471.
- Wagenlehner FM, Umeh O, Steenbergen J, et al. Ceftolozane-tazobactam compared with levofloxacin in the treatment of complicated urinary-tract infections, including pyelonephritis: a randomised, double-blind, phase 3 trial (ASPECT-cUTI). Lancet. 2015;385:1949–1956.
- Kollef MH, Nováček M, Kivistik Ü, et al. Ceftolozane-tazobactam versus meropenem for treatment of nosocomial pneumonia (ASPECT-NP): a randomised, controlled, double-blind, phase 3, non-inferiority trial. Lancet Infect Dis. 2019;19:1299–1311.
- Haidar G, Philips NJ, Shields RK, et al. Ceftolozane-tazobactam for the treatment of multidrug-resistant Pseudomonas aeruginosa infections: clinical effectiveness and evolution of resistance. Clin Infect Dis. 2017;65:110–120.
- Recarbrio. Imipenem-cilastatin-relebactam for injection for intravenous use. Prescribing Information. Merck & Co., Inc.; Whitehouse Station NJ 08889 USA; 2020.
- Recarbrio. Imipenem-cilastatin-relebactam(500 mg/500 mg/250 mg powder for solution for infusion). Summary of product characteristics. Merck Sharp & Dohme B.V. Waarderweg 39, 2031 BN Haarlem, The Netherlands; 2020.
- Sims M, Mariyanovski V, McLeroth P, et al. Prospective, randomized, double-blind, Phase 2 dose-ranging study comparing efficacy and safety of imipenem/cilastatin plus relebactam with imipenem/cilastatin alone in patients with complicated urinary tract infections. J Antimicrob Chemother. 2017;72:2616–2626.
- Motsch J, Murta de Oliveira C, Stus V, et al. RESTORE-IMI 1: a multicenter, randomized, double-blind trial comparing efficacy and safety of imipenem/relebactam vs colistin plus imipenem in patients with imipenem-nonsusceptible bacterial infections. Clin Infect Dis. 2020;70:1799–1808.
- Titov I, Wunderink RG, Roquilly A, et al. A randomized, double-blind, multicenter trial comparing efficacy and safety of imipenem/cilastatin/ relebactam versus piperacillin/tazobactam in adults with hospital-acquired or ventilator-associated bacterial pneumonia (RESTORE-IMI 2 Study). Clin Infect Dis. 2021;73:e4539–e4548.
- Kaye KS, Bhowmick T, Metallidis S, et al. Effect of meropenem-vaborbactam vs piperacillin-tazobactam on clinical cure or improvement and microbial eradication in complicated urinary tract infection: the TANGO I randomized clinical trial. JAMA. 2018;319:788–799.
- Wunderink RG, Giamarellos-Bourboulis EJ, Rahav G, et al. Effect and safety of meropenem-vaborbactam versus best-available therapy in patients with carbapenem-resistant Enterobacteriaceae infections: the TANGO II randomized clinical trial. Infect Dis Ther. 2018;7:439–455.
- Alosaimy S, Lagnf AM, Morrisette T, et al. Real-world, multicenter experience with meropenem-vaborbactam for Gram-negative bacterial infections including carbapenem-resistant Enterobacterales and Pseudomonas aeruginosa. Open Forum Infect Dis. 2021;8:ofab371.
- Stone GG, Newell P, Gasink LB, et al. Clinical activity of ceftazidime/avibactam against MDR Enterobacteriaceae and Pseudomonas aeruginosa: pooled data from the ceftazidime/avibactam Phase III clinical trial programme. J Antimicrob Chemother. 2018;73:2519–2523.
- Page MGP. The role of iron and siderophores in infection, and the development of siderophore antibiotics. Clin Infect Dis. 2019;69(Suppl 7):S529–S537.
- Cornelis P, Dingemans J. Pseudomonas aeruginosa adapts its iron uptake strategies in function of the type of infections. Front Cell Infect Microbiol. 2013;3:75.
- Luscher A, Moynié L, Auguste PS, et al. TonB-dependent receptor repertoire of Pseudomonas aeruginosa for uptake of siderophore-drug conjugates. Antimicrob Agents Chemother. 2018;62:e00097–18.
- Ito A, Ishibashi N, Ishii R, et al. Changes of responsible iron-transporters for the activity of cefiderocol against Pseudomonas aeruginosa depending on the culture conditions. Presented at ASM Microbe 2019; 2019 Jun 20–24; San Francisco, CA, USA; Poster AAR-772.
- Matsumoto S, Kanazawa S, Sato T, et al. Activities of cefiderocol with simulated human plasma concentrations against carbapenem-resistant gram-negative bacilli in an in vitro chemostat model. Antimicrob Agents Chemother. 2020;64(11):e01128–20.
- Sato T, Yamawaki K. Cefiderocol: discovery, chemistry, and in vivo profiles of a novel siderophore cephalosporin. Clin Infect Dis. 2019;69(Suppl 7):S538–S543.
- Pybus CA, Felder-Scott C, Obuekwe V, et al. Cefiderocol retains antibiofilm activity in multidrug-resistant Gram-negative pathogens. Antimicrob Agents Chemother. 2021;65:e01194–20.
- Banin E, Vasil ML, Greenberg EP. Iron and Pseudomonas aeruginosa biofilm formation. Proc Natl Acad Sci U S A. 2005;102:11076–11081.
- Hackel MA, Tsuji M, Yamano Y, et al. In vitro activity of the siderophore cephalosporin, cefiderocol, against a recent collection of clinically relevant Gram-negative bacilli from North America and Europe, including carbapenem-nonsusceptible isolates (SIDERO-WT-2014 study). Antimicrob Agents Chemother. 2017;61:e00093–17.
- Hackel MA, Tsuji M, Yamano Y, et al. In vitro activity of the siderophore cephalosporin, cefiderocol, against carbapenem-nonsusceptible and multidrug-resistant isolates of Gram-negative bacilli collected worldwide in 2014 to 2016. Antimicrob Agents Chemother. 2018;62:e01968–17.
- Kazmierczak KM, Tsuji M, Wise MG, et al. In vitro activity of cefiderocol, a siderophore cephalosporin, against a recent collection of clinically relevant carbapenem-non-susceptible Gram-negative bacilli, including serine carbapenemase- and metallo-β-lactamase-producing isolates (SIDERO-WT-2014 Study). Int J Antimicrob Agents. 2019;53:177–184.
- Karlowsky JA, Hackel MA, Takemura M, et al. In vitro susceptibility of Gram-negative pathogens to cefiderocol in five consecutive annual multinational SIDERO-WT surveillance studies (2014–2019). Antimicrob Agents Chemother. 2022 Feb 15;66(2):e0199021.
- Longshaw C, Tsuji M, Hackel MM, et al. 679. In vitro activity of cefiderocol (CFDC), a novel siderophore cephalosporin, against difficult-to-treat-resistant (DTR) Gram-negative bacterial pathogens from the multi-national sentinel surveillance study, SIDERO-WT (2014–2017). Open Forum Infect Dis. 2019;6(Suppl 2):S309–S310.
- Soriano M, Nguyen SN, Hayes J, et al. Comparative activity of cefiderocol against Pseudomonas aeruginosa by infection source and census region in the United States: SIDERO-WT-2014–2017. Presented at ASM Microbe 2019; 2019 June 20–24; San Francisco, CA, USA; Poster Saturday AAR-766.
- Rolston KVI, Gerges B, Shelburne S, et al. Activity of cefiderocol and comparators against isolates from cancer patients. Antimicrob Agents Chemother. 2020;64:e01955–19.
- Lonsway DR, Bhatnagar A, Balbuena R, et al. Characterization of a pan-resistant Pseudomonas aeruginosa containing blaNDM-1 and blaIMP-1. Presented at ASM Microbe 2019; 2019 June 20–24; San Francisco, CA, USA; Poster AAR-622.
- Simner PJ, Patel R, Burnham C-AD. Cefiderocol antimicrobial susceptibility testing considerations: the Achilles’ heel of the Trojan horse? J Clin Microbiol. 2020;59:e00951–20.
- Matsumoto S, Singley CM, Hoover J, et al. Efficacy of cefiderocol against carbapenem-resistant Gram-negative bacilli in immunocompetent-rat respiratory tract infection models recreating human plasma pharmacokinetics. Antimicrob Agents Chemother. 2017;61:e00700–17.
- Nakamura R, Ito-Horiyama T, Takemura M, et al. In vivo pharmacodynamic study of cefiderocol, a novel parenteral siderophore cephalosporin, in murine thigh and lung infection models. Antimicrob Agents Chemother. 2019;63(9):e02031–18.
- Monogue ML, Tsuji M, Yamano Y, et al. Efficacy of humanized exposures of cefiderocol (S-649266) against a diverse population of Gram-negative bacteria in a murine thigh infection model. Antimicrob Agents Chemother. 2017;61:e01022–17.
- Ghazi IM, Monogue ML, Tsuji M, et al. Humanized exposures of cefiderocol, a siderophore cephalosporin, display sustained in vivo activity against siderophore-resistant Pseudomonas aeruginosa. Pharmacology. 2018;101:278–284.
- Fetroja. Cefiderocol for injection for intravenous use. Prescribing Information. Shionogi Inc., Florham Park, NJ 07932, USA; 2020.
- Fetcroja. Cefiderocol (1 g powder for concentrate for solution for infusion). summary of product characteristics. Shionogi B.V., 151 Kingsfordweg, 1043GR Amsterdam, The Netherlands; 2020.
- Portsmouth S, van Veenhuyzen D, Echols R, et al. Cefiderocol versus imipenem-cilastatin for the treatment of complicated urinary tract infections caused by Gram-negative uropathogens: a phase 2, randomised, double-blind, non-inferiority trial. Lancet Infect Dis. 2018;18:1319–1328.
- Wunderink RG, Matsunaga Y, Ariyasu M, et al. Cefiderocol versus high-dose, extended-infusion meropenem for the treatment of Gram-negative nosocomial pneumonia (APEKS-NP): a randomised, double-blind, phase 3, non-inferiority trial. Lancet Infect Dis. 2021;21:213–225.
- Bassetti M, Echols R, Matsunaga Y, et al. Efficacy and safety of cefiderocol or best available therapy for the treatment of serious infections caused by carbapenem-resistant Gram-negative bacteria (CREDIBLE-CR): a randomised, open-label, multicentre, pathogen-focused, descriptive, phase 3 trial. Lancet Infect Dis. 2021;21:226–240.
- Matsunaga Y, Ariyasu M, Takemura M, et al. Cefiderocol treatment for serious infections caused by carbapenem-resistant bacteria: post-hoc analysis of outcomes by pathogen in the CREDIBLE-CR study. Open Forum Infect Dis. 2020;7(Suppl 1):S212.
- Edgeworth JD, Merante D, Patel S, et al. Compassionate use of cefiderocol as adjunctive treatment of native aortic valve endocarditis due to extremely drug-resistant Pseudomonas aeruginosa. Clin Infect Dis. 2019;68:1932–1934.
- Stevens RW, Clancy M. Compassionate use of cefiderocol in the treatment of an intraabdominal infection due to multidrug-resistant Pseudomonas aeruginosa: a case report. Pharmacotherapy. 2019;39:1113–1118.
- Alamarat ZI, Babic J, Tran TT, et al. Long-term compassionate use of cefiderocol to treat chronic osteomyelitis caused by extensively drug-resistant Pseudomonas aeruginosa and extended-spectrum-β-lactamase-producing Klebsiella pneumoniae in a pediatric patient. Antimicrob Agents Chemother. 2020;64:e01872–19.
- Zingg S, Nicoletti GJ, Kuster S, et al. Cefiderocol for extensively drug-resistant Gram-negative bacterial infections: real-world experience from a case series and review of the literature. Open Forum Infect Dis. 2020;7:ofaa185.
- Bavaro DF, Romanelli R, Stolfa S, et al. Recurrent neurosurgical site infection by extensively drug-resistant P. aeruginosa treated with cefiderocol: a case report and literature review. Infect Dis (Lond). 2021;53:206–211.
- Grande Perez C, Maillart E, Miendje Deyi VY, et al. Compassionate use of cefiderocol in a pancreatic abscess and emergence of resistance. Infect Dis Now. 2021; 51: 399–401.
- Kufel WD, Steele JM, Riddell SW, et al. Cefiderocol for treatment of an empyema due to extensively drug-resistant Pseudomonas aeruginosa: clinical observations and susceptibility testing considerations. IDCases. 2020;21:e00863.
- Bodro M, Hernández-Meneses M, Ambrosioni J, et al. Salvage treatment with cefiderocol regimens in two intravascular foreign body infections by MDR Gram-negative pathogens, involving non-removable devices. Infect Dis Ther. 2021;10:575–581.
- Bleibtreu A, Dortet L, Bonnin RA, et al. Susceptibility testing is key for the success of cefiderocol treatment: a retrospective cohort study. Microorganisms. 2021;9:282.
- Grasa CD, Gómez-Gil MR, San Román Pacheco S, et al. Compassionate use of cefiderocol for VIM metallo-β-lactamase-producing Pseudomonas aeruginosa infection in a toddler with Burkitt lymphoma. J Glob Antimicrob Resist. 2021;26:91–92.
- Cipko K, Kizny Gordon A, Adhikari S, et al. Cefiderocol treatment of Pseudomonas aeruginosa and extensively drug-resistant Acinetobacter baumannii retained spinal hardware infection causing reversible acute interstitial nephritis: recto: cefiderocol causing acute interstitial nephritis. Int J Infect Dis. 2021;109:108–111.
- Chavda A, Gilchrist M, Samarasinghe D. Education: a compassionate use of cefiderocol to treat osteomyelitis caused by an XDR Pseudomonas aeruginosa. JAC Antimicrob Resist. 2021;3(Suppl 1):i18–i20.
- Streling AP, Al Obaidi MM, Lainhart WD, et al. Evolution of cefiderocol non-susceptibility in Pseudomonas aeruginosa in a patient without previous exposure to the antibiotic. Clin Infect Dis. 2021;73:e4472–e4474.
- Borghesi L, Viaggi V, Franzetti M, et al. Successful prolonged cefiderocol treatment of a chronic left pleural empyema caused by Pseudomonas aeruginosa in a patient affected by COVID-19: a case report. J Glob Antimicrob Resist. 2021;27:157–159.
- Meschiari M, Volpi S, Faltoni M, et al. Real-life experience with compassionate use of cefiderocol for difficult-to-treat resistant Pseudomonas aeruginosa (DTR-P) infections. JAC Antimicrob Resist. 2021;3:dlab188.
- Satlin MJ, Fam D, Longshaw C, et al. Clinical response by minimum inhibitory concentrations in carbapenem-resistant Pseudomonas aeruginosa infections under cefiderocol compassionate use program. Open Forum Infect Dis. 2021;8(Suppl 1): S717.
- Nordmann P, Shields RK, Doi Y, et al. MIC increase manuscript. Mechanisms of reduced susceptibility to cefiderocol among isolates from the CREDIBLE-CR and APEKS-NP clinical trials. Microbial Drug Resist. 2022;28:398–407.
- Kumar A, Ellis P, Arabi Y, et al. Initiation of inappropriate antimicrobial therapy results in a fivefold reduction of survival in human septic shock. Chest. 2009;136:1237–1248.
- Kumar A, Roberts D, Wood KE, et al. Duration of hypotension before initiation of effective antimicrobial therapy is the critical determinant of survival in human septic shock. Crit Care Med. 2006;34(6):1589–1596.
- Lucena A, Dalla Costa LM, Nogueira KS, et al. Nosocomial infections with metallo-beta-lactamase-producing Pseudomonas aeruginosa: molecular epidemiology, risk factors, clinical features and outcomes. J Hosp Infect. 2014;87:234–240.
- EAU Guidelines. Edn. presented at the EAU Annual Congress Milan Italy 2021. [ cited 2021 October 26]. Available from: https://uroweb.org/wp-content/uploads/EAU-Guidelines-on-Urological-infections-2021.pdf