Abstract
Objectives
To investigate associations between sensitivity to temporal fine structure (TFS) and performance in cognitive and speech-in-noise recognition tests.
Design
A binaural test of TFS sensitivity (the TFS-LF) was used. Measures of cognition included the reading span, Raven’s, and text-reception threshold tests. Measures of speech recognition included the Hearing in noise (HINT) and the Hagerman matrix sentence tests in three signal processing conditions.
Study sample
Analyses are based on the performance of 324/317 adults with and without hearing impairment.
Results
Sensitivity to TFS was significantly correlated with both the reading span test and the recognition of speech-in-noise processed using noise reduction, the latter only when limited to participants with hearing impairment. Neither association was significant when the effects of age were partialled out.
Conclusions
The findings are consistent with previous research in finding no evidence of a link between sensitivity to TFS and working memory once the effects of age had been partialled out. The results provide some evidence of an influence of signal processing strategy on the association between TFS sensitivity and speech-in-noise recognition. However, further research is necessary to assess the generalisability of the findings before any claims can be made regarding any clinical implications of these findings.
Introduction
The goal of this study is to investigate the associations between sensitivity to binaural temporal fine structure, and both cognitive ability and speech-in-noise recognition in adults with hearing impairment or normal hearing.
In order to perceive speech, it is necessary to extract information on both the spectral and temporal components of the signal. Temporal components of speech consist of two sources of information, the temporal envelope and the temporal fine structure. The temporal envelope is the amplitude contour of a signal and refers to fluctuations up to around 64 Hz (Ewert and Dau Citation2000), whilst temporal fine structure (TFS) refers to the faster fluctuations in the signal relating to phase and frequency. Although the upper-frequency boundary at which TFS cues are usable is still under debate (upper limits of 1500 Hz for monaural and between 1500 and 10,000 Hz for binaural processing have been suggested (see Verschooten et al. Citation2019).
TFS and cognition
Performance in TFS tests has been shown to correlate with performance on some cognitive tests, but results have been mixed. In a study of 30 adults with normal hearing, TFS sensitivity was associated with performance in the test of everyday attention, and the digit span (forwards and backwards), trail-making test (test B) and block design tests, however not to the reading span test (Füllgrabe, Moore, and Stone Citation2014). Neher et al. (Citation2012), based on a sample of 17 adults with hearing impairment found significant correlations between TFS and the map search subtest of the test of everyday attention and reading span test. However, once the effects of age had been partialled out, no significant correlations remained. Lőcsei et al. (Citation2016) also found no evidence of a link between the reading span test and TFS sensitivity in their study of younger adults with normal hearing, and older adults with hearing loss.
TFS and speech recognition
Relatively few studies have looked at the relation between TFS sensitivity and the perception of natural speech (‘natural’ meaning here that the temporal characteristics of the speech have not been manipulated, see for example Hopkins, Moore, and Stone Citation2008). Léger, Moore, and Lorenzi (Citation2012) found that performance in the binaural TFS-LF (TFS-low-frequency test; at 500 Hz but not 750 Hz) correlated with low- and mid-frequency VCV (vowel-consonant-vowel) recognition in speech-shaped noise in a sample of adults with normal low-to-mid frequency hearing, but there was no link between speech recognition in quiet and sensitivity to TFS (Léger, Moore, and Lorenzi Citation2012). However, Buss, Hall, and Grose (Citation2004) reported a significant correlation between word recognition in quiet and TFS sensitivity (measured using a binaural test of frequency modulation) in a study of 12 adults with mild-to-moderate hearing loss.
The majority of studies investigating the relation between TFS sensitivity and speech recognition have used matrix sentences as stimuli, however, findings have been mixed. Strelcyk and Dau (Citation2009) found that both binaural and monaural TFS sensitivity correlated significantly with speech recognition in the two-talker background, or in noise lateralised to one ear, but not with speech presented in amplitude-modulated noise. King et al. (Citation2017) also reported mixed results, finding that monaural TFS sensitivity was significantly correlated with natural speech but only when the target and masker were spatially separated. No significant correlations were found between binaural TFS sensitivity and speech recognition in that study (King et al. Citation2017). However, Neher et al. (Citation2012) observed a significant association between binaural TFS sensitivity and performance in a test of spatial speech recognition using the same sentence corpus as both King et al. (Citation2017) and Strelcyk and Dau (Citation2009). Both King et al. (Citation2017) and Neher et al. (Citation2012) used a masker of two female talkers. In contrast, Lőcsei et al. (Citation2016) found no evidence of a relation between binaural TFS sensitivity and matrix sentence recognition in different types of noise, both co-located and separated from the target. Hopkins and Moore (Citation2011) used more predictable sentences as stimuli in their study, finding that monaural, but not binaural, TFS sensitivity predicted speech-in-noise recognition in modulated but not steady noise.
The relative paucity of research, along with the small sample sizes and methodological differences between studies make it difficult to get a clear idea of the effects of TFS sensitivity on speech recognition, or of how this may differ depending on the nature of the stimuli or background noise used, or the cognitive and auditory skills of the listener. However, if the effects of TFS sensitivity on speech recognition are partly related to a more general age-related decline e.g. in temporal or cognitive processing or processing efficiency (see Moore et al. 2016; Kortlang, Mauermann, and Ewert Citation2016), it seems reasonable to think that the effects of TFS sensitivity may be more evident in tasks where cognitive skills can be used to aid performance, that is to say when contextual information, rather than simply auditory information, can be used to aid performance, particularly when the sound is degraded in some way, and the role of cognition is heightened (e.g. RöNnberg et al. Citation1989). If this were the case, we would expect TFS sensitivity to correlate most with high context sentence recognition, then lower context sentence recognition, and least with single word or nonsense syllable recognition. Alternatively, if the effects of TFS sensitivity on speech recognition are driven primarily by deficits in the auditory system, we would expect to see the opposite pattern of results whereby TFS sensitivity would be expected to correlate most with single word or nonsense syllable recognition, then lower context sentence recognition, and least with high context sentence recognition, although listeners may be able to use cognitive skills to ameliorate some of these effects.
The results of a factor analysis of a large-scale dataset indicate that temporal processing is associated more strongly with the perception of lower-context speech material than higher context speech material (Rönnberg et al. Citation2016). This finding provides support for the latter hypothesis. Interestingly, the temporal factor was also associated with a cognitive factor, however, whether this association drove the link between speech recognition and temporal processing is difficult to determine. As the goal of that paper was to get an overall picture of the results, only composite measures were used in that study. As such, those results do not allow for the examination of associations between individual tests and conditions, something that is of vital importance if the findings is to be used to inform practice in audiology clinics.
TFS and hearing impairment
Many studies have found that listeners with hearing loss perform more poorly on TFS tests than listeners with normal hearing do (Buss, Hall, and Grose Citation2004; Strelcyk and Dau Citation2009; Hopkins and Moore Citation2011; Lőcsei et al. Citation2016), however, only Hopkins and Moore (Citation2011) controlled for age differences between the participants with normal hearing and the (usually much older) participants with hearing loss. While numerous studies have shown that hearing loss is associated with reduced sensitivity to TFS, the degree of severity of the deficit does not seem to correlate to audiometric thresholds (Hopkins, Moore, and Stone Citation2008). Furthermore, reduced sensitivity to TFS has been shown to lead to poorer subjective ratings of hearing ability prior to hearing aid fitting, and larger subjective improvements in hearing ability at post-fitting assessments (Perez, McCormack, and Edmonds Citation2014). Perez, McCormack, and Edmonds (Citation2014) therefore suggest that testing TFS sensitivity prior to hearing aid fitting could provide useful information in terms of predicting, and managing individuals’ expectations of, hearing aid outcomes. Further support for the idea that information regarding TFS sensitivity should be considered prior to hear aid fitting is provided by Moore and Sęk (Citation2016) who found that participants with poorer sensitivity to TFS showed a tendency to prefer slow compression over fast compression (although there was a lot of variation between individuals) and Lopez-Poveda et al. (Citation2017), who found that temporal processing ability predicted aided speech intelligibility in noise in adults with hearing impairment.
TFS and age
Age has also been shown to affect sensitivity to TFS, even when audiometric thresholds are within the normal range (Füllgrabe Citation2013; Füllgrabe, Moore, and Stone Citation2014), or when the effects of hearing threshold are partialled out (Léger, Moore, and Lorenzi Citation2012; King, Hopkins, and Plack Citation2014). However, the relative contribution of age and hearing loss to deficits in TFS processing seems to vary according to whether TFS is processed monaurally or binaurally, with monaural TFS sensitivity being affected more by hearing loss than age, and binaural TFS sensitivity being affected more by age than hearing loss (Hopkins and Moore Citation2011; Moore, Vickers, and Mehta Citation2012; Moore et al. Citation2016).
Aims of the study
The aim of this study is to investigate how binaural TFS sensitivity correlates with diotic speech-in-noise recognition in different conditions (including different signal processing strategies) in a large sample of adults with hearing thresholds ranging from normal to severe hearing loss. These findings will contribute to our understanding of whether binaural sensitivity to TFS can be used during hearing screening or hearing aid fitting. We are also interested in how TFS sensitivity relates to performance in cognitive tests, and whether the pattern of results indicates that temporal processing may mediate the link between cognition and speech recognition. Additionally, we will investigate the effects of age and hearing loss on performance on the tasks, and relations between the variables.
Materials and methods
The data forming the basis of this paper are a subset of those collected as part of the n200 study (see Rönnberg et al. Citation2016) a large-scale study investigating the links between cognition and speech recognition in adults.
Participants
A total of 433 adults, with normal hearing or hearing impairment, were recruited from the University Hospital of Linköping to the initial stages of the n200. For the analyses based on speech recognition, 39 participants were excluded for not having completed the speech tests, and 5 more were excluded for not having completed the TFS sensitivity test. Of the remaining participants, 3 were excluded for missing audiogram data, and 62 were excluded for having performed below chance in the test of TFS sensitivity. For the analyses relating to performance on cognitive tests, 46 were excluded for not having completed the cognitive tests, and 6 participants were excluded for not having completed the TFS sensitivity test. Of the remaining participants, 3 were excluded for missing audiogram data, and 61 were excluded for having performed below the chance level in the TFS sensitivity test. Thus, the analyses based on speech recognition scores use a sample of 324 participants (137 F, 187 M; 33 to 78 years old, with a mean age of 60), and those based on cognitive scores use a sample of 317 participants (133 F, 184 M; 27 to 78 years old, with a mean age of 60). See for audiometric thresholds (based on participants included in the speech analyses). 170 of the participants included in the speech analyses and 172 of the participants in the cognitive analyses, those with the highest hearing thresholds, had been fitted bilaterally with hearing aids at least one year prior to testing.
Table 1. Descriptive statistics for TFS (threshold in degrees), age (years), PTA (dBHL, mean of both ears), audiometric threshold at 250 Hz (dBHL, mean of both ears), and performance in the Reading span (number correct), Raven’s (number correct), and TRT (% unmasked text).
Participants excluded for performing below chance on the TFS test
Participants scoring below the chance level on the TFS sensitivity test were excluded from further analysis. Relative differences between this group and the group that performed above chance on the TFS sensitivity task may affect the generalisability of the results and can provide insight into factors that may be associated with poorer sensitivity to TFS. The data reported here are based on participant groups in the speech analyses (as fewer participants were excluded for missing data than in the cognitive analyses).
62 participants performed below chance on the TFS sensitivity test. Of these, 40 (corresponding to 64.5%) were female, 21 (corresponding to 33.9%) were male, and data was missing for one participant. This gender split differs from that in the group that performed above chance in the TFS, where 42.3% were female and 57.7% male. Excluded participants were also slightly older, with a mean age of 66 compared to a mean age of 60 in the participants that scored above chance in the TFS sensitivity test. Audiometric data for the two groups can be seen in , and descriptive statistics for group performance in all tasks can be found in and .
Figure 1. Median audiometric thresholds of participants who performed below (dashed line) and above (solid line) chance on the TFS test. The shaded area represents the interquartile range of audiometric thresholds for the participants who scored above chance on the TFS test. The dotted lines represent the interquartile range of audiometric thresholds for the participants who scored below chance on the TFS test. These data are based on the participant groups in the speech analyses.
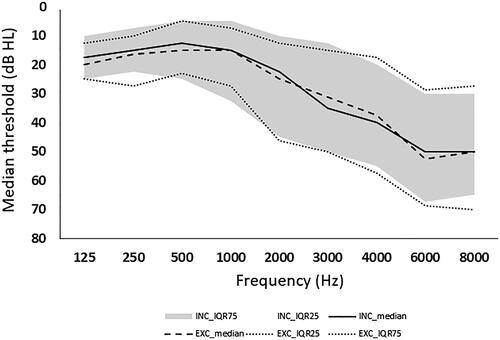
Procedure
Participants attended three test sessions, during which they completed a number of audiological, cognitive and speech recognition tests, a subset of which form the basis of the present article and are outlined in detail below. The selection of the cognitive tests was motivated by previous research in the field. The reading span test and a block design test (similar to Raven’s) have both been previously investigated in terms of their relation to TFS (see Füllgrabe, Moore, and Stone Citation2014; Neher et al. Citation2012; Lőcsei et al. Citation2016), so including similar tests in the current test battery allows for easier comparison of the results. The TRT was included as it has been shown to correlate to speech in noise recognition (see e.g. Besser et al. Citation2012), but the relation between performance on this test and TFS sensitivity had not yet been investigated.
Pure tone audiometry
Pure tone air conduction thresholds were obtained from both ears. The average threshold at 500, 1000, 2000, and 4000 Hz across both ears was then calculated. Mean thresholds (across both ears) at 250 Hz were also included in further analyses, as this frequency was used in the TFS test.
Temporal fine structure-low frequency (TFS-LF) test at 250 Hz
The TFS-LF test was developed by Hopkins and Moore (Citation2010) and is a measure of binaural sensitivity to temporal fine structure. An adaptive two-alternative forced-choice procedure was used in which participants were presented binaurally with two intervals, each containing four tones. In each trial, one of the intervals contained an interaural phase shift. The participant's task was to identify which of the two intervals contained this interaural phase shift. Depending on performance, one of two scoring methods was used as recommended by Hopkins and Moore (Citation2010). The primary scoring method was to calculate a threshold in degrees corresponding to 71% accuracy. If performance in the task was poor (indicated by reaching the maximum phase shift possible twice early in the test, or once later), the block was terminated. Forty new trials were then presented in which the maximum phase shift of 180 degrees was used in each trial, allowing a percentage correct score to be calculated. Scores calculated using the percentage correct method were converted to a d prime equivalent to 71% correct and then extrapolated to give a score in degrees to allow comparison to the standard adaptive scores (see Hopkins and Moore Citation2010, based on methods by Hacker and Ratcliff Citation1979; and Hafter and Carrier Citation1972). This procedure meant that extrapolated scores over 180 degrees indicated performance below chance level. Participants completed the TFS test twice, and their mean score was calculated and used as the outcome measure. Participants scoring below the chance level on either test were removed from further analyses.
Text reception threshold (TRT)
The TRT (Zekveld et al. Citation2007, Citation2018) requires participants to read sentences masked by a pattern of bars and has been shown to index executive processes including updating and inhibition (e.g. Mishra et al. Citation2013) along with lexical access and speeded sentence completion ability (Zekveld et al. Citation2018). The sentence stimuli were taken from the Swedish HINT test (Hällgren, Larsby, and Arlinger Citation2006; Nilsson, Soli, and Sullivan Citation1994), and were presented word by word in red text on a white background behind a stationary black bar pattern. The bar pattern was varied between trials adaptively, such that a correct response leads to a greater percentage of text in the following sentence being masked. Once the final word had been presented, the whole sentence was visible for 3500 ms. Participants read the sentence back to the experimenter, who scored a response as correct only when the entire sentence was correctly read. One practice list was administered, and the outcome measure was the average percentage of unmasked text in 16 trials.
Raven’s standard progressive matrices
Raven’s standard progressive matrices (e.g. Raven Citation2008) is a paper-and-pencil task that requires participants to pick the missing piece of a pattern from six options. Two sets (D and E) of the test were administered, each set consisting of 12 trials. These two subtests are thought to index analogical reasoning skills and strategy use (Van der Ven and Ellis Citation2000) or verbal analytic reasoning and visuospatial ability (Lynn, Allik, and Irwing 2004). Set A was used as a practice list, during which the experimenter gave feedback. The outcome measure was the total number of correctly answered trials, out of a maximum of 24. Participants were allowed a total of one hour to complete the task, but no time limit was given at the level of individual trials.
Reading span test
The reading span test (Daneman and Carpenter Citation1980; RöNnberget al. Citation1989) is a measure of complex working memory span, focussing on both processing and storage of information. The test consists of a series of sentences presented visually on a computer screen. The sentences were presented one word at a time at a rate of one word every 800 ms. The sentences presented are always grammatically correct, but half of the sentences are semantically meaningless (e.g. “The fox wrote poetry”). Immediately after having read the sentence, participants are asked to make a yes-no judgement about whether the sentence made sense (processing component). After each block of sentences, participants are asked to recall as many of the first or last words of each sentence as possible (storage component). All responses are typed by the participants, who were asked to respond as quickly and accurately as possible. The number of sentences in a block varies from two to five, with two blocks of each length. This gives a maximum score of 28. The blocks were presented in ascending order of difficulty.
HINT
Sentence-in-noise recognition was measured using the Swedish hearing-in-noise test (HINT, Hällgren, Larsby, and Arlinger Citation2006; Nilsson, Soli, and Sullivan Citation1994). The HINT consists of lists of ten everyday sentences (e.g. “The boys played at the beach”) presented diotically in stationary white noise. The participants’ task was to repeat the sentences back to the experimenter, who scored a response as correct if all words were correctly repeated. The stimuli were processed through an experimental hearing aid, which was programmed with individually prescribed settings to provide linear amplification (see Rönnberg et al. Citation2016 for details of the device and settings) and played through ER3 insert earphones (Etymotic Research). Both the signal and the noise were initially presented at 65 dB SPL, and the level of the noise was then varied adaptively in 2 dB increments. Participants completed one practice list and two test lists. The SNR required to achieve 50% correct was then calculated based on the SNRs of the last 15 sentences.
Hagerman test
The Hagerman test (Hagerman Citation1982) is a Swedish matrix sentence test. Each sentence is five words long and always takes the format of noun-verb-number-adjective-noun (e.g. ‘Peter bought eight black pens’). The sentences were presented diotically in a background of unmodulated speech-weighted noise or four-talker babble, and the SNR needed to correctly repeat 50% and 80% of the words was calculated. In this study, we report only the data from the 80% conditions, as we considered these conditions to be those that best represent everyday listening conditions. Responses were scored by the experimenter, who marked a response as correct if all words were repeated by the participant. The initial presentation level was set to 65 dB SPL, with an SNR of 0, and the sentences were varied adaptively using an interleaved procedure tracking both the 50% and 80% performance levels (Brand Citation2000). In order to investigate the influence of hearing aid settings, three signal processing strategies were used: linear amplification only (LA), linear amplification plus noise reduction (NR), and fast-acting nonlinear amplification (NA). Stimuli were again processed using an experimental hearing aid and presented through ER3A earphones (Etymotic Research). Further details of the signal processing algorithms can be found in Rönnberg et al. (Citation2016).
Results
Prior to analysis, the data were examined to determine whether the assumptions for parametric testing were satisfied. The TFS-LF scores were not normally distributed, thus all subsequent correlations and partial correlations are calculated using Spearman’s rank, and descriptive statistics are presented in terms of the median and interquartile range (IQR). All reported p-values are based on two-tailed significance using an alpha level of 0.05, and have been interpreted using the Bonferroni–Holm adjustment for multiple comparisons (Holm Citation1979). Analyses were conducted using IBM SPSS Statistics v25.
A significant positive correlation was observed between TFS-LF score and participants’ age in the cognition analyses (just failing to reach significance in the speech analyses once a correction for multiple comparisons was applied), yet not between TFS-LF score and PTA or audiometric threshold at 250 Hz in either the cognition or speech analyses. As such, all subsequent correlation analyses are supported by partial correlations controlling for the effects of age.
TFS sensitivity and the relation to cognition
Descriptive statistics are shown in , and correlations/partial correlations are presented in .
Table 2. Correlations and partial correlations (controlling for age) between TFS, age, PTA, audiometric threshold at 250 Hz, and performance in the cognitive tasks.
The correlation between the TFS-LF score and the reading span test was significant, such that better performance on the reading span test was associated with better sensitivity to TFS. However, after partialling out the effects of age, the two were not significantly correlated. Correlations between TFS sensitivity and both Raven’s test and the TRT were not significant.
TFS and the relation to aided speech recognition
Descriptive statistics are shown in , and correlations/partial correlations are presented in .
Table 3. Descriptive statistics for TFS (threshold in degrees), age (years), PTA (dBHL), audiometric threshold at 250 Hz (dBHL, mean of both ears), and performance in the HINT task (SNR 50%), and the Hagerman test in speech-shaped noise (SSN) and four-talker babble (4TB).
Table 4. Correlations and partial correlations (controlling for age) between TFS, age, PTA, audiometric threshold at 250 Hz, and performance in the Hagerman and HINT tasks.
Descriptive statistics () indicate that participants obtained better scores in the NR condition of the Hagerman test than in the LA or NL settings (see Yumba Citation2017 for further analysis of these differences).
When considering the participant group as a whole, no significant correlations were observed between TFS sensitivity and performance on any of the speech tasks. After partialling out the effects of age, correlations were further reduced.
Separate analyses were conducted to assess the association between TFS sensitivity and performance in the different signal processing conditions in the group of participants that used hearing aids (who also had greater hearing thresholds than the other participants). These analyses, shown in , showed a significant correlation between TFS sensitivity and performance in the noise reduction condition of the Hagerman test in speech-shaped noise, however, once age was controlled for this association was no longer significant.
Table 5. Correlations and partial correlations (controlling for age) between TFS and performance in the Hagerman test for the subset of participants with hearing impairment.
Discussion
The results of the study show that sensitivity to TFS correlates with age, but not with the degree of hearing impairment (indexed by pure tone average thresholds at 0.5, 1, 2, & 4 kHz) or audiometric threshold at the test frequency (250 Hz in this case). Performance on the reading span test correlated with TFS sensitivity. However, this association was no longer significant when the effects of age were partialled out. Regarding the association between TFS sensitivity and speech-in-noise recognition, when the analyses were conducted on the group as a whole, there were no significant correlations between TFS sensitivity and either performance in the HINT or any of the conditions of the Hagerman test. This pattern remained when age was controlled for. Given the non-linearity of two of the processing conditions, it was expected that any potential differences relating to signal processing in associations between TFS sensitivity and speech-in-noise recognition would be greater for participants with poorer hearing thresholds. As such, separate analyses were conducted on the subgroup of participants that wore hearing aids (which were also the participants with the poorer hearing thresholds). These analyses showed a significant correlation between TFS sensitivity and speech recognition in the noise reduction condition of the Hagerman test when a masker of speech-shaped noise was used, however, this association was no longer significant when the effects of age were controlled for. There were no significant associations, including when age was controlled for, between TFS sensitivity and speech-in-noise recognition in either the HINT or the linear amplification or non-linear compression conditions of the Hagerman test.
The relation of TFS sensitivity to age and hearing loss
The findings indicate that there is no influence of hearing thresholds on binaural TFS sensitivity. This is consistent with previous studies that have investigated the relation between TFS sensitivity and audiometric thresholds (e.g. Hopkins and Moore Citation2011; Moore, Vickers, and Mehta Citation2012). It is also important to note that when the effects of age were controlled for, the association between PTA and TFS sensitivity became stronger.
In addition to investigating the effects of severity of hearing loss, we also investigated the influence of age on sensitivity to TFS. Based on previous literature (see, Füllgrabe Citation2013; Füllgrabe, Moore, and Stone Citation2014), we would expect to find a significant effect of age on performance in the TFS sensitivity task, particularly since we used the TFS-LF test which is a measure of binaural TFS sensitivity thought to be more affected by age than performance in monaural TFS sensitivity tests (Moore et al. Citation2016). Our results were consistent with these studies, and age was significantly associated with performance on the TFS task. It is important to note, however, that our results do not allow us to determine which age-related factors drive the associations observed.
The relation of TFS to cognition
The analyses showed a significant association between sensitivity to TFS and performance in the reading span test, but not once the effects of age were partialled out. Associations between TFS sensitivity and performance on Raven’s test and the TRT were not significant. To our knowledge, of these measures, only the relation between the reading span test and TFS sensitivity has been previously investigated. Our findings are consistent with those that have previously been reported (Neher et al. Citation2012; Füllgrabe, Moore, and Stone Citation2014; Lőcsei et al. Citation2016) finding no evidence of a significant correlation between the reading span and TFS sensitivity once the effects of age have been partialled out.
Previous research on the relation between TFS sensitivity and cognition has reported significant correlations (after partialling out the effects of age) between TFS sensitivity and performance on the digit span, trail making test (test B), block design, and for the visual elevator and map search subtests of the test of everyday attention (Füllgrabe, Moore, and Stone Citation2014; however, note that Neher et al. Citation2012 found no evidence of a link between the latter two measures and TFS). The Raven’s test used in the current study is similar to the block design test used in the study reported by Füllgrabe, Moore, and Stone (Citation2014), but our findings did not provide evidence of a significant association between TFS sensitivity and performance in Raven’s test. Thus, the results of this study provide evidence of an association between the reading span test and TFS sensitivity, however, this can be explained by the association between age and working memory.
The relation of TFS sensitivity to aided speech recognition
In the present study, we investigated the relation between TFS sensitivity and performance on two aided sentence-in-noise recognition tasks, the HINT and the Hagerman. The HINT test is an open-set sentence recognition task, higher in contextual cues than the Hagerman test which is a closed-set matrix sentence recognition task. We found no clear evidence of an effect of contextual information, with no significant association being observed between TFS sensitivity and HINT, or between TFS sensitivity and performance in two of the Hagerman conditions. Thus, these results do not clearly support (or arguably contradict) either a processing efficiency, general temporal processing, or auditory-only based explanation of the link between speech recognition and TFS sensitivity. Previous studies investigating the link between TFS sensitivity and objective speech in noise recognition in listeners with hearing loss have often observed either limited (e.g. Léger, Moore, and Lorenzi Citation2012) or no significant link (e.g. Lőcsei et al. Citation2016) between the two. While the results of the present study seem to provide some evidence for such a link, there was no clear evidence for the idea that the amount of contextual information may affect the degree to which sensitivity to TFS correlated with speech recognition. Furthermore, it is interesting to note that the associations observed between TFS and speech recognition in this study are lower than the associations observed by Bernstein et al. (Citation2016), using data from a subset of the same participants, between speech recognition and performance in a test of spectro-temporal modulation, thus further research using similar tests may provide an interesting direction for future research into associations between temporal processing and speech recognition.
The results do, however, provide some evidence of an effect of signal processing condition on the association between TFS sensitivity and speech-in-noise recognition. Specifically, in a subgroup of participants consisting of those that use hearing aids (and have the poorest hearing thresholds), the association between TFS sensitivity and speech-in-noise recognition was only significant in the noise reduction condition, and not in the linear amplification or fast-compression settings. However, this association was not significant when the effects of age were partialled out. Furthermore, this association was only significant when speech-shaped noise was used as a masker rather than four-talker babble. That we only see evidence of a signal processing effect in the group of hearing aid users compared to the group as a whole is not surprising given the non-linear nature of the fast compression and noise reduction algorithms (meaning that both processes would have greater effect with increasing hearing thresholds). However, given that we only tested these three conditions, it is not possible to state whether the findings would generalise to other signal processing algorithms or whether it is specific to the noise reduction method used to process stimuli in this study. Furthermore, it should be noted that the noise reduction condition with the speech-shaped noise masker was the condition in which the lowest SNRs were needed to achieve recognition levels of 80% correct, thus, it is difficult to separate the influence of the SNR achieved by the signal processing from factors specific to the algorithm or overall signal processing strategy. Future research in which SNRs were controlled for, rather than accuracy, would be a useful addition to the literature.
To our knowledge, no previous study has investigated whether signal processing strategy affects the relation between TFS sensitivity and speech in noise recognition, however, Moore and Sęk (Citation2016) reported that older adults with poorer TFS sensitivity are more likely to prefer slow compression than those with better sensitivity to TFS. In this study, we did not collect data on subjective preferences for signal processing strategies, but it would interesting for future research to further investigate the relationship between subjective and objective outcome measures.
Methodological considerations
Many participants were excluded from our analyses as they were unable to complete the TFS-LF test, limiting the generalisability of the findings. This group was on average five years older than the group that performed above chance on the TFS test, suggesting that the association between age and sensitivity to TFS is likely stronger than that reported here. Among those that were able to complete the test, in some cases there were large differences between performance on the two tests administered, suggesting that it may not always give reliable results for some people. Furthermore, depending on performance in the TFS-LF test, scores were either expressed as a threshold in degrees or as a percentage correct (with poorer performance). Percent correct scores were then transformed to allow them to be analysed together with the threshold scores. It is possible that scores based on the two methods are not directly comparable even after transformation. In order to overcome difficulties associated with participants being unable to complete the standard version of the TFS-LF test, Füllgrabe et al. (Citation2017) have developed a modified version of the test which all tested participants (Füllgrabe et al. Citation2017; Füllgrabe and Moore Citation2017) were able to complete. This may be promising for use in future research.
The results of this study are based on performance in a binaural test of TFS sensitivity. There is some evidence to suggest that monaural and binaural TFS sensitivity tests differ in the extent to which they are associated with different auditory processing tasks (e.g. Lopez-Poveda et al. Citation2017; Ewert, Paraouty, and Lorenzi Citation2020). Monaural processing of TFS is important for recognising speech in background noise and perceiving pitch, while binaural TFS is important for localising sound and obtaining benefit from the binaural masking level difference (see Moore et al. Citation2016 for a review). Thus, it may be that a different pattern of results would have been observed had we used a test of monaural TFS sensitivity or speech tasks in which the role of spatial processing was emphasised. Future research, investigating the role of both binaural and monaural TFS sensitivity on the recognition of speech processed by different signal processing algorithms, and in binaural, diotic, and monaural conditions would be a welcome addition to the literature.
Clinical implications
Previous studies have found links between TFS sensitivity and subjective ratings of hearing ability and hearing aid benefit (Perez, McCormack, and Edmonds Citation2014), and preference for slow versus fast compression (Moore and Sęk Citation2016). These results suggest that it may be beneficial to consider sensitivity to TFS prior to hearing aid fitting. The results of this study provide some evidence of an effect of signal processing strategy on the association between TFS sensitivity and speech-in-noise recognition. However, the generalisability of these findings, along with a further assessment of the influence of SNRs on the findings, will need to be investigated before any claims can reasonably be made about whether or how sensitivity to TFS could be used to inform clinical decisions about the suitability of different signal processing options.
Conclusions
An analysis of the relation between TFS sensitivity and performance on a cognitive test battery showed a link between TFS sensitivity and working memory, however, this was no longer significant when the effects of age were partialled out. No association was found between TFS sensitivity and either Raven’s test or the TRT. The results also provide some evidence of an association between sensitivity to TFS and aided speech-in-noise recognition in listeners with hearing loss, however, only when speech was presented in a background of speech-shaped noise, and processed using a noise reduction algorithm. Again, this association was not significant when the effects of age were partialled out. Further research is necessary to assess the generalisability of these findings and to determine precisely how factors associated with age affect these associations.
Parts of this work were previously presented in an article published in IJA (DOI: 10.1080/14992027.2019.1672899) that the authors subsequently retracted due to unintentional errors in the analyses.
Acknowledgements
The authors would like to thank Henrik Danielsson, Victoria Stenbäck, Rina Blomberg and Elaine Ng for answering questions about the N200 database. We would also like to thank Birger Kollmeier and the anonymous reviewers for helpful comments on earlier versions of this manuscript.
Disclosure statement
No potential conflict of interest was reported by the author(s).
References
- Bernstein, J. G., H. Danielsson, M. Hällgren, S. Stenfelt, J. Rönnberg, and T. Lunner. 2016. “Spectrotemporal Modulation Sensitivity as a Predictor of Speech-Reception Performance in Noise with Hearing Aids.” Trends in Hearing 20: 233121651667038. doi:10.1177/2331216516670387.
- Besser, J., A. A. Zekveld, S. E. Kramer, J. Rönnberg, and J. M. Festen. 2012. “New Measures of Masked Text Recognition in Relation to Speech-in-Noise Perception and their Associations with Age and Cognitive Abilities.” Journal of Speech, Language, and Hearing Research 55 (1): 194–209. doi:10.1044/1092-4388(2011/11-0008).
- Brand, T. 2000. Analysis and Optimization of Psychophysical Procedures in Audiology. Oldenburg, Germany: BIS Verlag.
- Buss, E., J. W. Hall, III, and J. H. Grose. 2004. “Temporal Fine-Structure Cues to Speech and Pure Tone Modulation in Observers with Sensorineural Hearing Loss.” Ear and Hearing 25 (3): 242–250. doi:10.1097/01.AUD.0000130796.73809.09
- Daneman, M., and P. A. Carpenter. 1980. “Individual Differences in Working Memory and Reading.” Journal of Verbal Learning and Verbal Behavior 19 (4): 450–466. doi:10.1016/S0022-5371(80)90312-6.
- Ewert, S. D., and T. Dau. 2000. “Characterizing Frequency Selectivity for Envelope Fluctuations.” The Journal of the Acoustical Society of America 108 (3 Pt 1): 1181–1196. doi:10.1121/1.1288665.
- Ewert, S. D., N. Paraouty, and C. Lorenzi. 2020. “A Two‐Path Model of Auditory Modulation Detection Using Temporal Fine Structure and Envelope Cues.” European Journal of Neuroscience 51 (5): 1265–1214. Advance online publication, doi:10.1111/ejn.13846.
- Füllgrabe, C. 2013. “Age-Dependent Changes in Temporal-Fine-Structure Processing in the Absence of Peripheral Hearing Loss.” American Journal of Audiology 22 (2): 313–315. doi:10.1044/1059-0889(2013/12-0070).
- Füllgrabe, C., A. J. Harland, A. P. Sęk, and B. C. Moore. 2017. “Development of a Method for Determining Binaural Sensitivity to Temporal Fine Structure.” International Journal of Audiology 56 (12): 926–935. doi:10.1080/14992027.2017.1366078.
- Füllgrabe, C., and B. C. Moore. 2017. “Evaluation of a Method for Determining Binaural Sensitivity to Temporal Fine Structure (TFS-AF Test) for Older Listeners with Normal and Impaired Low-Frequency Hearing.” Trends in Hearing 21: 2331216517737230. doi:10.1177/2331216517737230.
- Füllgrabe, C., B. C. Moore, and M. A. Stone. 2014. “Age-Group Differences in Speech Identification despite Matched Audiometrically Normal Hearing: Contributions from Auditory Temporal Processing and Cognition.” Frontiers in Aging Neuroscience 6: 347. doi:10.3389/fnagi.2014.00347.
- Hacker, M. J., and R. Ratcliff. 1979. “A Revised Table of d’ for M-Alternative Forced Choice.” Attention, Perception & Psychophysics 26 (2): 168–170. doi:10.3758/BF03208311.
- Hafter, E. R., and S. C. Carrier. 1972. “Binaural Interaction in Low-Frequency Stimuli: The Inability to Trade Time and Intensity Completely.” Journal of the Acoustical Society of America 51 (6): 1852–1862. doi:10.1121/1.1913044.
- Hagerman, B. 1982. “Sentences for Testing Speech Intelligibility in Noise.” Scandinavian Audiology 11 (2): 79–87. doi:10.3109/01050398209076203.
- Hällgren, M., B. Larsby, and S. Arlinger. 2006. “A Swedish Version of the Hearing in Noise Test (HINT) for Measurement of Speech Recognition.” International Journal of Audiology 45 (4): 227–237. doi:10.1080/14992020500429583.
- Holm, S. 1979. “A Simple Sequential Rejective Multiple Test Procedure.” Scandinavian Journal of Statistics 6: 65–70.
- Hopkins, K., and B. C. Moore. 2010. “Development of a Fast Method for Measuring Sensitivity to Temporal Fine Structure Information at Low Frequencies.” International Journal of Audiology 49 (12): 940–946. doi:10.3109/14992027.2010.512613.
- Hopkins, K., and B. C. Moore. 2011. “The Effects of Age and Cochlear Hearing Loss on Temporal Fine Structure Sensitivity, Frequency Selectivity, and Speech Reception in Noise.” Journal of the Acoustical Society of America 130 (1): 334–349. doi:10.1121/1.3585848.
- Hopkins, K., B. C. Moore, and M. A. Stone. 2008. “Effects of Moderate Cochlear Hearing Loss on the Ability to Benefit from Temporal Fine Structure Information in Speech.” Journal of the Acoustical Society of America 123 (2): 1140–1153. doi:10.1121/1.2824018.
- King, A., K. Hopkins, and C. J. Plack. 2014. “The Effects of Age and Hearing Loss on Interaural Phase Difference Discrimination.” Journal of the Acoustical Society of America 135 (1): 342–351. doi:10.1121/1.4838995.
- King, A., K. Hopkins, C. J. Plack, N. H. Pontoppidan, L. Bramsløw, R. K. Hietkamp, M. Vatti, et al. 2017. “The Effect of Tone-Vocoding on Spatial Release from Masking for Old, Hearing-Impaired Listeners.” Journal of the Acoustical Society of America 141 (4): 2591–2603. doi:10.1121/1.4979593.
- Kortlang, S., M. Mauermann, and S. D. Ewert. 2016. “Suprathreshold Auditory Processing Deficits in Noise: Effects of Hearing Loss and Age.” Hearing Research 331: 27–40. doi:10.1016/j.heares.2015.10.004.
- Léger, A. C., B. C. Moore, and C. Lorenzi. 2012. “Abnormal Speech Processing in Frequency Regions Where Absolute Thresholds Are Normal for Listeners with High-Frequency Hearing Loss.” Hearing Research 294 (1–2): 95–103. doi:10.1016/j.heares.2012.10.002.
- Lőcsei, G., J. H. Pedersen, S. Laugesen, S. Santurette, T. Dau, and E. N. MacDonald. 2016. “Temporal Fine-Structure Coding and Lateralized Speech Perception in Normal-Hearing and Hearing-Impaired Listeners.” Trends in Hearing 20: 2331216516660962. doi:10.1177/2331216516660962.
- Lopez-Poveda, E. A., P. T. Johannesen, P. Perez-González, J. L. Blanco, S. Kalluri, and B. Edwards. 2017. “Predictors of Hearing-Aid Outcomes.” Trends in Hearing 21: 2331216517730526. doi:10.1177/2331216517730526.
- Mishra, S., T. Lunner, S. Stenfelt, J. Rönnberg, and M. Rudner. 2013. “Seeing the Talker’s Face Supports Executive Processing of Speech in Steady State Noise.” Frontiers in Systems Neuroscience 7: 96. doi:10.3389/fnsys.2013.00096.
- Moore, B. C. 2016. “Effects of Age and Hearing Loss on the Processing of Auditory Temporal Fine Structure.” In Physiology, Psychoacoustics and Cognition in Normal and Impaired Hearing, Advances in Experimental Medicine and Biology, edited by P. van Dijk, 894, Cham, Switzerland: Springer.
- Moore, B. C., and A. Sęk. 2016. “Preferred Compression Speed for Speech and Music and Its Relationship to Sensitivity to Temporal Fine Structure.” Trends in Hearing 20: 2331216516640486. doi:10.1177/2331216516640486.
- Moore, B. C., D. A. Vickers, and A. Mehta. 2012. “The Effects of Age on Temporal Fine Structure Sensitivity in Monaural and Binaural Conditions.” International Journal of Audiology 51 (10): 715–721. doi:10.3109/14992027.2012.690079.
- Neher, T., T. Lunner, K. Hopkins, and B. C. Moore. 2012. “Binaural Temporal Fine Structure Sensitivity, Cognitive Function, and Spatial Speech Recognition of Hearing-Impaired Listeners (L) a.” Journal of the Acoustical Society of America 131 (4): 2561–2564. doi:10.1121/1.3689850.
- Nilsson, M., S. D. Soli, and J. A. Sullivan. 1994. “Development of the Hearing in Noise Test for the Measurement of Speech Reception Thresholds in Quiet and in Noise.” Journal of the Acoustical Society of America 95 (2): 1085–1099. doi:10.1121/1.408469.
- Perez, E., A. McCormack, and B. A. Edmonds. 2014. “Sensitivity to Temporal Fine Structure and Hearing-Aid Outcomes in Older Adults.” Frontiers in Neuroscience 8: 7. doi:10.3389/fnins.2014.00007.
- Raven, J. 2008. The Raven Progressive Matrices Tests: their Theoretical Basis and Measurement Model. Uses and Abuses of Intelligence. Studies Advancing Spearman and Raven’s Quest for Non-Arbitrary Metrics, 17–68. Unionville, USA: Royal Fireworks Press.
- RöNnberg, Jerker, Stig Arlinger, Björn Lyxell, and Catharina Kinnefors, 1989. “Visual Evoked Potentials: Relation to Adult Speechreading and Cognitive Function.” Journal of Speech, Language, and Hearing Research 32 (4): 725–735. doi:10.1044/jshr.3204.725.
- Rönnberg, J., T. Lunner, E. H. N. Ng, B. Lidestam, A. A. Zekveld, P. Sörqvist, B. Lyxell et al. 2016. “Hearing Impairment, Cognition and Speech Understanding: Exploratory Factor Analyses of a Comprehensive Test Battery for a Group of Hearing Aid Users, the n200 Study.” International Journal of Audiology 55 (11): 623–620. doi:10.1080/14992027.2016.1219775.
- Strelcyk, O., and T. Dau. 2009. “Relations between Frequency Selectivity, Temporal Fine-Structure Processing, and Speech Reception in Impaired Hearing.” Journal of the Acoustical Society of America 125 (5): 3328–3345. doi:10.1121/1.3097469.
- Van der Ven, A. H. G. S., and J. L. Ellis. 2000. “A Rasch Analysis of Raven’s Standard Progressive Matrices.” Personality and Individual Differences 29 (1): 45–64. doi:10.1016/S0191-8869(99)00177-4.
- Verschooten, E., S. Shamma, A. J. Oxenham, B. C. Moore, P. X. Joris, M. G. Heinz, and C. J. Plack. 2019. “The Upper Frequency Limit for the Use of Phase Locking to Code Temporal Fine Structure in Humans: A Compilation of Viewpoints.” Hearing Research 377: 109–121. doi:10.1016/j.heares.2019.03.011.
- Yumba, W. K. 2017. “Cognitive Processing Speed, Working Memory, and the Intelligibility of Hearing Aid-Processed Speech in Persons with Hearing Impairment.” Frontiers in Psychology 8: 1308. doi:10.3389/fpsyg.2017.01308.
- Zekveld, A. A., E. L. George, S. E. Kramer, S. T. Goverts, and T. Houtgast. 2007. “The Development of the Text Reception Threshold Test: A Visual Analogue of the Speech Reception Threshold Test.” Journal of Speech, Language, and Hearing Research 50 (3): 576–584. doi:10.1044/1092-4388(2007/040).
- Zekveld, A. A., M. Pronk, H. Danielsson, and J. Rönnberg. 2018. “Reading Behind the Lines: The Factors Affecting the Text Reception Threshold in Hearing Aid Users.” Journal of Speech, Language, and Hearing Research 61 (3): 762–775. doi:10.1044/2017_JSLHR-H-17-0196.