Abstract
Background: CXC-chemokines bearing the glutamic acid-leucine-arginine (ELR) motif (ELR+CXC chemokines) are potent neutrophil chemoattractants and hence may play a role in mucosal injury seen with intestinal ischemia-reperfusion (I/R). Methods: Serum concentrations of ELR+ CXC chemokines (keratinocyte-derived chemokine(KC)/CXC ligand (CXCL) 1, macrophage inflammatory protein (MIP)-2/CXCL 2/3, lipopolysaccharide-induced CXC chemokine (LIX)/CXCL5, and lungkine/CXCL15) were measured in a murine intestinal I/R model. Fifteen 4-week-old wild-type mice were studied in three subgroups: sham, ischemia (superior mesenteric artery [SMA] clamping for 60 min) and ischemia-reperfusion (SMA clamping for 60 min followed by reperfusion for 90 min). Results: Concentrations of KC/CXCL1 and MIP-2/CXCL2/3 in sham-treated animals (145 ± 123 and 107 ± 55 pg/mL, respectively) and the ischemia subgroup (646 ± 413 and 226 ± 129 pg/mL) were similar, but concentrations were significantly higher with reperfusion (6398 ± 2297, p < .001 and 874 ± 790 pg/mL, p = .04). LIX/CXCL5 and lungkine/CXCL15 concentrations did not change significantly with ischemia or following I/R. KC/CXCL1 and MIP-2/CXCL2/3 concentrations correlated positively with the severity of mucosal injury and with each other, whereas a negative relationship was observed between LIX/CXCL5 concentrations and microscopic injury scores. Conclusions: Development of mucosal injury in intestinal I/R is associated with increased serum concentrations of KC/CXCL1 and MIP-2/CXCL2/3, but not with those of LIX/CXCL5 and lungkine/CXCL15.
Intestinal ischemia-reperfusion (I/R) occurs in a variety of neonatal conditions, including perinatal asphyxia, shock, sepsis, cold injury, patency of the ductus arteriosus (per se or with remedial measures such as indomethacin), cardiovascular surgery, small bowel transplantation, and possibly with neonatal necrotizing enterocolitis (NEC) [Citation[1–10]]. Intestinal I/R causes local mucosal injury, increased epithelial permeability, and impairment of motility and often also triggers a generalized inflammatory response leading to multiorgan failure [Citation[1], Citation[11]].
Neutrophils play a major role in I/R injury. Activated neutrophils show increased emargination and tissue infiltration, and they release high concentrations of elastase, myeloperoxidase, and oxygen free radicals, factors that can amplify tissue injury [Citation[12]]. The degree of neutrophil infiltration correlates with the microvascular changes, mucosal dysfunction, and tissue damage [Citation[12], Citation[13]]. These observations have led to a renewed interest in neutrophil-specific chemokines, such as interleukin (IL)-8/CXC ligand (CXCL) 8, which are known to play an important role in acute inflammation [Citation[14]]. Indeed, studies in a rat model of intestinal I/R show that plasma concentrations of IL-8/CXCL8 correlate with the degree of mucosal injury, and neutralization of its activity by a specific monoclonal antibody can lead to diminution in tissue damage [Citation[15]].
Chemokines are small (8–10 kDa) peptides that derive their nomenclature from their function as chemotactic cytokines. These are classified by the number and arrangement of cysteine residues near the N-terminal end into CXC (two cysteines with a middle variable amino acid), CC, C, and CX3C families. Within the CXC family, the chemokines are further subclassified by the presence or absence of a glutamic acid-leucine-arginine (ELR) tripeptide motif, wherein the peptides that carry this sequence (ELR+ CXC chemokines) function as neutrophil chemoattractants. In humans, there are seven known ELR+ CXC chemokines: IL-8/CXCL8: granulocyte chemotactic protein-2 (GCP-2)/CXCL6; growth related oncoprotein (GRO)-α/CXCL1; GRO-β/CXCL2; GRO-γ/CXCL3; epithelial cell derived neutrophil chemoattractant-78 (ENA-78)/CXCL5; and neutrophil activating peptide-2 (NAP-2)/CXCL7. Of these, IL-8/CXCL8 is the best-studied member, but there is increasing information that the other ELR+ CXC chemokines play distinct roles according to the tissue, stimulus, and chronicity of inflammation [Citation[16–18]]. The redundancy in ELR+ CXC chemokines makes these mediators attractive targets for therapeutic manipulation, as it might be possible to selectively modulate some of the chemokines to minimize inflammation, but without seriously compromising innate immunity. However, the redundancy also makes it important that the differential expression of these chemokines is better understood, as it might be essential to target all upregulated chemokines to achieve maximal therapeutic efficacy [Citation[19]].
In experimental studies, several animal models have been used to study I/R [Citation[20–23]]. In this study, we have adapted an established rat model of intestinal I/R to weanling mice, as the murine genetic constitution is better understood and gene knockout animals are readily available [Citation[24]]. The original intestinal ischemia model has been subsequently modified by Dimmitt and colleagues [Citation[25]], who included an initial step of superior mesenteric artery occlusion with intraluminal injection of platelet activating factor, followed by a period of reperfusion. The mucosal injury observed in these rat pups has been considered a model for NEC as seen in premature human neonates and is graded on a five-point scale of severity () [Citation[24], Citation[25]]. We have simplified the model for use in mouse pups and excluded the step on PAF injection. We propose to study the intestinal mucosal injury in these mice as a general model of intestinal I/R in neonates, which also is a commonly encountered clinical problem [Citation[1–10]].
TABLE 1 Microscopic Injury Scoring Criteria
In mice, four ELR+ CXC chemokines are known: keratinocyte derived chemokine (KC)/CXCL1, macrophage inflammatory protein (MIP)-2/CXCL 2/3, lipopolysaccharide-inducible CXC chemokine (LIX)/CXCL5, and lungkine/CXCL15. KC/CXCL1 is the murine homologue of human GRO-α and is involved in the upregulation of neutrophil adhesion molecules. At higher concentrations, KC/CXCL1 also induces neutrophil chemotaxis. Its constitutive expression might be involved in the basal trafficking of neutrophils [Citation[26], Citation[27]]. MIP-2/CXCL2/3, similar to the human chemokines GRO-β/CXCL2 and GRO-γ/CXCL3, is a potent neutrophil activator and chemoattractant and is induced during inflammation [Citation[28]]. LIX/CXCL5, expressed by a variety of mesenchymal cells, is involved in neutrophil chemotaxis and also in the induction of other proinflammatory cytokines through the activation of nuclear factor-κB (NF-κB) [Citation[29]]. Lungkine/CXCL15 is a novel ELR+ CXC chemokines described in the developing lung with neutrophil chemotactic properties [Citation[30]]. In this study, we explored the effect of small intestinal I/R on the serum concentrations of all the four known murine ELR+ CXC chemokines and correlated the levels with the degree of tissue injury. We hypothesized that all ELR+ CXC chemokines are upregulated during I/R and that these concentrations would correlate with each other.
MATERIALS AND METHODS
The animal experiments were performed at the University of Alabama, Birmingham, and the frozen sera and tissues were transported on dry ice to the University of South Florida/All Children's Hospital Children's Research Institute, St. Petersburg, Florida. The study was approved by the Institutional Animal Care and Use Committees at both sites.
Ischemia/Reperfusion
Ten 4-week-old dam-fed wild-type mice (BALB/c, Jackson Laboratory, Bar Harbor, ME, USA) underwent laparotomy under halothane anesthesia, and the superior mesenteric artery (SMA) was externally occluded for 60 min of ischemia. Then 5 of these animals were sacrificed at this stage, and the other 5 underwent a second phase of reperfusion for 90 min wherein the SMA clamp was released. For comparison, another 5 animals that received only anesthesia and laparotomy were maintained as controls (sham). Serum samples were collected from each of these animals after euthanasia. Sections of the small intestine also were obtained for histologic examination and processed further by snap freezing/OTC embedding.
Histopathology
Intestinal tissue samples were studied by hematoxylin-eosin preparation to confirm the presence of NEC-like lesions. These lesions were graded for microscopic injury () by a masked observer (Dr. Lacson) [Citation[24], Citation[25]].
Chemokine ELISAs
Serum samples were collected after centrifuging blood samples for 10 min at 1000x g. These samples were separated into aliquots and stored at –80°C until the time of assay.
ELISA Determinations
Serum concentrations of KC/CXCL1, MIP-2/CXCL2/3, LIX/CXCL5, and lungkine/CXCL15 were quantified by sandwich ELISA. Commercially available ELISA kits were used for the measurement of KC/CXCL1 and MIP-2/CXCL2/3 (Quantikine M; R&D Systems, Minneapolis, MN, USA); the ELISA protocols for LIX/CXCL5 and lungkine/CXCL15 were standardized in our laboratory.
LIX/CXCL5 ELISA was prepared using monoclonal rat IgG2b capture antibody (Clone 61905) and biotinylated polyclonal goat IgG detection antibody (R&D). Ninety-six well plates were coated overnight with 4 mcg/mL of the capture antibody suspended in 0.2 µm filtered phosphate buffered saline (PBS). After washing, these plates were blocked overnight with 1% bovine serum albumin in PBS with 5% sucrose and 0.05% sodium azide. Samples or standards (recombinant mouse LIX/CXCL5, R&D) were applied (100μL) for 2 hrs. The plates were subsequently treated with 100 µL diluted biotinylated detection antibody (100 ng/mL) for another 2 hrs. Then 100 µL of diluted streptavidin-horse radish peroxidase solution (R&D) was applied for 20 min, following which 100 µL of substrate solution (1:1 solution of hydrogen peroxide and tetramethylbenzidine, R&D) was added for 20 min. These steps were followed by addition of 50 µL stop solution (1 M H2SO4), and the plates were read spectrophotometrically at 450/540 nm.
The lungkine/CXCL15 ELISA protocol was developed similarly using 4µg/mL monoclonal rat IgG2b capture antibody (Clone 96708) and 200 ng/mL biotinylated polyclonal goat IgG detection antibody (R&D). Recombinant mouse lungkine/CXCL15 (R&D) was used as standard.
Statistical Methods
ELISA protocols were standardized to select linear ranges of estimation after log transformation; r2 ≥ 0.95 was accepted. The lower limit of detection was estimated as the chemokine concentration corresponding to the mean + 2 standard deviation optical density for blanks; these values were calculated with 20 samples. Intra- and interassay precision was evaluated on three plates using diluted standards. These ELISA protocols were validated for serum samples by spiking with known concentrations of the standard.
The ELISA results were analyzed by descriptive methods. Correlation analyses included the Pearson and Spearman methods. The results were depicted using standard error-bar diagrams (means with standard errors). The groups were compared by one-way analysis of variance (with post hoc Bonferroni and Dunnett's t tests) for parametric, and the Kruskall-Wallis test for nonparametric data. For all statistical procedures, an α-error up to 0.05 was accepted. All the figures were rounded off to last significant figure according to sensitivity of the test employed.
RESULTS
Intestinal Injury
The animals in ischemia and the I/R groups developed gross ischemic injury in 60–100% of the length of the small intestine. Tissue samples were collected from the affected jejunal/ileal areas for microscopic assessment of intestinal injury (). The microscopic injury scores in the three groups differed significantly [median (range): 0 (0–0), 1 (1–3), and 3 (2–4), respectively; p = .003].
Figure 1 Intestinal section (H&E) showing mucosal injury following ischemia-reperfusion. (a) Sham subgroup, wherein the animals underwent anesthesia and laparotomy. These animals had intact mucosal architecture. (b) Ischemia subgroup. These animals developed superficial mucosal necrosis. The photomicrograph shows partial thickness mucosal necrosis (grade 2). (c) Ischemia-reperfusion subgroup. These animals developed severe mucosal injury, ranging from full thickness mucosal necrosis to transmural necrosis extending through the muscularis. The photomicrograph shows partial thickness muscularis necrosis (grade 3). Magnification: 100x.
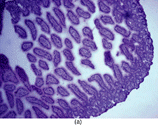
ELISA Protocols
The LIX/CXCL5 ELISA protocol had a lower limit of detection of 9 pg/mL and the OD-LIX/CXCL5 concentration relationship remained linear up to 1000 pg/mL. The test consistently detected 95–98% of the added LIX/CXCL5 in serum samples. In the lungkine/CXCL15 ELISA, the lower limit of detection was 16 pg/mL, and the test results remained linear in the range 31.2–500 pg/mL. This ELISA detected 90–95% of lungkine/CXCL15 in serum samples. The results are summarized in .
TABLE 2 Relevant Information for Each Chemokine ELISA
Chemokine Concentrations
The concentrations of KC/CXCL1 and MIP-2/CXCL2/3 were comparable in the sham (145 ± 123 and 107 ± 55 pg/mL, respectively) and ischemia groups (646 ± 413 and 226 ± 129 pg/mL) but were increased significantly in the I/R group (6398 ± 2297, p < .001 and 874 ± 790 pg/mL, p = .04). In contrast, the concentrations of LIX/CXCL5 (10492 ± 1878, 8128 ± 1226, and 6790 ± 3480 pg/mL in the three subgroups, respectively) and lungkine/CXCL15 (1271 ± 1120, 1744 ± 1636, and 1404 ± 1181 pg/mL, respectively) did not change significantly with ischemia or following I/R. These results are summarized in .
Figure 2 Error-bar diagrams show mouse serum ELR+ CXC chemokine concentrations in each surgical group (sham, ischemia, or ischemia-reperfusion). The results are depicted as means±SEM. (a) KC/CXCL1, (b) MIP-2/CXCL2/3, (c) LIX/CXCL5, (d) lungkine/CXCL15.*** signifies p < .001,* signifies p < .05.
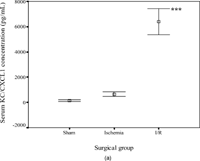
The concentrations of KC/CXCL1 and MIP-2/CXCL2/3 correlated positively with the degree of mucosal injury (KC/CXCL1: Spearman's r = 0.791, p < .001; MIP-2/CXCL2/3: r = 0.710, p = .003). LIX/CXCL5 concentrations were negatively correlated (r = −0.555, p = .032), whereas lungkine/CXCL15 concentrations were not significantly related to injury scores. The serum concentrations of KC/CXCL1 and MIP-2/CXCL2/3 correlated positively (r = 0.754, p < .001) with each other, but there was no relationship with the other two chemokines.
DISCUSSION
The pathophysiologic role of ELR+ CXC chemokines has received increasing recognition in I/R in several organ systems, including the kidney, central nervous system, liver, and myocardium [Citation[20–23]]. The focus of most of these studies, however, has remained primarily on IL-8/CXCL8, and the potential roles of other ELR+ CXC chemokines have not been evaluated. As murine I/R models lack a homologue for IL-8/CXCL8, these are well suited for investigating the role(s) of these other chemokines. In this study we have adapted an established rat model of intestinal ischemia wherein intestinal injury can be induced at 14–26 postnatal days, in mice [Citation[24]]. We chose a time point of 4 weeks in view of the limitations imposed by the smaller size and higher mortality in mouse pups. Even at this age, we were still somewhat limited by the number of serial blood samples that could have been drawn. In our study, the animals in the I/R group were sampled at 150 min from SMA occlusion (compared with 60 min in the sham and ischemia groups). Therefore, the time elapsed since SMA clamping also could have influenced chemokine levels in the “reperfusion” subgroup.
In our study, of the 4 ELR+ CXC chemokines, only KC/CXCL1 and MIP-2/CXCL2/3 showed a direct relationship with the development of mucosal lesions. Serum LIX/CXCL5 concentrations, on the contrary, correlated inversely with the severity of intestinal injury. These findings are at a slight variance to observations in I/R in other organs. In the liver, for instance, KC/CXCL1 transcription may be delayed after reperfusion as compared with MIP-2/CXCL2/3 [Citation[31]]. In myocardial I/R injury in rat, LIX/CXCL5 is induced early on along with MIP-2/CXCL2/3, while KC/CXCL1 expression is delayed [Citation[23]]. The expression and induction kinetics of KC/CXCL1, MIP-2/CXCL2/3 and LIX/CXCL5 differ in various tissues, possibly due to distinct regulatory pathways [Citation[32]]. There are also known differences in the potency of NF-κB activation for increasing the expression of each of these chemokines, and in their sensitivity to suppression by glucocorticoids [Citation[32], Citation[33]]. These observations, in conjunction with our findings of differential expression patterns within the ELR+ CXC chemokine subfamily, suggest that these chemokines might have different biological roles during I/R [Citation[32]].
It is also possible that these differences in chemokine concentrations might be due to differences in the triggers for expression, or their potency, in the cells producing these mediators. KC/CXCL1 and MIP-2/CXCL2/3 are produced primarily by the cells of monocyte-macrophage lineage, whereas LIX/CXCL5 and lungkine/CXCL15 are expressed more by fibroblasts and epithelial cells [Citation[30], Citation[34–36]]. Last, gene transcription for LIX/CXCL5 can have a delayed peak in certain experimental models such as acute endotoxinemia [Citation[32]], which raises the possibility that a late-onset rise in LIX/CXCL5 levels could have been missed in our experiment by sacrificing the animals immediately after the procedure. However, even with a delayed peak, the onset of LIX/CXCL5 transcription begins early in these models, which would not be consistent with the negative trend in serum LIX/CXCL5 concentrations observed in our experiment. This area needs further study, with measurement of chemokine mRNA and protein expression in the involved tissues.
There were no obvious patterns in serum lungkine/CXCL15 concentrations. The information on lungkine/CXCL15 is scant in the literature, as it has been described only recently [Citation[30]]. Its expression is developmentally regulated in the lung, and it might possibly play a role in pulmonary maturation [Citation[30]]. Its role, however, in inflammation in other tissues is not yet known.
The effect of intestinal I/R on distant organs such as the lung and liver needs to be explored further. Intestinal I/R often leads to secondary lung injury due to neutrophil sequestration and increased microvascular permeability and can be associated with adult respiratory distress syndrome [Citation[37], Citation[38]]. These changes have been postulated to be secondary to the transhepatic passage of portal venous blood from ischemic intestine, resulting in hepatic Kupffer cell activation and cytokine secretion [Citation[38]]. Similar changes also have been described in the kidney, where intestinal I/R might lead to renal tubular dysfunction and a reduction in renal blood flow [Citation[39]]. It is, therefore, very likely that the circulating ELR+ CXC chemokine concentrations are an expressed sum of the cytokine upregulation in various organs.
Selective targeting of neutrophil chemoattractant chemokines (or their receptors) is an attractive strategy for reducing I/R related injury. Further studies are needed to establish the relationship(s) of the tissue concentrations of these chemokines with the degree of neutrophil infiltration and the severity of I/R induced damage.
REFERENCES
- Hassoun HT, Zou L, Moore FA, Kozar RA, Weisbrodt NW, Kone BC. Alpha-melanocyte-stimulating hormone protects against mesenteric ischemia-reperfusion injury. Am J Physiol Gastroint Liver Physiol. 2002; 282: G1059–1068. [CSA]
- Deeg KH, Gerstner R, Bundscherer F, Harai G, Singer H, Gutheil H. [Doppler sonographic detection of reduced flow velocity in the celiac trunk of the newborn infant with patent ductus arteriosus Botalli compared to a healthy control group]. Monatsschr Kinderheilkd. 1987; 135: 24–29. [PUBMED], [INFOTRIEVE]
- Fellman V, Raivio KO. Reperfusion injury as the mechanism of brain damage after perinatal asphyxia. Pediatr Res. 1997; 41: 599–606. [PUBMED], [INFOTRIEVE], [CSA]
- Stechmiller JK, Treloar D, Allen N. Gut dysfunction in critically ill patients: a review of the literature. Am J Crit Care. 1997; 6: 204–209. [PUBMED], [INFOTRIEVE]
- Dyess DL, Peeples GL, Ardell JL, et al, Indomethacin-induced blood flow distribution in premature and full-term piglets. J Pediatr Surg. 1993; 28: 1396–1400. [PUBMED], [INFOTRIEVE]
- Langer JC, Sohal SS, Mumford DA. Mucosal permeability in the immature rat intestine: effects of ischemia-reperfusion, cold stress, hypoxia, and drugs. J Pediatr Surg. 1993; 28: 1380–1383, discussion 1384–1385. [PUBMED], [INFOTRIEVE]
- Powell RW, Dyess DL, Collins JN, et al, Regional blood flow response to hypothermia in premature, newborn, and neonatal piglets. J Pediatr Surg. 1999; 34: 193–198. [PUBMED], [INFOTRIEVE], [CSA], [CROSSREF]
- Sileri P, Morini S, Schena S, et al, Intestinal ischemia/reperfusion injury produces chronic abnormalities of absorptive function. Transplant Proc. 2002; 34: 984, [PUBMED], [INFOTRIEVE], [CSA], [CROSSREF]
- Clark DA, Fornabaio DM, McNeill H, Mullane KM, Caravella SJ, Miller MJ. Contribution of oxygen-derived free radicals to experimental necrotizing enterocolitis. Am J Pathol. 1988; 130: 537–542. [PUBMED], [INFOTRIEVE]
- Hsueh W, Caplan MS, Tan X, MacKendrick W, Gonzalez-Crussi F. Necrotizing enterocolitis of the newborn: pathogenetic concepts in perspective. Pediatr Dev Pathol. 1998; 1: 2–16. [PUBMED], [INFOTRIEVE], [CSA], [CROSSREF]
- Zhao H, Montalto MC, Pfeiffer KJ, Hao L, Stahl GL. Murine model of gastrointestinal ischemia associated with complement-dependent injury. J Appl Physiol. 2002; 93: 338–345. [PUBMED], [INFOTRIEVE]
- Musemeche C, Caplan M, Hsueh W, Sun X, Kelly A. Experimental necrotizing enterocolitis: the role of polymorphonuclear neutrophils. J Pediatr Surg. 1991; 26: 1047–1049, discussion 1049–1050. [PUBMED], [INFOTRIEVE], [CROSSREF]
- Kubes P, Hunter J, Granger DN. Ischemia/reperfusion-induced feline intestinal dysfunction: importance of granulocyte recruitment. Gastroenterology. 1992; 103: 807–812. [PUBMED], [INFOTRIEVE]
- Yoshidome H, Lentsch AB, Cheadle WG, Miller FN, Edwards MJ. Enhanced pulmonary expression of CXC chemokines during hepatic ischemia/reperfusion-induced lung injury in mice. J Surg Res. 1999; 81: 33–37. [PUBMED], [INFOTRIEVE], [CSA], [CROSSREF]
- Tsuruma T, Yagihashi A, Tarumi K, Hirata K. Anti-rat IL-8 (CINC) monoclonal antibody administration reduces ischemia-reperfusion injury in small intestine. Transplant Proc. 1998; 30: 2644–2645. [PUBMED], [INFOTRIEVE], [CSA], [CROSSREF]
- Rudack C, Jorg S, Sachse F. Biologically active neutrophil chemokine pattern in tonsillitis. Clin Exp Immunol. 2004; 135: 511–518. [PUBMED], [INFOTRIEVE], [CSA], [CROSSREF]
- Turler A, Kalff JC, Heeckt P, et al, Molecular and functional observations on the donor intestinal muscularis during human small bowel transplantation. Gastroenterology. 2002; 122: 1886–1897. [PUBMED], [INFOTRIEVE], [CSA], [CROSSREF]
- Wuyts A, Struyf S, Gijsbers K, et al, The CXC chemokine GCP-2/CXCL6 is predominantly induced in mesenchymal cells by interleukin-1beta and is down-regulated by interferon-gamma: comparison with interleukin-8/CXCL8. Lab Invest. 2003; 83: 23–34. [PUBMED], [INFOTRIEVE], [CSA]
- Remick DG, Green LB, Newcomb DE, Garg SJ, Bolgos GL, Call DR. CXC chemokine redundancy ensures local neutrophil recruitment during acute inflammation. Am J Pathol. 2001; 159: 1149–1157. [PUBMED], [INFOTRIEVE]
- De Vries B, Matthijsen RA, Wolfs TG, Van Bijnen AA, Heeringa P, Buurman WA. Inhibition of complement factor C5 protects against renal ischemia-reperfusion injury: inhibition of late apoptosis and inflammation. Transplantation. 2003; 75: 375–382. [PUBMED], [INFOTRIEVE], [CSA], [CROSSREF]
- Popivanova BK, Koike K, Tonchev AB, et al, Accumulation of microglial cells expressing ELR motif-positive CXC chemokines and their receptor CXCR2 in monkey hippocampus after ischemia-reperfusion. Brain Res. 2003; 970: 195–204. [PUBMED], [INFOTRIEVE], [CROSSREF]
- Lentsch AB, Kato A, Yoshidome H, McMasters KM, Edwards MJ. Inflammatory mechanisms and therapeutic strategies for warm hepatic ischemia/reperfusion injury. Hepatology. 2000; 32: 169–173. [PUBMED], [INFOTRIEVE], [CROSSREF]
- Chandrasekar B, Smith JB. Freeman GL. Ischemia-reperfusion of rat myocardium activates nuclear factor-KappaB and induces neutrophil infiltration via lipopolysaccharide-induced CXC chemokine Circulation. 2001; 103: 2296–2302
- Musemeche CA, Baker JL, Feddersen RM. A model of intestinal ischemia in the neonatal rat utilizing superior mesenteric artery occlusion and intraluminal platelet-activating factor. J Surg Res. 1995; 58: 724–727. [PUBMED], [INFOTRIEVE], [CSA], [CROSSREF]
- Dimmitt RA, Glew R, Colby C, Brindle M, Skarsgard E, Moss RL. Serum cytosolic beta-glucosidase activity in a rat model of necrotizing enterocolitis. Pediatr Res. 2003; 54: 462–465. [PUBMED], [INFOTRIEVE], [CSA], [CROSSREF]
- Oquendo P, Alberta J, Wen DZ, Graycar JL, Derynck R, Stiles CD. The platelet-derived growth factor-inducible KC gene encodes a secretory protein related to platelet alpha-granule proteins. J Biol Chem. 1989; 264: 4133–4137. [PUBMED], [INFOTRIEVE]
- Bozic CR, Kolakowski LF, Jr., Gerard NP, et al, Expression and biologic characterization of the murine chemokine KC. J Immunol. 1995; 154: 6048–6057. [PUBMED], [INFOTRIEVE]
- Endlich B, Armstrong D, Brodsky J, Novotny M, Hamilton TA. Distinct temporal patterns of macrophage-inflammatory protein-2 and KC chemokine gene expression in surgical injury. J Immunol. 2002; 168: 3586–3594. [PUBMED], [INFOTRIEVE]
- Chandrasekar B, Melby PC, Sarau HM, et al, Chemokine-cytokine cross-talk. The ELR + CXC chemokine LIX (CXCL5) amplifies a proinflammatory cytokine response via a phosphatidylinositol 3-kinase-NF-kappa B pathway. J Biol Chem. 2003; 278: 4675–4686. [PUBMED], [INFOTRIEVE], [CROSSREF]
- Rossi DL, Hurst SD, Xu Y, et al, Lungkine, a novel CXC chemokine, specifically expressed by lung bronchoepithelial cells. J Immunol. 1999; 162: 5490–5497. [PUBMED], [INFOTRIEVE]
- Lentsch AB, Yoshidome H, Cheadle WG, Miller FN, Edwards MJ. Chemokine involvement in hepatic ischemia/reperfusion injury in mice: roles for macrophage inflammatory protein-2 and KC. Hepatology. 1998; 27: 1172–1177. [PUBMED], [INFOTRIEVE], [CROSSREF]
- Rovai LE, Herschman HR, Smith JB. The murine neutrophil-chemoattractant chemokines LIX, KC, and MIP-2 have distinct induction kinetics, tissue distributions, and tissue-specific sensitivities to glucocorticoid regulation in endotoxemia. J Leukoc Biol. 1998; 64: 494–502. [PUBMED], [INFOTRIEVE], [CSA]
- Chandrasekar B, Melby PC, Sarau HM, et al, Chemokine-cytokine cross-talk. The ELR + CXC chemokine LIX (CXCL5) amplifies a proinflammatory cytokine response via a phosphatidylinositol 3-kinase-NF-kappa B pathway. J Biol Chem. 2003; 278: 4675–4686. [PUBMED], [INFOTRIEVE], [CROSSREF]
- Introna M, Bast RC, Jr., Tannenbaum CS, Hamilton TA, Adams DO. The effect of LPS on expression of the early “competence” genes JE and KC in murine peritoneal macrophages. J Immunol. 1987; 138: 3891–3896. [PUBMED], [INFOTRIEVE]
- Smith JB, Herschman HR. Glucocorticoid-attenuated response genes encode intercellular mediators, including a new C-X-C chemokine. J Biol Chem. 1995; 270: 16756–16765. [PUBMED], [INFOTRIEVE], [CROSSREF]
- Wolpe SD, Sherry B, Juers D, Davatelis G, Yurt RW, Cerami A. Identification and characterization of macrophage inflammatory protein 2. Proc Natl Acad Sci USA. 1989; 86: 612–616. [PUBMED], [INFOTRIEVE], [CSA]
- Johnston TD, Fischer R, Chen Y, Reed RL, 2nd. Lung injury from gut ischemia: insensitivity to portal blood flow diversion. J Trauma. 1993; 35: 508–511. [PUBMED], [INFOTRIEVE]
- Tullis MJ, Brown S, Gewertz BL. Hepatic influence on pulmonary neutrophil sequestration following intestinal ischemia-reperfusion. J Surg Res. 1996; 66: 143–146. [PUBMED], [INFOTRIEVE], [CSA], [CROSSREF]
- Lai IR, Ma MC, Chen CF, Chang KJ. The effect of an intestinal ischemia-reperfusion injury on renal nerve activity among rats. Shock. 2003; 19: 480–485. [PUBMED], [INFOTRIEVE], [CROSSREF]