ABSTRACT
Shoots of Calluna vulgaris, Erica carnea, Juniperus communis subsp. nana, Picea abies, and Pinus mugo subsp. mugo covered with felty, melanized epiphytic mycelia typical for brown felt blight caused by Herpotrichia pinetorum were collected at several locations in the Swiss Alps. Most cultures prepared from the mycelia on J. communis subsp. nana and P. abies were H. pinetorum, whereas the majority of cultures from P. mugo subsp. mugo and C. vulgaris were identified by internal transcribed spacer ITS1-5.8S-ITS2 sequencing and morphology as Allantophomopsis cytisporea. The fungus tolerates low temperatures, has an optimum between 16°C and 24°C, and ceases to grow at 28°C. 35°C is lethal. A. cytisporea is known as the causal agent of cranberry black rot on Vaccinium macrocarpon but has never been described as a snow mold. A. cytisporea is an endophyte in C. vulgaris but seems able to kill leaflets and whole shoots during winter. The epiphytic mycelium can expand from C. vulgaris to neighboring shoots of P. mugo subsp. mugo and J. communis subsp. nana below the snow where it forms epiphytic mycelial mats reminiscent of H. pinetorum. H. pinetorum has a strong antibiotic effect against A. cytisporea at 4°C and 20°C, whereas A. cytisporea grows faster at these temperatures. The effects of climate change on the interaction between the two snow mold fungi and their consequences on regeneration of woody plants at timberline are discussed.
Introduction
Snow blight pathogens such as Herpotrichia pinetorum and Gremmenia (Phacidium) infestans affect natural regeneration of conifers in alpine, subalpine, and boreal zones and probably play a decisive role in determining conifer regeneration near timberline (Hartig Citation1888; Gäumann, Roth, and Anliker Citation1934; Roll-Hansen Citation1989). During a study about the population structure of H. pinetorum in the region of Arosa, Switzerland, three of more than eighty strains prepared from melanized mycelial mats covering shoots of juniper (Juniperus communis subsp. nana), dwarf mountain pine (Pinus mugo subsp. mugo), and Norway spruce (Picea abies) in the Swiss Alps were not H. pinetorum but a species of Allantophomopsis, indicating that H. pinetorum is the main but not the only fungus associated with brown felt blight (Schneider et al. Citation2009). Consequently, not all of the brown to black mycelial mats covering dead shoots of young conifers are due to colonization by H. pinetorum. This observation was the starting point for the present study. Melanized, superficial mycelia on J. communis subsp. nana, P. mugo subsp. mugo, P. abies, Erica carnea (winter heath), and Calluna vulgaris (common heather) were collected during snowmelt at different locations in the alpine zone in Switzerland over several years. C. vulgaris was included because this plant species and its accompanying vegetation close to melting snow patches in the alpine zone were repeatedly observed to be covered by mats of felty gray brown mycelia reminiscent of H. pinetorum ( and ). Whereas the brown mycelial mats formed on conifers by H. pinetorum are visible to the naked eye mostly during the whole vegetation period, the mats on C. vulgaris and E. carnea are macroscopically visible for a very short period of time only after the snow melts away (). Recognition of the mats is best right at the edge of the retreating snow cover in spring.
Table 1. Sampling sites, collection dates and plant species collected.
Figure 1. (a) The San Bernardino site (1930 m a.s.l.) during snowmelt on 8 June 2014; arrowheads indicate places where felty brown to black mycelial mats of Allantophomospsis cytisporea as shown in (b), (c), and (d) can be observed. (b) Mycelial mat right at the edge of melting snow. (c) Mycelial mat covering horsts of dead leaves of the grass Nardus stricta. (d) Mycelial mat covering Calluna vulgaris. (e) Shoot blight on C. vulgaris observed at the San Bernardino site on 24 August 2014. (f) Close-up of (e).
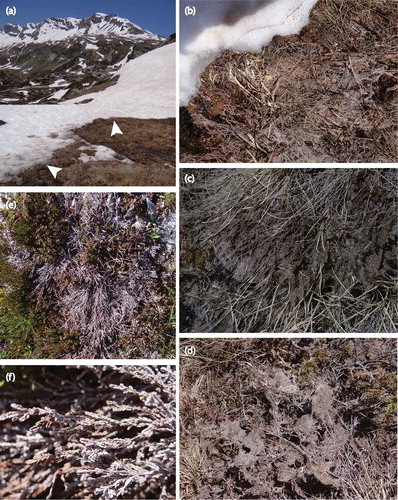
The aims of this study were to (1) identify the fungi forming the melanized mycelial mats, (2) describe the fungus most frequently found besides H. pinetorum, (3) determine its temperature requirements for growth, and (4) identify the most closely related fungal species. The ecology of the fungus and its possible significance in the context of climate change are discussed.
Materials and methods
Isolates
Shoots of C. vulgaris, E. carnea, J. communis subsp. nana, P. abies, and P. mugo subsp. mugo covered with brown to black superficial mycelia reminiscent of brown felt blight caused by H. pinetorum and H. coulteri were collected during snowmelt at the edge of retreating snow patches at different locations between 1,800 and 2,400 meters above sea level (m.a.s.l.) in the Alps between 2002 and 2015 ( and ). Shoots were stored at 4°C and processed within 72 hours. Small tissue samples covered by epiphytic mycelium were incubated on water agar (15 gl−1 agar, 50 mgl−1 terramycine [oxytetracycline]) at 4°C for at least 5 weeks. At least three replicates were prepared per collection. Some dead leaves of C. vulgaris from shoots covered with melanized mycelium were dipped into 96 percent ethanol for 1 minute prior to incubation to reduce the number of living microbes on the leaf surface. Single hyphal tip cultures were prepared from emerging melanized mycelia on malt extract agar (MEA; 20 gl−1 malt extract, 15 gl−1 agar). The three Allantophomopsis strains (A_AR_1_06, A_AR_2_06, A_AR_3_06) isolated by Schneider et al. (Citation2009) were also included ().
Table 2. Allantophomopsis strains isolated from mycelial mats covering shoots of various plant species at various sites in the Swiss Alps during snow melt.
Growth rates and temperature requirements
Growth rates (mmd−1) of 16 Allantophomopsis cytisporea strains were determined on MEA at 20°C (). The growth increment was measured every 2 to 4 days along two perpendicular axes through the inoculum of each of two colonies per isolate to determine the mean daily growth rate. In addition, strains A_AR_1_06 and A_SB_5_02 were used to study the temperature requirements. Growth rates of these strains were examined at 0, 4, 8, 12, 16, 20, 24, 28, 32, and 36°C on MEA.
Morphology
Conidial morphology was studied for eight selected A. cytisporea strains. Conidia were examined both in water and in concentrated lactic acid and observed under phase contrast optics using a Zeiss Axiophot microscope. Ordinary fountain pen ink (Pelikan, blue) was used to visualize conidial appendages.
Mycelial interaction between Allantophomopsis cytisporea and Herpotrichia pinetorum
Interactions between each of three A. cytisporea isolates (A_AR_1_06, A_DA_21_15, A_SB_7_12) and H. pinetorum (73-Fo, 18a, 95-Fi; Schneider et al. Citation2009) strains were tested on MEA at 4°C and 20°C. Colonized 5-mm-diameter plugs from the margin of fresh colonies on MEA were placed at a distance of 30 mm from each other on MEA and incubated. The progress of mycelial growth was monitored every 2 to 5 days.
Sequencing the internal transcribed spacer
For DNA extraction, aerial mycelium was harvested directly from single hyphal tip culture after 12 weeks of incubation at 4°C and lyophilized. DNA was extracted using the NucleoSpin 96 Plant II Kit (MACHEREY-NAGEL, Düren, Germany) according to the manufacturer’s protocol. Nucleotide sequences of the internal transcribed spacer ITS1-5.8S-ITS2 region were determined as described previously using primers ITS1 and ITS4 (White et al. Citation1990; Ibrahim, Sieber, and Schlegel Citation2017). Amplification of DNA was performed in 20 µl reaction volumes using approximately 2 ng of template DNA. After an initial denaturation step for 2 minutes at 94°C, thirty-five cycles were performed each consisting of a denaturation step at 94°C for 30 seconds, an annealing step at 60°C for 1 minute, and an extension step at 72°C for 1 minute, followed by a final extension step for 10 minutes at 72°C. Polymerase chain reaction (PCR) products were directly purified using the Montage PCR Life Science Kit (Millipore Corporation, Bedford, MA) following the manufacturer’s instructions. Sequencing of purified PCR products was performed by Microsynth (Balgach, Switzerland). The sequences were BLASTed against sequences deposited in GenBank to find the most closely matching ones. Closely related species were included in phylogenetic analysis to show the relative position of the collected strains among strains found by other authors (). Maximum parsimony analyses were performed in PAUP 4.0a. Bootstrapping to generate 100 pseudosamples was used for accuracy estimations. Gremmenia (Phacidium) infestans (U92305) served as the outgroup.
Table 3. Collection details and GenBank accession numbers of isolates included in the phylogenetic analysis.
Results
Brown to black superficial mycelial mats were found on all the five plant species examined. Whereas the mats remained clearly visible on J. communis subsp. nana, P. abies. and P. mugo subsp. mugo throughout the vegetation period, discovery of mycelial mats on E. carnea and C. vulgaris was pure coincidence because, during dry weather, the mats were conspicuous only for a few hours after the snow cover had melted (–). The exposed mycelial mats on these ericaceous plants dried and collapsed and the hyphae were torn apart, and the presence of epiphytic hyphae could only be recognized using magnification glasses. Conspicuous gray patches consisting of dead shoots of C. vulgaris or living shoots bearing dead leaves were often observed in spring and summer. A closer look at these shoots revealed the presence of mycelial fragments indicative of the former presence of mycelial mats (, ). In addition, examination of leaves under the light microscope showed presence of melanized, septate mycelium on and within the leaves (). Incubation of such leaves usually led to the emergence of A. cytisporea.
Figure 2. (a) Mycelium of Allantophomopsis cytisporea colonizing dead tissue of a Calluna vulgaris leaf (scale bar: 20 µm); the bright meandering lines are the cell walls of the puzzle piece–like epidermal cells of C. vulgaris. (b) Interaction between Herpotrichia pinetorum (strain 95-Fi; Schneider et al. Citation2009) to the left and A. cytisporea (strain A_DA_21_15) to the right after 21 days at 4°C on MEA. Conidia of A. cytisporea strains (c) A_SB_8_12 and (d) A_SB_6_12 in H2O stained with fountain pen ink (Pelikan, blue) and observed using phase contrast optics (scale bar: 10 µm); arrowheads indicate the slimy appendages of the conidia.
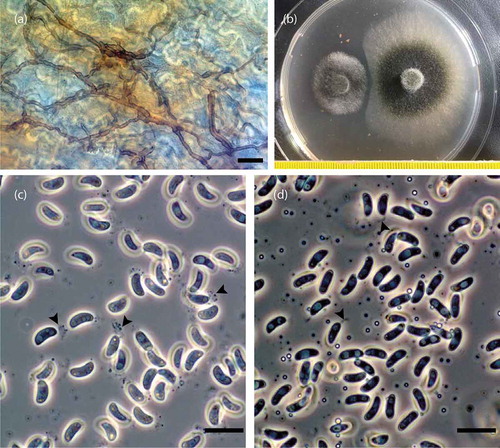
Melanized mycelia that grew comparatively well at 4°C could be isolated from most samples. Most of these isolates grew fast at 20°C on MEA (5.5–7.5 mmd−1), forming olive gray colonies without or with sparse aerial mycelium (). The ITS sequences of a majority of these isolates were identical, and nonidentical sequences differed by maximally 0.6 percent (). The ITS sequences differed by maximally 0.6 percent from that of an Allantophomopsis lunata ex-type isolate (NR132922), by up to 1.7 percent from that of an A. cytisporea ex-type isolate (KJ663830), and by up to 1.2 percent from that of an Allantophomopsis lycopodina isolate (AB041243). Thus, based on DNA sequences of the ITS regions, at least three different species names are possible for the Allantophomopsis species isolated during this study: A. lycopodina, A. cytisporea, and A. lunata.
Figure 3. The most parsimonious tree showing the phylogenetic relationships among snow mold strains collected for this study (bold) and related species as inferred from ITS1-5.8s-ITS2 (489 character states including gaps) sequences. The scale bar shows the number of changes, and bootstrap support values of greater than 50 percent from 100 replicates are shown at the nodes. Taxon names are preceeded by GenBank accession numbers. The species boundaries are delimited with colored blocks. The tree was rooted to Gremmenia (Phacidium) infestans (GenBank accession U92305).
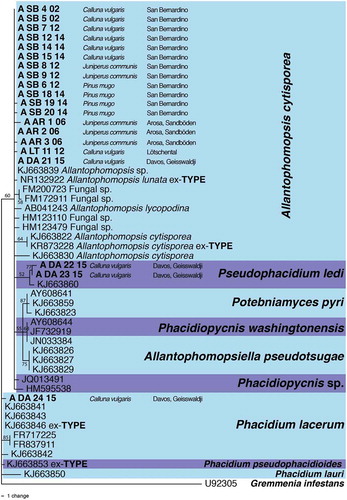
Fertile pycnidia developped within 4 to 8 weeks of cultivation. Conidial dimensions did not differ significantly among strains (4.9–7.8 × 1.9–3.0 µm; mean: 6.2 × 2.5 µm) and corresponded well with those of the conidia of A. cytisporea (6–8 × 2–2.5 µm; Carris Citation1990) and A. lunata (6–9 × 2–3.5 µm; Nag Raj Citation1993) (, ). The conidia of A. lycopodina did not fit because these are distinctly longer and measure (7–)8–15(–17) × 2–3.5 µm (mean: 11.2 × 2.9 µm; Carris Citation1990). Thus, the snow mold strains isolated during this study are referred to as A. cytisporea because A. cytisporea and A. lunata are considered synonymous, with A. cytisporea being the older name (Carris Citation1990; Nag Raj Citation1993).
Three snow mold isolates from Davos Geisswaldji (A_DA_22_15, A_DA_23_15, and A_DA_24_15) grew more slowly and produced olive gray colonies with a lot of aerial mycelium, and conidiomata never formed (). These three strains are closely related but not conspecific with A. cytisporea because, in addition to the lack of conidia formation in culture and different colony morphology, their ITS regions differed from those of all other A. cytisporea strains at several nucleotide positions. Strain A_DA_24_15 is most closely related to the ex-(neo)type strains of Phacidium lacerum and P. pseudophacidioides (Crous et al. Citation2015; ). The identity of the other two strains is less clear, but their ITS sequences were close to a sequence of Pseudophacidium ledi.
The ITS regions of Phacidiopycnis washingtonensis and Potebiamyces pyri, both known for causing postharvest rots on pome fruits (Xiao et al. Citation2005), and Allantophomopsiella pseudotsugae from dead bark and needles of conifers (Crous et al. Citation2014) are also closely related but formed reasonably well-supported clusters separate from the snow mold strains. In addition, the conidia of P. washingtonensis and P. pyri do not possess an apical appendage, and the conidia of P. pyri are distinctly larger (9.0–14.0 × 5.5–9.0 µm; Xiao et al. Citation2005).
Due to the unpredictability of the discovery of mycelial mats, the number of samples varied considerably among the individual plant species and sites (). Most strains from C. vulgaris and P. mugo subsp. mugo were A. cytisporea, with Herpotrichia pinetorum being the second most abundant species. In contrast, H. pinetorum dominated on J. communis subsp. nana and P. abies. Neither A. cytisporea nor H. pinetorum were found on E. carnea. Other fungi such as Cladosporium spp. and Mucor hiemalis were quite often isolated from the two ericaceous hosts. More rarely found fungi were Truncatella angustata (GenBank accession number: MK790184), Phaeosphaeria lycopodina (MK790186), Botrytis cinerea, and a species of Mycocentrospora (MK790185).
Table 4. Frequency (percentage) of incubated mycelial samples giving rise to Allantophomopsis cytisporea, Herpotrichia pinetorum, and other fungi.
A. cytisporea was isolated from J. communis subsp. nana at Arosa Sandböden (Schneider et al. Citation2009); from C. vulgaris, J. communis subsp. nana, and P. mugo subsp. mugo at San Bernardino; from C. vulgaris in the Lötschental; and at Davos Geisswaldji (). A. cytisporea was found on C. vulgaris at the San Bernardino site in all 3 years of collection (2002, 2012, and 2014). In contrast, A. cytisporea was not found at Arosa Maraner Hauptji, at Davos except at the Geisswaldji site, or at Göscheneralp.
Optimum temperature for growth of the collected A. cytisporea strains was between 16°C and 24°C. Growth rates were much higher than those of H. pinetorum even at 0°C and 4°C (growth rate of approximately 0.7 mmd−1 at 0°C; ). A. cytisporea did not grow at 28°C or higher, and temperatures 35°C and higher were lethal. Interaction of the two species on MEA at 4°C showed a strong antibiotic effect of H. pinetorum against A. cytisporea expressed by a distinct inhibition zone (). A similar effect was observed at 20°C. The type of interaction was always the same independent of the strain combination.
Discussion
Unequivocal species identification of the newly discovered snow molds by ITS sequencing was impossible, but they certainly belong to the Allantophomopsis cytisporea sensu lato species complex (Crous et al. Citation2015). A. lycopodina has distinctly longer conidia than those of the strains found in the present study. Thus, the snow mold fungus is either A. cytisporea, A. lunata, or a hitherto undescribed species. A. cytisporea and A. lunata are considered conspecific by some authors (Carris Citation1990; Nag Raj Citation1993). In fact, the ITS regions of the ex-type cultures of the two species differ only at a few nucleotide positions (99 percent similarity) and thus probably represent two operational taxonomic units of one and the same species (Schlegel, Queloz, and Sieber Citation2018). Therefore, the older name A. cytisporea is used here. A multigene phylogeny combined with morphological investigations on a larger and geographically more diverse collection of snow molds and closely related taxa, preferentially type specimens, would be required to decide whether the snow mold fungus is a new species. A. cytisporea is known from various ericaceous hosts and Pinus species and is often associated with cranberry (Vaccinium macrocarpon) black rot (Carris Citation1990; Nag Raj Citation1993; Olatinwo, Hanson, and Schilder Citation2003), which is a serious postharvest disease. C. vulgaris and J. communis subsp. nana can now be added to the list of hosts.
Extented networks of melanized mycelium of A. cytisporea can be observed in and on dead leaf tissue (). Interestingly, Pietrowski, Flessa, and Rambold (Citation2012) isolated an Allantophomopsis species as an endophyte from C. vulgaris leaves that possesses an ITS identical to that of A. cytisporea strain A_AR_1_06 isolated by Schneider et al. (Citation2009) from an epiphytic mycelial mat on J. communis subsp. nana reminiscent of H. pinetorum (, ). Similarly, Petrini (Citation1985) isolated A. cytisporea (as Apostrasseria lunata) as the second most frequent endophyte from healthy leaves of C. vulgaris. It can be speculated that A. cytisporea behaves as a harmless endophyte during the vegetation period but can kill leaves during winter. It probably is a seasonal parasite that profits from the weakness of the dormant host in winter. Under the snow, the mycelium emerges from dead leaves and can form huge mycelial mats, killing leaves and whole shoots of C. vulgaris. Adjacent vegetation is also attacked, including J. communis subsp. nana and P. mugo subsp. mugo. The fungus has been observed on several ericaceous species and conifers, but it has never been described as a snow mold or as the causal agent of a snow blight. Pathogenicity of A. cytisporea is assumed based on the observations made in the field but needs to be tested fulfilling Koch’s postulates.
Some Allantophomopsis species are asexual states of Phacidium species. Consequently, A. cytisporea belongs to the family Phacidiaceae. This family contains both pathogenic and saprotrophic species. Well-known pathogenic species are G. (Phacidium) infestans causing snow blight on various conifer species (Björkman Citation1942; Butin Citation2011), P. abietis causing snow blight on Abies balsamea (Smerlis Citation1962; Dicosmo, Raj, and Kendrick Citation1983), and P. coniferarum causing bark necrosis on Douglas fir, Japanese larch, pines, and firs (Smerlis Citation1968; Butin Citation2011). Allantophomopsis pusilla was observed on blackened stems of Rubus fruticosus in Germany (Nag Raj Citation1993), and Phacidiopycnis washingtonensis is described from diseased twigs and decayed fruits of Malus spp. (Xiao et al. Citation2005).
There are several possible reasons why A. cytisporea has so far been overlooked as a snow mold: (1) The mycelial mats on C. vulgaris are difficult to observe, because they remain visible only for a very short time after the snow has melted; (2) dark, superficial mycelia were regarded as H. pinetorum without further investigation; and (3) the frequency of the snow mold caused by A. cytisporea has increased in the course of climate change. Regarding reason 1: The detection of mycelial mats of A. cytisporea on C. vulgaris requires being at the right place at the right time and searching for snow molds on C. vulgaris, which apparently never had been performed previously. Regarding reason 2: In the area of San Bernardino, in summer, long after the snowmelt, branches of P. mugo subsp. mugo covered with dark mycelium mats were collected several times assuming that the causal agent would be H. pinetorum. Surprisingly, only A. cytisporea grew from a majority of these samples. Regarding reason 3: An increase in the course of climate change seems plausible, because the snow season now starts 12 days later and ends 26 days earlier on average than in 1970 at elevations between 1,100 and 2,500 m in the Swiss Alps (Klein et al. Citation2016). A. cytisporea and H. pinetorum have always been present in the ecosystem, but by shortening the duration of snow cover due to climate change, both fungi have less time to spread under the snow, leading to a reduction of their biomass. The biomass reduction probably has more serious consequences for H. pinetorum than for A. cytisporea, because H. pinetorum requires 100 percent relative air humidity for mycelium growth, a prerequisite that is only given in the boundary layer between ground and snow cover (Gäumann, Roth, and Anliker Citation1934). The growth requirements of A. cytisporea regarding air humidity are not known, but this fungus seems active as an endophyte in Ericaceae (Petrini Citation1985) during the vegetation period while H. pinetorum is dormant. The absence of a resting period and the use of C. vulgaris as a refuge could provide an advantage for A. cytisporea. Under a climate change scenario, the way in which H. pinetorum and A. cytisporea interact is likely to change, as has been shown for other fungi (A’Bear et al. Citation2013; Hiscox et al. Citation2016). Interestingly, the frequency of A. cytisporea seems to be higher on the southern side of the Alps than on the northern side. This could be due to the warmer climate on the southern side of the Alps. If a more extended monitoring of A. cytisporea confirms the preference of A. cytisporea for higher temperatures (see ) under field conditions, it is expected that the frequency of this snow mold will further increase in the course of global warming. However, because global warming will affect all of the interacting organisms, the effects on the ecosystem level are impossible to predict. Climate change will certainly have a strong influence on the natural regeneration of woody plants, possibly with pronounced effects at timberline where inteaction with snow molds is significant. Therefore, the effect of increased temperature on virulence of A. cytisporea and the interaction between this fungus and H. pinetorum should be studied using inoculation experiments.
Acknowledgments
I thank Andrin Gross, Ottmar Holdenrieder, and Norbert Luschka for collecting snow mold samples and Anja Gall for lab assistance and sequencing of the ITS regions of lots of “snow mold” strains. I also express my gratitude to Ottmar Holdenrieder for reviewing the article and for his helpful comments. The Genetic Diversity Center of ETH Zürich provided excellent laboratory facilities for the molecular part of this work.
Disclosure statement
No potential conflict of interest was reported by the author.
References
- A’Bear, A. D., W. Murray, R. Webb, L. Boddy, and T. H. Jones. 2013. Contrasting effects of elevated temperature and invertebrate grazing regulate multispecies interactions between decomposer fungi. PloS One 8 (10):e77610. doi:10.1371/journal.pone.0077610.
- Björkman, E. 1942. Reinkulturversuche mit dem Schneeschüttepilz (Phacidium infestans Karst.). Svensk Botanisk Tidskrift 36 (2–3):108–23.
- Butin, H. 2011. Krankheiten der Wald- und Parkbäume, 319. Stuttgart: Ulmer.
- CABI. 2019. Index fungorum. Wallingford, Oxfordshire, United Kingdom. http://www.indexfungorum.org/names/names.asp.
- Carris, L. M. 1990. Cranberry black rot fungi: Allantophomopsis cytisporea and Allantophomopsis lycopodina. Canadian Journal of Botany 68 (10):2283–91. doi:10.1139/b90-291.
- Crous, P. W., L. M. Carris, A. Giraldo, J. Z. Groenewald, D. L. Hawksworth, M. Hernandez-Restrepo, W. M. Jaklitsch, M. H. Lebrun, R. K. Schumacher, J. B. Stielow, et al. 2015. The Genera of fungi - fixing the application of the type species of generic names - G 2: Allantophomopsis, Latorua, Macrodiplodiopsis, Macrohilum, Milospium, Protostegia, Pyricularia, Robillarda, Rotula, Septoriella, Torula, and Wojnowicia. IMA Fungus 6 (1):163–98. doi:10.5598/imafungus.2015.06.01.11.
- Crous, P. W., W. Quaedvlieg, K. Hansen, D. L. Hawksworth, and J. Z. Groenewald. 2014. Phacidium and Ceuthospora (Phacidiaceae) are congeneric: Taxonomic and nomenclatural implications. IMA Fungus 5 (2):173–93. doi:10.5598/imafungus.2014.05.02.02.
- Dicosmo, F., T. R. N. Raj, and B. Kendrick. 1983. Prodromus for a revision of the Phacidiaceae and related anamorphs. Canadian Journal of Botany 61 (1):31–44. doi:10.1139/b83-002.
- Gäumann, E., C. Roth, and J. Anliker. 1934. Ueber die Biologie der Herpotrichia nigra Hartig. Zeitschrift für Pflanzenkrankheiten und Pflanzenschutz 44 (3):97–116.
- Gernandt, D. S., F. J. Camacho, and J. K. Stone. 1997. Meria laricis, an anamorph of Rhabdocline. Mycologia 89:735–44.
- Han, J.-G., T. Hosoya, G.-H. Sung, and H.-D. Shin. 2014. Phylogenetic reassessment of hyaloscyphaceae sensu lato (Helotiales, Leotiomycetes) based on multigene analyses. Fungal Biology 118:150–167. doi: 10.1016/j.funbio.2013.11.004.
- Hartig, R. 1888. Herpotrichia nigra n. sp. Allgemeine Forst- und Jagdzeitung 64:15–17.
- Hiscox, J., G. Clarkson, M. Savoury, G. Powell, I. Savva, M. Lloyd, J. Shipcott, A. Choimes, X. A. Cumbriu, and L. Boddy. 2016. Effects of pre-colonisation and temperature on interspecific fungal interactions in wood. Fungal Ecology 21:32–42. doi:10.1016/j.funeco.2016.01.011.
- Ibrahim, M., T. N. Sieber, and M. Schlegel. 2017. Communities of fungal endophytes in leaves of Fraxinus ornus are highly diverse. Fungal Ecology 29:10–19. doi:10.1016/j.funeco.2017.05.001.
- Klein, G., Y. Vitasse, C. Rixen, C. Marty, and M. Rebetez. 2016. Shorter snow cover duration since 1970 in the Swiss Alps due to earlier snowmelt more than to later snow onset. Climatic Change 139 (3–4):637–49. doi:10.1007/s10584-016-1806-y.
- Koukol, O., M. Kolarik, Z. Kolarova, and P. Baldrian. 2012. Diversity of foliar endophytes in wind-fallen Picea abies trees. Fungal Diversity 54:69–77. doi: 10.1007/s13225-011-0112-2.
- Nag Raj, T. R. 1993. Coelomycetous anamorphs with appendage-bearing conidia, 1101. Waterloo, Ontario, Canada: Mycologue Publications.
- Okane, I., A. Nakagiri, and T. Ito. 2001. Identity of Guignardia sp inhabiting ericaceous plants. Canadian Journal of Botany 79:101–9. doi: 10.1139/b00-136.
- Olatinwo, R. O., E. J. Hanson, and A. M. C. Schilder. 2003. A first assessment of the cranberry fruit rot complex in Michigan. Plant Disease 87 (5):550–56. doi:10.1094/PDIS.2003.87.5.550.
- Petrini, O. 1985. Host specificity of endophytic fungi of some European Ericaceae. Botanica Helvetica 95 (2):213–38.
- Pietrowski, A., F. Flessa, and G. Rambold. 2012. Towards an efficient phenotypic classification of fungal cultures from environmental samples using digital imagery. Mycological Progress 11 (2):383–93. doi:10.1007/s11557-011-0753-2.
- Roll-Hansen, F. 1989. Phacidium infestans: A literature review. European Journal of Forest Pathology 19:237–50. doi:10.1111/efp.1989.19.issue-4.
- Schlegel, M., V. Queloz, and T. N. Sieber. 2018. The endophytic mycobiome of European ash and sycamore maple leaves - geographic patterns, host specificity and influence of ash dieback. Frontiers in Microbiology 9:2345. doi:10.3389/fmicb.2018.02345.
- Schneider, M., C. R. Grünig, O. Holdenrieder, and T. N. Sieber. 2009. Cryptic speciation and community structure of Herpotrichia juniperi, the causal agent of brown felt blight of conifers. Mycological Research 113:887–96. doi:10.1016/j.mycres.2009.04.008.
- Smerlis, E. 1962. Taxonomy and morphology of Potebniamyces balsamicola sp. nov. associated with a twig and branch blight of balsam fir in Quebec. Canadian Journal of Botany 40 (2):351–60. doi:10.1139/b62-034.
- Smerlis, E. 1968. Notes on Potebniamyces coniferarum. Canadian Journal of Botany 46 (10):1329–30. doi:10.1139/b68-177.
- U'ren, J. M., F. Lutzoni, J. Miadlikowska, and A. E. Arnold. 2010. Community analysis reveals close affinities between endophytic and endolichenic fungi in mosses and lichens. Microbial Ecology 60:340–53. doi:10.1007/s00248-010-9698-2
- Weber, R. W. S. 2011. Phacidiopycnis washingtonensis, cause of a new storage rot of apples in Northern Europe. Journal of Phytopathology 159 (10):682–86. doi:10.1111/j.1439-0434.2011.01826.x.
- White, T. J., T. D. Bruns, S. Lee, and J. Taylor. 1990. Amplification and direct sequencing of fungal ribosomal RNA genes for phylogenetics. In PCR protocols: A guide to methods and applications, ed. M. A. Innis, D. H. Gelfand, J. J. Sninsky, and T. J. White, 315–22. New York, NY: Academic Press, Inc., Harcourt Brace Jovanovich, Publishers.
- Xiao, C. L., J. D. Rogers, Y. K. Kim, and Q. Liu. 2005. Phacidiopycnis washingtonensis - a new species associated with pome fruits from Washington State. Mycologia 97 (2):464–73.
- Yuan, Z.-L., L.-B. Rao, Y.-C. Chen, C.-L. Zhang, and Y.-G. Wu. 2011. From pattern to process: species and functional diversity in fungal endophytes of Abies beshanzuensis. Fungal Biology 115:197–213. doi: 10.1016/j.funbio.2010.11.002.
- Zifcakova, L., P. Dobiasova, Z. Kolarova, O. Koukol, and P. Baldrian. 2011. Enzyme activities of fungi associated with Picea abies needles. Fungal Ecology 4:427–36.