ABSTRACT
Planktic diatom ecology in the mountain lakes of the northern European Alps has only been studied sparsely so far. To fill this knowledge gap, the driving parameters of planktic diatom assemblage composition in the euphotic zones of twenty lakes located between 955 and 2,060 m a.s.l. were assessed. The mean August water temperature, concentration of major ions, total phosphorous, and lake physical parameters explained significant amounts of variation within the diatom assemblages, as identified by redundancy analysis and consecutive backward selection. Cyclotella comensis was the most abundant taxon in these oligotrophic (<17 total phosphorus µg/L), phosphorous (P) limited, stratified study lakes, particularly when the calcium concentration was high (>35 mg/L). The results of generalized linear models and generalized additive models further revealed that August surface temperature, thermocline depth, and nitrate-N positively and significantly influenced C. comensis growth. These results shed light on the interplay between physical and chemical parameters as important drivers of C. comensis abundance in temperate mountain lakes. They may aid in the interpretation of past and the prediction of future climate-driven changes in planktic diatom composition in these lakes.
Introduction
Mountain lakes are often remote, and their catchments are therefore less affected by anthropogenic factors than the catchments of other lakes. This makes mountain lakes good sentinels for the study of environmental change (Catalan et al. Citation2013). Because long-term monitoring data on water properties are often lacking, temporal changes are usually inferred through abiotic and biotic remains in lake sediments (Smol, Birks, and Last Citation2002). In palaeolimnological studies, diatoms are well-established indicator organisms (Dixit et al. Citation1992; Scherer Citation2002) because their silica cell walls are preserved well in lake sediments and their characteristic cell structure allows for species identification by light microscopy (Scherer Citation2002). Diatoms have previously been used as indicators of pH, salinity, nutrient levels, mixing regimes, and light conditions (Flower and Battarbee Citation1983; Lotter et al. Citation1997, Citation1998; Curtis et al. Citation2009; Winder, Reuter, and Schladow Citation2009; Saros et al. Citation2016). However, interpretation of palaeolimnological results requires in-depth knowledge of the relationships between environmental factors and diatom distribution (Smol, Birks, and Last Citation2002).
A common approach to lake core analysis is the collection of training sets containing surface sediment samples with diatom assemblages representing the current state of lakes (e.g., Hall and Smol Citation1992). Though this approach has yielded valuable insights, it presents some limitations in terms of interpretation, because surface sediment samples commonly represent several years and many life cycles of diatoms, whereas chemical measurements are usually only taken once. Hence, the need for better integration of ecological information in paleoecology is increasingly acknowledged (Davidson et al. Citation2018).
Little is known about what controls the abundant planktic diatom Cyclotella comensis across ecological gradients (Saros and Anderson Citation2015). This species is an important component of many temperate lakes and shows pronounced reactions to climate change (Rühland, Paterson, and Smol Citation2008, Citation2015; Winder, Reuter, and Schladow Citation2009; Reavie et al. Citation2017). In terms of the distribution of this taxon, the interplay between environmental drivers such as lake temperature (Livingstone and Dokulil Citation2001), mixing patterns (Kraemer et al. Citation2015), nutrient composition, and turbidity (Whitehead et al. Citation2009) as well as competition with other planktic taxa (Jäger, Diehl, and Schmidt Citation2008; Stomp et al. Citation2011) are important.
Within the northern European Alps, only one comprehensive study investigating the ecology of planktic diatoms including C. comensis exists (Wunsam, Schmidt, and Klee Citation1995). The lakes sampled in that study were mostly located at elevations below 1,500 m a.s.l., although many lakes in the region are found at higher elevations. Therefore, the present study examined the ecological preferences of planktic diatom assemblages in twenty mountain lakes, with special emphasis on the ecology of C. comensis. This study was designed to survey lakes along an elevational gradient from 955 to 2,060 m a.s.l. Because the pH and total phosphorous (TP) gradients were short, it was hypothesized that changes in water temperature would influence planktic diatom assemblage composition along with concomitant changes in water chemistry (Reavie et al. Citation2017); that is, nitrate concentration and turbidity.
Study sites
The twenty study lakes are located in Bavaria (Germany) and Tyrol (Austria) along a longitudinal gradient of 220 km and a latitudinal gradient of 50 km ().
Figure 1. Geographical and zonal locations of the sampled lakes in the northern Alps in Austria and Germany. Background map based on Natural Earth data (Citation2020).
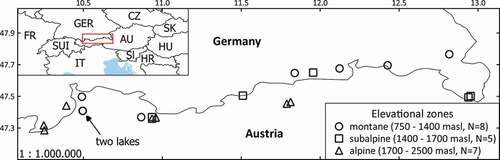
Most of the lakes were formed by cirque glaciers. Typically, they are small (<7 ha) and shallow (<20 m; ), consisting of one main basin. Two of the lakes are karstic; they are nearly round and have a smaller surface area–to-volume ratio than lakes of glacial origin. The elevation of the twenty lakes ranges from 955 to 2,060 m.a.s.l. (). Eight of the lakes are located in montane forest (750–1,400 m a.s.l.), five are located in subalpine forest (1,400–1,700 m a.s.l.), and seven are located in alpine meadows and rock basins (1,700–2,500 m a.s.l.). In the study region, the montane forest is dominated by beech (Fagus sylvatica), spruce (Picea abies), and maple (Acer pseudoplatanus), whereas the subalpine forest mainly consists of spruce and—at the ecotone between forest and bare meadows—pine (Pinus mugo ssp. mugo). In alpine meadows, shrubs (e.g., Rhododendron hirsutum), sedge meadows (e.g., Carex sempervirens), and fellfields predominate.
Table 1. Descriptive metrics of the measured and calculated environmental variables of the twenty-lake set
Methods
Sampling and laboratory procedures
The twenty lakes were sampled twice during the ice-free period, once between June and the middle of August and once between August and November 2016. On the first sampling date, bathymetric measurements were taken with an echo sounder (Lawrence HDS8, Oslo, Norway), and a buoy fixed with a rope on a stone was subsequently installed at the deepest point of each lake. Temperature loggers (Onset Pendant UA-001-64 HOBO, Bourne, MA) were mounted on the rope 0.5 m above the sediment surface and 0.5 m below the water surface. Temperature data were recorded at 30-minute intervals between the first and second sampling dates. On both sampling dates, physical parameters (temperature, oxygen saturation, pH, and conductivity [at 25°C]) were measured with a multiprobe (WTW 350, Weilheim, Germany) at 1-m intervals from the deepest point of each lake.
After measuring the Secchi depth of each lake, 0.5 L of a mixed water sample was collected with a hose sampler from the euphotic zone (ZEuph), which was defined as ZEuph = 5 × ZSecchi0.5 (DIN Technical Committee Water Analysis Citation2015). One half of the water sample was filtered (0.45 μm) on-site to analyze dissolved ions and the remaining sample was left unfiltered to analyze the concentration of TP. Both samples were stored at 4°C for further processing in the laboratory. Another liter of water was taken from the euphotic zone with the hose sampler and preserved with Lugol’s solution to analyze planktic diatom communities (Throndsen Citation1978). At the end of the second sampling day, the temperature loggers, together with the stones, were retrieved from the lakes.
All chemical analyses were carried out in the laboratory of the Limnological Research Station in Iffeldorf. Standard colorimetric methods were applied using a Hitachi 150-200 photometer (Chiyoda, Japan) to determine the concentrations of TP (Murphy and Riley Citation1962), nitrate-N (Navone Citation1964), ammonia-N (Deutsches Institut für Normung e.V. Citation1983), and silica (Deutsches Institut für Normung e.V. Citation1983). The concentrations of major ions (calcium, magnesium, and sodium) were measured using a cation chromatograph (ICS-1100, Thermo Scientific, Waltham, MA).
Planktic diatom samples were concentrated with 0.45 µm syringe filters before further processing (Nixdorf et al. Citation2014). Diatoms were prepared according to van der Werff and Macan (Citation1955). To analyze the composition of the diatom samples, 500 valves (including broken valves representing more than half of a complete valve) were identified in each case using a Leica DNM microscope at 1,000× magnification. Taxa were counted at the species level. Standard literature was used for identification (Krammer and Lange-Bertalot Citation1991a, Citation1991b, Citation1997a, Citation1997b; Krammer Citation2000, Citation2002; Lange-Bertalot Citation2001; Lange-Bertalot et al. Citation2017).
Data analysis
The atomic N/P ratios in lake water were calculated to determine pelagic nutrient limitation status by using the dissolved inorganic nitrogen–TP ratio (Bergström Citation2010; Kolzau et al. Citation2014). Our data set included only concentrations of nitrate-N and ammonia-N; nitrite-N was not measured. Therefore, the real dissolved inorganic nitrogen–TP ratio is probably higher than the results suggest. However, because nitrite occurs only at very low amounts under aerobic conditions and the conditions in the euphotic zones of the sampled lakes were mostly aerobic, this bias is probably negligible. Moreover, all lakes were found to be phosphorous (P) limited and integration of nitrite-N would have shifted the N/P ratio further in this direction. The lake mixing regime was assessed by calculating thermocline depth and buoyancy frequency at the thermocline and within the epilimnion based on temperature profiles using the R package rLakeAnalyzer (Winslow et al. Citation2019). Sodium was excluded from further analysis because it could only be detected in a few samples.
With the obtained set of nineteen abiotic parameters, a Pearson correlation matrix with Bonferroni-adjusted probabilities was computed (Haynes Citation2013) using the R package corrplot (Wei and Simko Citation2017). For significantly correlated parameters, coefficients of linear regression models were included. To identify relationships among environmental variables, principal component analysis (PCA) was carried out with the data sets of nineteen variables (Terbraak and Prentice Citation1988). Before analysis, all environmental variables except pH and elevation were log transformed to correct for nonnormal distribution (pH is a log scale and elevation showed a normal distribution). Further, the parameters were normalized by subtraction of the mean and dividing the result by the standard deviation for each variable (Oksanen et al. Citation2018).
The share of species with planktic life cycles was higher than 10 percent in all assemblages. For all calculations, benthic or tychoplanktic species were excluded and relative abundances were calculated based on the sum of obligate planktic species (Table S2). Before numerical analysis, the data set was Hellinger transformed (Prentice Citation1980) and the obtained Hellinger distance matrices were used for PCA (Legendre and Gallagher Citation2001). Prior to constrained analysis, variance inflation factors were calculated for the environmental data with the R package usdm (Naimi et al. Citation2014). To evaluate collinearity problems, the function “vifcor” of the usdm package was applied. According to the obtained results, no variable had collinearity problems. To evaluate whether diatom distribution followed a linear or unimodal trend, detrended correspondence analysis (DCA) was applied (Hill and Gauch Citation1980). For gradient lengths less than 3, linear constrained methods are considered appropriate; for gradient lengths between 3 and 4, linear or unimodal methods can fit; for higher gradient lengths, unimodal methods should be used (Terbraak and Prentice Citation1988). The DCA gradient length was 3.51 for the first axis and 2.91 for the second axis. Based on the results of DCA and variance inflation factors, an initial redundancy analysis (RDA) with the fourteen initially measured variables was computed. The significance for the whole model and for the first and second RDA axes was assessed by means of a Monte Carlo permutation test with 999 permutations; all tests were significant (p < .01). For further evaluation of the importance of each single explanatory variable, backward selection was applied (Blanchet et al. Citation2008). With the selected variables, another RDA was performed. Because p values decrease and R2 values increase with the number of explanatory variables, adjusted model values (R2adj) were obtained through Bonferroni–Holm correction (Haynes Citation2013). All models and each of the first two axes were significant as inferred by Monte Carlo permutation tests (p < .01). The explanatory potential and significance value of each single variable were obtained by setting the variable as the sole explanatory variable with all other variables as covariables in another round of Monte Carlo permutation tests. All multivariate analyses were carried out with the R package vegan (Oksanen et al. Citation2018).
To evaluate the correlation between environmental variables and C. comensis, generalized linear models (GLMs) were applied. For parameters with a significant influence on C. comensis based on GLM results and for further assumed important ecological drivers, generalized additive models (GAMs) were applied using the R package gam (Hastie Citation2019). GAMs were used instead of GLMs when the responses of C. comensis to interacting parameters were assumed to be nonlinear. All data analysis was carried out using the free statistics software R v3.6.3 (R Core Team Citation2013).
Results
Physical and chemical features
The lakes were shallow (maximum depth 20.7 m, median depth 7.4 m) and small (<6.4 ha). Though most of the lakes can be assumed to stratify during summer (the sampling period), at least four of the lakes probably mix irregularly because they are very shallow (<4 m). All lakes are P limited and well buffered, as reflected by pH values between 8 and 9 and high concentrations of major ions (). The Secchi depths of the lakes ranged from 1.5 m to 11.3 m (median = 4.6 m). At seven sites, the Secchi depth was equal to the maximum lake depth; that is, light reached the bottom of the lake. Concentrations of alkaline earth ions reached 49.2 mg/L for Ca2+ and 18.4 mg/L for Mg2+. Accordingly, electrical conductivity averaged 251.5 µS/cm (reference temperature = 25°C). The maximum conductivity level, which was more than 500 µS/cm, was recorded in one of the karstic lakes (Sieglsee groß; see Table S1), which was strongly influenced by groundwater. Nitrate-N and TP concentrations were generally low, with a mean of 0.39 mg/L and 6 µg/L, respectively, classifying most lakes as oligotrophic according to Vollenweider (Citation1968). Based on N/P ratios, all lakes were P limited (N/P > 43; sensu Klausmeier et al. Citation2004) and all but two lakes (Engeratsgundsee and Rappensee; see Table S1) were co-limited by silica (Si/N < 1.12; sensu Brzezinski Citation1985). The ammonia-N concentration was low in most lakes (median 26.7 µg/L), but showed a wide range (3.8–126.9 µg/L), possibly due to oxygen depletion in the hypolimnion of a few lakes. The means of the average August surface and bottom water temperatures were 15.1°C and 9.4°C, respectively.
The chemical parameters of the twenty study lakes mainly varied along the elevation gradient, which was correlated with PCA axis 1 (λ = 4.59, 24.17 percent; ). Elevation was negatively correlated with conductivity, ammonia-N, nitrate-N, N/P ratio, August surface temperature, and Ca and Mg concentrations. It was positively correlated with pH and the Si/N ratio (Figure S1, Figure S2). The second PCA axis (λ = 3.97, 20.91 percent) was positively correlated with lake depth and Secchi depth but negatively correlated with August surface and bottom temperatures, thermocline depth, and buoyancy frequency. The positive correlation between lake depth and Secchi depth was highly significant and can be explained by seven lakes that had equal Secchi depths and maximum depths (Table S1).
Figure 2. Principal component analysis correlation biplot for nineteen environmental variables measured in twenty mountain lakes in the northern European Alps. Principal components 1 and 2 (PC1, PC2) are plotted. λ indicates eigenvalues, var indicates percentage of explained variance. For variable and lake abbreviations, see Table S1.
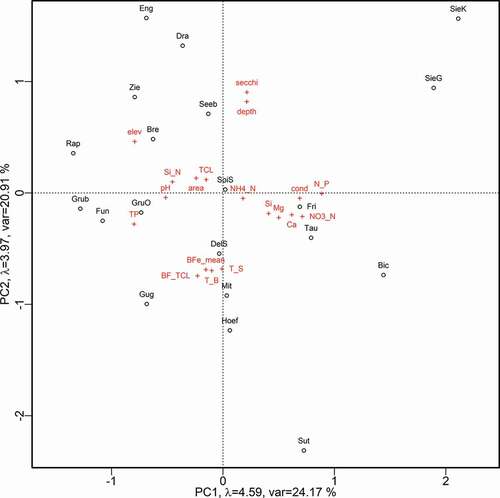
Bottom water temperature decreased significantly with maximum lake depth and Secchi depth and was positively correlated with surface water temperature. Buoyancy frequency at the thermocline and within the epilimnion were negatively correlated with Secchi depth, whereas thermocline depth was not correlated with any of the measured variables. Correlations among nutrients were significant for nitrate-N and ammonia-N (positive), nitrate-N and TP (negative), ammonia-N and Ca (positive), and nitrate-N and Ca (positive; Figure S1).
Diatom assemblages
Obligate planktic taxa occurred with relative abundances of 10.4 to 100 percent (median = 79.8 percent, n = 20; Table S2). Of the fourteen identified planktic taxa, Cyclotella comensis was the most abundant (33 percent of all planktic taxa, present in ten lakes, abundance range = 0.4–100 percent, median = 19 percent). Also abundant were Fragilaria nanana (21 percent), Fragilaria delicatissima (12 percent), Discostella woltereckii (10 percent), Fragilaria gracilis (8 percent), and Discostella stelligeroides (7 percent; Table S2).
Diatom–environment relationships
Variation in planktic taxa distribution was significantly influenced by the August surface temperature, lake area, Ca concentration, Secchi depth, Mg concentration, August bottom temperature, and TP, which explained 11.6, 7.6, 6.6, 5.3, 5.2, 5.1, and 4.6 percent of variation, respectively. The adjusted R2 value of the full RDA model was 37.8 percent ().
Table 2. Adjusted R² values for a planktic diatom RDA model with backward selected variables. Explained variance and p values were inferred from Monte Carlo permutation test
For the planktic diatom data set, the first constrained RDA axis (λ = 0.172, variance = 29.99 percent, p = .001) was negatively correlated with August surface and bottom temperatures and TP. The second axis (λ = 0.079, variance = 11.26 percent, p = .001) was negatively correlated with Mg concentration and positively correlated with Secchi depth and lake area (). The RDA biplot revealed a preference of C. comensis for warm, calcium-rich lakes, whereas Fragilaria nanana showed the opposite preference. Discostella woltereckii was abundant in lakes with high Secchi depths, whereas Fragilaria delicatissima showed a preference for magnesium-rich waters ().
Figure 3. RDA correlation biplot of species scores and environmental parameters that significantly and independently influenced diatom taxon composition as determined by backward selection applied to RDA models for planktic diatom assemblages of twenty lakes. For variable, species, and lake abbreviations, see Table S1 and Table S2.
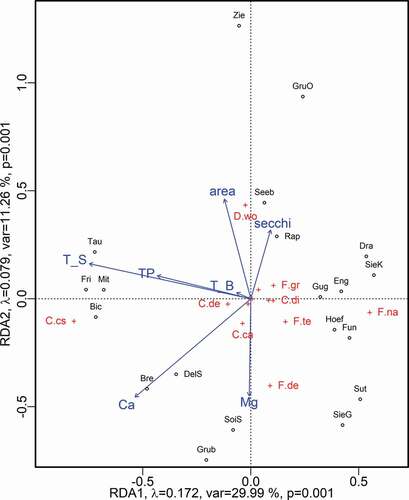
The results of the GLMs confirmed the tight coupling of C. comensis abundance and August surface temperature (p = .025, R2 = 0.66) and further revealed a significant positive correlation of thermocline depth on C. comensis abundance (p = .047, R2 = 0.41; ). None of the models including the other parameters significantly predicted C. comensis abundance; however, high relative abundances of C. comensis were found in samples with Ca values above 35 mg/L, Secchi depths of 4 to 5 m, and nitrogen-N levels of 0.5 to 1 mg/L. GLMs were also applied to all other taxa in the data set; however, no model identified a significant correlation between these taxa and any of the measured variables.
Figure 4. Relative abundances of Cyclotella comensis plotted against August surface temperature and thermocline depth. R2 values and p values are given for generalized linear models; model prediction curves are plotted for significant models (p < .05). For abbreviations and units, see .
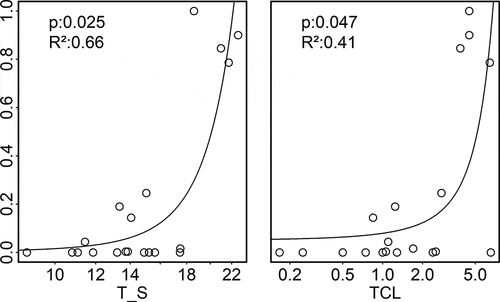
According to the GLM analysis, the relative abundance of C. comensis was significantly correlated with August surface temperature and thermocline depth. The GAMs also included nitrate-N because it was deemed to have an effect on C. comensis based on literature. For all three parameters, a significant GAM was found, with thermocline depth having the lowest Akaike information criterion (AIC) score and highest R2adj (). All combinations of parameters produced higher AIC scores, with August surface temperature and nitrate-N scoring lowest among all model combinations. The models suggested a pronounced increase in C. comensis abundance at lake surface temperatures above 18°C and nitrate-N values above 0.8 mg/L (). Cyclotella comensis was also particularly dominant in the planktic diatom assemblages when August surface water temperatures and nitrate-N concentrations were high ().
Table 3. Model coefficients for generalized additive models for abundance of cyclotella comensis in response to environmental variabels that proved to be important for Cyclotella comensis abundance based on GLM results and are known to be ecologically important factors influencing the taxon. For abbreviations see
Discussion
Cyclotella comensis occurred at high abundance in the plankton communities of alkaline montane lakes with high August surface temperatures (>18°C) and thermocline depths of 4 to 5 m. The lakes were P limited but rich in nitrogen (>0.8 mg/L), giving high N/P ratios. Further, most of the lakes had intermediate Secchi depths (4–5 m, range = 1.5–11.3 m). The ecology of C. comensis has been the subject of numerous studies involving the combination of palaeolimnological lake data and recent observations (Lotter and Bigler Citation2000), the compilation of training sets (Hall and Smol Citation1992; Wunsam, Schmidt, and Klee Citation1995; Werner and Smol Citation2006; Catalan et al. Citation2009; Curtis et al. Citation2009), investigations of in situ growth (Williamson et al. Citation2010a), and mesocosm experiments (Jäger, Diehl, and Schmidt Citation2008).
Apart from one study that found C. comensis under slightly acidic conditions (Battarbee Citation1984), this taxon prefers alkaline conditions with an optimum pH between 8 and 9 (Scheffler, Nicklisch, and Schonfelder Citation2005; Werner and Smol Citation2006; Saros and Anderson Citation2015). In line with this, all study lakes were alkaline in the present study (pH range = 7.9–9.0, median = 8.5), and C. comensis was especially abundant at the higher end of the Ca2+ gradient (35–49 mg/L; range = 15–49 mg/L, median = 27 mg/L).
Cyclotella comensis is generally rare at TP concentrations above ca. 10 µg/L (Hall and Smol Citation1992; Wunsam, Schmidt, and Klee Citation1995; Ramstack et al. Citation2003; Werner and Smol Citation2006; Saros et al. Citation2012; Saros and Anderson Citation2015). In the present study, the taxon occurred only in one lake with a TP concentration above 10 µg/L (range = 1–17 µg/L, median = 5 µg/L). The results are in line with those of the only other comprehensive study on lake diatom ecology within the study region, which identified a TP optimum of <15 µg/L for C. comensis (range = 2–266 µg TP/L; Wunsam, Schmidt, and Klee Citation1995).
Despite the insights into C. comensis ecology that the present results provide, the responses of this taxon to environmental changes remain unclear in some respects. In particular, the interaction of epilimnetic water temperature with C. comensis (and the Cyclotella sensu lato complex in general) remains controversial, because there are various examples of increasing abundances of these taxa with higher temperatures (e.g., the present study; Pienitz, Smol, and Birks Citation1995; Catalan, Pla, et al. Citation2002; Koinig et al. Citation2002), though there is also evidence for the opposite pattern (Weckstrom, Korhola, and Blom Citation1997). Various studies have noted that increases in C. comensis abundance with increasing water temperature may reflect indirect limnological factors, such as interactive effects between water temperature and thermocline depth (Catalan and Fee Citation1994; Huisman and Weissing Citation1995; Huisman, Van Oostveen, and Weissing Citation1999; Catalan, Pla et al. Citation2002). The results of the present study demonstrate that thermocline depth has a significant effect on the abundance of C. comensis (; GLM: p = .047, R2 = 0.41; GAM: p < .001, R2adj = 0.963, lowest AIC among all significant parameters). The thermocline depth in small lakes in turn depends on several factors, including water temperature (Catalan, Ventura, et al. Citation2002), turbidity (Sadro and Melack Citation2012; Strock et al. Citation2017), and fetch (France Citation1997). In our data set, none of the assessed variables, including mean August water temperature, were significantly correlated with thermocline depth (Figure S1). This finding indicates that multiple drivers may be relevant and that a single dominating driver of C. comensis abundance seems unlikely. However, turbidity and fetch were not assessed in the present study. Therefore, further research in these lakes should consider the importance of factors that control thermocline depth separately.
Interestingly, the results of the GAMs indicate interactive effects between thermocline depth, nutrients and C. comensis abundance (FTCL = 20.221, FNO3 = 6.774, pTCL < .001, pNO3 = .021, R2adj = 0.88, AIC = 228.7). The positive interactive effect of thermocline depth and nitrogen on C. comensis growth may be attributed to weaker phosphorous upcycling from the hypolimnion (Jager, Diehl, and Emans Citation2010); phosphorus is mostly released through internal hypolimnetic processes. Nitrogen input, in contrast, mainly comes from external sources (Rogora et al. Citation2018; Schwefel et al. Citation2019). It was found that an increase in the N/P ratio can select for small centrics that have competitive advantages due to more effective nutrient uptake mechanisms (Tolotti et al. Citation2007, Citation2012; Malik, Northington, and Saros Citation2017). In experiments in which light and nutrient concentrations were varied, Cyclotella bodanica and C. radiosa showed especially high growth rates under P limitation and moderate to low light conditions but required sufficient nitrogen; that is, their growth declined under co-limitation of nitrogen (Malik, Northington, and Saros Citation2017). This can be explained by the fact that nitrogen is an important component of the light-harvesting apparatus of these taxa, which supports sustained growth even under P limitation. An increase in phosphorous can even be detrimental because it increases susceptibility to photoinhibition (Litchman Citation2003). Hence, it is likely that increased thermocline depths offer superior nutrient conditions for C. comensis, leading to competitive advantages within the phytoplankton assemblage (Catalan, Pla et al. Citation2002a; Huisman et al. Citation2004; Jäger, Diehl, and Schmidt Citation2008; Jager, Diehl, and Emans Citation2010; Williamson et al. Citation2010b; Cantin et al. Citation2011). Under climate change, extreme precipitation events will become more frequent (Gobiet et al. Citation2014) and thus external input of nitrogen compounds into the study lakes will likely increase. Furthermore, the input of atmospheric nitrogen into high-altitude lakes is increasing and it is likely that this trend will continue (Catalan et al. Citation2013). As a result, C. comensis may become more abundant in lakes at higher elevations within the study region.
This study further demonstrated a significant positive correlation between August surface water temperature and the relative abundance of C. comensis (; GLM: p = .025, R2 = 0.66; GAM: FT_S = 34.27, p < .001, R2adj = 0.941, AIC = 215.3). Epilimnetic water temperature was found to be correlated with various parameters that may in turn be relevant to C. comensis growth. These include the duration of ice cover (Catalan, Pla, et al. Citation2002; Koinig et al. Citation2002; Sorvari et al. Citation2002), the strength of summer stratification (Catalan et al. Citation2013; Korhola et al. Citation2002; Reavie et al. Citation2017; Rühland, Paterson, and Smol Citation2008, Citation2015; Saros et al. Citation2016; Thompson, Kamenik, and Schmidt Citation2005), elevation and consequently catchment characteristics (e.g., Kamenik et al. Citation2001; Livingstone, Lotter, and Walker Citation1999), as well as light availability (Winder, Reuter, and Schladow Citation2009; Tolotti et al. Citation2012; Saros and Anderson Citation2015; Malik, Northington, and Saros Citation2017). All of these parameters in turn influence lake biogeochemical cycling (Catalan, Pla et al. Citation2002; Catalan, Ventura et al. Citation2002). Based on the data of the present study, buoyancy frequency within the epilimnion (which corresponds to the strength of summer stratification) did not significantly influence C. comensis growth. However, August surface water temperature was negatively correlated with elevation, implicating possible interactions with variables that were not assessed, such as the duration of ice cover, which in turn depends on catchment characteristics and can influence light availability. Further research should integrate these possible secondary effects to further specify the influence of epilimnetic temperature on C. comensis.
Conclusions
The present study underscores the potential of the diatom C. comensis to be used as an indicator of rising lake temperatures under elevated nitrate concentrations. C. comensis abundance is indicative of relatively deep thermoclines, whereas the strength of summer stratification was not an important driver of C. comensis abundance within the sampled lakes. At present, the conditions in montane lakes of the northern European Alps are especially favorable for C. comensis. As temperatures rise, the tree line will shift upwards, catchment vegetation and soil formation will be enhanced, and extreme precipitation events will become more frequent. All of these consequences of climate change will lead to higher input of organic compounds, including nitrate, into mountain lakes. Therefore, it is possible that C. comensis will also increase in abundance and possibly dominate lake plankton in subalpine or even alpine lakes in the future, if thermoclines are sufficiently deep and epilimnetic water temperatures continue to rise.
Supplemental Material
Download Zip (853.8 KB)Acknowledgments
We thank our colleagues Andrea Hofmann and Wolfgang Küfner, who helped during fieldwork, and our many students who helped during fieldwork and partly with hydrochemical analysis and diatom counts.
Supplementary material
Supplemental material for this article can be accessed on the publisher’s website
Additional information
Funding
References
- Battarbee, R. W. 1984. Diatom analysis and the acidification of lakes. Philosophical Transactions of the Royal Society of London. 305:451–77. doi:https://doi.org/10.1098/rstb.1984.0070.
- Bergström, A.-K. 2010. The use of TN:TP and DIN:TP ratios as indicators for phytoplankton nutrient limitation in oligotrophic lakes affected by N deposition. Aquatic Sciences 72 (3):277–81. doi:https://doi.org/10.1007/s00027-010-0132-0.
- Blanchet, F. G., P. Legendre, and D. Borcard. 2008. Forward selection of explanatory variables. Ecology 89 (9):2623–32. doi:https://doi.org/10.1890/07-0986.1.
- Brzezinski, M. A. 1985. The Si:C:N ratio of marine diatoms: interspecific variability and the effect of some environmental variables. Journal of Phycology 21:347–57. doi:https://doi.org/10.1111/j.0022-3646.1985.00347.x.
- Cantin, A., B. E. Beisner, J. M. Gunn, Y. T. Prairie, and J. G. Winter. 2011. Effects of thermocline deepening on lake plankton communities. Canadian Journal of Fisheries and Aquatic Sciences 68:260–76. doi:https://doi.org/10.1139/F10-138.
- Catalan, J., and E. Fee. 1994. Interannual variability in limnic ecosystems: Origin, patterns, and predictability. Amsterdam: Elsevier.
- Catalan, J., M. Ventura, A. Brancelj, I. Granados, H. Thies, U. Nickus, A. Korhola, A. F. Lotter, A. Barbieri, E. Stuchlík, et al. 2002. Seasonal ecosystem variability in remote mountain lakes: Implications for detecting climatic signals in sediment records. Journal of Paleolimnology 28 (1):25–46. doi:https://doi.org/10.1023/A:1020315817235.
- Catalan, J., M. G. Barbieri, F. Bartumeus, P. Bitusik, I. Botev, A. Brancelj, D. Cogalniceanu, M. Manca, A. Marchetto, N. Ognjanova-Rumenova, et al. 2009. Ecological thresholds in European alpine lakes. Freshwater Biology 54 (12):2494–517. doi:https://doi.org/10.1111/j.1365-2427.2009.02286.x.
- Catalan, J., S. Pla, M. Rieradevall, M. Felip, M. Ventura, T. Buchaca, L. Camarero, A. Brancelj, P. G. Appleby, A. Lami, et al. 2002. Lake Redó ecosystem response to an increasing warming in the Pyrenees during the twentieth century. Journal of Paleolimnology 28:129–45. doi:https://doi.org/10.1023/A:1020380104031.
- Catalan, J., S. Pla-Rabes, A. P. Wolfe, J. P. Smol, K. M. Ruhland, N. J. Anderson, J. Kopacek, E. Stuchlik, R. Schmidt, K. A. Koinig, et al. 2013. Global change revealed by palaeolimnological records from remote lakes: a review. Journal of Paleolimnology 49 (3):513–35. doi:https://doi.org/10.1007/s10933-013-9681-2.
- Curtis, C. J., S. Juggins, G. Clarke, R. W. Battarbee, M. Kernan, J. Catalan, R. Thompson, and M. Posch. 2009. Regional influence of acid deposition and climate change in European mountain lakes assessed using diatom transfer functions. Freshwater Biology 54 (12):2555–72. doi:https://doi.org/10.1111/j.1365-2427.2009.02317.x.
- Davidson, T. A., H. Bennion, M. Reid, C. D. Sayer, and T. J. Whitmore. 2018. Towards better integration of ecology in palaeoecology: from proxies to indicators, from inference to understanding. Journal of Paleolimnology 60 (2):109–16. doi:https://doi.org/10.1007/s10933-018-0032-1.
- Deutsches Institut für Normung e.V. 1983. Deutsche Einheitsverfahren Zur Wasser-, Abwasser- Und Schlammuntersuchung; Kationen (Gruppe E); Bestimmung Des Ammonium-Stickstoffs (E 5). Din 38406–5: 1983–10. Berlin: Beuth Verlag.
- DIN Technical Committee Water Analysis. 2015. Water Quality - Guidance on Quantitative and Qualitative Sampling of Phytoplankton from Inland Waters. Available https://standards.globalspec.com/std/9962395/EN%2016698.
- Dixit, S. S., J. P. Smol, J. C. Kingston, and D. F. Charles. 1992. Diatoms - powerful indicators of environmental-change. Environmental Science & Technology 26 (1):22–33. doi:https://doi.org/10.1021/es00025a002.
- Flower, R. J., and R. W. Battarbee. 1983. Diatom evidence for recent acidification of two Scottish lochs. Nature 305 (5930):130–33. doi:https://doi.org/10.1038/305130a0.
- France, R. 1997. Land water linkages: Influences of riparian deforestation on lake thermocline depth and possible consequences for cold stenotherms. Canadian Journal of Fisheries and Aquatic Sciences 54 (6):1299–305. doi:https://doi.org/10.1139/f97-030.
- Gobiet, A., S. Kotlarski, M. Beniston, G. Heinrich, J. Rajczak, and M. Stoffel. 2014. 21st century climate change in the European alps—a review. Science of the Total Environment 493:1138–51. doi:https://doi.org/10.1016/j.scitotenv.2013.07.050.
- Hall, R., and J. P. Smol. 1992. A weighted-averaging regression and calibration model for inferring total phosphorus concentration from diatoms in British Columbia (Canada) lakes. Freshwater Biology 27 (3):417–34. doi:https://doi.org/10.1111/j.1365-2427.1992.tb00551.x.
- Hastie, T. 2019. Generalized Additive Models.
- Haynes, W. 2013. Bonferroni correction. In Encyclopedia of systems biology, ed. W. Dubitzky, O. Wolkenhauer, K.-H. Cho, and H. Yokota, 1–11. New York: Springer.
- Hill, M. O., and H. G. Gauch. 1980. Detrended correspondence analysis: An improved ordination technique. Vegetatio 42 (1–3):47–58. doi:https://doi.org/10.1007/BF00048870.
- Huisman, J., and F. J. Weissing. 1995. Competition for nutrients and light in a mixed water column: A theoretical analysis. The American Naturalist 146 (4):536–64. doi:https://doi.org/10.1086/285814.
- Huisman, J., J. Sharples, J. M. Stroom, P. M. Visser, W. E. A. Kardinaal, J. M. H. Verspagen, and B. Sommeijer. 2004. Changes in turbulent mixing shift competition for light between phytoplankton species. Ecology 85 (11):2960–70. doi:https://doi.org/10.1890/03-0763.
- Huisman, J., P. van Oostveen, and F. J. Weissing. 1999. Critical depth and critical turbulence: Two different mechanisms for the development of phytoplankton blooms. Limnology And Oceanography 44 (7):1781–87. doi:https://doi.org/10.4319/lo.1999.44.7.1781.
- Jäger, C. G., S. Diehl, and G. M. Schmidt. 2008. Influence of water-column depth and mixing on phytoplankton biomass, community composition, and nutrients. Limnology and Oceanography 53 (6):2361–73. doi:https://doi.org/10.4319/lo.2008.53.6.2361.
- Jager, C. G., S. Diehl, and M. Emans. 2010. Physical determinants of phytoplankton production, algal stoichiometry, and vertical nutrient fluxes. The American Naturalist 175 (4):E91–E104. doi:https://doi.org/10.1086/650728.
- Kamenik, C., R. Schmidt, G. Kum, and R. Psenner. 2001. The influence of catchment characteristics on the water chemistry of mountain lakes. Arctic, Antarctic, and Alpine Research 33 (4):404–09. doi:https://doi.org/10.1080/15230430.2001.12003448.
- Klausmeier, C. A., E. Litchman, T. Daufresne, and S. A. Levin. 2004. Optimal nitrogen-to-phosphorus stoichiometry of phytoplankton. Nature 429 (6988):171–74. doi:https://doi.org/10.1038/nature02454.
- Koinig, K. A., C. Kamenik, R. Schmidt, A. Agustí‐Panareda, P. Appleby, A. Lami, M. Prazakova, N. Rose, Ø. A. Schnell, R. Tessadri, et al. 2002. Environmental changes in an alpine lake (Gossenköllesee, Austria) over the last two centuries – the influence of air temperature on biological parameters. Journal of Paleolimnology 28 (1):147–60. doi:https://doi.org/10.1023/A:1020332220870.
- Kolzau, S., C. Wiedner, J. Rücker, J. Köhler, A. Köhler, A. M. Dolman, and S. Ban. 2014. Seasonal patterns of nitrogen and phosphorus limitation in four German lakes and the predictability of limitation status from ambient nutrient concentrations. PLOS ONE 9 (4):E96065. doi:https://doi.org/10.1371/journal.pone.0096065.
- Korhola, A., S. Sorvari, M. Rautio, P. G. Appleby, J. A. Dearing, Y. Hu, N. Rose, A. Lami, and N. G. Camerson. 2002. A multi-proxy analysis of climate impacts on the recent development of subarctic Lake Saanajärvi in Finnish Lapland. Journal of Paleolimnology 28:59–77. doi:https://doi.org/10.1023/A:1020371902214
- Kraemer, B. M., O. Anneville, S. Chandra, M. Dix, E. Kuusisto, D. M. Livingstone, A. Rimmer, S. G. Schladow, E. Silow, L. M. Sitoki, et al. 2015. Morphometry and average temperature affect lake stratification responses to climate change. Geophysical Research Letters 42 (12):4981–88. doi:https://doi.org/10.1002/2015GL064097.
- Krammer, K. 2000. Diatoms of the European inland waters and comparable habitats: The genus Pinnularia. Rugell: A.R.G. Gartner Verlag K.G.
- Krammer, K. 2002. Diatoms of the European inland waters and comparable habitats: Cymbella. Rugell: A.R.G. Gartner Verlag K.G.
- Krammer, K., and H. Lange-Bertalot. 1991a. Bacillariophyceae, 3. Teil: Centrales, Fragilariaceae, Eunotiaceae. Stuttgart, Jena: Gustav Fischer Verlag.
- Krammer, K., and H. Lange-Bertalot. 1991b. Bacillariophyceae, 4. Teil: Achnanthaceae. Kritische Ergänzungen Zu Navicula (Lineolatae) Und Gomphonema. Stuttgart, Jena: Gustav Fischer Verlag.
- Krammer, K., and H. Lange-Bertalot. 1997a. Bacillariophyceae, 1. Teil: Naviculaceae. Stuttgart, Jena: Gustav Fischer Verlag.
- Krammer, K., and H. Lange-Bertalot. 1997b. Bacillariophyceae, 2. Teil: Bacillariaceae. Epithemiaceae, Surirellaceae. Stuttgart, Jena: Gustav Fischer Verlag.
- Lange-Bertalot, H. 2001. Diatoms of the European inland waters and comparable habitats: Navicula sensu stricto, 10 genera separated from Navicula Sensu Stricto, Frustulia. Rugell: A.R.G. Gartner Verlag K.G.
- Lange-Bertalot, H., G. Hofmann, M. Werum, and M. Cantonati. 2017. Freshwater benthic diatoms of central Europe. Over 800 common species used in ecological assessment. Oberreifenberg: Koeltz Botanical.
- Legendre, P., and E. D. Gallagher. 2001. Ecologically meaningful transformations for ordination of species data. Oecologia 129 (2):271–80. doi:https://doi.org/10.1007/s004420100716.
- Litchman, E. 2003. Competition and coexistence of phytoplankton under fluctuating light: experiments with two cyanobacteria. Aquatic Microbial Ecology 31:241–48. doi:https://doi.org/10.3354/ame031241.
- Livingstone, D. M., A. F. Lotter, and I. R. Walker. 1999. The decrease in summer surface water temperature with altitude in Swiss Alpine lakes: A comparison with air temperature lapse rates. Arctic, Antarctic, and Alpine Research 31 (4):341–52. doi:https://doi.org/10.1080/15230430.1999.12003319.
- Livingstone, D. M., and M. T. Dokulil. 2001. Eighty years of spatially coherent Austrian lake surface temperatures and their relationship to regional air temperature and the North Atlantic oscillation. Limnology And Oceanography 46 (5):1220–27. doi:https://doi.org/10.4319/lo.2001.46.5.1220.
- Lotter, A. F., and C. Bigler. 2000. Do diatoms in the Swiss Alps reflect the length of ice-cover? Aquatic Sciences 62 (2):125–41. doi:https://doi.org/10.1007/s000270050002.
- Lotter, A. F., H. J. B. Birks, W. Hofmann, and A. Marchetto. 1997. Modern diatom, cladocera, chironomid, and chrysophyte cyst assemblages as quantitative indicators for the reconstruction of past environmental conditions in the Alps. I. Climate. Journal of Paleolimnology 18 (4):395–420. doi:https://doi.org/10.1023/A:1007982008956.
- Lotter, A. F., H. J. B. Birks, W. Hofmann, and A. Marchetto. 1998. Modern diatom, cladocera, chironomid, and chrysophyte cyst assemblages as quantitative indicators for the reconstruction of past environmental conditions in the Alps. II. Nutrients. Journal of Paleolimnology 19 (4):443–63. doi:https://doi.org/10.1023/A:1007994206432.
- Malik, H. I., R. M. Northington, and J. E. Saros. 2017. Nutrient limitation status of Arctic lakes affects the responses of Cyclotella sensu lato diatom species to light: implications for distribution patterns. Polar Biology 40 (12):2445–56. doi:https://doi.org/10.1007/s00300-017-2156-6.
- Murphy, J., and J. P. Riley. 1962. A modified single solution method for the determination of phosphate in natural waters. Analytica Chimica Acta 27:31–36. doi:https://doi.org/10.1016/S0003-2670(00)88444-5.
- Naimi, B., N. Hamm, T. A. Groen, A. K. Skidmore, and A. G. Toxopeus. 2014. Where is positional uncertainty a problem for species distribution modelling? Ecography 37 (2):191–203. doi:https://doi.org/10.1111/j.1600-0587.2013.00205.x.
- Natural Earth. 2020. Physical vector data, 10 meter grid. Available at www.naturalearthdata.com.
- Navone, R. 1964. Proposed method for nitrate in potable waters. Journal American Water Works Association 56 (6):781–83. doi:https://doi.org/10.1002/j.1551-8833.1964.tb01270.x.
- Nixdorf, B., E. Hoehn, U. Riedmüller, U. Mischke, and I. Schönfelder. 2014. Probenahme Und Analyse Des Phytoplanktonsin Seen Und Flüssen Zur Ökologischen Bewertunggemäß Der Eu-Wrrl. In Handbuch Angewandte Limnologie, ed., M. Hupfer, W. Calmano, and H. Klapper, 1–24. Weinheim: Wiley‐VCH Verlag GmbH & Co. KGaA.
- Oksanen, J., G. F. Blanchet, M. Friendly, R. Kindt, P. Legendre, D. Mcglinn, P. R. Minchin, R. B. O’Hara, G. L. Simpson, P. Solymos, et al. 2018. VegAssessment of freshwater diatoms as quantitative indicators of past climatic change in the Yukon and Northwest Territories, Canada Community Ecology Package. R Package Version 2.5-2 Ed.
- Pienitz, R., J. P. Smol, and H. J. B. Birks. 1995. Assessment of freshwater diatoms as quantitative indicators of past climatic change in the Yukon and Northwest Territories, Canada. Journal of Paleolimnology 13 (1):21–49. doi:https://doi.org/10.1007/BF00678109.
- Prentice, I. C. 1980. Multidimensional scaling as a research tool in quaternary palynology: A review of theory and methods. Review of Palaeobotany and Palynology 31:71–104. doi:https://doi.org/10.1016/0034-6667(80)90023-8.
- R Core Team. 2013. R: A language and environment for statistical computing. Vienna: R Foundation For Statistical Computing. 3.4.3 Ed.
- Ramstack, J. M., S. C. Fritz, D. R. Engstrom, and S. A. Heiskary. 2003. The application of a diatom-based transfer function to evaluate regional water-quality trends in Minnessota since 1970. Journal of Paleolimnology 29 (1):79–94. doi:https://doi.org/10.1023/A:1022869205291.
- Reavie, E. D., G. V. Sgro, L. R. Estepp, A. J. Bramburger, V. L. Shaw Chraïbi, R. W. Pillsbury, M. Cai, C. A. Stow, and A. Dove. 2017. Climate warming and changes in Cyclotella sensu lato in the Laurentian Great Lakes. Limnology And Oceanography 62 (2):768–83. doi:https://doi.org/10.1002/lno.10459.
- Rogora, M., F. Buzzi, C. Dresti, B. Leoni, F. Lepori, R. Mosello, M. Patelli, and N. Salmaso. 2018. Climatic effects on vertical mixing and deep-water oxygen content in the subalpine lakes in Italy. Hydrobiologia 824 (1):33–50. doi:https://doi.org/10.1007/s10750-018-3623-y.
- Rühland, K., A. M. Paterson, and J. P. Smol. 2008. Hemispheric-scale patterns of climate-related shifts in planktonic diatoms from North American and European lakes. Global Change Biology 14:2740–54.
- Rühland, K. M., A. M. Paterson, and J. P. Smol. 2015. Lake diatom responses to warming: Reviewing the evidence. Journal of Paleolimnology 54 (1):1–35. doi:https://doi.org/10.1007/s10933-015-9837-3.
- Sadro, S., and J. M. Melack. 2012. The effect of an extreme rain event on the biogeochemistry and ecosystem metabolism of an oligotrophic high-elevation lake. Arctic, Antarctic, and Alpine Research 44 (2):222–31. doi:https://doi.org/10.1657/1938-4246-44.2.222.
- Saros, J. E., J. R. Stone, G. T. Pederson, K. E. H. Slemmons, T. Spanbauer, A. Schliep, D. Cahl, C. E. Williamson, and D. R. Engstrom. 2012. Climate-induced changes in lake ecosystem structure inferred from coupled neo- and paleoecological approaches. Ecology 93 (10):2155–64. doi:https://doi.org/10.1890/11-2218.1.
- Saros, J. E., and N. J. Anderson. 2015. The ecology of the planktonic diatom Cyclotella and its implications for global environmental change studies. Biological Reviews 90 (2):522–41. doi:https://doi.org/10.1111/brv.12120.
- Saros, J. E., R. M. Northington, D. S. Anderson, and N. J. Anderson. 2016. A whole-lake experiment confirms a small centric diatom species as an indicator of changing lake thermal structure. Limnology And Oceanography Letters 1 (1):27–35. doi:https://doi.org/10.1002/lol2.10024.
- Scheffler, W., A. Nicklisch, and I. Schonfelder. 2005. Articles on the morphology, ecology and ontogenesis of the planktonic diatom Cyclotella comensis Grunow. Studies on the historic and recently sampled material. Diatom Research 20:171–200. doi:https://doi.org/10.1080/0269249X.2005.9705626.
- Scherer, R. 2002. The Diatoms: applications for the environmental and earth sciences E. F. Stoermer and J. P. Smol Cambridge University Press, Cambridge 1999 (469 Pp) £70/Us$115 Isbn 0 521 58281 4. Journal Of Quaternary Science 17 (3):283–84. doi:https://doi.org/10.1002/jqs.632.
- Schwefel, R., B. Muller, H. Boisgontier, and A. Wuest. 2019. Global warming affects nutrient upwelling in deep lakes. Aquatic Sciences 81 (3):11. doi:https://doi.org/10.1007/s00027-019-0637-0.
- Smol, J. P., H. J. Birks, and W. M. Last. 2002. Tracking environmental change using lake sediments: Terrestrial, algal, and siliceous indicators. Dordrecht: Springer.
- Sorvari, S., A. Korhola, and R. Thompson. 2002. Lake diatom response to recent Arctic warming in Finnish Lapland. Global Change Biology. 8:171–81. doi:https://doi.org/10.1046/j.1365-2486.2002.00463.x.
- Stomp, M., J. Huisman, G. G. Mittelbach, E. Litchman, and C. A. Klausmeier. 2011. Large–scale biodiversity patterns in freshwater phytoplankton. Ecology 92 (11):2096–107. doi:https://doi.org/10.1890/10-1023.1.
- Strock, K. E., N. Theodore, W. G. Gawley, A. C. Ellsworth, and J. E. Saros. 2017. Increasing dissolved organic carbon concentrations in northern boreal lakes: Implications for lake water transparency and thermal structure. Journal of Geophysical Research: Biogeosciences 122 (5):1022–35. doi:https://doi.org/10.1002/2017JG003767.
- Terbraak, C. J. F., and I. C. Prentice. 1988. A theory of gradient analysis. Advances In Ecological Research 18:271–317.
- Thompson, R., C. Kamenik, and R. Schmidt. 2005. Ultra-sensitive Alpine lakes and climate change. Journal of Limnology 64. doi:https://doi.org/10.4081/jlimnol.2005.139.
- Throndsen, R. 1978. Preservation and storage. In Phytoplankton manual monographs on oceanographic methodology, ed., A. Sournia, 69–74. Paris: Unesco.
- Tolotti, M., F. Corradini, A. Boscaini, and D. Calliari. 2007. Weather-driven ecology of planktonic diatoms in Lake Tovel (Trentino, Italy). Hydrobiologia 578 (1):147–56. doi:https://doi.org/10.1007/s10750-006-0441-4.
- Tolotti, M., H. Thies, U. Nickus, and R. Psenner. 2012. Temperature modulated effects of nutrients on phytoplankton changes in a mountain lake. Hydrobiologia 698 (1):61–75. doi:https://doi.org/10.1007/s10750-012-1146-5.
- van der Werff, A., and T. T. Macan. 1955. A new method of concentrating and cleaning diatoms and other organisms. Proceedings of the International Association of Theoretical and Applied Limnology (Sil) 12:276–77.
- Vollenweider, R. A. 1968. Scientific Fundamentals of the Eutrophication of Lakes and Flowing Waters, With Particular Reference to Nitrogen and Phosphorus as Factors in Eutrophication: Technical Report (Das/Csi/68.27), Organisation for Economic Co-Operation and Development Directorate for Scientific Affairs.
- Weckstrom, J., A. Korhola, and T. Blom. 1997. Diatoms as quantitative indicators of Ph and water temperature in subarctic Fennoscandian lakes. Hydrobiologia 347 (1/3):171–84. doi:https://doi.org/10.1023/A:1003091923476.
- Wei, T., and V. Simko 2017. R Package “Corrplot”: Visualization of a Correlation Matrix.
- Werner, P., and J. P. Smol. 2006. The distribution of the diatom Cyclotella comensis in Ontario (Canada) lakes. Nova Hedwigia 130:373–91.
- Whitehead, P. G., R. L. Wilby, R. W. Battarbee, M. Kernan, and A. J. Wade. 2009. A review of the potential impacts of climate change on surface water quality. Hydrological Sciences Journal-Journal Des Sciences Hydrologiques 54 (1):101–23. doi:https://doi.org/10.1623/hysj.54.1.101.
- Williamson, C. E., C. Salm, S. L. Cooke, and J. E. Saros. 2010a. How do UV radiation, temperature, and zooplankton influence the dynamics of alpine phytoplankton communities? Hydrobiologia 648 (1):73–81. doi:https://doi.org/10.1007/s10750-010-0147-5.
- Williamson, C. E., C. Salm, S. L. Cooke, and J. E. Saros. 2010b. Erratum to: How do UV radiation, temperature, and zooplankton influence the dynamics of alpine phytoplankton communities? Hydrobiologia 652 (1):395–96. doi:https://doi.org/10.1007/s10750-010-0336-2.
- Winder, M., J. E. Reuter, and S. G. Schladow. 2009. Lake warming favours small-sized planktonic diatom species. Proceedings of the Royal Society B: Biological Sciences 276 (1656):427–35. doi:https://doi.org/10.1098/rspb.2008.1200.
- Winslow, L., R. Read, R. Woolway, J. Brentrup, T. Leach, J. Zwart, S. Albers, and D. Collinge. 2019. Rlakeanalyzer: Lake Physics Tools. R Package Version 1.11.4.1 Ed.
- Wunsam, S., R. Schmidt, and R. Klee. 1995. Cyclotella-taxa (Bacillariophyceae) in lakes of the Alpine region and their relationship to environmental variable. Aquatic Sciences 57 (4):360–86. doi:https://doi.org/10.1007/BF00878399.