ABSTRACT
Bryophytes are ecologically essential to northern ecosystem restoration after disturbance. In this study, native bryophytes were used to revegetate two Arctic restoration sites. Different propagation types (small, medium, large fragments) and substrates (crushed rock, lake sediment, processed kimberlite in Canada; volcanic silt loam and crushed lava rock in Iceland) with two erosion control treatments (with and without cheesecloth) were evaluated. After two growing seasons, large bryophyte fragments resulted in the greatest density and total and live cover with erosion control and medium fragments resulted in the highest density and species occurrence without erosion control. Erosion control significantly increased live cover, total cover, species occurrence, and density, including a tempering effect on soil volumetric water content and temperature. Substrates with more heterogeneous surfaces (crushed rock, volcanic silt loam, crushed lava rock) yielded higher live cover, density, and spontaneous colonization than more homogeneous substrates (processed kimberlite, lake sediment) and can be more suitable for use in arctic ecosystems revegetation. The positive outcomes in both Canada and Iceland led to the conclusion that bryophyte propagation with large to medium fragments, erosion control with cheesecloth, and substrates with heterogeneous surfaces would be effective restoration approaches where bryophyte revegetation is a focus.
Introduction
Development in relatively undisturbed northern ecosystems is occurring at an unprecedented rate to extract natural resources (metals, minerals, energy; Lett et al. Citation2021; Dhar et al. Citation2022; Hnatowich, Lamb, and Stewart Citation2022). Reducing the impact of this expansion, through timely restoration of decommissioned sites, is critical for the north. Restoration through natural processes in Arctic regions is limited due to extreme weather conditions, short growing seasons, long and cold winters, low rainfall, and slow nutrient release (Forbes Citation2015; Lett et al. Citation2021; Miller, Wilkinson, and Naeth Citation2021). Restoration may take 100 to 1,000 years or more depending on the scale and degree of impact (Forbes and Jefferies Citation1999; Johnstone and Kokelj Citation2008), or revegetated ecosystems may not recover to functional states equivalent to predisturbance conditions (Fenger-Nielsen et al. Citation2019). Challenging environmental conditions and limited knowledge of Arctic ecotypes and ecosystem processes, along with the lack of local plant materials (Hnatowich, Lamb, and Stewart Citation2022), impede northern restoration.
As pioneer species, bryophytes are critical to the function and structure of northern ecosystems (Jägerbrand et al. Citation2011; Turetsky et al. Citation2012; Lett et al. Citation2021) and play important roles in facilitating soil and microhabitat development (Jandt et al. Citation2008; Rydgren et al. Citation2011). They provide biomass and ground cover, reducing erosion and increasing nutrient cycling and retention, and provide food for consumers and decomposers (Turetsky et al. Citation2012; May et al. Citation2018; Lamarre, Dhar, and Naeth Citation2023). Bryophytes can form symbiotic relationships with nitrogen-fixing microorganisms, trading a favorable habitat for nitrogen and growth regulators (Gornall et al. Citation2007); this could be of critical importance in early stages of soil formation when substrates are void of nitrogen. Early presence of bryophytes can facilitate establishment and growth of other flora, such as lichens and vascular plants (Jägerbrand et al. Citation2011; Lett et al. Citation2021). Bryophytes provide protection and microsites for propagule germination and are important for plant assemblage and establishment (Forbes and Jefferies Citation1999; Caners, Crisfield, and Lieffers Citation2019; Gavini et al. Citation2019). Tundra bryophytes are important for sustaining faunal life (Soininen et al. Citation2013), including Arctic rodents (Ihl and Barboza Citation2007) and Rangifer tarandus (caribou; Bjørkvoll et al. Citation2009), and may provide remediation solutions for metal-affected substrates, absorbing and holding metals and providing habitat for metal tolerant plants (Adams and Lamoureux Citation2005).
Research on bryophyte propagation for northern restoration is lacking, with only a few studies using manual spreading (Aradóttir Citation2012; Aradóttir and Óskarsdóttir Citation2013; Hnatowich, Lamb, and Stewart Citation2022). Manual spreading of bryophyte turf material had little impact on donor sites and provided an equivalent or better outcome over time for restored sites (Aradóttir Citation2012). Fragmentation of bryophyte turf material may be effective but has never been tested for use in Arctic ecosystem restoration. Fragmentation encourages totipotent cells to differentiate into a meristematic state and reprogram for development of whole new organisms (La Farge, Williams, and England Citation2013). Propagation from detached leaves and fragmented sizes promoted bryophyte growth in a few studies (Wilmot-Dear Citation1980; Giordano et al. Citation1996; Hugonnot and Celle Citation2012; Ónody et al. Citation2016).
Disturbed soils in Arctic regions are prone to wind and water erosion due to low soil organic matter and aggregation and lack of vegetation (Cerdá and García-Fayos Citation2002; Moreno-de Las Heras, Nicolau, and Espigares Citation2008); thus, erosion control is likely to be essential for retaining bryophyte fragments in the windswept tundra to improve restoration success. Common erosion control methods include rapid establishment of a vegetation cover, recontouring and creating soil surface roughness, adding or incorporating soil amendments and conditioners, and placing erosion control blankets or mulch (Cohen-Fern´andez and Naeth Citation2013; Roberts and Seastedt Citation2019). Common erosion control methods for bryophytes are straw and coconut mats that are too thick to grow through; therefore, cheesecloth was used as an alternative for bryophyte revegetation in some studies (Schenk Citation1997; Glime Citation2017). Although cheesecloth has never been employed as an industrial erosion control material, if effective it could provide a fast-decomposing, weed-free, cost-effective alternative for northern restoration.
Although some research has been conducted on revegetation of northern or Arctic ecosystems with native forbs, shrubs, and grass species (Adams and Lamoureux Citation2005; Drozdowski, Naeth, and Wilkinson Citation2012; Naeth and Wilkinson Citation2014; Ficko and Naeth Citation2021; Miller, Wilkinson, and Naeth Citation2021; Hnatowich, Lamb, and Stewart Citation2022), there have been no attempts to establish bryophytes despite their major role in tundra ecosystems. The objective of this study was to determine how native bryophyte revegetation responded at a disturbed Arctic site (Diavik Diamond Mine) and determine whether similar results would occur at less disturbed sites (Heiðmörk). Specific objectives were to determine which fragment sizes (small, medium, large), substrates (crushed rock, lake sediment, processed kimberlite in Canada; volcanic silt loam and crushed lava rock in Iceland), and erosion control material (with and without cheesecloth) were more effective for bryophyte propagation and growth.
Materials and methods
Site descriptions
The study was conducted at Diavik Diamond Mine (hereafter Diavik) in Canada and Heiðmörk in Iceland. The Diavik site was located in low arctic tundra (64°49′ N and 110°27′ W) approximately 320 km northeast of Yellowknife in the Northwest Territories, Canada, approximately 100 km north of the tree line. The Diavik site consisted of a denuded and decommissioned area where three waste substrates (crushed rock, lake sediment, processed kimberlite) were deposited and leveled. The Heiðmörk sites were located (64°06’ N 21°75’ W) approximately 10 km from Reykjavík in southeast Iceland. The Heiðmörk sites were frequently disturbed by human activities; they were designated as a municipal conservation area and needed restoration. Both sites were located at the same latitude (64° N); the sun remains below the horizon for 24 hours per day in winter, and daylight is continuous for much of the summer. Both are polar islands, one oceanic and one lacustrine, with almost similar mean humidity (~70.0 percent) and wind speed (~19 km h−1). January is generally the coldest (mean −27.2°C Diavik; −2°C Heiðmörk) and July the warmest (mean 13.2°C Diavik; 14.0°C Heiðmörk) month. Mean annual precipitation is 305.8 mm at Diavik and 870 mm at Heiðmörk; temperature and precipitation during the study years were within the normal range of variability. The climate at Diavik is characteristic of continental polar environments, and that at Heiðmörk is maritime with mild fluctuations in mean monthly temperature (Einarsson Citation1984). Diavik is dry heath tundra to the north and wet tussock tundra to the south; major species are dwarf shrubs, willow (Salix sp), bog bilberry (Vaccinium uliginosum L.), crowberry (Empetrum nigrum L.), sedges, and bryophytes Aulacomnium turgidum (Wahlenb.) Schwägr. and Dicranum groenlandicum. Heiðmörk sites are on postglacial basaltic lava, and the surrounding ecosystem consists of dwarf shrub and moss heath dominated by large Racomitrium lanuginosum hummocks interspersed with resin birch (Betula glandulosa Michx.).
Treatment and experimental design
The experimental design consisted of three bryophyte fragment sizes, three substrates at Diavik and two at Heiðmörk, with and without erosion control. Large bryophyte fragments were 2.1 to 40 mm, medium were 1.1 to 2.0 mm, and small were <1 mm. Diavik substrates were applied at 50 cm depth over gravel pads (Figure S2). Gravel pads consisted of a layer of boulders over tundra, followed by a layer of small to mid-sized rocks, topped with 50 cm of gravel (Dhar et al. Citation2022), and had been built to protect the permafrost. Crushed rock overburden from mining consisted of granite <20 mm in size, with 76.4 percent sand, 19.7 percent silt, and 4.0 percent clay with a sand to loamy sand texture and 0.19 percent by weight organic matter. Lake sediment removed from pits after diking comprised 62.6 percent sand, 29.9 percent silt, and 7.5 percent clay with a sandy loam texture and 0.11 percent by weight organic matter. Processed kimberlite residual material from diamond removal was <1 mm with 79.6 percent sand, 15.3 percent silt, and 5.1 percent clay and a sandy texture and 0.10 percent by weight organic matter (Figure S2). Heiðmörk sites were on a volcanic silt loam plateau (3 m high, naturally eroded by frost heaving) with 48.0 percent sand, 48.0 percent silt, and 4.0 percent clay with a sandy loam texture and an unused road of crushed lava rock atop 5 to 15 cm of volcanic silt loam with 81 percent sand, 16.0 percent silt, and 3.0 percent clay of loamy sand texture (Figure S2). Erosion control material was natural, white, 100 percent cotton, twenty threads per inch, 1 mm2 mesh cheesecloth overlayed on soil. Cloth was secured with metal staples and expected to degrade and fuse to soil with time.
For each of the substrates, two 6 m × 3 m plots were established, each with four rows of 50 × 50 cm quadrats, separated by 20 cm (Figure S1), one randomly selected for erosion control material and one for no erosion control. In the middle of each quadrat, 10 cm × 10 cm areas were delineated and randomly assigned replicates and fragment size treatments. Ten replicates of each fragment size treatment with and without erosion control were on each of the substrates, for a total of sixty quadrats (3 fragment size × 2 erosion control × 10 replicate) per substrate. Two plots were selected as an unplanted treatment with and without erosion controls in each substrate. Sites were fenced with 2.5 cm2 mesh plastic wire attached to 0.5-m-tall wooden or metal stakes. Natural microtopography was retained, except when extremely large boulders were present or when any existing vegetation needed to be uprooted and removed.
Native bryophyte sample collection preparation and field application
Native bryophytes were collected from low, medium, and high disturbance areas within a kilometer of each research site (Table S1). After collection, bryophyte samples were separated from substrate and material was placed in a marked paper bag for transport to the laboratory for identification and preparation for field application. Equal portions of donor material were taken from areas of low, medium, and high disturbance to ensure diverse species composition. In total, thirty bryophytes were selected, of which eighteen were planted at Diavik and twenty-one at Heiðmörk (). Nine planted bryophytes were common to both sites.
Table 1. Bryophyte species collected at high, medium, and low disturbance areas with microhabitat preferences near Diavik, Canada, and Heiðmörk, Iceland.
Bryophyte material was roughly separated and then fragmented into large, medium, and small sizes. Large fragments (2.1–40 mm) were hand crumbled, approximating individual bryophytes; medium (1.1–2.0 mm) leaf and stem fragments were created by pushing bryophytes through a 1-mm sieve; and small (<1 mm) dust or particle-sized fragments were created by grinding dried material in a handheld coffee grinder. Before mixing with slurry, an equal amount of dried biomass of each bryophyte (eighteen at Diavik, twenty-one at Heiðmörk) was mixed together based on fragment size categories. Slurries were made in plastic buckets by hand mixing with a ratio of 2.0 g bryophytes with 100 mL clean water. The bryophyte mix was soaked for at least 20 minutes for full hydration. The plot for erosion control was overlaid with a single layer of cheesecloth. Quadrats were saturated with water before slurry application to slow material desiccation and promote adherence to soil. Slurries were applied to plots, directly on cheesecloth and substrate, using 10 cm × 10 cm square wooden frames and tablespoons to ensure homogeneity among replicates. A total of 15.0 mL of slurried small and medium fragments and 22.5 mL of slurried large fragments (contained more airspace) were added to each quadrat. Volumes were determined by wet slurry material weight. After application, all quadrats were hydrated and then photographed. Plots without erosion control material were left bare, and those with erosin control were covered with a second layer of cloth.
Soil analyses
Soil volumetric water content, electrical conductivity, and temperature sensors were installed to document surface soil conditions in the second growing season of the experiment. 5TE soil water sensors and Em50 digital analog data loggers were installed horizontally at approximately 5-cm depth adjacent to the research plots on each substrate. Two data loggers with five sensors each were installed in each substrate, one with erosion control and one without. Data were logged at 30-minute intervals during the growing season (June–August) for both locations. In June 2015, soil was collected from five random locations, mixed in a plastic bag to make a composite sample, and repeated six times per substrate. Soil pH and electrical conductivity were assessed in the laboratory using an Oakton Portable Waterproof pH/CON 300 Meter (Hendershot, Lalande, and Duquette Citation2007).
Vegetation assessments
Vegetation was assessed in August of year 2 of the study in both locations. Density was assessed by counting individuals in the 10 cm × 10 cm quadrat, and cover of living (only green) and total (all dead, not green, live, uncertain) bryophyte material was ocularly estimated, using a bryophyte cover guide designed for calibration of observations. During assessment, a photograph was taken of each plot, from a distance of approximately 20 cm (Figure S2). Species were counted, collected with forceps, and brought to the laboratory for identification and confirmation of regeneration. All plots with erosion control material were cut open to assess and photograph each layer of material. Care was taken to reduce disturbance to the plots when each layer was removed. Assessments were conducted directly on the substrate, under the two layers of erosion control cloth (hereafter under layer), and in the middle of the two layers (hereafter middle layer). Ten 10 cm × 10 cm plots without slurry were randomly selected between the planted grid on each substrate, half with erosion control and half without. Unplanted plots were assessed in the same way as planted plots.
Statistical analyses
Statistical analyses were conducted in R (R Core Team Citation2021) with a significance level α = .05 to assess impacts of substrates, erosion control, and bryophyte fragment size on bryophyte establishment on each site separately. Data and residuals were assessed for normality using histograms and the Shapiro-Wilk tests. Most data were not normally distributed; therefore, nonparametric permutational analyses of variance were conducted. Each test was run ten times to confirm significance of permutational output and most common output selected. Pairwise comparisons were conducted when significant differences were found. All interaction levels were considered, including site, substrate, erosion control, and fragment size.
For the native tundra bryophyte community investigation, nonmetric multidimensional scaling (NMDS) ordination was implemented to elucidate patterns of species composition among substrates. A two-dimensional solution was selected for ordinations and MetaMDS was used to execute multiple runs to seek stable configurations (McCune and Grace Citation2002). NMDS analyses based on a Bray-Curtis dissimilarity distances matrix were conducted using the vegan package (Oksanen et al. Citation2019). The similarity percentages (SIMPER), a nonparametric multivariate analysis of changes in community structure (Clarke Citation1993) was conducted to determine which species contributed most to Bray-Curtis dissimilarity among substrates that appeared in NMDS. The SIMPER analysis was performed using PAST software (Hammer et al. Citation2001).
Results and discussion
Soil
Icelandic soils were more acidic (pH 5.3–5.6) than Canadian soils (pH 6.4–8.5), as expected with soils of volcanic origin. Mean volumetric water content was similar among substrates and erosion control treatments (0.1–0.2 m3 m−3 in Diavik and Heiðmörk), which indicated that substrates or erosion control had no effect on volumetric water content (Table S2). Mean summer (June–August) soil temperatures were slightly higher at Diavik (13.6°C) than at Heiðmörk (11.8°C). In most cases, erosion control material elevated mean and minimum soil temperatures by < 0.5°C in most substrates (Table S2) and likely helped to regulate fluctuations in soil temperature. Mean electrical conductivity was extremely low (0.002–0.1 dS cm−1) and similar among substrates and sites.
Fragment size effects
Fragment size had a significant effect on live (p < .001) and total cover (p < .001) at both Diavik and Heiðmörk. Large fragments had significantly greater live and total cover after two growing seasons, followed by medium and small fragments (). With erosion control, large fragment sizes had the highest density (24.7/quadrat); without erosion control, medium fragments had the highest density (13.6/quadrat). Medium and small fragments were sifted through the erosion control material and may have been unable to access enough sunlight or water to propagate. Fragment size had an impact on species abundance, which was highest in medium fragments (247 occurrences), followed by large (232 occurrences) and small fragments (165 occurrences). The species occurring most frequently in small and large fragments was Ceratodon purpureus, and in medium fragments Racomitrium lanuginosum was slightly higher (Table S3).
Figure 1. Mean (± SE) (a) live cover and (b) total cover for fragment size, erosion control, and substrate treatments at Diavik, Canada (crushed rock, lake sediment, processed kimberlite), and Heiðmörk, Iceland (volcanic silt loam, crushed lava rock), research sites.
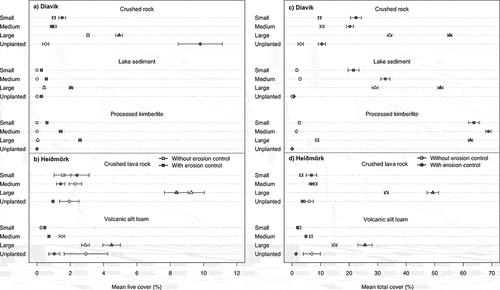
Magnúsdóttir and Aradóttir (Citation2011) found promising results for regeneration from fragments of Racomitrium lanuginosum in a greenhouse. Some studies suggested that larger fragments would imitate translocation of whole or partial plants by soil or water movement or by transportation on another living organism (Magnúsdóttir and Aradóttir Citation2011; Glime Citation2017). Although large fragments with erosion control provided better results, medium fragments could be used when erosion control is not available. Medium fragments with no erosion control treatment could find protection in micro depressions in the substrate and may have had quickest regeneration potential or better capacity to remain hydrated and retain nutrients (Naeth and Wilkinson Citation2014). On the contrary, large fragments were likely more susceptible to wind displacement in the absence of erosion control because Arctic regions are prone to wind and water erosion (Cerdá and García-Fayos Citation2002; Moreno-de Las Heras, Nicolau, and Espigares Citation2008). Thus, either of these bryophyte application approaches could facilitate short-term propagation of bryophytes with great potential for long-term stability and continued growth and development, not only of the bryophytes but of later successional communities. However, success of bryophyte propagation and colonization was highly dependent on species-specific microhabitat requirements where environment invariably impacts restoration outcomes, with wind, precipitation, and temperature having the most impact (Magnúsdóttir and Aradóttir Citation2011).
Substrate effects
Substrate had a significant effect on live cover (p < .001), total cover (p < .001), and density (p < .001) at Diavik but not at Heiðmörk. At Diavik, crushed rock had significantly greatest live cover (p < .001) and density (p < .001), whereas processed kimberlite had greatest total cover (p < .001; ). Greater total cover in processed kimberlite might be due to its better water retention characteristics. Other studies showed that processed kimberlite had greater water retention and water content than lake sediment and crushed rock (Drozdowski, Naeth, and Wilkinson Citation2012; Miller, Wilkinson, and Naeth Citation2021; Dhar et al. Citation2022); greater water retention in processed kimberlite relative to crushed rock and lakebed sediment was due to its composition of particles under 2 mm (Johnson Citation1987; Truett and Kertell Citation1992; Miller and Naeth Citation2019). However, less live cover and density in processed kimberlite might be due to its chemical properties, which could limit plant establishment and survival (Naeth and Wilkinson Citation2014; Miller, Wilkinson, and Naeth Citation2021). Miller, Wilkinson, and Naeth (Citation2021) found that processed kimberlite had high second-year mortality (60 percent). Greater live cover, total cover, and density in crushed rock indicates its suitability for initial revegetation. The success of crushed rock substrate might be because its rough surface creates seed traps and favorable microsites for seed germination and plant growth and shelter for seeds and seedlings (Jumpponen et al. Citation1999; Drozdowski, Naeth, and Wilkinson Citation2012; Naeth and Wilkinson Citation2014; Miller, Wilkinson, and Naeth Citation2021). Drozdowski, Naeth, and Wilkinson (Citation2012) found successful establishment and initial growth of vegetation on unamended crushed rock. Similarly, Miller, Wilkinson, and Naeth (Citation2021) reported that plant density on crushed rock was considerably greater than that on processed kimberlite and lake sediment. The two substrates at Heiðmörk may have been too similar to provide major differences. At Heiðmörk, crushed lava rock had slightly greater live cover, total cover, and density ().
Erosion control effects
Erosion control significantly affected live cover (p = 002), total cover (p < .001), and density (p < .001) at Diavik but only density (p = .003) at Heiðmörk. At Diavik, erosion control had greater live and total cover and density across substrates (), with similar live and total cover but significantly greater density at Heiðmörk (). The erosion control layer effect on density was significant at both sites under the cloth (Diavik: p = .011, Heiðmörk: p < .001), with no difference for the middle layer. Our study suggests that using erosion control materials can enhance live cover, total cover, and density irrespective of substrate type. Though some studies suggested that mulching, mesh netting, and erosion matting could reduce wind erosion and promote germination of the vascular seed bank (Whitall Citation1995; Lavendel Citation2002; Cook, Valdes, and Lee Citation2006), others reported no effect on vegetation when soil lynx was used as erosion control (Miller, Wilkinson, and Naeth Citation2021).
Substrate type influenced effectiveness of erosion control on bryophyte density at Diavik, with the middle erosion control layer (p < .001) and underlayer (p < .001) significantly different from the control (); no such effect was found at Heiðmörk (). At Diavik, erosion control underlayers in crushed rock had greater bryophyte density, followed by no erosion control and middle layers (). More homogeneous (microtopographically flat surface) substrates (processed kimberlite, lake sediment) had greater total cover with erosion control but less regeneration than more heterogeneous (microtopographically rocky, not flat surface) substrates (crushed rock, crushed lava rock, volcanic silt loam); perhaps these could provide more microtopographic protection from wind through surface heterogeneity. These substrates had the lowest retention of bryophyte material with erosion control. In addition to providing protective microsites, the microbiota in crushed rock, crushed lava rock, and volcanic silt loam likely had a positive impact on bryophyte regeneration. Other studies suggested that microtopographic heterogeneity could create sheltered areas for seeds, protect seeds and seedlings from erosion and desiccation, and, with time, increase plant establishment, organic matter, and soil nutrients (Elmarsdottir, Aradottir, and Trlica Citation2003; Caners, Crisfield, and Lieffers Citation2019), suggesting that texturally heterogeneous (1 to 5 cm surface variability) soils would be ideal for bryophyte revegetation. Thus, microtopographic heterogeneity provides diverse conditions for seed germination and vegetation establishment such as buffering soil temperatures, reducing seed erosion, and increasing grass and forb seedling emergence (Tilman Citation1994; Dhar et al. Citation2018; Melnik, Landhäusser, and Devito Citation2018; Naeth et al. Citation2018) and may have greater implications for ecosystem restoration than microtopographically homogeneous surfaces. Melnik, Landhäusser, and Devito (Citation2018) found that variability in microtopography created favorable growing conditions at an operational scale and helped to germinate a wider range of species from the propagule bank.
Effect on bryophytes community
At Diavik, nine out of eighteen planted species were found (eight in crushed rock, six in lake sediment, six in processed kimberlite) one year after planting; at Heiðmörk, eleven of twenty-one were found (ten in crushed lava rock, ten in volcanic silt loam). Species that were not planted but occurred in the plots included Bryum argenteum Hedwig at Diavik and Diplophyllum obtusifolium (Hook.) Dumort, Polytrichum piliferum, Polytrichum strictum, and Polytrichum commune Hedw. at Heiðmörk (Table S4). In total, nine bryophyte colonizers at Diavik and ten at Heiðmörk found in planted plots were also found in unplanted plots. Overall, erosion control had a positive impact on individual species occurrence or abundance, with significantly greater (417) species occurrences than without erosion control (228). Ceratodon purpureus and Racomitrium lanuginosum were the most frequent species with (87, 40 occurrences) and without (76, 33 occurrences) erosion control. In unplanted plots without erosion control, there were fewer colonizers, including Bryum argenteum (4), Bryum pseudotriquetrum (2), Cephalozia sp. (2), Ceratodon purpureus (2), Diplophyllum obtusifolium (1), Polytrichum strictum (1), Racomitrium fasciculare (2), and Racomitrium lanuginosum (4).
The NMDS ordination showed processed kimberlite was clearly separated from other substrates at Diavik, whereas no clear distinction was found for Heiðmörk (). NMDS ordinations yielded a two-dimensional solution and explained 88.1 percent of total variation in the original data matrix in Diavik and 77.4 percent in Heiðmörk. SIMPER analysis showed average dissimilarity in species composition between substrates from 35.7 to 42.2 percent at Diavik and 27.6 percent at Heiðmörk, where three to four species at Diavik and six species at Heiðmörk accounted for approximately 75 percent of the dissimilarity (). According to the vectors, Aulacomium turgidum and Bryum pseudotriquetrum had a higher correlation with crushed rock and Racomitrium lanuginosum with processed kimberlite at Diavik (), and Brachythecium albicans, Ceratodon purpureus, and Polytrichum strictum had a higher correlation with crushed lava rock and Diplophyllum obtusifolium with volcanic silt loam at Heiðmörk (). Among these species, Diplophyllum obtusifolium, Polytrichum strictum, and Bryum argenteum were new colonizers. Three species successfully propagated in all substrates: Bryum pseudotriquetrum, Ceratoron purpureus, and Racomitrium lanuginosum, of which Bryum pseudotriquetrum (Olech and Massalski Citation2001) and Ceratoron purpureus (Kershaw and Kershaw Citation1987; Olech and Massalski Citation2001) are known to colonize disturbed tundra. Success of each species on a given substrate is dependent on its related preference for microhabitat (). Sphagnum capillifolium, Sphagnum warnstorfii, Tetralophozia setiformis, and Calliergon richardsonii were not found at Diavik. Sphagnum capillifolium, Sphagnum warnstorfii, and Calliergon richardsonii are peatland species, collected from a low disturbance wetland at Diavik and added in expectation that they would help hold soil water for other bryophytes. Tetralophozia setiformis was collected at Diavik medium and low disturbance sites mixed with Ptilidium ciliare and other bryophytes. Tetralophozia setiformis is common on exposed rocks (Atherton et al. Citation2010) and may require protective presence of other mosses and liverworts to flourish. Bartramia ithyphylla, Dicranum scoparium, Diplophyllum albicans, Fissidens sp., Ptilidium ciliare, Racomitrium canescens, Rhytidiadelphus loreus, Polytrichum juniperinum, and Tortella tortuosa were not found at Heiðmörk. These species were collected from medium and low disturbance sites and could not survive ().
Figure 4. NMDS ordination showing bryophyte community composition in (a) Diavik and (b) Heiðmörk by substrate. The most common species that contributed to differences between substrates according to SIMPER analysis were fitted to vectors along the direction of correlation. Bry.arg = Bryum argenteum; Aul.tur = Aulacomium turgidum; Bry.pse = Bryum pseudotriquetrum; Rac.lan = Racomitrium lanuginosum; Bra.alb = Brachythecium albicans; Dip.obt = Diplophyllum obtusifolium; Cer.pur = Ceratodon purpureus; Pol.str = Polytrichum strictum.
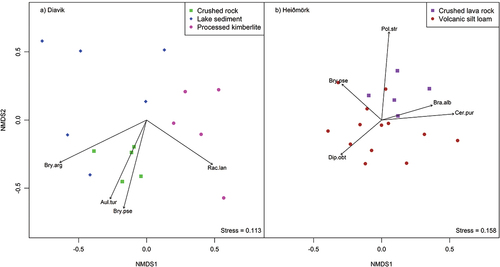
Table 2. Species contributions as average dissimilarities to the overall average dissimilarity between substrate in Diavik and Heiðmörk as indicated by SIMPER analysis.
Five bryophyte species, Bryum argenteum at Diavik and Diplophyllum obtusifolum, Polytrichum piliferum, Polytrichum strictum, and Polytrichum commune at Heiðmörk, that were not planted colonized in the research sites (Table S4). Among them, Bryum argenteum can grow widespread disturbed habitats (Atherton et al. Citation2010) and was found in crushed rock and lake sediment. Spread and abundance of Bryum argenteum indicates that it was probably dispersed by wind. According to Glime (Citation2017), this species colonizes exposed areas. Found on both substrates at Heiðmörk, Diplophyllum obtusifolum is a liverwort pioneer of open, crumbling acidic soil (Atherton et al. Citation2010). Polytrichum piliferum is a known colonizer, especially in exposed, loose, dry, and acidic substrates; Polytrichum strictum and Polytrichum commune are usually found in open, damp, acidic, peat areas (Atherton et al. Citation2010); all three occurred infrequently at Heiðmörk. These species could be targeted as successful propagators because they were collected and planted in their original climate.
Although conservative long-term projections based on this short-term study can be made, it would be difficult to extrapolate results from the early stages of the bryophyte revegetation process to make a strong longer-term conclusion. Long-term or continued multiyear studies of bryophyte revegetation methods in disturbed northern ecosystems will be required to better assess the effect of early bryophyte establishment on community development in Artic restoration sites.
Conclusions
Preparation methods for bryophyte revegetation assessed in this short-term study promoted propagation and growth at Diavik, Canada, and Heiðmörk, Iceland. However, disturbances had less impact on bryophyte revegetation than substrate, fragment size, and erosion control, because both sites showed almost similar responses. Large (2.1–40 mm) and medium (1.1–2 mm) fragments had the highest regrowth potential. Live cover was higher after two growing seasons with large fragments, whereas medium fragments yielded higher density and species abundance. Most frequent species were Ceratodon purpureus at Diavik and Racomitrium lanuginosum at Heiðmörk. Cheesecloth showed great potential for use as an erosion control material in northern ecosystems because it minimized fluctuations in soil temperature and promoted bryophyte colonization. More homogeneous substrates (lake sediment, processed kimberlite) had highest total bryophyte cover but did not show much regeneration. Substrates with more heterogeneous surfaces (crushed rock, volcanic silt loam, crushed lava rock) had highest live cover, density, and spontaneous colonization because they provided better control on microclimate conditions. The findings of this study suggest that mixtures of medium to large fragments and substrate with more heterogeneous (microtopographic) than homogeneous surfaces would be most beneficial and should be considered during Artic tundra restoration. However, field application of cheesecloth as a means of erosion control may require further study at larger scales before widespread use.
Author contributions
JJML worked on site selection, collected data, analyzed data, and wrote the thesis; AD analyzed the data and prepared, reviewed, and edited the article; MAN conceptualized the study, developed the experimental design, supervised all the work, and reviewed and edited the article.
Supplemental Material
Download Zip (2.5 MB)Acknowledgments
We thank the Environment Department of Diavik Diamond Mine and Dr. Ingibjörg S. Jónsdóttir and Ana J. Russi-Colmenares for logistical support and site information.
Disclosure statement
No potential conflict of interest was reported by the author(s).
Data availability statement
The data presented in this study may be available on request from the corresponding author. The data are not publicly available due to copyright issues.
Supplementary material
Supplemental data for this article can be accessed online at https://doi.org/10.1080/15230430.2023.2209394.
Additional information
Funding
References
- Adams, P. W., and S. Lamoureux. 2005. A literature review of the use of native northern plants for revegetation of Arctic mine tailings and mine waste. https://www.semanticscholar.org/paper/A-Literature-Review-of-the-Use-of-Native-Northern-Adams-Lamoureux/763417ddb6a03d28d8853b0ff5d9e54846a69364 (accessed June 3, 2022).
- Aradóttir, Á. L. 2012. Turf transplants for restoration of alpine vegetation: Does size matter? Journal of Applied Ecology 49, no. 2: 439–13. doi:10.1111/j.1365-2664.2012.02123.x.
- Aradóttir, Á. L., and G. Óskarsdóttir. 2013. The use of turf transplants for roadside revegetation in a subarctic area. Iceland Agricultural Sciences 26: 59–67.
- Atherton, I., S. Bosanquet, and M. Lawley. 2010. Mosses and liverworts of Britain and Ireland: A field guide. Plymouth, United Kingdom: British Bryological Society.
- Bjørkvoll, E., B. Pedersen, H. Hytteborn, I. S. Jónsdóttirand, and R. Langvatn. 2009. Seasonal and interannual dietary variation during winter in female Svalbard reindeer (Rangifer tarandus platyrhynchus). Arctic, Antarctic, and Alpine Research 41, no. 1: 88–96. doi:10.1657/1523-0430-41.1.88.
- Caners, R., V. Crisfield, and V. Lieffers. 2019. Habitat heterogeneity stimulates regeneration of bryophytes and vascular plants on disturbed minerotrophic peatlands. Canadian Journal of Forest Research 49, no. 3: 281–95. doi:10.1139/cjfr-2018-0426.
- Cerdá, A., and P. García-Fayos. 2002. The influence of seed size and shape on their removal by water erosion. Catena 48, no. 4: 293–301. doi:10.1016/S0341-8162(02)00027-9.
- Clarke, K. R. 1993. Non-parametric multivariate analyses of changes in community structure. Australian Journal of Ecology 18: 117–43. doi:10.1111/j.1442-9993.1993.tb00438.x.
- Cohen-Fernández, A. C., M. A. Naeth, and S. R. Wilkinson. 2013. Anthroposol development from limestone quarry substrates. Canadian Journal of Soil Science 93, no. 5: 555–66. doi:10.4141/cjss2012-120.
- Cook, H. F., G. S. B. Valdes, and H. C. Lee. 2006. Mulch effects on rainfall interception, soil physical characteristics and temperature under Zea mays L. Soil and Tillage Research 91, no. 1–2: 227–35. doi:10.1016/j.still.2005.12.007.
- Dhar, A., P. G. Comeau, J. Karst, B. Pinno, S. Chang, M. A. Naeth, R. Vassov, and C. Bampfylde. 2018. Plant community development following reclamation of oil sands mine sites in the boreal forest: A review. Environmental Reviews 26, no. 3: 286–98. doi:10.1139/er-2017-0091.
- Dhar, A., V. S. Miller, S. R. Wilkinson, and M. A. Naeth. 2022. Substrate and topsoil impact on soil water and soil temperature in Arctic diamond mine reclamation. Soil Systems 6, no. 1: 12. doi:10.3390/soilsystems6010012.
- Drozdowski, B. L., M. A. Naeth, and S. R. Wilkinson. 2012. Evaluation of substrate and amendment materials for soil reclamation at a diamond mine in the Northwest Territories, Canada. Canadian Journal of Soil Science 92, no. 1: 77–88. doi:10.4141/cjss2011-029.
- Einarsson, M. A. 1984. Climate of Iceland. World Survey of Climatology 15: 673–97.
- Elmarsdottir, A., A. L. Aradottir, and M. J. Trlica. 2003. Microsite availability and establishment of native species on degraded and reclaimed sites. Journal of Applied Ecology 40, no. 5: 815–23. doi:10.1046/j.1365-2664.2003.00848.x.
- Fenger-Nielsen, R., J. Hollesen, H. Matthiesen, E. Alexander, S. Andersen, A. Westergaard-nielsen, H. Harmsen, A. Michelsen, and B. Elberling. 2019. Footprints from the past: The influence of past human activities on vegetation and soil across five archaeological sites in Greenland. Science of the Total Environment 654: 895–905. doi:10.1016/j.scitotenv.2018.11.018.
- Ficko, S. A., and M. A. Naeth. 2021. Root development on cuttings of seven Arctic shrub species for revegetation. Arctic, Antarctic, and Alpine Research 53, no. 1: 237–51. doi:10.1080/15230430.2021.1976711.
- Forbes, B. C. 2015. Arctic vegetation cover: Patterns, processes and expected change. In The new Arctic (ebook), ed. B. Evengård, J. N. Larsen, and Ø. Paasche. 117–32. New York: Springer. http://www.springer.com/cn/book/9783319176017 (accessed June 2, 2022).
- Forbes, B. C., and R. L. Jefferies. 1999. Revegetation of disturbed Arctic sites: Constraints and applications. Biological Conservation 88, no. 1: 15–24. doi:10.1016/S0006-3207(98)00095-0.
- Gavini, S. S., G. M. Suárez, C. Ezcurra, and M. A. Aizen. 2019. Facilitation of vascular plants by cushion mosses in high-Andean communities. Alpine Botany 129, no. 2: 137–48. doi:10.1007/s00035-019-00222-6.
- Giordano, S., F. Alfano, A. Esposito, V. Spagnuolo, A. Basile, and R. C. Cobianchi. 1996. Regeneration from detached leaves of Pleurochaete squarrosa (Brid.) Lindb. in culture and in the wild. Journal of Bryology 19, no. 2: 219–27. doi:10.1007/s00035-019-00222-6.
- Glime, J. M. 2017. Construction. In Bryophytes Ecology, and J. M. Glime. Houghton, MI: Michigan Technological University, 1–35. http://digitalcommons.mtu.edu/bryophyte-ecology/ (accessed June 9, 2022).
- Gornall, J., I. Jónsdóttir, S. Woodin, and R. van der Wal. 2007. Arctic mosses govern below-ground environment and ecosystem processes. Oecologia 153, no. 4: 931–41. doi:10.1007/s00442-007-0785-0.
- Hammer, Ø., D. A. T. Harper, and P. D. Ryan. 2001. PAST: Paleontological statistics software package for education and data analysis. Palaeontologia Electronica 4: 1–9. https://palaeo-electronica.org/2001_1/past/issue1_01.htm (accessed February 12, 2023).
- Hendershot, W. H., H. Lalande, and M. Duquette. 2007. Soil reaction and exchangeable acidity. In Soil sampling and methods of analysis, ed. M. R. Carter and E. G. Gregorich, 2nd ed., 5. Boca Raton, United States: CRC Press, Taylor & Francis.
- Hnatowich, I. G., E. G. Lamb, and K. J. Stewart. 2022. Vegetative growth and belowground expansion from transplanted low-Arctic tundra turfs. Restoration Ecology e13716. doi:10.1111/rec.13716.
- Hugonnot, V., and J. Celle. 2012. Asexual reproduction by leaf fragmentation in Mnium stellare Hedw. Journal of Bryology 34, no. 1: 67–70. doi:10.1179/1743282011Y.0000000036.
- Ihl, C., and P. S. Barboza. 2007. Nutritional value of moss for Arctic ruminants: A test with muskoxen. Wild 71: 752–8. doi:10.2193/2005-745.
- Jägerbrand, A. K., R. G. Björk, T. Callaghan, and R. D. Seppelt. 2011. Effects of climate change on tundra bryophytes. In Bryophyte ecology and climate change, ed. Z. Tuba, N. G. Slack, and L. R. Stark, 211–36. Cambridge: Cambridge University Press. doi:10.1017/CBO9780511779701.012.
- Jandt, R., K. Joly, C. R. Meyers, and C. Racine. 2008. Slow recovery of lichen on burned caribou winter range in Alaska tundra: Potential influences of climate warming and other disturbance factors. Arctic, Antarctic, and Alpine Research 40, no. 1: 89–95. doi:10.1657/1523-0430(06-122)[jandt]2.0.co;2.
- Johnson, L. 1987. Management of northern gravel sites for successful reclamation. Arctic, Antarctic, and Alpine Research 19, no. 4: 530–6. doi:10.2307/1551421.
- Johnstone, J. F., and S. V. Kokelj. 2008. Environmental conditions and vegetation recovery at abandoned drilling mud sumps in the Mackenzie Delta region, Northwest Territories, Canada. Arctic 61: 199–211. doi:10.14430/arctic35.
- Jumpponen, A., H. Vare, K. G. Mattson, R. Ohtonen, and J. M. Trappe. 1999. Characterization of ‘safe sites’ for pioneers in primary succession on recently deglaciated terrain. The Journal of Ecology 87: 98–105. doi:10.1046/j.1365-2745.1999.00328.x.
- Kershaw, G. P., and L. J. Kershaw. 1987. Successful plant colonizers on disturbances in tundra areas of northwestern Canada. Arctic, Antarctic, and Alpine Research 19, no. 4: 451–60. doi:10.1080/00040851.1987.12002627.
- La Farge, C., K. H. Williams, and J. H. England. 2013. Regeneration of Little Ice Age bryophytes emerging from a polar glacier with implications of totipotency in extreme environments. Proceedings of the National Academy of Sciences of the United States of America 110, no. 24: 9839–44. doi:10.1073/pnas.130419911.
- Lamarre, J. J. M., A. Dhar, and M. A. Naeth. 2023. A propagation technique for native tundra bryophytes for Arctic ecosystem restoration. Arctic Science 379, no. 6639. doi:10.1126/science.adf6999.
- Lavendel, B. 2002. The business of ecological restoration. Ecological Restoration 20, no. 3: 173–8. doi:10.3368/er.20.3.173.
- Lett, S., I. S. Jónsdóttir, A. Becker-Scarpitta, C.T. Christiansen, H. During, F.Ekelund, G. H. R. Henry, S.I Lang, A. Michelsen, K. Rousk, et al. 2021. Can bryophyte groups increase functional resolution in tundra ecosystems? Arctic Science 1–29. doi:10.1139/as-2020-0057.
- Magnúsdóttir, M., Á. L. Aradóttir. 2011. Propagation of the moss, Racomitrium lanuginosum, for restoration. In Restoring the north – Challenges and opportunities; international restoration conference, Iceland, October 20-22, 2011; book of abstracts, and G. Halldórsson, 53. Reykjavik, Iceland: Soil Conservation Service of Iceland and Agricultural University of Iceland.
- May, J. L., T. Parker, S. Unger, and S. F. Oberbauer. 2018. Short term changes in moisture content drive strong changes in normalized difference vegetation index and gross primary productivity in four Arctic moss communities. Remote Sensing of Environment 212: 114–20. doi:10.1016/j.rse.2018.04.041.
- McCune, B., and J. B. Grace. 2002. Analysis of ecological communities. MjM software design, Gleneden Beach, Orlando. Journal of Experimental Marine Biology and Ecology 289: 303–5. doi:10.1016/S0022-0981(03)00091-1.
- Melnik, K., S. M. Landhäusser, and K. Devito. 2018. Role of microtopography in the expression of soil propagule banks on reclamation sites. Restoration Ecology 26: S200–S210. doi:10.1111/rec.12587.
- Miller, V. S., and M. A. Naeth. 2019. Hydrogel and organic amendments to increase water retention in anthroposols for reclamation. Applied and Environmental Soil Science 2019: 4768091. doi:10.1155/2019/4768091.
- Miller, V. S., S. R. Wilkinson, and M. A. Naeth. 2021. Micro topography, organic amendments and an erosion control product for reclamation of waste materials at an Arctic diamond mine. Ecological Engineering 172: 106399. doi:10.1016/j.ecoleng.2021.106399.
- Moreno-de Las Heras, M., J. M. Nicolau, and T. Espigares. 2008. Vegetation succession in reclaimed coal-mining slopes in a Mediterranean-dry environment. Ecological Engineering 34, no. 2: 168–78. doi:10.1016/j.ecoleng.2008.07.017.
- Naeth, M. A., A. C. Fernandez, F. P. O. Mollard, L. Yao, S. R. Wilkinson, and Z. Jiao. 2018. Enriched topographic microsites for improved native grass and forb establishment in reclamation. Rangeland Ecology and Management 71, no. 1: 12–18. doi:10.1016/j.rama.2017.08.004.
- Naeth, M. A., and S. R. Wilkinson. 2014. Establishment of restoration trajectories for upland tundra communities on diamond mine wastes in the Canadian Arctic. Restoration Ecology 22, no. 4: 534–43. doi:10.1111/rec.12106.
- Oksanen, J., F. G. Blanchet, M. Friendly, R. Kindt, P. Legendre, D. McGlinn, P. R. Minchin, et al. 2019. Vegan: Community ecology package. R Package Version 2.5.6. http://CRAN.R-project.org/package=vegan
- Olech, M. A., and A. Massalski. 2001. Plant colonization and community development on the Sphinx glacier forefield. Geographia 25: 111–19.
- Ónody, E., B. Fülöp-Pocsai, A. Tillyné Mándy, B. Papp, E. Tóth, and M. Ördögh. 2016. Comparison of propagation methods of different moss species used as wall and ground covering ornamental plants. International Journal of Horticultural Science 22, no. 3–4: 57–63. doi:10.31421/IJHS/22/3-4./1192.
- R Core Team. 2021. R: A language and environment for statistical computing. Vienna, Austria: R Foundation for Statistical Computing. www.R-project.org
- Roberts, J. W., and T. R. Seastedt. 2019. Effects on vegetative restoration of two treatments: Erosion matting and supplemental rock cover in the alpine ecosystem. Restoration Ecology 27, no. 6: 1339–47. doi:10.1111/rec.13010.
- Rydgren, K., R. Halvorsen, A. Odland, and G. Skjerdal. 2011. Restoration of alpine spoil heaps: Successional rates predict vegetation recovery in 50 years. In Restoring the north – Challenges and opportunities. International Restoration Conference, Iceland, October 20-22, 2011; Book of Abstracts, ed. G. Halldórsson, 34. Reykjavik, Iceland: Soil Conservation Service of Iceland and Agricultural University of Iceland.
- Schenk, G. 1997. Moss gardening: Including lichens, liverworts, and other miniatures. Portland, United States: Timber Press.
- Soininen, E. M., L. Zinger, L. Gielly, E. Bellemain, K. A. Bråthen, C. Brochmann, L. S. Epp, et al. 2013. Shedding new light on the diet of Norwegian lemmings: DNA metabarcoding of stomach content. Polar Biology 36, no. 7: 1069–76. doi:10.1007/s00300-013-1328-2.
- Tilman, D. 1994. Competition and biodiversity in spatially structured habitats. Ecology 75, no. 1: 2–16. doi:10.2307/1939377.
- Truett, J. C., and K. Kertell. 1992. Tundra disturbance and ecosystem production: Implications for impact assessment. Environmental Management 16, no. 4: 485–94. doi:10.1007/BF02394124.
- Turetsky, M. R., B. Bond-Lamberty, E. Euskirchen, J. Talbot, S. Frolking, A. D. McGuire, and E. S. Tuittila. 2012. The resilience and functional role of moss in boreal and Arctic ecosystems. New Phytologist 196, no. 1: 49–67. doi:10.1111/j.1469-8137.2012.04254.x.
- Whitall, D. 1995. High alpine restoration work at McDonald basin. Ecological Restoration 13, no. 1: 29–31. doi:10.3368/er.13.1.29.
- Wilmot-Dear, C. M. 1980. A study of regeneration from leaves in some species of Pogonatum and Polytrichum. Journal of Bryology 11: 145–60. doi:10.1179/jbr.1980.11.1.145.