ABSTRACT
Climate change research on fisheries is often focused on changes in species abundance and distribution, yet the impacts of severe weather events are also important. Climate models indicate that storm frequency and intensity may increase in the North Atlantic; however, uncertainties remain and consequences in Iceland are not well studied. This research represents a first attempt to understand local to regional implications of storminess on Icelandic fisheries industries. Using an interdisciplinary approach, the analysis (1) provides regional future wind speed projections in Iceland and surroundings under the Shared Socioeconomic Pathway (SSP) 3–7.0 scenario, (2) documents current local experiences using exploratory interviews with various stakeholders in fisheries and aquaculture, and (3) explores research priorities for understanding climate-change adaptations in coastal communities. Projections show that whereas areas of the west, south, and east of Iceland may experience fewer storm days, the region north/northeast of Iceland features an increase in storm days. Potential adaptations include a flexible management system that does not punish fishers for lost time due to bad weather, cooperative market arrangements among sectors, and job security considerations for fishers and fish processing workers. Future projections are needed for other variables such as precipitation, and future socioeconomic studies should address the predictions and impacts of storminess.
HIGHLIGHTS
Using a state-of-the-art climate model, this study suggests that wind speed will increase north of Iceland, whereas the south will be calmer in the future under the SSP3–7.0 emission scenario.
Impacts of storm events on fisheries including infrastructure damage, personal safety, land-based transportation disruptions, or temporary income losses can have disproportionate impacts on small-scale operations and rural communities.
Future climate model research should focus on assessing changes in the most extreme storms, wind direction, and precipitation over land, as well as evaluating the robustness of results across different climate models.
Future socioeconomic adaptation research should focus on the combined impacts of fish stock movements and changing weather conditions, and regional variations across Iceland as some areas are projected to be calmer.
Introduction
A growing number of studies show that marine social–ecological systems around the world face an increasing number of threats due to climate change, such as rising ocean temperatures, rising sea levels, ocean acidification, and increasing storm and extreme weather events (Chang et al. Citation2013; Hobday et al. Citation2016; Macusi et al. Citation2020; Turner, McConney, and Monnereau Citation2020; Heck, Beck, and Reguero Citation2021). Much of the research on negative impacts of climate change on marine systems is focused on changes of species abundance, distribution, and life cycles due to changes in sea-ice coverage, ocean acidification, and/or changing sea surface temperatures (Heck, Beck, and Reguero Citation2021). These projected biological changes have clear implications for human social and economic systems that are reliant on marine resources (Johnson and Welch Citation2009; Sainsbury et al. Citation2018; Hall-Spencer and Harvey Citation2019; Jara et al. Citation2020).
A second, but less explored, impact of climate change on marine social–ecological systems is the effects of more frequent and/or intense storm events. Though it is known that ocean warming may potentially alter fisheries catches over the next 50 to 100 years, increasing storminess may cause impacts in the shorter term (Sainsbury et al. Citation2018). Severe coastal storms can lead to physical disturbances of fish habitat and impacts on species richness, biomass, and density, which in turn impact socioeconomic aspects of marine resource–dependent communities (Sainsbury et al. Citation2018; Heck, Beck, and Reguero Citation2021). Current scholarship focuses on the vulnerability of capture fisheries to climate change, but only a few studies have directly considered impacts due to storms (Johnson and Welch Citation2009; Chang et al. Citation2013; Sainsbury et al. Citation2018; Jara et al. Citation2020; Macusi et al. Citation2020; Turner, McConney, and Monnereau Citation2020; Heck, Beck, and Reguero Citation2021), mostly because large uncertainties remain regarding the science around changes in storminess (Turner, McConney, and Monnereau Citation2020; Heck, Beck, and Reguero Citation2021). Coastal storms have adverse effects on fishing efforts, navigation, access to markets, loss or damage of fishing infrastructure, risks that fishers encounter at sea, and fishers’ livelihoods (Sainsbury et al. Citation2018; Macusi et al. Citation2020; Heck, Beck, and Reguero Citation2021).
Countries in Southeast Asia, the Caribbean, and Oceania are considered most at risk of storms and extreme weather events, with some Small Island Developing States ranking the highest (Heck, Beck, and Reguero Citation2021). However, in recent years, climate change–induced variations in climatic conditions have also been observed in many northern countries (Intergovernmental Panel on Climate Change [IPCC] Citation2021), with some studies projecting that the intensity of winter storms over the North Atlantic will increase until the year 2100 (Feser et al. Citation2015; Barange et al. Citation2018). The storminess over Iceland is closely connected to the phases of the North Atlantic Oscillation (NAO; Alexander Citation2005). The NAO index, defined by Bjerknes (Citation1964) with updated definitions by Rogers (Citation1984) and Hurrell (Citation1995), describes the sea-level pressure gradient between Iceland and the Azores islands. In the positive phase of the NAO, the westerlies over the North Atlantic are stronger and more storms occur over Iceland, whereas the negative NAO phase is connected to reduced storminess in the subpolar North Atlantic. The NAO shows large decadal to multidecadal variations, which makes it difficult to identify clear trends from observations (Feser et al. Citation2015) and to project robust trends for the future. The latest report of the IPCC (Citation2021) indicates a low-confidence increase of the NAO in high-emission scenarios. With somewhat more confidence, the latest generation of climate models simulates a future reduction of atmospheric blocking situations in the subpolar North Atlantic. This might be linked to more frequent storms in the subpolar region, including Iceland. A tendency toward more positive NAO conditions could lead to less favorable weather conditions for fisheries activities around Iceland and thus pose problems for economic activity within the fishery sector (Báez et al. Citation2021). However, the large internal variability of the NAO and a “tug of war” between two opposing processes, which have an opposing effect on the NAO, lead to uncertainty regarding the expected changes in the NAO and thus regional climate in the future (IPCC Citation2021). The first process is the Arctic amplification, which decreases the low-level meridional temperature gradient and might lead to more negative NAO conditions (e.g., Inoue, Hori, and Takaya Citation2012; Mori et al. Citation2014; García-Serrano et al. Citation2015; King, Hell, and Keenlyside Citation2016); the amplitude of this effect on the NAO is debated, however (Barnes Citation2013; Cohen et al. Citation2014; McCusker, Fyfe, and Sigmond Citation2016; Ogawa et al. Citation2018; Koenigk and Fuentes‐Franco Citation2019). The second process is enhanced warming in upper tropical levels, thus increasing the upper-level meridional temperature gradient, which is expected to lead to more NAO-positive conditions (Barnes Citation2013; Cohen et al. Citation2014; McCusker, Fyfe, and Sigmond Citation2016; Ogawa et al. Citation2018; Koenigk and Fuentes‐Franco Citation2019).
Because of uncertainty in climate projections, climate change adaptation experts note that continued research into regional impacts must also be combined with forward-thinking adaptation planning (Sainsbury et al. Citation2018). In the northern North Atlantic, fisheries are a substantial income source as well as the basis of cultural identity and general well-being of many coastal communities (Koivurova, Tervo, and Stepien Citation2008; Sainsbury et al. Citation2018; Jara et al. Citation2020). It is important therefore to gain an early understanding of local perceptions, vulnerability, and adaptive capacity in those fishing communities to manage the risk of extreme weather events in a timely manner as well as advance climate change adaptation in those areas. In an attempt to enhance such adaptation efforts in coastal fishing communities in countries in higher latitudes, this article represents a first effort to explore how extreme weather events and storms affect fishing communities in Iceland, responding to the literature outlining the need for such exploration and documentation (Hobday et al. Citation2016; Macusi et al. Citation2020; IPCC Citation2021) by combining preliminary socioeconomic adaptation mapping with a large ensemble of climate simulations with a focus on Iceland. Specifically, we answer the following research questions: First, what are the components of a regional climate model for Iceland specific to fisheries and aquaculture? Second, what broad socioeconomic aspects related to informants’ experiences and perceptions of climate impacts and threats should be considered in future climate adaptation research on Icelandic fisheries and aquaculture?
Methods
Climate modeling and related analysis
To assess potential changes of weather conditions relevant for fishing activities, we analyzed a single-model initial-condition large ensemble, the SMHI Large Ensemble (SMHI-LENS; Wyser et al. Citation2021). SMHI-LENS employs the general circulation model EC-Earth3.3.1 (Döscher et al. Citation2022), a state-of-the-art global climate model that was used for a wide range of model experiments in the Sixth Phase of the Coupled Model Intercomparison Project (CMIP6; Eyring et al. Citation2016) and related efforts. EC-Earth3.3.1 makes use of an atmospheric model component, based on the Integrated Forecast System (cycle 36r4) of the European Center for Medium-Range Weather Forecasts coupled to NEMO3.6 for the ocean (Rousset et al. Citation2015) and LIM3 for sea ice (Rousset et al. Citation2015). For SMHI-LENS, EC-Earth3.3.1 is integrated with a horizontal resolution of approximately 80 km in the atmosphere and a tripolar 1° grid in the ocean, the latter featuring grid refinement of 1/3° near the Equator. In the vertical, the ocean component operates with seventy-five layers, and the atmospheric model features ninety-one levels from the Earth’s surface up to a model top at 1 Pa.
SMHI-LENS consists of fifty ensemble members, each individual simulation starting from different initial conditions but all following identical external forcings, such as greenhouse gas concentrations, aerosols, solar variability, etc. One of the main advantages of such a single-model large ensemble is isolating the forced response and the internal variability in the coupled climate system (Maher, Milinski, and Ludwig Citation2021). Though each individual simulation features its own phase of internal variability, they all show a response to the identical external forcing, so that analysis of, for example, the ensemble mean results in averaging out the “noise” of internal (natural) variability. The simulation period of SMHI-LENS covers the recent historical period (1970–2014)—for which observed external forcings in line with CMIP6 recommendations are applied—and the remainder of the twenty-first century (2015–2100) following the forcing specifications given in the ScenarioMIP protocol (O’Neill et al. Citation2018). SMHI-LENS was produced employing a wide range of different future scenarios (currently eight different scenarios are publicly available). For this study, we present analysis of Shared Socioeconomic Pathway (SSP) 3–7.0, which is a scenario marked by a continuous increase of greenhouse gas emissions throughout the twenty-first century, resulting in an atmospheric CO2 concentration of above 800 ppm in 2100, which is associated with an anthropogenically induced radiative forcing of approximately 7 W m−2 and a relatively strong global mean temperature increase of roughly 4°C compared to preindustrial levels. We chose SSP3–7.0 because one aim of the research was to present an example of potential future wind changes, for which it is more illustrative to use a higher emission scenario, and SSP3–7.0 belongs to the Tier 1 scenarios of CMIP6. However, there are a range of other CMIP6-Tier 1 emission and land use scenarios in existence that would lead to smaller global mean temperature increases (e.g., SSP1–2.6, SSP2–4.5, SSP4–6.0) or to an even higher temperature increase (SSP5–8.5).
From the exploratory interviews (described below), it was common knowledge that a mix of climatic conditions including wind speed and direction, wave height and period, as well as sea and air temperature affect fishing activities at sea, and therefore it can be difficult to set accurate thresholds in which these factors cause disruptions. Using rough thresholds identified by key informants, the decision was made to focus on wind speed as the primary variable of weather and sea conditions in which fishing could not be safely carried out. The absolute values of wind speeds output by climate models cannot be directly compared to observed wind speeds. Climate models solve the underlying physical equations only for representative points in a rather coarse grid (approximately 80 km in our case) and typically feature biases in absolute wind speeds (and other variables). Thus, we decided to use thresholds based on the climatology of the model itself to define a proxy for storm days on which fishing is not safe. This proxy threshold is marked by the ninety-fifth percentile of daily wind speeds of the fifty members of the historical data experiment (reference period 1970–2014), calculated individually for every grid box of the climate model (see Appendix including a comparison to the equivalent ninety-fifth percentile for the observation-based ERA5 reanalysis; Hersbach et al. Citation2020). Therefore, we define thresholds matching wind speeds that are exceeded on average 18 days a year (5 percent of 365 days). For the future scenarios we keep these thresholds and analyze whether exceedances occur more or less frequently in the climate model.
The quantitative analysis of the climate model simulations is complemented by an assessment of statistical significance. For this purpose, we employed a block bootstrapping approach over the available fifty ensemble members. We created 1,000 samples (with the identical size of fifty members) of thirty-year periods at the end of the historical period and the scenarios, respectively, by randomly choosing (with replacement) from the fifty members of SMHI-LENS (the thirty-year period of the respective members is sampled as a block and stays intact). These 1,000 samples provide us with a range of climate signals, which allows us to calculate the statistical confidence intervals. We consider results statistically significant for a p value <.05; that is, if less than 5 percent of the 1,000 bootstrapping samples show a climate signal of zero or opposite sign compared to the climate signal of the original SMHI-LENS fifty-member ensemble.
Exploratory interviews
Exploratory interviews were carried out with members of fishing communities in the Westfjords of Iceland (), a large peninsula and administrative district in northwestern Iceland with a strong historical connection to fisheries that exists to this day (Smith Citation2014). Several of Iceland’s largest growing aquaculture companies are located in the area, as well as four large seafood companies, a calcified algae factory, a number of smaller seafood companies, small boat fisheries (typically vessels around 15 GT), and fisheries support service companies. Fisheries and aquaculture activities are important economically and culturally to the 7,000 inhabitants of the region (Hersbach et al. Citation2020).
A total of twelve exploratory interviews were conducted with various stakeholders in fisheries, aquaculture, harbor operations, search and rescue, transportation and logistics, community development, and fisheries and aquaculture processing and sales. Exploratory interviews offered an opportunity to gain insights into the different fisheries sectors in an open and informal setting to understand how people are affected by extreme weather and storm events and to outline threats and problems faced by Icelandic fishing communities in regard to storms and extreme weather events. This study was considered an exploratory study, based in one local region and with a smaller number of key informants, and is therefore limited in scope. Informants were chosen based on their significant roles in the specific industries. The interview guide followed general informed consent protocols and was designed to cover the following areas: (1) experiences related to extreme weather and storm events, (2) perceptions of institutional responses to them, and (3) local and experience-based knowledge that would support future adaptive capacity and resilience. Detailed notes were taken during the interviews and later coded thematically in Word roughly following the thematic framework provided by Macusi et al. (Citation2020), which includes the four major thematic areas of threats, vulnerabilities, problems, and adaptations.
Results
Climate modeling wind speed extremes
Analyzing SMHI-LENS for potential future changes in wind speed extremes (comparing the thirty-year period 2071–2100 to 1981–2010) yields heterogeneous results around Iceland (). We chose the 1981–2010 period because this is the thirty-year period in the historical simulations of the model that is closest to present day. Though wide areas of the North Atlantic west, south, and east of Iceland show fewer storm days (according to the proxy definition described in the Methods section) in a warmer future climate, the region north to northeast of Iceland features an increase in storm days. The general pattern of these changes is robust across different future scenarios in SMHI-LENS. The positive response of 4.5 more storm days in SSP3–7.0 northeast of Iceland essentially means approximately 25 percent more days with disruptions of fishing activities in this area. Conversely, west, south, and east of Iceland, 15 to 35 percent fewer storm days are diagnosed in SMHI-LENS for SSP3–7.0 in our proxy analysis. Given the large sample size of SMHI-LENS (fifty members times thirty years for this analysis) most of these results are statistically significant (p < .05). Only comparably small regions along the zero line of the climate change signal are insignificant (hatched areas in ; based on 1,000-fold bootstrapping). We assume that the pronounced signals over land areas are mainly related to changes in land use or vegetation used for the climate scenarios and—also given their very localized character—refrain from discussing these in the context of changes relevant for fishing activities.
Figure 2. Climate change signal in number of storm days per year (see definition in the Methods section) for the period 2071–2100 relative to 1980–2010 according to SMHI-LENS and SSP3–7.0; results that are statistically insignificant (p > .05 according to 1,000-fold bootstrapping) are hatched. The rough outline of Iceland is center, with the east coast of Greenland on the left. We define storm days as days exceeding the ninety-fifth percentile of daily wind speeds of the fifty members of the historical data experiment (reference period 1970–2014), calculated individually for every grid box of the climate model. For the future scenarios we keep these thresholds and analyze whether exceedances occur more or less frequently in the climate model.
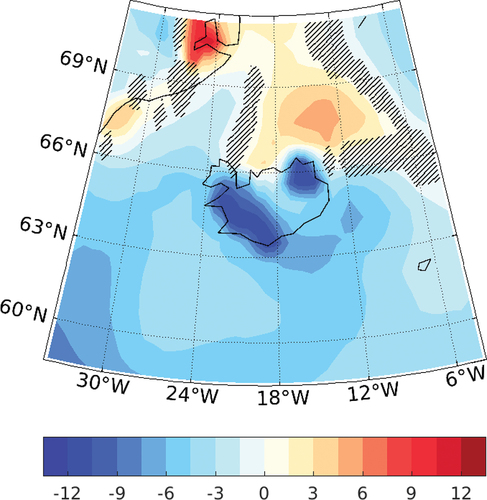
It should be noted that the results presented here from the SMHI-LENS should only be seen as one potential outcome of future change in storminess and that different climate models might provide different answers on future storminess changes. A recent study by Blackport, Fyfe, and Screen (Citation2022) showe that CMIP6 models simulate a wide range of trends for sea-level pressure (SLP) and wind trends in the North Atlantic region. However, they generally strongly underestimate or even fail to simulate the observed increasing trend toward stronger zonal winds in the North Atlantic and a more positive NAO index between 1950 and 2020. Though it is not entirely clear whether these observed trends are a response to increased greenhouse gas forcing or only part of potential centennial variability (IPCC Citation2021), it might indicate that climate models underestimate future wind changes in the North Atlantic region.
Further, Fuentes-Franco et al. (Citation2023) showed, based on CMIP6 future projections, that the variability of the NAO will decrease in the future and that the SLP pattern connected to the NAO changes as well. Across all CMIP6 models, SLP increases in the Nordic Seas and Barents Sea and decreases toward the Labrador Sea. Fuentes-Franco et al. (Citation2023) also showed a linkage between the simulated changes in NAO variability and precipitation extremes. For the Iceland area, more precipitation extremes are occurring in all scenarios linked to the changing NAO variability. Models with a stronger reduction in NAO variability show a particularly strong increase in precipitation extremes. Though the process behind this has not fully been untangled yet, it seems to be linked to changes in the sea surface temperature gradients in the North Atlantic.
Studies using large ensembles of single climate model simulations (e.g., Koivurova, Tervo, and Stepien Citation2008; Cohen et al. Citation2014; Wyser et al. Citation2021; Blackport, Fyfe, and Screen Citation2022) showed that variability of future trends across ensemble members is of almost the same size for many variables as the range of trends across the entire CMIP6 model ensemble. To qualitatively illustrate that, we provide in the Appendix showing the annual number of storm days according to SMHI-LENS for a specific grid point southeast of Iceland featuring a climate change signal of decreasing storminess. The huge interannual variability of storminess is obvious in this plot. Despite the systematic downward trend of the ensemble mean, it is possible for almost every model year to find single ensemble members featuring substantially (up to thirty-five and beyond) more storm days than on average and others with almost no storm days. Given that we analyzed exceedances of a threshold relative to the underlying model’s climatology, we expect that including other CMIP6 models would not substantially increase the ensemble spread even though their climate signal may be different.
Interviews
Results from interviews are organized roughly around the analytical framework provided by Macusi et al. (Citation2020) and grouped into threats, problems, impacts, and adaptations of fisheries to increased storminess and extreme weather events ().
Figure 3. Overview of results. Perceived impacts, threats, problems, and adaptation strategies of fisheries and aquaculture businesses in the Westfjords to storms and extreme weather events, using a modified version of the analytical framework from Macusi et al. (Citation2020).
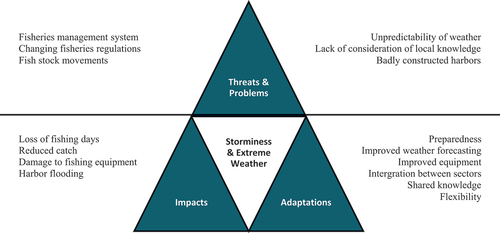
Experiences of local weather conditions and impacts
Storm conditions were generally defined by the interviewees as having winds of 23 to 25 m/s or greater. Interviewees also noted that wind speed affects different marine sectors differently, where larger boats can withstand and operate in greater storms with adverse sea conditions and small boats may not be able to safely leave the harbor in 15 to 20 m/s wind speeds (see as an illustration of wave heights tolerable for the largest boats, which would not be safe for smaller boats). In addition to wind strength, the wind direction plays a critical role for both fisheries and aquaculture. For example, for sea pen servicing in one specific fjord, interviewees noted that it is unsafe to go out in strong southerly or westerly winds but acceptable to go out in similar conditions if the wind is blowing from the north or the east with less fetch and where land provides shelter.
Figure 4. The GK 57 trawler in high waves in the south of Iceland. Photo credit: Jón Steinar Sæmundsson.
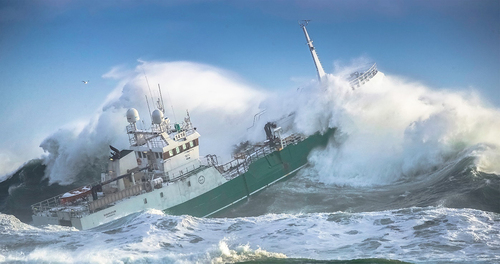
There was a common perception among informants that in Iceland there have been decades of bad weather in the past where there were lost fishing days, so when first asked, fishers were often hesitant to link any current experiences to climate change. However, informants did discuss several important changes that have been observed in recent years. Before the 1970s, trawlers tipping over due to icing on the vessel was a big concern, but this is no longer considered a hazard. Fishers noted that storms have become shorter in duration but more frequent, and large temperatures fluctuations from +10°C to −10°C within one week in the winter months are now more common. Shorter duration storms have greater impact for smaller vessels because they cannot weather even short storms and would need to return to harbor. Large temperature fluctuations were linked in informants’ minds to increased unpredictability, which in turn impacts at-sea operations. Small-scale fishers noted a decrease in the number of fishing trips in one fishing year due to bad weather and storm events that force the boats to stay in the harbor. As an example, one longline fisher mentioned that they used to be normal to go on fifteen fishing trips in thirty days in the months of December and January; however, due to increasing storminess the number of fishing trips has decreased to ten per month.
Though having to avoid storms by either staying in the harbor or sheltering in the fjord can lead to temporary loss of income for both large- and small-scale fisheries, there are other risks associated with storminess. In moderately stormy weather a captain may make the decision to go out to sea, even in waves 4 to 5 m high. It was noted that the catch decreases during bad weather because fish can be lost off the lines. Damage to fishing infrastructure can also occur in harbor during a storm. There have been incidents where the harbor areas were flooded due to a combination of a low-pressure system and especially high spring tides, causing damage to port electricity and other infrastructure that, in turn, affected provisions for necessary facilities and services for boats.
In sea pen aquaculture, damage due to icing during storms creates a financial risk for the aquaculture companies. In one recent case, a heavy storm caused icy buildup around the pens that damaged the nets. In another incident, a storm caused an electricity outage on a land-based aquaculture site, which led to a high fish mortality rate due to impeded nutrient circulation. Furthermore, as one informant emphasized, a failure of the equipment during an extreme weather event or storm can also have adverse effects on the company’s public image if the failure causes an economic or environmental risk. An aquaculture stakeholder noted that the equipment used for aquaculture pens can surprisingly withstand quite bad weather conditions. At the same time, another noted that decreased quality of fish was observed in pens located farther outside the fjord in a site more exposed to extreme weather conditions. Furthermore, if storms become more intense and frequent, staff might be sent out to repair the damage to pens during unfavorable weather conditions, which increases the risk of personal injuries at sea.
Indeed, for all operations at sea, a major concern is the safety of the crew. All informants agreed that storm-related accidents at sea have decreased over time due to the availability of improved equipment and a well-developed weather forecasting system. Furthermore, the requirement of all those working at sea to attend safety school greatly minimizes the risk to maritime workers. Good forecasting and safety training, together with the increased willingness of Icelandic fishers to contact the Coast Guard in risk situations, has led to a decreasing number of rescue missions. However, the Coast Guard noted that assistance missions have increased over time, which is partially due to storm-related problems but is also due to other issues such as tired sailors who run aground or failed equipment. It was also mentioned in the interviews that decisions to go out in stormy weather are often made because of (in the wording of informants) greed and competition among fishing crews.
External threats and problems
Related to the general areas of impacts listed above, informants identified several threats and problems in fisheries and aquaculture that have connections to storm events. Informants agreed that the fact that storms are becoming more intense and frequent is only one part of climate change that should not be considered by itself but as part of a combination of climate-related threats. Many informants were concerned about the compound effects of rising sea temperature and fish stock movements alongside increasingly harsh and unpredictable weather conditions. As reported above, though weather forecasting has improved in recent years, informants perceived that weather patterns are changing more rapidly, making it more difficult to predict the weather. According to informants, the established knowledge of weather patterns has to be adjusted constantly, and seventy-two-hour forecasts that could be used in the past are not precise enough anymore. The increasing unpredictability of the weather could eventually increase the risk to people at sea. On the other hand, it was also noted in interviews that people who work in the marine industries have already adapted to monitoring the weather more carefully and are thus usually able to prepare for storms in a timely manner.
It was also mentioned that though fishermen usually do not risk going out when bad weather is forecast, there can always be miscalculations for one reason or another, and this can be partially attributed to the management systems. As several informants noted, the individual transferable quota (ITQ) system is based on yearly catch allowances, so it is possible that there is reduced pressure for fishers to go out to sea during bad weather. For those with sufficient quota, it is not a major stress on the fishing operation to take a few days off fishing due to bad weather. However, in the summer coastal fishery, fishers are constrained by daily catch limits and may feel pressure to fish in adverse weather. In this fishery, each boat is given forty-eight days within a four-month time period, but the overall quota for the fishery is often reached before the forty-eight days, so there is pressure to go fishing before the quota limit is reached.
Other informants highlighted that even though they were aware of the adverse effects of climate change, such as increasing sea temperatures, rising sea levels, or migrating fish stocks, they were mostly concerned about the impacts that governmental regulations and the fisheries management system might have on fisheries. The concern was expressed that small boats would be replaced by bigger boats in the future to withstand adverse weather conditions. Others noted that the current discussion within the fishing industry is mostly focused on issues with the quota system and the problem of employment, pushing climate change adaptation needs into the background.
Finally, although it was not a major aim of this study to address impacts of the COVID-19 pandemic on fisheries, it was often discussed in interviews, and the experiences of members of the fisheries industry throughout the pandemic provided interesting comparisons to potential future climate change threats. For small-scale processors of fresh fish exports, the hardest time during the pandemic was when the U.S. market was closed for three weeks in early 2020 because of the cancellation of flights. In one example, a small-scale processing company was able to diversify into frozen exports but did lose money due to the halting of the export of fresh fish. Though they did have to slow down operations and consequently lost money, they managed to avoid employee layoffs in the processing factory.
Adaptation efforts and needs
Preparedness
During the interviews, several adaptation efforts and requirements regarding storminess and bad weather events were mentioned. A small boat fisher noted that it is important to take the necessary measures and prepare the boats well prior to a storm for them to be safe inside the harbor. It was further mentioned that it would be important to improve the harbors to prepare better for storms. Preparedness was also highlighted as an essential asset in the aquaculture sector. In aquaculture, because it is unsafe to send staff out to secure the equipment during a storm, the only option is to prepare well for the storm by using the best available technology and techniques and thus minimize the impacts.
From the point of view of search and rescue and the Coast Guard, it was assumed that if storms were to become more frequent and intense, fishers would still have to go to sea to sustain the fishery, which could result in an increasing number of rescue/assistance missions. Irrespective of whether storms and extreme weather events become more frequent and intense in the future, it was deemed necessary by several informants to base helicopters in locations additional to the base in Reykjavik to respond faster in risk situations in more remote locations. Until the financial resources are made available to locate helicopters outside Reykjavik, it will remain important that volunteers from local search and rescue teams in small remote towns continue to be able to operate smaller rescue boats.
Flexibility and diversity
Ideas of how to ensure flexibility and diversity were varied. Some informants predicted that if the weather changed and people were not able to fish anymore, the present fisheries management system based on ITQs would not be able to handle the potential large changes. Attention was drawn in the interviews to the need to adapt fisheries management policies to changing climatic conditions and a potential decline in fish stocks. Some informants noted that the expansion of the aquaculture sector could be considered a buffer against climate change impacts. Other informants pointed out that both marine fisheries and aquaculture businesses are impacted by extreme weather and intense storms similarly and thus share the same climate change risks.
A diversity of market options is also a key flexibility strategy for fisheries and aquaculture companies. Many large- and small-scale fisheries stakeholders highlighted the importance of the flexibility to fish new species and to invest in new onshore food processing technologies. In the large boat sector, companies with several vessels and integrated onshore processing have several flexibility options in place. In bad weather, catcher-processor trawlers need to find shelter close to the shore without coming to harbor, and while they wait for the weather to improve they might keep on processing the last catch. Fresh fish trawlers that deliver to onshore processing quite often have to adapt to the weather. Thus, for example, they might start trips a day earlier or use bad weather days for landing and then return to the grounds when the weather calms down. Again, accurate weather forecasting is crucial to make it possible for the trawlers to use weather windows. There is a partially coordinated effort in larger companies, where the shore-based fleet manager monitors temperature and wind speed, and with accurate forecasts the company has four to five days’ warning of bad storms and therefore has time to make needed changes.
Mitigations within companies and between sectors
In a local processing plant, there is a special fund to support the processing plant workers if there is a work shortage due to an inadequate supply of fish. For every fiscal year, during the first three days of a work stoppage due to low fish supply, the processing plant workers get paid full salary. After those three days, the company’s special fund kicks in and the people get paid 40 to 50 percent of their full salary while there are no fish. In one example from many years ago, the fund kicked in because the fishers could not fish for over twenty consecutive days because the cod stock was low. This was not related to the weather, but several informants noted that this special fund could be used as a buffer if increased storminess leads to raw material shortages for the local processing plants.
Knowledge exchange and cooperation among marine industries is already present and was also suggested as a future strategy to support traditional capture fisheries in the process of adapting to changing storminess. In one example of cooperation between large and small boat processing companies, if one processing plant does not have the necessary raw materials, they can get supplies from the other in a special agreement falling under the fisheries management rules on special community quota. Therefore, when the small boats cannot fish and supply fish to their processing plant, the large boats that can still fish in severe weather can supply raw material to the small processing plant.
Discussion
Planning in uncertainty and the importance of flexibility
Though the models produced for this study represent the best available projections for storminess in Icelandic waters, climate projections, as previously mentioned, are always accompanied by a particular level of uncertainty. The greater the uncertainty about the future is, the harder it is for managers to respond flexibly to large and unexpected shocks (Grafton Citation2010). This study revealed that uncertainty around the compounded impacts of storms and climate change persists and that potential interactions among the different impacts are still poorly understood. This is in line with other recent studies around the world (Turner, McConney, and Monnereau Citation2020; Heck, Beck, and Reguero Citation2021). To date, the interplay between changing storminess and other impacts of climate change and how this interplay affects marine ecosystems remain largely unexplored and would require a sophisticated interdisciplinary effort (Sainsbury et al. Citation2018).
Furthermore, it remains difficult to predict how people, economies, and the ecosystems they depend on might respond to changes in the climate. Informants, for example, mentioned their concerns regarding changing governmental regulations and a predicted impaired functionality of the fisheries management system that might result from the compounded effects of climate change impacts. Current research suggests that fisheries management tools like ITQ will most likely not be sufficient on their own and/or in their current state to support sustainable fisheries through increasing climatic and nonclimatic stressors (Grafton Citation2010; Young et al. Citation2018). It is becoming increasingly important to develop strategies that account for climate change–induced uncertainty. Decision making in uncertain conditions requires that new information and circumstances feed back into an adaptive process to produce adaptive responses (Grafton Citation2010). These adaptive responses, as outlined by Grafton (Citation2010), can include (1) risk sharing, a process that was also mentioned by the managing director of the aquaculture company, whereby fishers mitigate shocks via cooperation; (2) avoidance, whereby fishing communities are provided with constantly improved weather information and forecasting systems; (3) development of planning approaches that go beyond day-to-day operational planning; and (4) bridging knowledge gaps by sharing information among stakeholders, involving local knowledge in decision-making processes, and increasing knowledge in terms of predictions and forecasts (Grafton Citation2010).
In general, increasing uncertainty and variability underscores the need for fishery and aquaculture management to be flexible to respond to and learn from unexpected shocks (Cochran et al. Citation2009; Grafton Citation2010). Adaptive co-management could be adapted as a framework that provides some of the necessary flexibility to cope with such climate change–induced variability and uncertainty (Armitage et al. Citation2009; Cochran et al. Citation2009). Many informants noted the very diverse local impacts one storm might have. Depending on wind speed and direction or tidal surges, one harbor may be suitable for the small boats to exit, whereas in another community the next fjord over, the boats may be stuck. These local impacts point to the importance of local adaptation plans that take into account the very detailed local knowledge and understanding of how different forms of wave action and local oceanographic conditions affect at-sea operations. The inclusion of local knowledge in decision making was also deemed a necessity by interviewees from aquaculture, who pointed out that the people who work in the industry have the best knowledge of what happens on the ground. Local knowledge of weather processes could establish schemes that allow locals to assess whether it is safe to operate the net pen sites. If storms were to increase in intensity and frequency in the future, informants noted that it would be necessary to enhance the knowledge of the local area in addition to constantly upgrade and maintain the equipment which is being used.
Future research needs to advance climate adaptation of fisheries
Though this study highlights the need for more flexible management due to uncertainties and variability in the weather, it also reveals the need for extensive research to advance climate adaptation of fisheries and to enable such flexible management systems. Such research based on an interdisciplinary approach lays the groundwork for more effective decision making in adaptation planning (). Below, we discuss two areas of future needs regarding the findings of this research: climate models and fishers’ behavior and adaptation strategies.
Climate models, weather data, fishers’ behavior
To plan adaptation strategies, it is beneficial to have access to that most up-to-date climate projections. As weather is experienced subjectively by individuals and impacts across marine sectors vary, precise and professional long-term climate modeling is necessary to advance adaptation strategies of Icelandic fishing communities. Due to the high variability in weather conditions in different locations in the Westfjords, local models are crucial to the development of local adaptation strategies. However, it is necessary to keep in mind that even state-of-the-art climate models contain a certain level of uncertainty that needs to be accounted for when planning adaptation strategies. A high uncertainty in storminess from regional and global models is due to poor historical observational data, widespread variations in analytical methods, and the difficulty of determining whether changes were externally forced or part of natural climate variability (Sainsbury et al. Citation2018; Pinnegar et al. Citation2020).
The climate model EC-Earth3 that has been used to calculate future wind changes is a state-of-the-art climate model. Here, we used a large ensemble of fifty different climate simulations for each of the future emission scenarios. This data set is thus efficiently able to separate the climate change signal from internal variability of the wind and is thus well suited to analyze future storminess in Icelandic waters. The seemingly strong split between wind changes in the north and the south of Iceland highlights the regional nature of climate impacts. Here it is important to note that this regional pattern might differ between different climate models. Thus, before deciding on adaptation measures, it is necessary to analyze the robustness of the future wind changes across several climate models.
The interview results presented above map a preliminary outline of adaptation strategies for stormier weather based on interviews in the Westfjords. However, more detailed data are needed to assess the adaptation potential for other regions with a different set of socioeconomic and environmental factors, and the decision-making processes for climate adaptation planning must also be clarified. If the climate predictions in the models produced for this research become a reality, the Westfjords will in fact have fewer windy days, whereas communities in the north will experience more. This study revealed that though all fishery sectors are affected by storms and bad weather events, there are large sectoral variations. Small-scale fishers, for instance, reported a higher number of lost fishing days due to storminess than operators of larger fishing vessels. To develop and implement adaptation strategies that take different regional impacts and variations between sectors into account, on-ground research and action on local scales is required. For larger boats and larger companies, the available fishing area is effectively the entirety of the national waters of Iceland. However, for small boats that are often bound to rural communities (Chambers, Einarsson, and Karlsdóttir Citation2020), local storms may have a disproportionate impact. This information is important for fisheries managers, export companies, and other decision-makers who look at national trends in fisheries. Furthermore, future models should also address extreme precipitation events. In rural areas, snowstorms can also close roadways, sometimes for several days in a row, which limits access to the main markets by limiting capacity to transport fish products to the capital region of Reykjavík (S. Kristjánsdóttir, Westfjords Regional Development Office, pers. Comm, May 2021).
Marine fisheries and aquaculture businesses both rely heavily on sea state and weather forecasts (Savo, Morton, and Lepofsky Citation2017; Reid-Musson, Finnis, and Neis Citation2021). People who work in the marine industry in the Westfjords adapt to monitoring the weather more carefully, utilize the early warning system of the Coast Guard, and thus prepare for storms and react to changes in the weather in a timely manner. However, measures to mitigate the impact caused by limited weather forecasting need to be developed in advance. A potential cooperation and co-production between meteorologists and certain fishery sectors has been explored by Reid-Musson, Finnis, and Neis (Citation2021) and could, for example, lead to a better system that can better serve certain industry sectors. This could eventually result in improved weather forecasting, which remains especially crucial with regard to climate change–induced variability. Local variations regarding weather conditions during which fishing boats can be operated or aquaculture pens can be maintained could thus be included in the co-managed decision-making process.
Similarly, better socioeconomic data are needed to support climate change adaptation; for example, the decision-making processes individual fishers make regarding weather-related decisions are not well studied (Sainsbury et al. Citation2018). Though some aspects that influence fishers’ decisions were noted throughout this study, more detailed information on how different stakeholders in the Icelandic maritime industries rely on weather forecasting, and how such information influences their decision making, needs to be gathered. Crucial knowledge gaps still exist, such as mapping fishers’ decisions to remain in the harbor during storms, the effect of weather on the spatial distribution of fishing activity, the performance of different gear and equipment in bad weather, and perceptions of economic and physical risk (Sainsbury et al. Citation2018). Additionally, recent adaptation scholarship has pointed out that extreme events are often attributed to climate change and other place-based vulnerabilities are downplayed (Lahsen and Ribot Citation2021). To avoid disasters due to changing storminess being blamed solely on climate change when other factors may be involved, it is necessary to gather socioeconomic data to understand vulnerabilities in fishing communities around Iceland (Lahsen and Ribot Citation2021).
Adaptation strategies
In this article, several adaptation strategies for fisheries and aquaculture businesses to changing storminess that require further assessment and research were highlighted. Some informants pointed out that the expansion of the aquaculture sector could be considered a buffer against climate change impacts, and other informants were quick to note that storms and extreme weather also have negative impacts on sea pens and thus it does not qualify as an adaptation strategy. According to Reid et al. (Citation2019), most aquaculture activities are inherently vulnerable because of their reliance on specific areas of marine space. To adapt to uncertainties in storm extremes, current aquaculture operations need to incorporate the best available projections of weather thresholds in their structural design plans. Sea pens might have to be relocated to locations less impacted by intense and frequent storm activity (Reid et al. Citation2019). However, locating sea pens deeper into the fjords, which might lead to less water circulation around the pens, could result in stronger adverse effects on the ecosystem. In addition, moderately warmer conditions might lead to an increased growth rate in sea pen salmon and may also cause the fish to be more vulnerable to sea lice (Callaway et al. Citation2012). In this regard, functional adaptive strategies can be developed that capitalize on the advantage that aquaculture has over marine fisheries (Reid et al. Citation2019).
Throughout the interviews, the importance of improving the harbors was predominantly mentioned as an adaptation need and important preparedness measure to face the potential of more frequent and intense storms in the future. Because harbors can be vulnerable to storm surges, flooding, and sea-level rise, impacts of climate change need to be anticipated and harbors need to be proactively prepared for increased flooding and sea-level rise, as well as more frequent storm events (Kontogianni et al. Citation2019). To adapt to changing storminess in the future, the local harbors in the Westfjords should be reassessed holistically, taking geophysical as well as socioeconomic parameters into account, and adaptation measures should be carried out. A study conducted by Eskafi et al. (Citation2020) on flexible and adaptive port planning that includes a case study of the Ísafjörður port network presented an array of methods that are crucial to achieve sustainability in port development and management. This study showed that in the future, it will be essential for port development in Iceland to anticipate the impacts of climate change to guarantee the development of efficient and resilient harbor spaces (Kontogianni et al. Citation2019).
This study further highlighted the need to continuously involve and train search and rescue volunteers in small rural communities in Iceland to ensure a fast response to unexpected weather-induced risk situations. Because volunteer search and rescue groups are nearly always the first responders in Iceland, providing additional training, resources, and support is and will remain crucial, especially under changing storminess conditions (Ford and Clark Citation2019). According to the interview with the Icelandic Coast Guard, it has not yet been financially feasible to develop helicopter bases in rural regions outside the capital city. This underlines the need for competent search and rescue volunteers in rural regions in Iceland and calls for in-depth research on how to ensure and advance the continuous inclusion of volunteers in rescue operations.
Finally, adaptation options such as partner-to-partner agreements are good examples of adaptation measures. Such arrangements have the possibility to allow fishing companies to flexibly respond to unexpected shocks and minimize economic losses. For example, the ability to switch production to frozen fish during COVID-19 market changes may have interesting comparisons for future storm adaptations when small-scale fishers are not able to land fresh fish. However, building and testing responsive and effective partnership models may require adjustments to existing fishery management schemes which tend to be static and centralized (Lomonico et al. Citation2021). According to Icelandic regulations, there is a limit on how much raw material is allowed to be sold to a third party; however, a special agreement between companies, as described in the Results section, could be an interesting consideration for companies and fishery management. Additionally, the cooperation, rather than competition, between small and large fishing fleets deserves further attention and consideration. To use such arrangements to adapt to changing storminess in the future, more research that explores potential collaborations and their feasibility under existing fishery management rules is required. Furthermore, it will be necessary to explore how current fishery management tools and regulatory pathways can be altered to allow for faster responsiveness to unexpected shocks by promoting effective collaboration among fishery sectors.
Conclusions
This study has shown that future projections of storminess around Iceland vary by region and that potential impacts of storms on fisheries and aquaculture businesses vary between sectors. To develop and implement suitable adaptation strategies to changing storminess, further on-ground research and action at local scales is necessary. As well as the research needs mentioned in the Discussion, additional research is necessary with regard to impacts of storms and extreme weather events on other maritime sectors important to local economies such as fish processing, transportation, shipping, cruise ships, marine tourism, food production, and net mending. Climate model simulations are the best source for projections of future storminess in Icelandic waters and lay the baseline for further climate-related adaptation research in Icelandic fisheries. The model used in this study shows regional variations in wind speed projections between the north and south of Iceland. Though this study focused on the Westfjords, future studies should focus on other northern regions of Iceland, which, based on our modeling findings, may be subject to more pressure from increased storm events. Additional future research should focus on climate models that address changes in the most extreme storms, changes in wind direction related to storms, changes in precipitation over land linked to storms, and understanding of the robustness of results across different climate models.
Author contributions
Conceptualization, CC; methodology, CC, TE, ToK, TiK; data collection, NS, CC, TE, ToK, TiK; data analysis, NS, CC, TE, TiK; draft preparation, NS, CC; review and editing, NS, CC, TE, ToK, TiK, NE, AO; funding acquisition, AO. All authors have read and agreed to the published version of the article.
Human research participants
Human research ethics protocols were guided by funding agency (Nordforsk) standards. For the Icelandic research, the project was based at a governmental research institution (Stefansson Arctic Institute) that does not have a specific research review board. Therefore, guidelines for personal data protection were obtained from the Icelandic Data Protection Authority (Persónuvernd). According to the Icelandic Data Protection Authority Act on Data Protection and the Processing of Personal Data (No. 90 of 27 June 2018), research projects that use informed consent do not need to apply for ethics or data protection approval. Original Icelandic: “Persónuvernd vekur athygli á að ekki þarf að sækja um leyfri fyrir vinnslu persónuupplýsinga ef vinnslan fer fram á grundvelli upplýsts samþykkis eða ef lagaheimild stendur til vinnslunnar (https://www.personuvernd.is/hafa-samband/). English translation: “Act on Data Protection and the Processing of Personal Data, No. 90 of 27 June 2018” (https://www.personuvernd.is/media/uncategorized/Act_No_90_2018_on_Data_Protection_and_the_Processing_of_Personal_Data.pdf).
The informed consent process included informing the potential participants about the goal of the project, the risks and benefits of being in the study, and the anonymous and voluntary nature of the study. Oral consent was obtained before the interviews, and participants had the opportunity to review preliminary data.
Supplemental Material
Download ()Acknowledgments
The authors thank the informants who took part in the interviews for their time and knowledge. The SMHI-LENS simulations with EC-Earth were performed on resources provided by the Swedish National Infrastructure for Computing (SNIC) at the National Supercomputer Centre at Linköping University (NSC).
Disclosure statement
No potential conflict of interest was reported by the authors.
Supplementary material
Supplemental data for this article can be accessed online at https://doi.org/10.1080/15230430.2023.2269689.
Additional information
Funding
References
- Alexander, L. V. 2005. Recent observed changes in severe storms over the United Kingdom and Iceland. Geophysical Research Letters 32, no. 13: L13704. doi:10.1029/2005GL022371.
- Armitage, D. R., R. Plummer, F. Berkes, R. I. Arthur, A. T. Charles, I. J. Davidson-Hunt, A. P. Diduck, et al. 2009. Adaptive co-management for social–ecological complexity. Frontiers in Ecology and the Environment 7, no. 2: 95–16. doi:10.1890/070089.
- Báez, J. C., L. Gimeno, and R. Real. 2021. North Atlantic Oscillation and fisheries management during global climate change. Reviews in Fish Biology and Fisheries 31, no. 2: 319–36. doi:10.1007/s11160-021-09645-z.
- Barange, M., T. Bahri, M. C. M. Beveridge, K. L. Cochrane, S. Funge-Smith, and F. Poulain. 2018. Impacts of climate change on fisheries and aquaculture. United Nations’ Food and Agriculture Organization 12, no. 4: 628–35.
- Barnes, E. A. 2013. Revisiting the evidence linking Arctic amplification to extreme weather in midlatitudes: Arctic amplification and weather. Geophysical Research Letters 40, no. 17: 4734–9. doi:10.1002/grl.50880.
- Bjerknes, J. 1964. Atlantic air-sea interaction. Advances in Geophysics 10, 1–82. doi:10.1016/S0065-2687(08)60005-9.
- Blackport, R., J. C. Fyfe, and J. A. Screen. 2022. Arctic change reduces risk of cold extremes. Science 375, no. 6582: 729–729. doi:10.1126/science.abn2414.
- Callaway, R., A. P. Shinn, S. E. Grenfell, J. E. Bron, G. Burnell, E. J. Cook, M. Crumlish, et al. 2012. Review of climate change impacts on marine aquaculture in the UK and Ireland: Climate change and marine aquaculture in the UK and Ireland. Aquatic Conservation: Marine and Freshwater Ecosystems 22: 389–421. doi:10.1002/aqc.2247.
- Chambers, C., N. Einarsson, and A. Karlsdóttir. 2020. Small-scale fisheries in Iceland: Local voices and global complexities. In Small-scale fisheries in Europe: Status, resilience and governance. ed. J. J. Pascual-Fernández, C. Pita, and M. Bavinck, 329–49. Cham: Springer International Publishing. doi:10.1007/978-3-030-37371-9_16.
- Chang, Y., M.-A. Lee, K.-T. Lee, and K.-T. Shao. 2013. Adaptation of fisheries and mariculture management to extreme oceanic environmental changes and climate variability in Taiwan. Marine Policy 38: 476–82. doi:10.1016/j.marpol.2012.08.002.
- Cochrane, K. L., R. I. Perry, T. M. Daw, D. Soto, M. Barange, and S. S. De Silva, Food and Agriculture Organization of the United Nations, ed. 2009. Climate change implications for fisheries and aquaculture: Overview of current scientific knowledge. Rome: Food and Agriculture Organization of the United Nations.
- Cohen, J., J. A. Screen, J. C. Furtado, M. Barlow, D. Whittleston, D. Coumou, J. Francis, et al. 2014. Recent Arctic amplification and extreme mid-latitude weather. Nature Geosciences 7, no. 9: 627–37. doi:10.1038/ngeo2234.
- Döscher, R., M. Acosta, A. Alessandri, P. Anthoni, T. Arsouze, T. Bergman, R. Bernardello, et al. 2022. The EC-Earth3 Earth system model for the coupled model intercomparison project 6. Geoscientific Model Development 15, no. 7: 2973–3020. doi:10.5194/gmd-15-2973-2022.
- Eskafi, M., G. F. Ulfarsson, A. Dastgheib, P. Taneja, G. Stefansson, and R. I. Thorarinsdottir. 2020. Flexible and adaptive port planning an Icelandic case of the Ports of Isafjordur network. https://www.vegagerdin.is/vefur2.nsf/Files/NR_1800_784_Sveigjanleg_og_adlogunarhaef_skipulagsgerd_fyrir_hafnir_-_utdrattur/$file/NR_1800_784_Sveigjanleg%20og%20a%C3%B0l%C3%B6gunarh%C3%A6f%20skipulagsger%C3%B0%20fyrir%20hafnir%20-%20%C3%BAtdr%C3%A1ttur.pdf (accessed April 7, 2022).
- Eyring, V., S. Bony, G. A. Meehl, C. A. Senior, B. Stevens, R. J. Stouffer, and K. E. Taylor. 2016. Overview of the Coupled Model Intercomparison Project Phase 6 (CMIP6) experimental design and organization. Geoscientific Model Development 9, no. 5: 1937–58. doi:10.5194/gmd-9-1937-2016.
- Feser, F., M. Barcikowska, O. Krueger, F. Schenk, R. Weisse, and L. Xia. 2015. Storminess over the North Atlantic and northwestern Europe—A review. Quarterly Journal of the Royal Meteorological Society 141, no. 687: 350–82. doi:10.1002/qj.2364.
- Ford, J., and D. Clark. 2019. Preparing for the impacts of climate change along Canada’s Arctic coast: The importance of search and rescue. Marine Policy 108: 103662. doi:10.1016/j.marpol.2019.103662.
- Fuentes-Franco, R., D. Docquier, T. Koenigk, K. Zimmermann, and F. Giorgi. 2023. Winter heavy precipitation events over Northern Europe modulated by a weaker NAO variability by the end of the 21st century. npj Climate and Atmospheric Science6, no. 1. doi:10.1038/S41612-023-00396-1.
- García-Serrano, J., C. Frankignoul, G. Gastineau, and A. de la Cámara. 2015. On the predictability of the winter Euro-Atlantic climate: Lagged influence of autumn Arctic Sea Ice. Journal of Climate 28, no. 13: 5195–216. doi:10.1175/JCLI-D-14-00472.1.
- Grafton, R. Q. 2010. Adaptation to climate change in marine capture fisheries. Marine Policy 34, no. 3: 606–15. doi:10.1016/j.marpol.2009.11.011.
- Hall-Spencer, J. M., and B. P. Harvey. 2019. Ocean acidification impacts on coastal ecosystem services due to habitat degradation. Emerging Topics in Life Sciences 3, no. 2: 197–206. doi:10.1042/ETLS20180117.
- Heck, N., M. W. Beck, and B. Reguero. 2021. Storm risk and marine fisheries: A global assessment. Marine Policy 132: 104698. doi:10.1016/j.marpol.2021.104698.
- Hersbach, H., B. Bell, P. Berrisford, S. Hirahara, A. Horányi, J. Muñoz‐Sabater, J. Nicolas, et al. 2020. The ERA5 global reanalysis. Quarterly Journal of the Royal Meteorological Society 146, no. 730: 1999–2049. doi:10.1002/qj.3803.
- Hobday, A. J., K. Cochrane, N. Downey-Breedt, J. Howard, S. Aswani, V. Byfield, G. Duggan, et al. 2016. Planning adaptation to climate change in fast-warming marine regions with seafood-dependent coastal communities. Reviews in Fish Biology and Fisheries 26, no. 2: 249–64. doi:10.1007/s11160-016-9419-0.
- Hurrell, J. W. 1995. Decadal trends in the North Atlantic oscillation: Regional temperatures and precipitation. Science 269, no. 5224: 676–9. doi:10.1126/science.269.5224.676.
- Inoue, J., M. E. Hori, and K. Takaya. 2012. The role of Barents Sea Ice in the wintertime cyclone track and emergence of a warm-Arctic cold-Siberian anomaly. Journal of Climate 25, no. 7: 2561–8. doi:10.1175/JCLI-D-11-00449.1.
- Intergovernmental Panel on Climate Change [IPCC]. 2021. Climate Change 2021: The Physical Science Basis. Contribution of Working Group I to the Sixth Assessment Report of the Intergovernmental Panel on Climate Change, IPCC, Cambridge, United Kingdom and New York, NY, USA: Cambridge University Press. doi:10.1017/9781009157896.
- Jara, H. J., J. Tam, B. G. Reguero, F. Ganoza, G. Castillo, C. Y. Romero, M. Gévaudan, and A. A. Sánchez. 2020. Current and future socio-ecological vulnerability and adaptation of artisanal fisheries communities in Peru, the case of the Huaura province. Marine Policy 119: 104003. doi:10.1016/j.marpol.2020.104003.
- Johnson, J. E., and D. J. Welch. 2009. Marine fisheries management in a changing climate: A review of vulnerability and future options. Reviews in Fisheries Science 18, no. 1: 106–24. doi:10.1080/10641260903434557.
- King, M. P., M. Hell, and N. Keenlyside. 2016. Investigation of the atmospheric mechanisms related to the autumn sea ice and winter circulation link in the Northern Hemisphere. Climate Dynamics 46, no. 3–4: 1185–95. doi:10.1007/s00382-015-2639-5.
- Koenigk, T., and R. Fuentes‐Franco. 2019. Towards normal Siberian winter temperatures? International Journal of Climatology 39, no. 11: 4567–74. doi:10.1002/joc.6099.
- Koivurova, T., H. Tervo, and A. Stepien. 2008. A background paper: Indigenous peoples in the Arctic. Arctic Transform. https://www.arctic-transform.eu/download/IndigPeoBP.pdf.
- Kontogianni, A., D. Damigos, T. Kyrtzoglou, C. Tourkolias, and M. Skourtos. 2019. Development of a composite climate change vulnerability index for small craft harbours. Environmental Hazards 18, no. 2: 173–90. doi:10.1080/17477891.2018.1512469.
- Lahsen, M., and J. Ribot. 2021. Politics of attributing extreme events and disasters to climate change. WIREs Climate Change 13. doi:10.1002/wcc.750.
- Lomonico, S., M. G. Gleason, J. R. Wilson, D. Bradley, K. Kauer, R. J. Bell, and T. Dempsey. 2021. Opportunities for fishery partnerships to advance climate-ready fisheries science and management. Marine Policy 123: 104252. doi:10.1016/j.marpol.2020.104252.
- Macusi, E. D., E. S. Macusi, L. A. Jimenez, and J. P. Catam-isan. 2020. Climate change vulnerability and perceived impacts on small-scale fisheries in eastern Mindanao. Ocean & Coastal Management 189: 105143. doi:10.1016/j.ocecoaman.2020.105143.
- Maher, N., S. Milinski, and R. Ludwig. 2021. Large ensemble climate model simulations: Introduction, overview, and future prospects for utilising multiple types of large ensemble. Earth System Dynamics 12: 401–18. doi:10.5194/esd-12-401-2021.
- McCusker, K. E., J. C. Fyfe, and M. Sigmond. 2016. Twenty-five winters of unexpected Eurasian cooling unlikely due to Arctic sea-ice loss. Nature Geosciences 9, no. 11: 838–42. doi:10.1038/ngeo2820.
- Mori, M., M. Watanabe, H. Shiogama, J. Inoue, and M. Kimoto. 2014. Robust Arctic sea-ice influence on the frequent Eurasian cold winters in past decades. Nature Geosciences 7, no. 12: 869–73. doi:10.1038/ngeo2277.
- Ogawa, F., N. Keenlyside, Y. Gao, T. Koenigk, S. Yang, L. Suo, T. Wang, et al. 2018. Evaluating impacts of recent Arctic Sea Ice loss on the Northern Hemisphere winter climate change. Geophysical Research Letters 45, no. 7: 3255–63. doi:10.1002/2017GL076502.
- O’Neill, B. C., C. Tebaldi, D. P. van Vuuren, V. Eyring, P. Friedlingstein, G. Hurtt, R. Knutti, et al. 2016. The Scenario Model Intercomparison Project (ScenarioMIP) for CMIP6. Geoscientific Model Development 9, no. 9: 3461–82. doi:10.5194/gmd-9-3461-2016.
- Pinnegar, J. K., P. J. Wright, K. Maltby, and A. Garrett. 2020. The impacts of climate change on fisheries, relevant to the coastal and marine environment around the UK. MCCIP Science Review 2020 26. doi:10.14465/2020.ARC20.FIS.
- Reid, G., H. Gurney-Smith, M. Flaherty, A. Garber, I. Forster, K. Brewer-Dalton, D. Knowler, et al. 2019. Climate change and aquaculture: Considering adaptation potential. Aquacultural Environment Interactions 11: 603–24. doi:10.3354/aei00333.
- Reid-Musson, E., J. Finnis, and B. Neis. 2021. Bridging fragmented knowledge between forecasting and fishing communities: Co-managed decisions on weather delays in Nova Scotia’s lobster season openings. Applied Geography 133: 102478. doi:10.1016/j.apgeog.2021.102478.
- Rogers, J. C. 1984. The association between the North Atlantic Oscillation and the Southern Oscillation in the Northern Hemisphere. Monthly Weather Review 112, no. 10: 1999–2015. doi:10.1175/1520-0493(1984)112<1999:TABTNA>2.0.CO;2.
- Rousset, C., M. Vancoppenolle, G. Madec, T. Fichefet, S. Flavoni, A. Barthélemy, R. Benshila, et al. 2015. The Louvain-La-Neuve sea ice model LIM3.6: Global and regional capabilities. Geoscientific Model Development 8, no. 10: 2991–3005. doi:10.5194/gmd-8-2991-2015.
- Sainsbury, N. C., M. J. Genner, G. R. Saville, J. K. Pinnegar, C. K. O’Neill, S. D. Simpson, and R. A. Turner. 2018. Changing storminess and global capture fisheries. Nature Climate Change 8, no. 8: 655–9. doi:10.1038/s41558-018-0206-x.
- Savo, V., C. Morton, and D. Lepofsky. 2017. Impacts of climate change for coastal fishers and implications for fisheries. Fish and Fisheries 18, no. 5: 877–89. doi:10.1111/faf.12212.
- Smith, J. G. 2014. Where are all the fish? - A political ecology analysis of local fish networks and the gift economy in the Westfjords of Iceland. https://skemman.is/bitstream/1946/19613/1/Smith_Thesis_05262014-FINAL.pdf (accessed May 15, 2022).
- Turner, R., P. McConney, and I. Monnereau. 2020. Climate change adaptation and extreme weather in the small-scale fisheries of Dominica. Coastal Management 48, no. 5: 436–55. doi:10.1080/08920753.2020.1795970.
- Wyser, K., T. Koenigk, U. Fladrich, R. Fuentes-Franco, M. P. Karami, and T. Kruschke. 2021. The SMHI Large Ensemble (SMHI-LENS) with EC-Earth3.3.1. Geoscientific Model Development 14, no. 7: 4781–96. doi:10.5194/gmd-14-4781-2021.
- Young, O. R., D. G. Webster, M. E. Cox, J. Raakjær, L. Ø. Blaxekjær, N. Einarsson, R. A. Virginia, et al. 2018. Moving beyond panaceas in fisheries governance. Proceedings of the National Academy of Sciences of USA 115, no. 37: 9065–73. doi:10.1073/pnas.1716545115.
Appendix
Figure A1. Ninety-fifth percentile of daily mean 10 m wind speed (in m/s) calculated over the reference period 1970 to 2014 for (left) SMHI-LENS and (right) the ERA5 reanalysis.
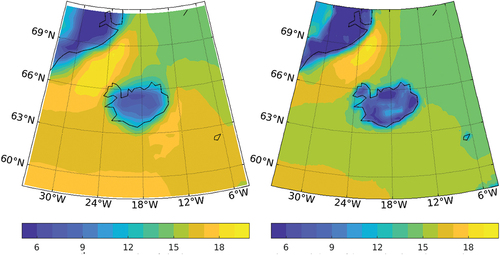
Figure A2. Number of storm days per year for an example grid box southeast of Iceland according to individual SMHI-LENS ensemble members (thin lines) and ensemble mean (thick line) for the historical period 1970 to 2014 (gray) and the future climate 2015–2100 according to four different SSP scenarios (colored).
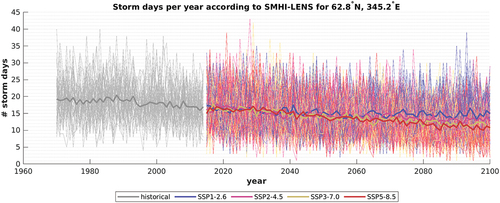