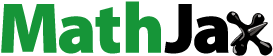
Abstract
During the operation of a fusion reactor, first wall components are exposed to the plasma and therefore tritium, resulting in generation of tritiated materials that would be classified as intermediate level waste (ILW) following their removal from the vessel. Investigations were undertaken into the thermal treatment of beryllium and tungsten representative of the materials used within the Joint European Torus (JET) fusion reactor to assess if tritium from these materials can be removed in the Material Detritiation Facility at the United Kingdom Atomic Energy Authority. This detritiation process may allow the reclassification of these materials as low level waste (LLW). When heated in the presence of oxygen, both tungsten and beryllium readily oxidize as temperature increases. The oxide layers that are formed on tungsten and beryllium surfaces are thought to act as a tritium barrier, reducing the amount of tritium that can be removed by thermal treatment. As such, the generation of oxide layers may need to be minimized for treatment of tungsten and beryllium, potentially via thermal treatment at lower temperatures. Additionally, the formation of beryllium oxide presents health and safety concerns due to its toxicity and physical form. Experiments were undertaken using tungsten and beryllium samples from previous JET campaigns. The samples were heated in a pyrolyzer, and the tritium released was captured in a series of bubblers. The remaining tritium in the material was characterized by acid dissolution to allow for detritiation factors (which are defined as the fraction of tritium inventory in the sample before and after the thermal treatment) to be calculated. Tritium was successfully removed from the samples by thermal treatment in air. Future trials will use samples with larger tritium inventory to confirm obtained results and demonstrate the feasibility of thermal treatment as a detritiation method for tungsten and beryllium on higher-activity samples. This should allow for samples representative of the JET ITER-like wall (current JET configuration) to be detritiated and could demonstrate the ability of the process to reduce the tritium inventory of JET materials and allow reclassification of components from ILW to LLW.
Keywords:
I. INTRODUCTION
This paper summarizes the investigations into beryllium and tungsten thermal treatment to remove tritium inventory, hereby referred to as detritiation, at United Kingdom Atomic Energy Authority (UKAEA). Key results from these investigations are reported.
Beryllium and tungsten are both important materials within the design and operation of nuclear fusion reactors. Both materials are used within the inner vessel as plasma-facing materials for various components due to their favorable properties such as high melting points and low hydrogen permeability. Despite these properties, significant tritium inventory [intermediate level waste (ILW)] levels ranging from 0.54 to 22.43 MBq/g[Citation1] are expected within these materials following the cessation of operations.[Citation2]
A Geological Disposal Facility (GDF) is currently planned to be used for the disposal of ILW in the United Kingdom. This facility is not yet constructed and operational, and hence, current stocks of ILW materials are awaiting final disposal in interim storage. It is currently forecast that the Joint European Torus (JET) fusion reactor system will be decommissioned and hence generate ILW materials prior to the GDF being operational.
To avoid interim storage of tritiated ILW fusion waste, thermal treatment has been demonstrated as a method to remove the tritium and therefore render materials suitable for disposal as low level waste (LLW). This technique is currently implemented for other former JET components at ILW levels such as stainless steel, carbon fiber composite (CFC), and Inconel at the UKAEA’s Materials Detritiation Facility (MDF). The MDF is an operational facility on the UKAEA Culham site built to thermally treat ILW following the first major deuterium-tritium experimental campaign (DTE1). The carbon-based ILW that was processed was successfully detritiated to allow reclassification from ILW to LLW. MDF utilizes an industrial-style furnace to heat the materials, which enhances the off-gassing of the tritium trapped in the surface and bulk of the materials.[Citation3] Not only would thermal treatment reduce the cost and environmental impact of disposal, but also the tritium removed from the materials can be recycled for reuse as fuel thus closing the fusion fuel cycle.
Although this technique is currently utilized for other metallic wastes at UKAEA, beryllium and tungsten pose an additional complexity due to the formation of oxide layers upon heating in air.Citation[4,Citation5] Oxide layers can act as a barrier for tritium permeation and therefore affect the detritiation.[Citation6] Higher temperatures have been shown to be more efficient at detritiating metals due to hydrogen isotopes from higher-energy trapping sites being released.Citation[7,Citation8] The temperature chosen is therefore a key consideration for thermal treatment to minimize oxide growth but maximize the removal of tritium from materials.
The samples used in the oxidation and detritiation trials were either “clean,” non-JET samples or samples that have originated from the ITER-likeFootnotea wall campaigns at JET. The clean samples were used for oxidation trials whose purpose was to enable selection of the heating profile that would limit the level of oxidation. These clean samples were pure tungsten (≥99.95%) purchased from Goodfellow and beryllium grade S-65-H with purity 99.2%. The tungsten samples from JET were extracted from the divertor region of the reactor where plasma is discharged (specifically bulk tungsten divertor tile 5). The beryllium samples originated from the confining walls of the reactor [wide poloidal limiter (WPL) tiles and inner wall guard limiter (IWGL) tiles]. [Citation9] and show tungsten and beryllium tiles and their position in the JET vessel.
The samples from JET, although having experienced plasma conditions, have not been exposed to tritium as the ITER-like wall campaigns utilized deuterium plasmas rather than any tritium-containing plasmas. This means that any tritium found within the materials would have originated from either D-D fusion reactions [EquationEq. (1)(1)
(1) ] or existing contamination from previous campaigns using tritium[Citation10]:
where D = deuterium, T = tritium, and p = proton. Therefore, most of the samples are not expected to be highly tritiated.
II. EXPERIMENTS
For both tungsten and beryllium materials, the thermal treatment experiments were separated into a number of trials. These trials were undertaken either to investigate oxide layer formation during heating or to quantify the detritiation efficiency of the thermal treatment. All experiments were undertaken in a ventilated enclosure using a Raddec 6 Trio pyrolyzer connected to a series of water bubblers to capture any tritiated species released. The simplified scheme of pyrolyzer tube and bubbler sets are shown in .
For the detritiation experiments, water from the bubbler is tested by liquid scintillation counting to quantify the tritium content captured. Acid dissolution of the sample post pyrolysis allows for the remaining tritium in the samples to be quantified by capturing any tritium gas released as the sample dissolves. In each trial, gas conditions and flow rate were kept consistent.
II.A. Oxidation
The oxidation of clean tungsten samples was investigated by heating various samples to different temperatures and soaking for set periods of time at those temperatures in the pyrolyzer and recording the mass and color changes. The results are shown in . Beryllium was tested in a similar manner to tungsten, with the results also given in .
TABLE I Tungsten and Beryllium Oxidation Trials
II.B. Detritiation
Using the information from the oxidation experiments to generate appropriate heating profiles, trials were undertaken using samples from JET ITER-like wall campaigns to investigate tritium removal. Detritiation factors (DFs) and extraction factors are defined as
where E = extraction factor, C0 and CF = tritium inventories in pretest and posttest samples, and DF = detritiation factor. Detritiation factors were calculated from the tungsten trials and are shown in . The results obtained by acid dissolution are all at the limit of detection (LOD) or below, which is 37 Bq. The LOD for W7 is higher (127 Bq) because this sample was analyzed through a modified method. The results and LOD values are provided by a laboratory that carried out the radiochemical analysis of samples.
TABLE II Tungsten Detritiation Trials
For beryllium, DFs were calculated for three samples that were used during these trials ().
TABLE III Beryllium Detritiation Trials
III. DISCUSSION
The tungsten samples all initially have a gray metallic luster. Upon heating, the tungsten transitioned to a dull black/gray color, expected to be a layer of nonstoichiometric tungsten oxide.[Citation5] Heating higher than 650°C gave a thick yellow oxide layer, thought to be tungsten trioxide.[Citation5] For all samples, initially, the mass decreased before increasing again as tungsten trioxide formed. This initial mass decrease could be due to fluids trapped in the samples being liberated as well as the potential formation of volatile oxides.
Beryllium shows a color change from a gray metallic luster to a dull black/gray color. At higher temperatures, the initiating of catastrophic oxidation is seen,[Citation4] with white grains seen in Trial 4 and a white film seen in Trial 5 (see ), both of which are expected to be beryllium oxide. For Trials 4 and 5, the mass decreased when heating, and the formation of this loose beryllium layer is hypothesized to be why there was significant white discoloration on the pyrolyzer tubes. This beryllium oxide formation is problematic as significant beryllium loss in the form of dust during detritiation could be hazardous. These oxidation characteristics need to be considered when planning any detritiation activities.
For the detritiation experiments, in each tungsten sample it was proven that tritium was removed as a result of thermal treatment. However, as previously discussed, these samples originate from the ILW1-3 campaigns, and therefore, the tritium content for all samples is low when considering the expected tritium levels in JET following deuterium-tritium experimental campaign 3 (DTE3),[Citation1] with some measurements at LOD levels. Because of the low tritium content, the DFs and extraction percentages are heavily limited by the LOD for the measurement techniques, with the limits of detection for the dissolution step being a significant percentage of the total tritium for some measurements. Despite this being the case, for samples W6 through W9, high extraction percentages were calculated, with the result for W7, the sample with the most tritium, being particularly promising.
For beryllium, tritium was removed from all samples, but the initial tritium levels were low. Sample Be8 had the most tritium content and showed promising results, with a detritiation factor of 121 and a tritium extraction of 99%.
The results from the detritiation experiments show that further investigation is required to display ILW to LLW detritiation due to the low initial tritium inventory. Calculated DFs and uncertainty values have generally been low as the levels of contamination have approached the LOD. However, the samples with higher tritium content have shown higher extraction factors close to proven metals such as Inconel and stainless steel.[Citation11]
Currently, the experiments with samples containing higher tritium concentrations are being carried out. The higher concentration in the samples is achieved by soaking samples in tritiated atmospheres by an external laboratory. Although it would not be the most accurate representation of how the tritium would be loaded into the materials inside the vessel, it will allow for DFs of a higher magnitude to be achieved as the samples will be ILW activity levels and will therefore better represent how effective detritiation of these materials in air will be. As well as detritiating these samples in air, inert gas experiments on high tritium concentration samples will take place to see if that is more effective than detritiating in air.
IV. CONCLUSION
Beryllium and tungsten are both important plasma-facing materials for fusion reactors and are currently used in JET and are planned to be implemented in ITER. These materials accumulate tritium during operations, meaning when operations cease and the materials are being managed as waste, they fall into the ILW category. To manage this, thermal treatment is being considered as a method of removing the tritium.
Upon heating, both tungsten and beryllium oxidize, forming an oxide film on the metal surface. This film acts to trap tritium within, reducing the effectiveness of thermal treatments. In the case of beryllium, it also leads to the formation of hazardous BeO dust particles. The formation of oxide film needs to be considered when choosing the parameters for detritiation.
Detritiation trials showed that for both beryllium and tungsten, tritium could be readily removed by thermal treatment. The scale at which tritium was removed was heavily limited by the total tritium in the samples, which was low in this experiment. Regardless, high DFs of 121 and 16.95 were achieved for samples of beryllium and tungsten representing a 99% and 94% reduction in total tritium content, respectively. Further trials will be undertaken with ILW samples to determine if ILW to LLW detritiation is achievable.
Acknowledgments
This work has been carried out in collaboration with the Tritium Analysis Laboratory, which is part of the Hydrogen-3 Advanced Technology Division, whose team provided equipment and time for carrying out these experiments safely and reliably. The work has been carried out as a part of Detritiation Research and Development for the JET Decommissioning and Repurposing Programme.
Disclosure Statement
No potential conflict of interest was reported by the authors.
Notes
a International Thermonuclear Experimental Reactor (ITER) is a fusion experimental reactor under construction in Cadarache, France. The in-vessel configuration of ITER was tested in JET; therefore, such configuration in JET is referred to as ITER-like wall.
References
- A. WIDDOWSON et al., “Evaluation of Tritium Retention in Plasma Facing Components During JET Tritium Operations,” Phys. Scr., 96, 12, 8 (2021); https://doi.org/10.1088/1402-4896/ac3b30.
- A. WIDDOWSON et al., “Overview of Fuel Inventory in JET with ITER-Like Wall,” Nucl. Fusion, 57, 8, 086045 (2017); https://doi.org/10.1088/1741-4326/aa7475.
- M. KRESINA et al., “Preparation for Commissioning of Materials Detritiation Facility at Culham Science Centre,” Fusion Eng. Des., 136, 1391 (2018); https://doi.org/10.1016/j.fusengdes.2018.05.019.
- C. TOMASTIK, W. WERNER, and H. STORI, “Oxidation of Beryllium—A Scanning Auger Investigation,” Nucl. Fusion, 45, 9, 1061 (2005); https://doi.org/10.1088/0029-5515/45/9/005.
- J. HABAINY and C. NILSSON, “Oxidation of Pure Tungsten in the Temperature Interval 400° to 900℃,” Lund University (2013).
- P. PACENTI, R. EDWARDS, and F. CAMPI, “Detritiation of Graphite and Beryllium Plasma Facing Components,” Proc. 19th Symp. Fusion Technology – Fusion Technology 1996, Lisbon, Portugal, September 16–20, 1996, p. 1705, North-Holland (1996).
- L. AVOTINA et al., “Thermal Desorption of Hydrogen Isotopes from the JET Be Plasma Facing Components,” Phys. Scr., T171, 014009 (2020).
- X. YE et al., “Determination of the Detrapping Energy of Tritium in Tungsten,” J. Nucl. Mater., 544, 152662 (2021).
- “Saving Time on the Way to ITER Operation,” ITER Newsline (May 15, 2017); https://www.iter.org/newsline/-/2722.
- S. ATZENI and J. MAYER-TER-VEHN, The Physics of Inertial Fusion, Oxford University Press (2009).
- S. REYNOLDS, “Materials Detritiation Facility Process Management and Achieved Detritiation Factors,” EUROfusion (2019).