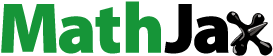
Abstract
Tritium is a radioactive isotope of hydrogen, and due to its limited supply and related high price, it is necessary to recover it from tritiated materials via detritiation technologies. The number of tritiated materials arises during fusion. Tritium from fusion reactors is deposited in the outer layer of plasma-facing materials, which must be dealt with during maintenance or decommissioning processes. A second source of waste is laboratory equipment that could be contaminated with tritium.
High-temperature treatment in an oxidation environment can achieve the release of tritium from metals or organic materials. The tritiated vapor is then captured in a series of water bubblers and reprocessed into pure T2. One of the high-temperature methods is molten salt oxidation (MSO), which uses high temperatures, alkaline salt, and an oxidative environment for flameless oxidation of different types of waste.
This work aimed to simulate the detritiation processes of tritiated organic materials in MSO technology. First, a series of experiments with D2O absorbed in ion resins was conducted. The organic waste was decomposed within the molten salt, and the flue gas was measured to determine the oxidation efficiency. The D2O was captured in a series of water bubblers, and the water was then analyzed with attenuated total reflectance (ATR)–Fourier transform infrared spectroscopy (FTIR). The results showed that all deuterium in the form of D2O was caught in the first water bubbler. The capture efficiency ranged from 22.32% to 61.13%. The lower efficiency capture can be explained as D2O in water can form a HDO molecule and its correct determination is problematic.
The second type of experiment was carried out with T2O with an activity of 851 Bq and with tritiated oil with an activity of 2495 Bq. The T2O was added to the set amount of ion resins and dosed into MSO. A peristaltic pump dosed the tritiated oil. The flue gas was measured to determine oxidation efficiency, and the T2O was captured within the water bubblers. The water was analyzed with liquid scintillation spectroscopy. The capture efficiency ranged between 76.42% to 97.87%. The results showed that this technology is suitable for the detritiation of tritiated organic materials.
I. INTRODUCTION
Detritiation is one of the most needed decommissioning technologies. Due to its high price, it is necessary to recover amounts of tritium from tritiated materials. These materials arise during fusion, and there are several methods that can obtain the tritium from tritiated materials. One of the methods for detritiation of the metal parts is high-temperature metal treatment.[Citation1] A high-temperature treatment can be used for detritiation of the housekeeping and processing waste arising from fusion facility operations.
These wastes include personal protective equipment (protective clothing, gloves, disposable foot coverings, etc.), and waste arising from decontamination. It is predicted that approximately 280 m3 of housekeeping and process waste will arise every year during the operation of ITER with (using the radioactive waste framework of the host country) the very low–level waste amount of 80 m3, low- and intermediate-level short-lived waste of up to 200 m3, and intermediate-level long-lived waste less than 1 m3. It can be anticipated that the amount of this type of waste in a future DEMO plant could be maintained at no higher than that in ITER.[Citation2,Citation3]
The demand for a low-cost procedure for tritium fuel is high for fusion reactors due to the high price of tritium, which is about $10 000 per 1 g.[Citation4] That is because the natural occurrence of tritium is low, and it still needs to be purified prior to its usage as a fuel. Tritium is primarily produced in a breeding blanket by the following reactions:
A breeding blanket is a cover of the interior of the fusion reactor vessel that ensures the reaction between neutrons and Li isotopes (reactions 1 and 2). Tritium generated during the reactions will be used in the reactor as fuel. The first reaction is preferable due to its high positive energy output and because it ensures energetical self-sufficiency, which is also important from the perspective of the reaction cross section for neutron energy. In EU-DEMO breeding blankets, the concept aimed for is lithium lead breeding blankets, which use higher enriched lithium content (6Li ≈ 90%) breeding blankets.[Citation5]
I.A. Molten Salt Oxidation
Molten salt oxidation (MSO) is a thermal treatment process of organic and organic radioactive waste. It is considered an alternative to conventional incineration, and uses the flameless oxidation of waste materials with the consequent capture of heavy metals and radionuclides in the alkaline salt, which can then be reprocessed. During the process, the organic wastes are dosed together with the oxidizing agent (O2, air) below the surface of molten salts, where flameless combustion and the conversion of wastes to CO2 and water vapor proceed. If the system is properly adjusted and operated under controlled conditions, the output components are CO2, CO, NOx, and SOx as flue gases, depending on the waste type and salt mixture with the captured elements or radioisotopes.
II. EXPERIMENTAL
II.A. Laboratory MSO Apparatus
The MSO laboratory apparatus consists of several parts, as shown in . The dosing device differs for solid organic waste and liquid organic waste. Liquid organic waste is dosed via a peristaltic pump, and the solid fuel dosing device with a hopper is used for ion exchange resin dosing. The dosing device consists of an engine that drives a conveyor screw. The ion exchange resin from the hopper is forced into the dosing tube, where it is mixed with the oxidizer, which in this case is air from a pressure bottle. The reactor part consists of a heating part, a reactor vessel, and a stainless steel crucible in which the alkali salt itself is placed.
Fig. 1. Schematic picture of the MSO laboratory apparatus: (1) air supply, (2) ion exchange resin dosing, (3) reactor 1, (4) reactor 2, (5) series of bubblers, (6) diaphragm pump, (7) flue gas analyzer, and (8) discharge to active air conditioning.
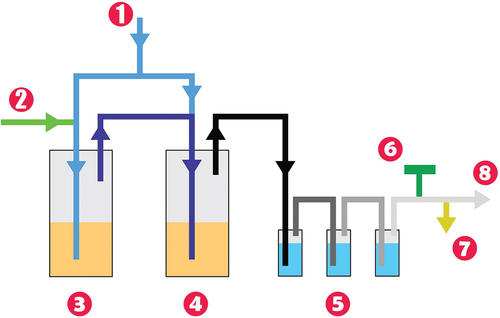
The fuel and air are dosed under the molten salt surface in the first reactor via a dosing tube. During the process, the sulfur released after the combustion of the ion exchange resin is captured in the molten salt, and the flue gas in the form of CO, CO2, H2O, D2O, and T2O leaves for the second reactor, again below the surface of the molten salt. To increase efficiency, it is possible to feed part of the air to the second reactor as well. From the second reactor, the flue gas goes to a set of three bubblers, which are filled with demineralized water. A diaphragm pump is located at the end of the apparatus for better air passage through the apparatus.
II.B. Chemicals
Each reactor used 2000 g of pure Na2CO3 (Lach-Ner s.r.o., Neratovice, Czech Republic) salt with a melting point of 1124 K. The highly regenerable mixed-bed ion exchange resin Lewatit NM 60 (Lenntech B.V., Delgauw, Netherlands) was chosen. We simulated the contaminated organic waste by absorbing tritium or deuterium water in the ion exchange resin. The ion exchange resin was dried, and then a specified amount of heavy water (D2O) was added to the weighed amount so that the ratio of the ion exchange resin to D2O was 2:1. This ratio was determined to be ideal in terms of the dosage and a sufficient volume of D2O to effectively analyze it.
For detritiation of solid organic waste, Lewatit NM 60 was also used with an added solution of T2O of activity 851 kBq (Hartmann Analytic GmbH., Braunschweig, Germany), which was used as a source of tritium. The ion exchange resin was dried, and then a specified amount of demineralized water and tritiated water was added to the weighed amount so that the ratio of the ion exchange resin to T2O was 2:1. For detritiation of liquid organic waste, the oil Mogul TB32 S (Paramo a.s., Kolín, Czech Republic) was tritiated to 999 MBq (Hartmann Analytic GmbH, Braunschweig, Germany) and diluted before each experiment.
II.C. Equipment
II.C.1. Digital Laboratory Scale
The scale RADWAG, WLC 6/A2 was used for weighing the chemicals and samples. The accuracy of the scale was ±1.0 × 10−3 kg. The expected uncertainty assigned corresponded to a coverage probability of 95% and the coverage factor k = 2.
II.C.2. Flue Gas Analysis
The flue gas composition was measured continuously on a portable Testo 350 analyzer, which was equipped with sensors for measuring O2, CO, CO2, NO, NOx, and SO2. For the O2 probe, the measurement accuracy was ±0.8% with a resolution of 0.01 vol %. The CO measurement probe was with H2 compensation and had the stated range from 0 to 10 000 ppm with an accuracy of ±5% at concentrations (200 to 2000 ppm) and ±10% (2001 to 10 000 ppm), and the resolution was given as 1 ppm (0 to 10 000 ppm).
The CO2 sensor used an infrared spectrum for analysis ranging from 0 to 50 vol % with an accuracy of ±0.3 vol % (0 to 25 vol %) and ±0.5 vol % (>25 to 50 vol %), with a resolution of 0.01 vol % (0 to 25 vol %) and an accuracy of 0.1 vol % (>25 vol %).
The NO sensor had a specified measuring range of up to 4000 ppm with an accuracy of ±5% (100 to 1999 ppm) and ±10% (2000 to 4000 ppm), and a resolution of 1 ppm. The NOx measurement had a measuring range of up to 500 ppm with an accuracy of ±5% (100 to 500 ppm), and a resolution of 0.1 ppm (0 to 500 ppm). The SO2 sensor had a measuring range of up to 5000 ppm with an accuracy of ±5% (100 to 2000 ppm) and ±10% (2001 to 5000 ppm), and a resolution of 1 ppm (0 to 5000 ppm).
II.C.3. Deuterium Analysis
The attenuated total reflectance (ATR)–Fourier transform infrared spectroscopy (FTIR) method for determination of the deuterium in the demineralized water from the bubblers was used. The spectra were measured on a Nicolet iS50 FTIR spectrometer (Thermo Fisher Scientific, United States) equipped with the ATR accessory containing the diamond crystal. The spectra were collected at wave numbers ranging from 4000 to 400 cm−1, with a resolution of 4 cm−1 and 120 scans. The background (air) was measured before each sample measurement.
II.C.4. Liquid Scintillation Spectroscopy
The Triathler Hidex analyzer, which uses a single-photon counting photomultiplier tube detector with an energy range of 2 to 2000 keV, was used to measure the tritiated samples. The maximum values of liquid scintillation were 2 000 000 counts per minute with a beta radiation efficiency of 48% for 3H. To increase the measurement efficiency, the device was shielded with a 10-mm lead box.
II.D. Experimental Setting
II.D.1. Deuterated Solid Organics
For each experiment, a batch of Na2CO3 with 2000 g was prepared in each reactor. The temperature was set at 950°C in each reactor. The airflow differed during each experiment, with stable pressure of 250 kPa from a cylinder. The data for each experiment are shown in .
TABLE I Data for Each Experiment for Deuterium Removal
II.D.2. Tritiated Solid Organics
One experiment was made with tritiated solid organics. The experimental setting was set with 2000 g of Na2CO3 in each reactor. The temperature was 950°C in each reactor. Air from the cylinder was fed to the first reactor at a pressure of 250 kPa, and the flow rate to the first reactor was set at 5.5 l/min. The airflow to the second reactor was set at 0.8 l/min. The amount of tritiated ion resins was 36.03 g with 11 g of H2O with 1 g of T2O with an activity of 851 kBq. The dosing speed was 1.1 g/min.
II.D.3. Tritiated Liquid Organics
Two experiments were made with a batch of 2000 g of Na2CO3 in each reactor. The temperature was set to 950°C in each reactor. Air from the cylinder was fed to the first reactor at a pressure of 250 kPa, and airflow to the first reactor was set to 2.5 l/min and airflow to the second reactor was set to 1 l/min. The flow through the peristaltic pump was set at 2 ml/min. Tritiated oil was diluted to an activity of 2495 Bq with a weight of 18.72 g, and dosed into the first reactor. The weight of the tritiated oil was the same for each experiment. Before dosing the tritiated oil, the nonradioactive oil was dosed to start the experiment, and after dosing the tritiated oil, it was used again to rinse out the radioactive oil.
III. RESULTS AND DISCUSSION
III.A. Deuterated Solid Organic Waste
III.A.1. Experiment 1
shows the flue gas measurement output. The flue gas measurement is needed for the determination of organic matter decomposition efficiency and for process optimization. If the efficiency is low, tar and char compounds could be formed and capture the tritium within. This would lead to low detritiation efficiency.
The first thing that happened during each experiment was the so-called “shock.” This condition occurred almost immediately after the start of the dosing (time about 300 s). This was caused by the automatic feature in the analyzer, which adjusts the volume of analyzed flue gas.
The maximum measured CO concentration was 74 900 ppm, while the SO2 concentration was a maximum of 5300 ppm. The CO values after stabilization of the oxidation process were reduced to 13 000 ppm. The SO2 values after dilution did not exceed 300 ppm. The NO and NOx values were negligible during the process and did not exceed 65 ppm.
Captured deuterium in the form of D2O and HDO was detected only in the first bubbler. The amount of D2O captured was 4.19 g, which was 31.9% of the dosed amount. More deuterium was most likely present in the form of HDO, which could be determined but could not be quantified.
III.A.2. Experiment 2
The results of the flue gas measurement are shown in . The experiment did not proceed according to the operator’s ideas, as the main flange at the first reactor did not seal properly. In the first minutes, therefore, there was a partial evaporation of the flue gases into the extractor fan. However, in the first shock, about 25 000 ppm of CO and about 500 ppm of SO2 were still measured. Compared to the previous experiment, the NO and NOx values were at lower levels, even when the molten salt was bubbled through the air.
Only a small amount of D2O, 3.09 g, was detected in the bubbler. No D2O was detected in the second bubbler. Although we anticipated the inability to quantify the deuterium content of HDO in the sample, the yield of D2O was lower, even compared to previous experiments, at 22.32%.
III.A.3. Experiment 3
The third experiment differed from the previous experiments by the use of the new dosing conveyor, which ensured the dosing of a lower amount, and thus increased the efficiency of the MSO process. Also, the air was dosing into the second reactor, which increased the combustion efficiency. shows the course of ion exchange resin combustion during the third experiment.
The CO values did not increase above 900 ppm, which was not the case in the previous experiments. Overall, the CO values were lower, on average around 200 ppm. The SO2 values were almost zero, so there was a total capture of sulfur in the molten salt as Na2SO4. However, the NO and NOx values were higher, and gradually increased to 170 ppm. These values corresponded to the previous experiments, where the NO and NOx values also ranged on the order of tens and hundreds of ppm. Experiment 3 showed that when dosing a lower amount of solid fuel, the combustion of wet ion exchange resin could be done very efficiently.
In this experiment, the amount of captured D2O was the highest of all the experiments at 7.33 g, which was 61.13% of the dosed amount, and as in all the other experiments, no deuterium was detected in the second bubbler (see ).
TABLE II Yield of D2O in All Experiments
III.B. Tritiated Solid Organic Waste
shows the course of the MSO process. The results of the experiment in terms of MSO efficiency were very promising. The CO values averaged around 300 ppm, and only in one case did the concentration increase to around 1300 ppm. The wet ion exchange resin tended to form clumps that adhered to the hopper wall and had to be mechanically detached from the wall. This was followed by the dosing of a larger amount of ion exchange resin, which caused a CO concentration increase in the flue gas. The SO2 values were again almost zero, which means an almost total capture of sulfur in the salt in the form of Na2SO4. The values of NO and NOx were higher, on the order of tens and hundreds of ppm. However, the values corresponded to other experiments.
The contents of the bubblers were analyzed on a liquid scintillation counter. The activity detected on the Triathler Hidex in the first bubbler was 719.4 Bq and in the second bubbler it was 113.5 Bq, which means a capture efficiency of 97.87%.
III.C. Tritiated Liquid Organic Waste
III.C.1. Experiment 1
A total of 46.02 g of nonradioactive oil and 18.72 g of tritiated oil were dosed during the first experiment. The flue gases were measured during the process, and the oxidation results are shown in .
During the first stage, the nonradioactive oil was dosed, and the CO values increased to 40 000 ppm, while the values of other pollutants remained around 600 ppm. The NO and NOx values remained low during the first dosing stage, but before the end of the dosing, these values increased to 1200 ppm.
In the second stage of dosing, an increase in the CO and SO2 values was experienced again. The CO values increased up to 60 000 ppm, while SO2 increased up to 900 ppm. This was followed by a decline in these concentrations, and the values of CO and SO2 remained at 20 000 ppm and 500 ppm, respectively.
In the second dosing stage, the tritiated oil and the oil for rinsing the dosing apparatus were gradually dosed. Overall, the oxidation did not proceed efficiently, as evidenced by the high concentrations of CO in the flue gas (see ). This was probably caused by lower airflow into each reactor. Even with the lower airflow into each reactor, the total capture efficiency was 76.42%.
IV. CONCLUSIONS
The aim of this work was to test the detritiation processes in the MSO process. The first experiments were carried out with deuterated ion resins that simulated solid organic waste. The next part of the detritiation experiments were carried out with tritiated ion resins, with applied experience from the deuterated ion resins. The last experiments were carried out with tritiated oil to simulate the detritiation of liquid organic waste.
The ion exchange resin combustion process in MSO did not proceed exactly according to earlier experience because adding moisture, in the form of D2O, to the process complicates it. For the first two experiments, the old type of conveyor screw was used, which supplied higher values of ion resins to the system. In the results, a high concentration of CO and SO2, and partly also NO and NOx, was formed. The CO values were quite high in each experiment, ranging from 12 000 to 30 000 ppm with dilution turned on.
In the initial phase of oxidation, the so-called shock values were from 30 000 to 75 000 ppm. The values of the other pollutants were considerably lower; however, they were still on the order of hundreds of ppm, which are still high values.
For the last experiment, the smaller conveyor screw was added, which slowed down the dosed amount of ion resins. During this experiment, the initial shock of ionex dosing was removed, and the overall CO values did not exceed, with one exception, 1000 ppm. The SO2 values were almost zero, and the NO and NOx values were almost identical to the previous experiments, on the order of tens and hundreds of ppm.
The FTIR instrumental method was used for the determination of the deuterium content in the samples. The capture efficiency varied according to the experiment, and ranged from 22.32% to 61.13%. The experiment with the lowest detection of 22.32% had leaks detected at the beginning of dosing, which were subsequently sealed.
The oxidation of tritiated ion resins within the molten salt was successful. The CO values averaged around 300 ppm, and only in one case did the concentration increase to around 1300 ppm. The values of NO and NOx were higher, on the order of tens and hundreds of ppm. However, the values corresponded to the other experiments. The activity detected on the Triathler Hidex in the first bubbler was 719.4 Bq, and in the second bubbler, it was 113.5 Bq, which meant a capture efficiency of 97.87%.
The oxidation process of the tritiated oil was not very effective. At the first peak, the CO values reached 40 000 ppm, then 60 000 ppm, and at the end of the experiment, 80 000 ppm. The SO2 values during the experiment averaged around 600 ppm. An increase in the concentration of NO and NOx, up to 1100 ppm, happened before the end of the oxidation, but after resuming the dosing, these values were negligible. After the experiments, it was found that during the process, the outlet paths from the reactor were clogged with soot. The activity measured in the first experiment was 1478.2 Bq in the first bubbler, while an activity of 355.8 Bq was measured in the second bubbler. The T2O capture efficiency in the first experiment was determined to be 76.42%.
Overall, the detritiation processes with solid organic waste were successful after optimization. The detritiation of liquid organic waste needs proper adjustments in the process and equipment for complete decomposition, but others will be carried out. The MSO process is suitable for the decomposition of organic waste, and with proper adjustments to the process, it is useable for different kinds of organic waste.
Acknowledgments
This work has been carried out within the framework of the EUROfusion Consortium, funded by the European Union via the Euratom Research and Training Programme (grant agreement no. 101052200 EUROfusion). The views and opinions expressed, however, are those of the authors only and do not necessarily reflect those of the European Union or the European Commission. Neither the European Union nor the European Commission can be held responsible for them.
Disclosure Statement
No potential conflict of interest was reported by the authors.
Additional information
Funding
References
- M. KRESINA et al., “Preparation for Commissioning of Materials Detritiation Facility at Culham Science Centre,” Fusion Eng. Des., 136, Part B, 1391 (Nov. 2018); https://doi.org/10.1016/j.fusengdes.2018.05.019.
- S. ROSANVALLON et al., “Waste Management Within the Framework of ITER in Cadarache,” Fusion Eng. Des., 69, 1–4, 531 (2003); http://dx.doi.org/10.1016/S0920-3796(03)00124-8.
- S. ROSANVALLON et al., “Waste Management Plans for ITER,” Fusion Eng. Des., 109–111, 1442 (2016); http://dx.doi.org/10.1016/j.fusengdes.2015.12.002.
- Book of Abstracts, First Tritium School, Jožef Stefan Institute (2019).
- K. BATTES, T. GIEGERICH, and C. DAY, “Lithium Enrichment Needs for Fusion and Fission Applications,” in Transat Project, First Tritium School (2019).