Abstract
Inadequate delivery of therapeutics into tumors has been suggested as a reason for poor response. We hypothesize that bevacizumab, an antibody to vascular endothelial growth factor (VEGF), can improve cetuximab uptake in squamous cell carcinoma tumors. Athymic nude mice were implanted with OSC19 and SCC1 human cancer lines in a subcutaneous flank model. Mice were imaged daily for 14 days after intravenous tail vein injections of the following groups: IgG-IRDye800 (Control), cetuximab-IRDye800 (CTX800 Only), bevacizumab-IRDye800 (BVZ800 Only), cetuximab-IRDye800 + bevacuzimuab-IRDye800 (Simultaneous), and unlabeled bevacizumab followed by cetuximab-IRDye800 3 days later (Neoadjuvant). Within single-agent groups, the CTX800 Only tumor-specific uptake (TSU) was significantly higher than BVZ800 Only at Day 13 (TSU 8.6 vs 2.8, P < 0.001). The Simultaneous treatment with BVZ800 and CTX800 demonstrated no increase in antibody delivery. However, administration of unlabeled bevacizumab 3 days prior to CTX800 (Neoadjuvant group) resulted in significantly higher tumor specific delivery than administration of both antibodies at the same time (11.8 vs Simultaneous 5.0, P < 0.001). This difference can be attributed to a slower decline in tumor fluorescence intensity (−6.8% vs. Simultaneous −11.5% per day, respectively). Structural changes in pericyte coverage and functional vessel changes demonstrating decreased proliferation and tumor growth corroborate these fluorescence results. Although simultaneous administration of bevacizumab with cetuximab failed to increase antibody delivery to the tumor, pretreatment with bevacizumab improved TSU reflecting an increase in tumor-specific uptake of cetuximab as a result of vessel normalization.
Abbreviations
EGFR | = | endothelial growth factor receptor |
VEGF | = | vascular endothelial growth factor |
HER2 | = | human epidermal growth factor receptor 2 |
HNSCC | = | head and neck squamous cell carcinoma |
TSU | = | tumor specific uptake |
CTX800 | = | cetuximab-IRDye800 |
BVZ800 | = | bevacuzimab-IRDye800 |
EPR | = | enhanced permeability and retention |
Introduction
Identification of cell surface molecular targets in solid tumors has led to the development of antibody-based therapies that directly modulate pathologic pathways but their therapeutic success has been mixed. Although expression levels and mutations in cell signaling have been rigorously studied to guide treatment, the paucity of predictive biomarkers limits our ability to monitor response. As a result, it is unclear whether a particular therapeutic is simply ineffective in a subset of patients or whether therapeutics that would otherwise be effective are unable to reach their therapeutic targets. Recent work has suggested that the antibody itself is unreliably delivered to the tumorCitation1 and that abnormalities in tumor angiogenesis and vessel structure contribute to multiple downstream effects that impede therapeutic delivery including leaky vasculature, elevated interstitial pressure, and heterogeneity in oxygen delivery and nutrient exchange.Citation2 These tumor vascular changes have been shown to alter the distribution of intravenously-administered small moleculeCitation3 and antibody-basedCitation4 therapeutics in preclinical models. It stands to reason, then, that interventions to modulate tumor vessels may improve therapeutic delivery and optimize tumor response.
Aberrant tumor vessel formation begins with tissue hypoxia. Hypoxia within tumors triggers an increased production and release of proangiogenic factors including VEGF. Elevated VEGF levels cause increased vascular branching and a higher proportion of immature vessels. A structural hallmark of immature tumor vasculature is the decreased number and the disassociation of pericytes from vascular endothelium, resulting in the loss of its normal barrier function.Citation5 The presence of VEGF in platelet-derived growth factor (PDGF)-mediated angiogenesis inhibits pericyte coverage in immature vessels.Citation6 Bevacizumab, an antibody to the VEGF ligand, has been investigated as a targeted mechanism to normalize vessels in both preclinical and some clinical studies.Citation7 While these studies support the principle of vessel normalization to facilitate delivery of small molecules,Citation8,9 very few studies demonstrate improved delivery of larger, targeted cancer therapeutics such as monoclonal antibodies. Furthermore, the use of fluorescently-labeled antibodies as a means of measuring therapeutic uptake in both the tumor and the systemic circulation have not been applied to studies in vessel normalization.
In this study, we hypothesize that bevacizumab, an antibody to vascular endothelial growth factor (VEGF), can modulate tumor vasculature and improve cetuximab uptake in head and neck squamous cell carcinoma (HNSCC) tumors. To measure tumor delivery we present a novel in-vivo near-infrared fluorescence imaging methodology to monitor tumor uptake of cetuximab by labeling it with a near-infrared fluorescent probe, IRDye800.
Results
To determine the relative delivery of fluorescently labeled antibodies, we assessed a range of FDA-approved therapeutic antibodies in an orthotopic model of head and neck tumors using an OSC-19 cell line. The IgG-IRDye800 group had the lowest TSU of 1.0 on day of sacrifice (Day 2). Representative images of each antibody-dye conjugate and mean tumor specific uptake (TSU) as measured by fluorescence intensity over background demonstrated that cetuximab-IRDye800 and panitumumab-IRDye800 bioconjugates had significantly greater uptake than bevacizumab or trastuzumab for both imaging modalities (SPY P < 0.001 and Pearl p = 0.03; ). Given similar tumor delivery as measured by optical fluorescence, cetuximab was chosen over panitumumab since it approved for clinical investigation in head and neck cancer in addition to its lower variance.
Figure 1. Imaging and Quantification of OSC-19 Orthotopic Model. (A) Imaging on Day 2 of the tongue using SPY (left) and Pearl (right). (B) Quantification of Tumor-to-Background Ratio (TBR) with mean ± SD plotted. (C) Immunohistochemistry and H&E stains demonstrating EGFR, HER2, and VEGF distributions in representative tumors.
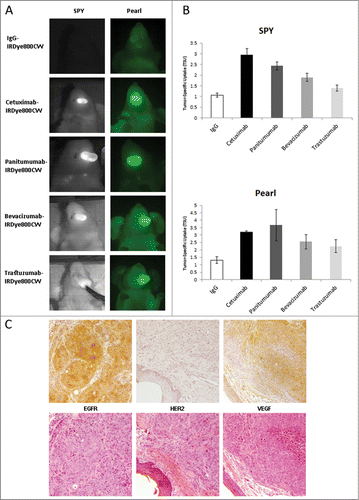
Immunohistochemical staining for the primary target for each of our antibodies confirmed high EGFR expression, minimal HER2 expression and moderate levels of VEGF within tumors of this cell line (), consistent with our optical findings. In addition, hematoxylin and eosin staining was used to co-localize the presence of cancer within tongue tumors (data not shown).
In order to study tumors of sufficient size for tumor vasculature study, a flank tumor model was utilized to study the potential benefit of anti-VEGF therapy to improve therapeutic delivery of cetuximab. Animals received systemic administration of cetuximab-IRDye800 (CTX800 Only), bevacizumab-IRDye800 (BVZ800 Only), or IgG-IRDye800 (control) with established OSC-19 flank tumors and daily TSU and representative images were obtained over a 14 day period ().
Figure 2. Quantification and Representative Images of OSC-19 Flank Model. (A) Daily imaging TSU values for all 5 treatment groups with mean ± SD plotted. (B) Representative images for all 5 treatment groups for Day 3, 7 and 14. Exposure times and aperture settings are equivalent for all images.
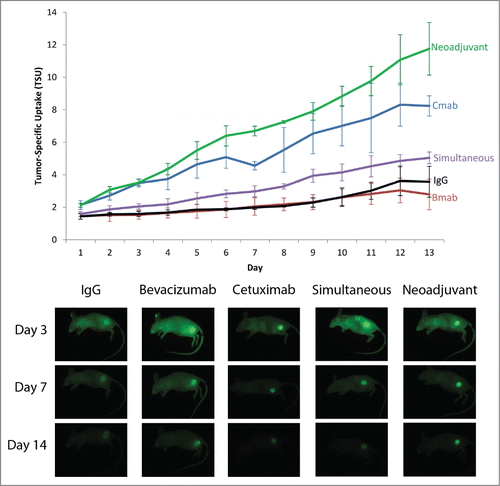
The control group using IgG-IRDye800 demonstrated a TSU of 3.7, this is consistent with conventional enhanced permeability and retention (EPR) effect given its lack of antigen specificity. Similar uptake values were demonstrated for the BVZ800 Only group, but this was a reflection of both high fluorescence values in the tumor and high fluorescence values in background tissue. As a result, the moderate levels of VEGF immunohistochemistry staining performed in the orthotopic model remains consistent with our fluorescence results when considered in the context of the entire animal. In contrast to BVZ800, the CTX800 Only group demonstrated significantly higher delivery as measured by TSU values compared to either the BVZ800 Only group (8.6 vs. 2.8, P < 0.001) or the IgG control group (8.6 vs. 3.7, P < 0.001) at 14 days.
To examine the effect of bevacizumab on cetuximab delivery, cetuximab-IRDye800 and bevacizumab-IRDye800 were administered together (Simultaneous group) which provided the same total amount of dye as the single-agent groups across 2 distinct mechanisms (anti-EGFR and anti-VEGF). Optical imaging over time demonstrated no synergistic effect when administered simultaneously (Simultaneous 5.0 vs. CTX800 Only 8.6, p = 0.001). Consistent with monotherapy findings, the tumor and background TSU values fell approximately halfway between the CTX800 Only and BVZ800 Only groups.
To determine the effects of pretreating with bevacizumab to allow time for vessel normalization, mice were given unlabeled bevacizumab 3 days prior to CTX800 (Neoadjuvant; ). The Neoadjuvant groups demonstrated the highest TSU (11.8, ANOVA post-hoc P < 0.004 vs all other groups). This increase was predominantly mediated by reduced egress of antibody post-administration (−6.8% per day). When compared to CTX800 Only (Signal −9.6% per day, ), the Neoadjuvant group had improved signal retention within the tumor. Background antibody uptake was higher in groups where BVZ800 was administered. However, background percentage decline was similar across all groups. Taken together, neoadjuvant bevacizumab treatment improved antibody fluorescence through enhanced intratumoral uptake of cetuximab-IRDye800. To corroborate these results, a percent injected dose per gram (%ID/g) was calculated for each group (IgG = 1.2% ± 0.4%, BVZ800 = 1.9% ± 0.5%, CTX800 = 3.4% ± 1.0%, Simultaneous = 2.6% ± 0.4%, Neoadjuvant = 6.5% ± 1.2%) and similarly demonstrated a significant difference between CTX800 and Neoadjuvant (p = 0.007).
Figure 3. Tumor, Background and TSU values of OSC-19 and SCC-1 Flank Model.Daily tumor (blue), background (red), and TSU (green line) plotted with mean ± SD. (A) Cetuximab group, OSC-19 cell line (B) Neoadjuvant group, OSC-19 cell line (C) Cetuximab group, SCC-1 cell line (D) Neoadjuvant group, SCC-1 cell line.
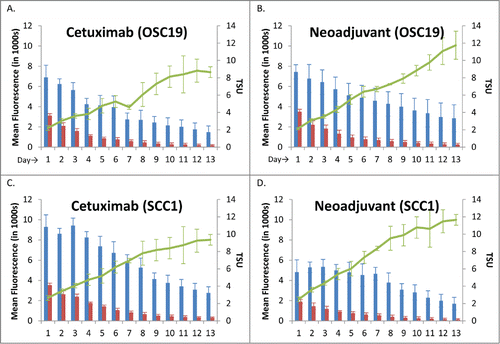
These results were verified using a second oral cavity squamous cell carcinoma line, SCC-1. The CTX800 only group had a TSU of 9.3 at Day 13 compared to the Neoadjuvant group TSU of 11.7 (p = 0.067) ().
To determine the effect of bevacizumab pretreatment on vascular biology, vessel normalization was examined for areas of each tumor following CD31 immunohistochemistry staining (). The Neoadjuvant group demonstrated higher pericyte coverage compared with CTX800 Only, consistent with vessel normalization (p = 0.009).
Figure 4. Vascular normalization by histology>(A) Tumors from the Cetuximab Only and Neoadjuvant groups were stained with CD31 (top, vascular endothelium) and NG2 (bottom, pericyte) to examine pericyte coverage. Magnification: 200×. (B) Areas of colocalized CD31 and NG2 were calculated with ImageJ and reported as a mean ratio of pericyte coverage. Error bars represent SD.
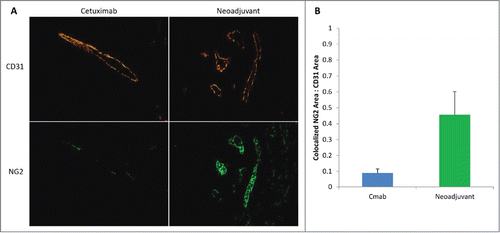
To determine if improved delivery translated therapeutic benefit, tumor response was measured for each group when using neoadjuvant bevacizumab. CTX800 Only and BVZ800 Only had lower percentage tumor volume growth compared to IgG (ANOVA p = 0.004, ). Treatment groups that included bevacizumab and cetuximab had significantly higher response to therapy compared to IgG (Simultaneous p = 0.005, Neoadjuvant p = 0.003) with the best response occurring when bevacuzimab was administered 3 days before cetuximab. Consistent with the tumor volume response, Ki67 staining was significantly reduced in the in the neoadjuvant group compared to the cetuximab group.
Figure 5. Therapeutic Effect of Vessel Normalization: Percent Change in Tumor Volume & Cellular Proliferation. (A) Tumor volume was assessed by caliper measurements and the mean percent volume change was compared between Day 0 and Day 14. Error bars represent SD. (B) Tumors from the Cetuximab Only and Neoadjuvant groups were stained with Ki67 (top, cellular proliferation) and DAPI (bottom, nucleus) to examine treatment effect of cetuximab. Magnification: 100×.
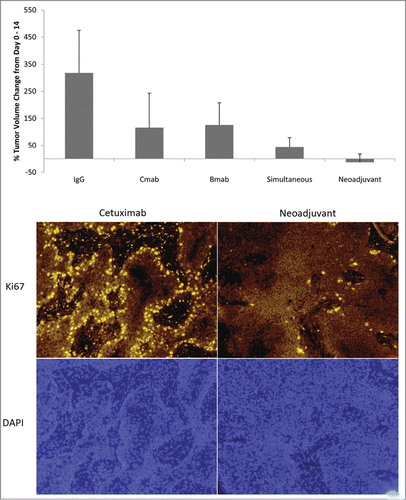
illustrates the specificity of fluorescence to the tumor with the overlying skin removed. Consistent with the above findings, the Neoadjuvant group demonstrates the highest TSU across all groups. Groups that included fluorescently-labeled bevacizumab-IRDye800 (BVZ800 only, Simultaneous) demonstrated increased background levels, consistent with increased off-target distribution.
Figure 6. Specificity of Dye Uptake Using FDA-Approved Open-Field Fluorescence Imaging.Representative images demonstrating tumors with skin flaps elevated to simulate a surgical field where the bright field image (left) demonstrates tumor location and the near-infrared fluorescence with the LUNA system(right) illustrates the specificity of cetuximab-IRDye800 to the tumor. Exposure time (1 second) and aperture are equivalent for all images.
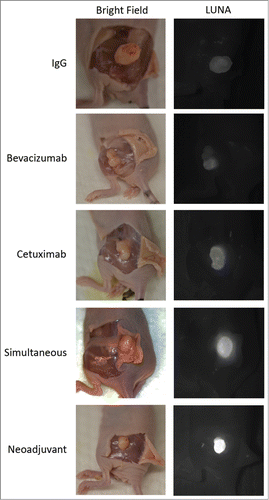
Discussion
Using fluorescently-labeled antibodies to track uptake in the tumor, we demonstrate that pretreatment with bevacizumab increases tumor-specific delivery of cetuximab into oral cavity tumor lines. Pretreatment with bevacizumab 3 days prior to cetuximab administration consistently improved tumor specific uptake and injected dose per gram of the antibody compared to controls. Although the concept of vessel normalization has been established, this is the first study to demonstrate improved antibody delivery and therapeutic response after bevacizumab treatment measured by optical fluorescence.
Measurement of changes in tumor specific uptake demonstrated that the decline of background fluorescence was consistent across groups and improvement in tumor fluorescence in bevacizumab-treated groups is likely attributable to an increased influx of dye into the tumor over time or a slower egress in tumor fluorescence over time. This finding is consistent with the physiology of vessel normalization and suggests that changes in the tumor vasculature are occurring independent of systemic vasculature changes.Citation10 Furthermore, histologic analysis of pericyte coverage demonstrates structural vessel changes in tumors pretreated with bevacizumab. Finally, differences in cellular proliferation and tumor volume demonstrate the therapeutic end-product of these vessel changes.
Anti-EGFR therapies are used to treat head and neck cancer, but the response rate has been disappointing despite the oncogenic importance of EGFR in the progression of this cancers.Citation11-13 The mechanism of action for cetuximab and panitumumab is well-characterized.Citation14 Anti-EGFR antibodies competitively bind to the extracellular portion of EGFR and inhibit downstream signaling cascades that are necessary for proliferation in addition to promoting antibody-dependent cellular cytoxicity in the case of cetuximab, an effect owing to its IgG1 backbone.Citation15 Given the low response rate in patients with confirmed EGFR overexpression and lack of intrinsic EGFR mutationCitation16-18, there are opportunities to improve treatment benefit.
Improved tumor responses seen in the current study are consistent with recent studies demonstrating significantly higher rates of complete response with the addition of neoadjuvant bevacizumab to cytotoxic chemotherapies for metastatic HER-2 negative breast cancer patients.Citation19-21 However, the mechanism for this improved response remains unclear and the literature surrounding vessel normalization in preclinical models is controversial. Multiple studies have demonstrated evidence of vessel normalization and increased therapeutic uptake.Citation22-24 There are also a series of studies that demonstrate the opposite effect of decreased uptake of therapeutic following anti-VEGF administration.Citation25 The notion that there is a therapeutic window based on dose and time likely explains these discrepant findings.Citation10,22,26 In our study, we used a relatively low human-equivalent dose of 5 mg/kg. Studies that demonstrate decreased tumor uptake of small molecule chemotherapeutics following bevacizumab administration and a lack of a survival benefit typically dose above 10 mg/kg.Citation27-29
Others have demonstrated decreased tumor uptake using radiolabelled antibodies with and without a 5 mg/kg dose of bevacizumab dosed once one day prior to and 2 additional bevacizumab treatments following antibody therapy.Citation30 Timing of bevacizumab therapy may limit the vascular remodeling required to improve therapeutic delivery. This finding may also explain why other studies that typically administer bevacizumab after their primary therapy are unable to demonstrate benefit.
It is conceivable that other variables such as the size of the therapeutic and its binding affinity may factor into rates of extravasation and subsequent retention of the molecule within the tumor. Xenograft models of intercellular openings in tumor vessels in a number of cancer types ranged from 380 and 780 nm in diameterCitation31,32, well in excess of both small molecules and antibodies (2 molecules of the Fab component of cetuximab had a diameter of 21.1 nm by x-ray diffraction).Citation33 Empirical studies on tumor vessel pore size and therapeutic uptake suggest that the optimal therapeutic size is somewhere between 12 nm-50 nm for maximal tumor penetrance.Citation34,35 When considered in the context of our finding that cetuximab was retained in the tumor following bevacuzimab administration, it seems plausible that vessel normalization reduces the tumor vessel pore size to a range that still permits extravasation while limiting intravasation, even with larger monoclonal antibodies.
Once in the extravascular space, binding affinity may then govern the distribution of the therapeutic within the substance of the tumor. Fujimori et al. demonstrated with mathematical modeling that high-affinity monoclonal antibodies may in fact limit the distribution of the therapeutic within the tumor, a phenomenon known as the ‘binding site barrier’ hypothesis.Citation36 Successful binding of the therapeutic to its target appears to hinder subsequent, downstream binding ultimately yielding heterogenous distribution within tumors.Citation37 Therefore, these studies conclude that antibodies with moderate affinity may optimize tumor distribution. This may explain the discrepant results seen in studies that show decreased tumor uptake of therapeutics following bevacuzimab.
Finally, one additional factor that may explain these discrepant preclinical findings in vessel normalization may involve the modality for tracking tumor penetration. Our results support the use of near-infrared fluorescence to monitor therapeutic delivery into tumors on the basis of 2 fundamental principles. This study was designed to directly visualize the therapeutic antibody delivery using fluorescence imaging. This model of imaging drug delivery allows monitoring off-target distribution. Optical parameters demonstrating improved antibody delivery was confirmed by demonstrating tumor response. Taken together, this study provides proof-of-principle that near-infrared fluorescent technology can be used to track therapeutic antibody delivery in tumors.
Materials and Methods
Cell line and cell culture
The oral cavity squamous cell carcinoma (SCC) cell line OSC-19 (University of Texas M. D. Anderson Cancer Center, Houston, Texas) and SCC-1 (University of Michigan, Ann Arbor, MI) were maintained in antibody-free Dulbecco's modified Eagle's medium (DMEM) containing 10% fetal bovine serum (FBS). The cells were incubated at 37°C in 5% CO2. Cells were harvested and 100 µL representative sample was manually counted with a hemacytometer and trypan blue stain.
Reagents, conjugation, & binding efficiency
Antibodies included bevacizumab (Avastin; Genentech, San Francisco, California), cetuximab (ImClone Systems, Branchburg, New Jersey), panitumumab (Vectibix; Amgen, Thousand Oaks, California; 147 kDa), trastuzumab (Herceptin; Genentech, San Francisco, California; 148 kDa) and purified IgG Antibody (Innovative. Ir-Hu-Gf, #30010BM; 146kDa). Conjugation of IRDye800 was performed according to manufacturing specifications. Briefly, this included incubation in the dark at room temperature with 1.00 M potassium phosphate buffer (pH 10.0), 1 × PBS, and ultrapure water for 2 hours on a tilt rocker. The solution was then passed through a PBS-rinsed desalting spin column (Pierce Biotechnology, Rockford, IL) and centrifuged to remove unconjugated dye. Conjugation efficiency was verified with a 2 µL sample placed in a microvolume spectrophotometer (ThermoScientific Nanodrop 2000 c, Waltham, MA). Dye-to-protein ratios were calculated for every sample synthesized and were consistent (1.30 to 1.62) across all antibody-dye reagents. Conjugated dyes were stored in the dark at 4°C.
Treatment groups
Five antibody-probe regimen were administered via tail vein injections: 200 µg IgG-IRDye800 (IgG800), 200 µg cetuximab-IRDye800 (CTX800 Only), 200 µg bevacizumab-IRDye800 (BVZ800 Only), and 100 µg cetuximab-IRDye800 + 100 µg bevacuzimuab-IRDye800 (Simultaneous). One group received pretreatment with 100 µg unlabeled bevacizumab at Day -3 then 200 µg cetuximab-IRDye800 at Day 0 (Neoadjuvant). In our mouse model, 200 µg corresponds to a 10 mg/kg dose.
Orthotopic tongue animal model
Athymic nude (nu/nu) mice (n = 19) aged 4 to 6 weeks (Charles River Laboratories, Hartford, CT) were implanted with 2 × 106 cells of OSC-19 into the tongue. Given the risk of respiratory sequelae in this model, these mice were monitored for 2 days, imaged, and tumors were harvested for histologic analysis. Institutional Ethics Committee approval was obtained prior to beginning the study.
Flank animal model
Athymic nude (nu/nu) mice (n = 28) aged 4 to 6 weeks (Charles River Laboratories, Hartford, CT) were subcutaneously implanted with 2 × 106 cells of OSC-19 (oral cavity SCC) or SCC-1 (oral cavity SCC) in the left flank. Once tumors grew to a total volume of 100–190 mm, treatment was administered via intravenous tail vein injection. Tumor volume was assessed on days 0, 4, 8, 12, and 14 with electronic calipers (Marathon Management Company). The largest diameter, followed by the diameter perpendicular to the longest diameter, and depth were recorded for each tumor. Institutional Ethics Committee approval was obtained prior to beginning the study.
Near-infrared fluorescence imaging
The orthotopic tongue model was imaged with the SPY (Novadaq) and Pearl (LICOR) near-infrared imaging systems. Mice were imaged on day 2. For the flank model, we utilized the LUNA system, also manufactured by Novadaq, for additional precision and sensitivity while continuing imaging with the Pearl (LICOR). Mice were imaged daily for 14 days after intravenous tail vein injections of the respective imaging agents were administered.
Data analysis for both SPY and LUNA systems were performed with the manufacturer-associated software package SPY-Q (Novadaq). Fluorescence was measured as the mean fluorescent signal for tumor and normal tissue types respectively. Similarly, Pearl system data was analyzed with the manufacturer-associated software package Image Studio (LICOR). Fluorescence was measured by selecting regions of interest (ROI), obtaining a signal count and dividing the signal by the 2-dimensional area of the ROI, yielding a Signal per Area endpoint. To measure uptake of cetuximab-IRDye800, we defined Tumor-Specific Uptake (TSU) as tumor fluorescence divided by background fluorescence for all imaging systems. A percent injected dose was calculated using the fluorescence within the flank tumor on Day 14 divided by the tumor weight (grams) at sacrifice (Day 14). Eight known concentrations of cetuximab-IRDye800 (0.0125–1.61 µg) were quantified by fluorescence to calculate a linear standard of total fluorescence counts-to-microgram of dye, yielding a microgram of dye value for each tumor. This was divided by the tumor weight (grams) at sacrifice (Day 14) and divided by the injected dose of IRDye800 (200 µg) to yield a percent injected dose per gram (%ID/g) of tumor tissue.
Histology
Ex-vivo tumor imaging and histology were performed at Day 14. Vasculature modulation (rabbit anti-CD31 with AlexaFluor-546 and mouse anti-NG2 with AlexaFluor-488) was evaluated by immunohistochemistry to confirm vessel normalization. The endpoint for NG2 and CD31 quantification was the area of NG2 circumferential area divided by CD31 circumferential area as measured by ImageJ (National Institutes of Health, Bethesda, MD). Given the presence of NG2 staining within the presumptive lumen of vessels, only areas where NG2 and CD31 colocalization were quantified using ImageJ. Cellular proliferation (rabbit anti-Ki67 with AlexaFluor-546) was analyzed by immunohistochemistry to visualize treatment effect.
Statistical analysis
Statistical analysis was performed using SPSS Statistics for Windows, Version 22.0 (IBM Corporation Inc., Armonk, NY). Mean and standard deviation (SD) were calculated and plotted for continuous variables including TSU, tumor volume, and quantification of immunohistochemistry. Comparisons of multiple means were performed using Tukey's HSD post-hoc test.
Conclusion
The time-dependent administration of bevacizumab structurally and functionally normalize vessels in head and neck squamous cell carcinoma xenograft models. Daily near-infrared fluorescence imaging demonstrated improved retention of cetuximab within the tumor and demonstrated minimal off-target effect of bevacizumab on the systemic distribution of cetuximab. Pre-treatment with bevacuzimab may offer a means of improving the oncologic outcome of antibody-based therapeutics for cancer.
Disclosure of Potential Conflicts of Interest
No potential conflicts of interest were disclosed.
Acknowledgments
The authors thank Marilyn Shackelford for her assistance with histology and slide preparation. Equipment was donated by Novadaq and LICOR Biosciences. IRDye800 was donated by LICOR Biosciences.
Presented at the 5th World Congress of the International Federation of Head and Neck Oncologic Societies and American Head and Neck Society Annual Meeting, New York, NY, July 28, 2014.
Funding
This study was supported with an NIH T32CA091078 and R21CA179171 grant.
References
- Lee CM, Tannock IF. The distribution of the therapeutic monoclonal antibodies cetuximab and trastuzumab within solid tumors. BMC Cancer 2010; 10:255; PMID:20525277; http://dx.doi.org/10.1186/1471-2407-10-255
- Carmeliet P, Jain RK. Principles and mechanisms of vessel normalization for cancer and other angiogenic diseases. Nat Rev Drug Discov 2011; 10:417-27; PMID:21629292; http://dx.doi.org/10.1038/nrd3455
- Minchinton AI, Tannock IF. Drug penetration in solid tumours. Nat Rev Cancer 2006; 6:583-92; PMID:16862189; http://dx.doi.org/10.1038/nrc1893
- Weinstein JN, Eger RR, Covell DG, Black CD, Mulshine J, Carrasquillo JA, Larson SM, Keenan AM. The pharmacology of monoclonal antibodies. Ann N Y Acad Sci 1987; 507:199-210; PMID:3327413; http://dx.doi.org/10.1111/j.1749-6632.1987.tb45802.x
- McDonald DM, Baluk P. Significance of blood vessel leakiness in cancer. Cancer Res 2002; 62:5381-5; PMID:12235011
- Greenberg JI, Shields DJ, Barillas SG, Acevedo LM, Murphy E, Huang J, Scheppke L, Stockmann C, Johnson RS, Angle N, et al. A role for VEGF as a negative regulator of pericyte function and vessel maturation. Nature 2008; 456:809-13; PMID:18997771; http://dx.doi.org/10.1038/nature07424
- Goel S, Wong AH, Jain RK. Vascular normalization as a therapeutic strategy for malignant and nonmalignant disease. Cold Spring Harbor Perspect Med 2012; 2:a006486; PMID:22393532; http://dx.doi.org/10.1101/cshperspect.a006486
- Wildiers H, Guetens G, De Boeck G, Verbeken E, Landuyt B, Landuyt W, de Bruijn EA, van Oosterom AT. Effect of antivascular endothelial growth factor treatment on the intratumoral uptake of CPT-11. Br J Cancer 2003; 88:1979-86; PMID:12799646; http://dx.doi.org/10.1038/sj.bjc.6601005
- Dickson PV, Hamner JB, Sims TL, Fraga CH, Ng CY, Rajasekeran S, Hagedorn NL, McCarville MB, Stewart CF, Davidoff AM. Bevacizumab-induced transient remodeling of the vasculature in neuroblastoma xenografts results in improved delivery and efficacy of systemically administered chemotherapy. Clin Cancer Res 2007; 13:3942-50; PMID:17606728; http://dx.doi.org/10.1158/1078-0432.CCR-07-0278
- Pastuskovas CV, Mundo EE, Williams SP, Nayak TK, Ho J, Ulufatu S, Clark S, Ross S, Cheng E, Parsons-Reponte K, et al. Effects of anti-VEGF on pharmacokinetics, biodistribution, and tumor penetration of trastuzumab in a preclinical breast cancer model. Mol Cancer Ther 2012; 11:752-62; PMID:22222630; http://dx.doi.org/10.1158/1535-7163.MCT-11-0742-T
- Vermorken JB, Trigo J, Hitt R, Koralewski P, Diaz-Rubio E, Rolland F, Knecht R, Amellal N, Schueler A, Baselga J. Open-label, uncontrolled, multicenter phase II study to evaluate the efficacy and toxicity of cetuximab as a single agent in patients with recurrent and/or metastatic squamous cell carcinoma of the head and neck who failed to respond to platinum-based therapy. J Clin Oncol 2007; 25:2171-7; PMID:17538161; http://dx.doi.org/10.1200/JCO.2006.06.7447
- Vermorken JB, Mesia R, Rivera F, Remenar E, Kawecki A, Rottey S, Erfan J, Zabolotnyy D, Kienzer HR, Cupissol D, et al. Platinum-based chemotherapy plus cetuximab in head and neck cancer. N Engl J Med 2008; 359:1116-27; PMID:18784101; http://dx.doi.org/10.1056/NEJMoa0802656
- Bourhis J, Rivera F, Mesia R, Awada A, Geoffrois L, Borel C, Humblet Y, Lopez-Pousa A, Hitt R, Vega Villegas ME, et al. Phase I/II study of cetuximab in combination with cisplatin or carboplatin and fluorouracil in patients with recurrent or metastatic squamous cell carcinoma of the head and neck. J Clin Oncol 2006; 24:2866-72; PMID:16717293; http://dx.doi.org/10.1200/JCO.2005.04.3547
- Baselga J. The EGFR as a target for anticancer therapy—focus on cetuximab. Eur J Cancer 2001; 37, Supplement 4:16-22; http://dx.doi.org/10.1016/S0959-8049(01)00233-7
- Patel D, Guo X, Ng S, Melchior M, Balderes P, Burtrum D, Persaud K, Luna X, Ludwig DL, Kang X. IgG isotype, glycosylation, and EGFR expression determine the induction of antibody-dependent cellular cytotoxicity in vitro by cetuximab. Hum Antibodies 2010; 19:89-99; PMID:21178280
- Pirker R, Pereira JR, Szczesna A, von Pawel J, Krzakowski M, Ramlau R, Vynnychenko I, Park K, Yu CT, Ganul V, et al. Cetuximab plus chemotherapy in patients with advanced non-small-cell lung cancer (FLEX): an open-label randomised phase III trial. Lancet 2009; 373:1525-31; PMID:19410716; http://dx.doi.org/10.1016/S0140-6736(09)60569-9
- Khambata-Ford S, Harbison CT, Hart LL, Awad M, Xu LA, Horak CE, Dakhil S, Hermann RC, Lynch TJ, Weber MR. Analysis of potential predictive markers of cetuximab benefit in BMS099, a phase III study of cetuximab and first-line taxane/carboplatin in advanced non–small-cell lung cancer. J Clin Oncol 2010; 28:918-27; PMID:20100958; http://dx.doi.org/10.1200/JCO.2009.25.2890
- Elie C, Geay JF, Morcos M, Le Tourneau A, Girre V, Broët P, Marmey B, Chauvenet L, Audouin J, Pujade-Lauraine E, et al. Lack of relationship between EGFR-1 immunohistochemical expression and prognosis in a multicentre clinical trial of 93 patients with advanced primary ovarian epithelial cancer (GINECO group). Br J Cancer 2004; 91:470-5; PMID:15226774; http://dx.doi.org/10.1038/sj.bjc.6601961
- von Minckwitz G, Eidtmann H, Rezai M, Fasching PA, Tesch H, Eggemann H, Schrader I, Kittel K, Hanusch C, Kreienberg R, et al. Neoadjuvant chemotherapy and bevacizumab for HER2-negative breast cancer. N Engl J Med 2012; 366:299-309; PMID:22276820; http://dx.doi.org/10.1056/NEJMoa1111065
- Bear HD, Tang G, Rastogi P, Geyer CE Jr, Robidoux A, Atkins JN, Baez-Diaz L, Brufsky AM, Mehta RS, Fehrenbacher L, et al. Bevacizumab added to neoadjuvant chemotherapy for breast cancer. N Engl J Med 2012; 366:310-20; PMID:22276821; http://dx.doi.org/10.1056/NEJMoa1111097
- Gerber B, Loibl S, Eidtmann H, Rezai M, Fasching PA, Tesch H, Eggemann H, Schrader I, Kittel K, Hanusch C, et al. Neoadjuvant bevacizumab and anthracycline–taxane-based chemotherapy in 678 triple-negative primary breast cancers; results from the geparquinto study (GBG 44). Ann Oncol 2013; 24:2978-84; PMID:24136883; http://dx.doi.org/10.1093/annonc/mdt361
- Huang Y, Yuan J, Righi E, Kamoun WS, Ancukiewicz M, Nezivar J, Santosuosso M, Martin JD, Martin MR, Vianello F, et al. Vascular normalizing doses of antiangiogenic treatment reprogram the immunosuppressive tumor microenvironment and enhance immunotherapy. Proc Natl Acad Sci 2012; 109:17561-6; PMID:23045683; http://dx.doi.org/10.1073/pnas.1215397109
- Argiris A, Kotsakis AP, Hoang T, Worden FP, Savvides P, Gibson MK, Gyanchandani R, Blumenschein GR Jr, Chen HX, Grandis JR, et al. Cetuximab and bevacizumab: preclinical data and phase II trial in recurrent or metastatic squamous cell carcinoma of the head and neck. Ann Oncol 2013; 24:220-5; PMID:22898037; http://dx.doi.org/10.1093/annonc/mds245
- Zhang Q, Bindokas V, Shen J, Fan H, Hoffman RM, Xing HR. Time-course imaging of therapeutic functional tumor vascular normalization by antiangiogenic agents. Mol Cancer Ther 2011; 10:1173-84; PMID:21586628; http://dx.doi.org/10.1158/1535-7163.MCT-11-0008
- Van der Veldt Astrid AM, Lubberink M, Bahce I, Walraven M, de Boer MP, Greuter HN, Hendrikse NH, Eriksson J, Windhorst AD, Postmus PE, et al. Rapid decrease in delivery of chemotherapy to tumors after anti-VEGF therapy: implications for scheduling of anti-angiogenic drugs. Cancer Cell 2012; 21:82-91; PMID:22264790; http://dx.doi.org/10.1016/j.ccr.2011.11.023
- Stylianopoulos T, Jain RK. Combining two strategies to improve perfusion and drug delivery in solid tumors. Proc Natl Acad Sci U S A 2013; 110:18632-7; PMID:24167277; http://dx.doi.org/10.1073/pnas.1318415110
- Brufsky AM, Hurvitz S, Perez E, Swamy R, Valero V, O'Neill V, Rugo HS. RIBBON-2: a randomized, double-blind, placebo-controlled, phase III trial evaluating the efficacy and safety of bevacizumab in combination with chemotherapy for second-line treatment of human epidermal growth factor receptor 2–negative metastatic breast cancer. J Clin Oncol 2011; 29:4286-93; PMID:21990397; http://dx.doi.org/10.1200/JCO.2010.34.1255
- Robert NJ, Diéras V, Glaspy J, Brufsky AM, Bondarenko I, Lipatov ON, Perez EA, Yardley DA, Chan SY, Zhou X, et al. RIBBON-1: randomized, double-blind, placebo-controlled, phase III trial of chemotherapy with or without bevacizumab for first-line treatment of human epidermal growth factor receptor 2–negative, locally recurrent or metastatic breast cancer. J Clin Oncol 2011; 29:1252-60; PMID:21383283; http://dx.doi.org/10.1200/JCO.2010.28.0982
- Miles DW, Chan A, Dirix LY, Cortés J, Pivot X, Tomczak P, Delozier T, Sohn JH, Provencher L, Puglisi F, et al. Phase III study of bevacizumab plus docetaxel compared with placebo plus docetaxel for the first-line treatment of human epidermal growth factor receptor 2–negative metastatic breast cancer. J Clin Oncol 2010; 28:3239-47; PMID:20498403; http://dx.doi.org/10.1200/JCO.2008.21.6457
- Arjaans M, Oude Munnink TH, Oosting SF, Terwisscha van Scheltinga AG, Gietema JA, Garbacik ET, Timmer-Bosscha H, Lub-de Hooge MN, Schröder CP, de Vries EG. Bevacizumab-induced normalization of blood vessels in tumors hampers antibody uptake. Cancer Res 2013; 73:3347-55; PMID:23580572; http://dx.doi.org/10.1158/0008-5472.CAN-12-3518
- Yuan F, Dellian M, Fukumura D, Leunig M, Berk DA, Torchilin VP, Jain RK. Vascular permeability in a human tumor xenograft: molecular size dependence and cutoff size. Cancer Res 1995; 55:3752-6; PMID:7641188
- Hobbs SK, Monsky WL, Yuan F, Roberts WG, Griffith L, Torchilin VP, Jain RK. Regulation of transport pathways in tumor vessels: role of tumor type and microenvironment. Proc Natl Acad Sci U S A 1998; 95:4607-12; PMID:9539785; http://dx.doi.org/10.1073/pnas.95.8.4607
- Li S, Schmitz KR, Jeffrey PD, Wiltzius JJW, Kussie P, Ferguson KM. Structural basis for inhibition of the epidermal growth factor receptor by cetuximab. Cancer Cell 2005; 7:301-11; PMID:15837620; http://dx.doi.org/10.1016/j.ccr.2005.03.003
- Schmidt MM, Wittrup KD. A modeling analysis of the effects of molecular size and binding affinity on tumor targeting. Mol Cancer Ther 2009; 8:2861-71; PMID:19825804; http://dx.doi.org/10.1158/1535-7163.MCT-09-0195
- Chauhan VP, Stylianopoulos T, Martin JD, Popović Z, Chen O, Kamoun WS, Bawendi MG, Fukumura D, Jain RK. Normalization of tumour blood vessels improves the delivery of nanomedicines in a size-dependent manner. Nature Nanotechnol 2012; 7:383-8; PMID:22484912; http://dx.doi.org/10.1038/nnano.2012.45
- Fujimori K, Covell DG, Fletcher JE, Weinstein JN. A modeling analysis of monoclonal antibody percolation through tumors: a binding-site barrier. J Nuclear Med 1990; 31:1191-8; PMID:2362198
- Berk DA, Yuan F, Leunig M, Jain RK. Direct in vivo measurement of targeted binding in a human tumor xenograft. Proc Natl Acad Sci U S A 1997; 94:1785-90; PMID:9050856; http://dx.doi.org/10.1073/pnas.94.5.1785