ABSTRACT
Dehydroepiandrosterone (DHEA), an adrenal hormone, has a protective role against cancer. We previously shown that DHEA inhibits the proliferation and migration of cell lines derived from breast cancer; however, the role of DHEA in others events related with these effects are unknown. We hypothesized that DHEA inhibits the expression of proteins and some events related with cell migration and metastasis. We determined the migration in Boyden chambers, the invasion in matrigel, anchorage-independent growth and the formation of spheroids in 3 cell lines (MCF-7, MDA-MB-231, ZR-75-30) derived from breast cancer exposed to DHEA. The secretion of metalloproteinases (MMPs), tissue inhibitors of metalloproteinases (TIMPs), and several pro-inflammatory molecules in the secretome of these cells was also evaluated. DHEA inhibited the migration in transwells and the invasion in matrigel of MCF-7 and MDA-MB-231 cells. Besides, DHEA inhibited the anchorage-independent growth on agar and decreased the size of spheroids, and also reduced the secretion of IL-1α, IL-6, IL-8, and TNF-α in all cell lines. Metalloproteinase-1 (MMP-1) secretion was slightly decreased by DHEA treatment in MDA-MB-231 cells. Our results also showed that inhibition of migration and invasion induced by DHEA in breast cancer cells is correlated with the decrease of cytokine/chemokine secretion and the diminution of tumor cells growth. MCF-7 cells were the most responsive to the exposure to DHEA, whereas ZR-75-30 cells responded less to this hormone, suggesting that DHEA could be used in the treatment of breast cancer in early stages.
Abbreviations
MCF-7 and MDA-MB-231 | = | human breast adenocarcinoma cell line |
ZR-75-30 | = | human breast ductal carcinoma cell line |
DHEA | = | trans-dehydroandrosterone |
MMPs | = | metalloproteinases |
TIMPs | = | tissue inhibitors of metalloproteinases |
Introduction
Dehydroepiandrosterone (DHEA), a steroid produced mainly in adrenal cortex, declines profoundly with age in human beings of both sexes, and this has been associated with an increase of the incidence of most cancers.Citation1 It has been suggested that a relative deficiency of DHEA and its sulfated ester (DHEA-S) can be related to the development of chronic diseases generally associated with aging, including insulin resistance, obesity, cardiovascular disease, cancer, reductions of the immune defense, depression and a general deterioration in the sensation of well-being.Citation2 It has been shown that DHEA has many protective effects against these diseases; however, mechanisms involved with these effects remain unknown. In previous studies, we and others have reported that DHEA has anti-inflammatory and anti-oxidant properties on endothelial cells.Citation3,4 Due to this, its role in inflammatory diseases such as atherosclerosis and cancer, it has been discussed. In cancer, DHEA inhibits some processes which contribute to tumor development.Citation5 Despite several protective effects against cancer have been described in models in vivo and in vitro, some studies have shown that DHEA can induce cancer. All these different effects depend on the exposure time, concentration, and cell type or model in vivo used. For example, we previously showed that DHEA at supra-physiological concentrations, inhibits the proliferation of breast cancer cells (MCF-7); in contrast, physiological concentrations increased it.Citation6 Our work group showed that supra-physiological concentrations of DHEA inhibited the proliferation and migration of breast and cervical cancer cell lines.Citation7,8
Breast cancer is the most common type of cancer, and constitutes a serious health problem of women in worldwide. In Mexico, breast cancer accounts for more deaths than cervical cancer since 2006. It is the second cause of death among women aged 30 to 54, and affects all socioeconomic groups,Citation9 and its metastatic potential is the main cause of death. Breast cancer cells spread from the primary tumor in the breast through the lymphatic or blood system to other parts of the body such as bones, liver, lung or brain.Citation10 Process of metastasis comprises of a series of sequential steps. Metastasis starts with the local invasion of surrounding host tissue by cells originating from the primary tumor and continues until the tumor cells invade and intravasate into blood or lymphatic vessels.Citation10,11 Invasive tumor cells must first alter their cell-to-cell adhesion and cell adhesion to the extracellular matrix (ECM). The adherence of tumor cells to the ECM is mediated through integrins and proteins that bind to these such as fibronectin, laminin, collagen, fibrinogen and vitronectin.Citation12 Invasion is preceded by degradation of the ECM to enable the penetration of tissue boundaries. The degradation of ECM is carried out mainly through metalloproteinases (MMPs) and the urokinase plasminogen activator (uPA) system.Citation13,14 On the other hand, the tissue inhibitors of metalloproteinases (TIMPs), which were characterized based on their ability to inhibit MMP activity, have been shown to carry out a number of MMP-independent functions.Citation15 For example, TIMPs can bind directly to cell surface receptors to stimulate cell-signaling pathways, leading changes in cell growth, proliferation, and apoptosis; also, they control cell-ECM interactions, specifically under conditions that promote tissue turnover.Citation16
It has been suggested that pro-inflammatory molecules are involved in the growth and progression of many malignancies.Citation17 Inflammation increases the risk of cancer because cells infiltrating the tumor microenvironment can produce several molecules, such as cytokines, growth factors, chemokines (which maintain a sustained proliferation avoiding apoptosis), proangiogenic factors, and MMPs that promote different stages of metastatic process.
In this work, the capacity of DHEA to inhibit several processes involved with growth, migration and invasion of breast cancer cells was evaluated.
Results
DHEA decreased the migration
The migration of tumor cells was tested using Boyden chamber assay (transwells). DHEA decreased the migration of MCF-7 cells from 18 h having the maximal effect at 48 h with an inhibition of 60%. DHEA also diminished the migration of MDA-MB-231 cells but the effect was smaller and slower than MCF-7 cells with an inhibition of 45% at 48 h. In contrast, DHEA did not have effect on the migration of ZR-75-30 cells ().
Figure 1. DHEA decreased the cellular migration. Cells were cultured without (Control) and with 100 μM of DHEA and the migration was evaluated by transwell assay at 18, 24 and 48 h. Data are shown as percentage of migration in comparison with control cells (100%), and were expressed as mean ± SEM. This is a representative experiment of 3 performed in an independent way. *P < 0.01 compared with the control. On the right side, photographs of migrating cells on the underside of the membranes stained with crystal violet are showed at 10x magnification.
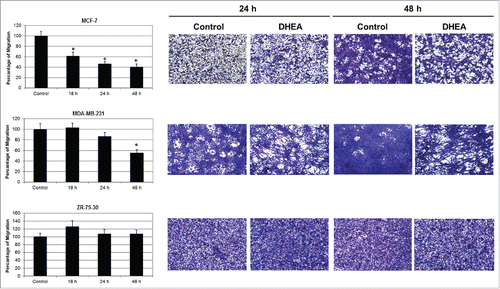
DHEA decreased the invasion
The invasion was evaluated mediating matrigel invasion chambers. DHEA decreased the invasion of MCF-7 and MDA-MB-231 cells, but did not affect the invasion of ZR-75-30 cells (). It was very interesting to observe that ZR-75-30 cells were the most invasive and MCF-7 cells the less invasive of the 3 cell lines.
Figure 2. DHEA inhibited the cellular invasion. Cells were cultured without (Control) and with 100 μM of DHEA and the invasion was evaluated by 8 μm pore-size transwells membranes coated with matrigel at 24 and 48 h. Data are shown as percentage of invasion in comparison with control cells (100%), and were expressed as mean ± SEM. This is a representative experiment of 3 performed in an independent way. *P < 0.01 compared with the control. On the right side, photographs of invading cells on the underside of the membranes stained with crystal violet are showed at 10x magnification.
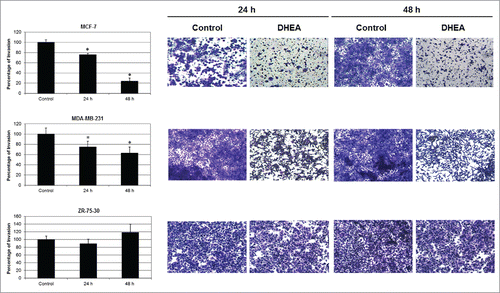
DHEA did not affect the secretion of MMPs and TIMPs
Since MMPs and their protein inhibitors have the capacity to completely remodel the ECM affecting biologic processes involved in cancer such as angiogenesis, cell migration, proliferation, invasion, and apoptosis, among others,Citation18 the secretion of MMPs and TIMPs was evaluated. DHEA did not modify the secretion of any of MMPs and TIMPs in all the cell lines evaluated (). Only a weak decrease was observed in MMP-1 in MDA-MB-231 cells exposed to DHEA (, see boxes). It was very interesting to observe that ZR-75-30 cells had a high expression of MMP-1, MMP-3, MMP-9, MMP-10 and MMP-13, and the all evaluated TIMPs in comparison with MCF-7 and MDA-MB-231 cells ().
Figure 3. DHEA did not alter the secretion of MMPs and TIMPs. Cells were cultured without (Control) and with 100 μM of DHEA, and the secretome was collected and processed after 48 h according to materials and methods. The secretion of MMP-1, MMP-2, MMP-3, MMP-8, MMP-9, MMP-10, MMP-13, TIMP-1, TIMP-2 and TIMP-4 was determined mediating the human matrix metalloproteinase antibody array C1, in MCF-7 (A), MDA-MB-231 (B), and ZR-73-30 cells (C). The dot blots and the densitometric analysis expressed as arbitrary units are showed. This is a representative experiment of 3 performed in an independent way. P < 0.01 compared with the control.
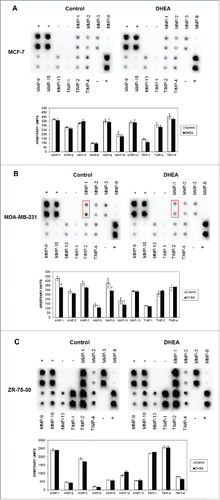
DHEA decreased the secretion of inflammatory molecules
In order to determine which other molecules could be involved with the decrease of migration induced by DHEA, the secretion of specific cytokines involved in inflammatory or immune responses was detected. DHEA decreased the secretion of IL-12 p70, sIL-2 ra, IL-1α, IL-6 and IL-8 in all cell lines. IL-1 ra and IL-1β were diminished in MDA-MB-231 and ZR-75-30 cells exposed to DHEA, while IL-2 decreased in MCF-7 and MDA-MB-231 cells. Interestingly, DHEA greatly reduced the secretion of VEGF in MCF-7 cells ().
DHEA decreased the anchorage-independent growth
Since tumor cells are capable of proliferating independently of both external and internal signals that normally restrain growth, and that anchorage-independent growth is one of the hallmarks of cell transformation,Citation19 we evaluated if DHEA alters the soft agar colony formation. Results showed that MDA-MB-231 had a high capacity to proliferate in soft agar. Contrary to our expectations, ZR-75–30 cells did not proliferate. DHEA decreased strongly, in around 50%, the growth of MCF-7 cells at all the tested concentrations, while in MDA-MB-231 cells had an effect dependent of concentration, reaching 50% of inhibition with 100 μM of DHEA ().
Figure 4. DHEA diminished the anchorage-independent growth of MCF-7 and MDA-MB-231 cells. Cells were cultured without (0) and with 1, 10 and 100 μM of DHEA and the formation of colonies in agar was evaluated after 7 d. The results are expressed as mean ± SEM of 3 independent experiments. * Indicates a P < 0.01 compared with control cells.
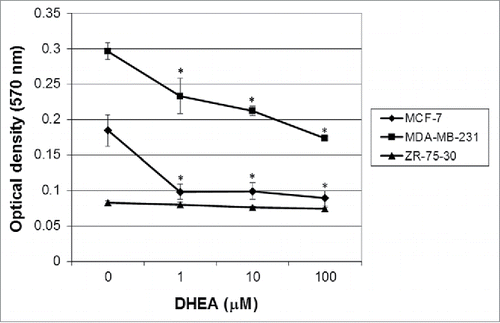
DHEA decreased the size of spheroids
Solid tumors grow in a 3-dimensional (3D) spatial conformation, resulting in a heterogeneous exposure to oxygen and nutrients. Spheroids are a model to study groups of tumor cells in vitro simulating solid tumors;Citation20,21 therefore, the effect of DHEA on spheroid formation was evaluated. The diameter of spheroid of the 3 cell lines used decreased. In MCF-7 cells, DHEA inhibited the size of spheroids from 3 d of culture and the effect was stronger at 15 d (). In MDA-MB-231 and ZR-75-30 cells this inhibition was observed only at 15 d of exposure and the effect was smaller compared with MCF-7 cells (). It was interesting to observe that MCF-7 cells formed the largest spheroids.
Figure 5. DHEA decreased the growth of multicellular spheroids. Cells were cultured as spheroids using liquid overlay assay without (control) or with 100 μM DHEA for 3, 6, and 15 d. After these times, the diameter of spheroids was measured and photographs (15 days) were taken at a 10X magnification in MCF-7 (A), MDA-MB-231 (B), and ZR 75.30 (C) cells. The results show the diameter (μm), and are expressed as mean ± SEM of 3 independent experiments. * Indicates a P < 0.01 compared with control cells.
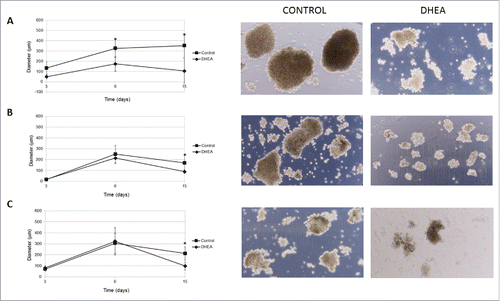
Discussion
The goal of this work was to study the inhibitory effect of DHEA on the growth, migration, and invasion of breast cancer cells. Migration, invasion, anchorage-independent growth, growth of spheroids, and secretion of inflammatory molecules and several proteins related with these processes were evaluated in 3 cell lines derived from human breast cancer with different characteristics. We selected MCF-7 cells, considering that they have a low metastatic potential, low migration and invasive capacities.Citation22 On the other hand, MDA-MB-231 and ZR-75-30 were selected because they have a high capacity to form tumors in in vivo animal models.Citation22,23
Our results showed that DHEA inhibited the migration of MCF-7 and MDA-MB-231 cells, evaluated mediating transwells (). MCF-7 cells were the most responsive and their migration was inhibited since 18 h after treatment. On the other hand, ZR-75-30 cells migration was not modified by DHEA. These results correlated with the lesser invasive potential described to MCF-7 cells,Citation24 suggesting that DHEA had the capacity to inhibit the migration of less invasive but not of more invasive cells.
During migration, it is important the cellular shape, the stability of cell adhesion and the matrix rigidity. Cellular shape plays a pivotal role in cell migration behavior by regulating the traction force at the cell front and rear.Citation25 It has been described that single tumor cells migrate mainly in 2 ways, by protease-dependent mesenchymal movement or by protease-independent amoeboid movement. The movement of cells which have an elongated fibroblast-like shape, is facilitated by channels which are produced in the extracellular matrix (ECM) by matrix degrading enzymes, such as MMPs.Citation26 In contrast, cells with amoeboid movement are round cells and they push and squeeze through pores in the matrix by relying mostly on shape deformations and structural changes in the ECM.Citation27 We observed the morphology of the breast cancer cells used and it is notable that MCF-7 cells have a rounded shape, while MDA-MB-231 and ZR-75-30 cells present a mesenchymal-like elongated shape. We suggest that this elongated shape can favors the motility and migration across the transwells membranes, because we observed that untreated MDA-MB-231 and ZR-75-30 cells migrated faster across membranes and matrigel. Considering that ZR-75-30 cells had a strong migratory potential and a high secretion of MMPs (), DHEA was not able to block their migration at the evaluated times. It will very interesting to determine if DHEA modifies the expression of adhesion molecules involved with the migratory process, because focal adhesions have an important role in cell motility.
Similar to the migration results, matrigel invasion assay showed that DHEA also affected invasion of MCF-7 and MDA-MB-231 cells (). Matrigel is an extract derived from Engelbreth-Holm-Swarm tumor that is equivalent to the basement membrane which contains structural proteins as laminin-111 and collagen-IV, and other proteins that can regulate the cellular behavior.Citation28,29 Some proteolytic enzymes involved with the invasive capacity of cells are MMPs, which degrade different extracellular matrix (ECM) facilitating the invasion.Citation13 We observed that ZR-75-30 cells secrete a high quantity of MMPs in comparison with the other cells (). DHEA did not modified MMPs secretion in none cell line; however, the high secretion of these proteins in ZR-75-30 was correlated with the inability of DHEA to inhibit their invasion. Besides, DHEA did not affect the secretion of TIMPs. These molecules have other functions not related with the inhibition of MMPs, even it has been described that TIMP-1 can regulate positively and negatively processes associated with metastasis depending of its glycosylation state.Citation30 High amount of TIMPs present in the secretome derived from ZR-75-30 cells suggests that these molecules do not realize an inhibitory function of the invasion. These results indicate that the inhibitory effect of DHEA on invasion of breast cancer not include the modification of invasion molecules secretion such as MMPs and TIMPs.
The inhibitory effect on migration and invasion induced by DHEA was not associated with the status of sexual steroid receptors, since analyzing the expression of receptors to estrogen (ER), progesterone (PR) and androgen (AR), MCF-7 and ZR-75-30 cells are positive for the 3 receptors (); however, the effect induced by DHEA on migration and invasion of both cell lines was contrary. On the other hand, MDA-MB-231 cells are triple-negative breast cancer [TNBC: ER−, PR−, human epidermal growth factor receptor (HER2−)], but their migration and invasion were decreased by DHEA. These results indicate that the inhibition of migration and invasion induced by DHEA is independent of the expression of sexual steroid receptors. It has been suggested that DHEA inhibits breast cancer cells proliferation mediating its conversion to estrogen;Citation31 nevertheless, we previously showed that the inhibition of proliferation induced by DHEA on this kind of cells was androgen- and estrogen-receptor independent.Citation6
Table 2. Characteristics of breast cancer cell lines. Positivity (+) or negativity (−) in the expression of estrogen receptor α (ERα), progesterone receptor (PR), androgen receptor (AR) and human epidermal growth factor receptor 2 (HER2) are showed. Status of P53 gene, subtype and tamoxifen sensitivity are also showed. ND = non-determined.
It is interesting to highlight that HER2 status is different in the cell lines used. MCF-7 and MDA-MB-231 cells are negative; in contrast, ZR-75-30 cells are positive. HER2 gene occurs in 10–35% of human breast cancers, and it is strongly related with a more aggressive phenotype and a poorer prognosis.Citation32 Thus, HER2 status could be related with the resistance of ZR-75-30 cells to inhibitory effects of DHEA, in comparison with MCF-7 and MDA-MB-231 cells which were more responsive.
We and others have described that DHEA has an anti-inflammatory effect on different cell types.Citation3,33 Cancer is characterized by a chronic inflammation; therefore, in order to evaluate if DHEA could affect some molecules involved with inflammatory process, such as cytokines, chemoattractant factors, and growth factors, a milliplex assay was performed. Results showed that DHEA caused a decrease in secretion of cytokines as IL-12 p70, IL-1α, IL-6 and IL-8, and the cytokine modulator sIL-2ra (),Citation34-36 which participate in the initiation of cancer and are strongly related with tumor angiogenesis and metastasis, and are associated with inflammation markers.Citation37 Besides, it has been shown that IL-6 induces migration of MCF-7 and MDA-MB-231 cells;Citation38 therefore, the decrease of IL-6 induced by DHEA in our study suggest that can be related with the inhibition of migration. In ZR-75-30 cells, the changes in the secretion of inflammatory molecules, seems not have relation with their migration and invasion properties. On the other hand, in solid tumors, VEGF expression denotes poor prognosis and a tendency for metastasis.Citation39 We observed that MCF-7 and MDA-MB-231 cells have a high secretion of VEGF, and that DHEA almost completely inhibited it in MCF-7 cells, suggesting that this decrease could be related with the strong inhibitory effect observed in these cells.
Table 1. Proteins detected in the secretome of breast tumor cell lines. Cells were cultured without (Control) and with 100 μM of DHEA, and the secretome was collected and processed after 48 h according to materials and methods. Expression of proteins (pg/ml) was determined by MILLIPLEX MAP Human Cytokine/Chemokine Magnetic Bead Panel-Immunology Multiplex Assay. This is a representative experiment of 3 performed in an independent way. Arrows mean a statistical significance of P < 0.01 compared with the control.
In spite of DHEA decreased the secretion of several cytokines in breast cancer cells in vitro, the consequences of these changes could be more relevant in an in vivo model, where the tumor microenviroment modulation implies relationships between various cell types including fibroblasts, immune cells and adipocytes; therefore, DHEA effects on secretion of molecules in vivo it would have to determine.
Anchorage-independent growth is one of the hallmarks of transformation, which is considered the most accurate and stringent in vitro assay for detecting malignant transformation of cells.Citation40 We used a soft agar colony formation assay as a method to monitor anchorage-independent growth, which measures proliferation in a semisolid culture media. ZR-75-30 cells did not proliferate in semisolid medium. We have observed in our experiments that these cells require of a high cellular density to proliferate indicating that need of autocrine signals to growing. In soft agar colony formation assay, a relatively low number cell was seeded; therefore, it is possible that for this reason ZR-75-30 cells did not proliferate in soft agar medium. In contrast, MCF-7 and MDA-MB-231 cells had a high proliferation rate in this medium, and DHEA was able to inhibit it (). We previously showed that DHEA at 100 μM inhibits the proliferation of breast and cervical cancer cells evaluated by crystal violet,Citation7,8 indicating that DHEA had a strong effect on proliferation, independent of anchorage.
In order to evaluate another anchorage-independent growth assay we measured the formation of spheroids. Spheroid culture systems can mimic some of in vivo microenvironmental characteristics of solid tumors such as 3-dimensional organization and function, which are fundamental to tumor progression.Citation41 Spheroids represent a clonal cell population in which gradients of oxygen, glucose, lactate, metabolites and pH create a continuum of different microenvironments. At the internal microenvironment of spheroids the oxygen is low, producing hypoxic conditions, and these gradients are much larger than those obtained during 2-dimensional growth, where a larger surface area-to-volume ratio exists and all cells have equal contact with the medium.Citation42 Only the outer layer of cells in spheroids has direct contact with the medium, simulating in vivo conditions. Our results showed that DHEA decreased the size of spheroids of all the cell lines used (). When the size of the spheroids decrease, a smaller number of microenvironments is produced. Specifically, we think that hypoxic and starvation conditions are less acute, indicating that DHEA could be avoiding the positive selection of more aggressive tumor cells, which is additional to the inhibitory effect of DHEA on proliferation, migration and secretion of pro-inflammatory molecules.
It has been described that hypoxia induces genetic expression of pro-angiogenic genes.Citation43 Spheroids growing have shown that VEGF is inducible by hypoxia.Citation44 We think that DHEA could be decreasing the VEGF secretion in MCF-7 cells in 2 conditions: 1) decreasing its high secretion in normoxic conditions (), and 2) possibly decreasing expression and secretion of VEGF induced by hypoxia, due to the reduction of size of spheroids ().
Some studies have demonstrated that DHEA, at supra-physiological concentrations, has a protective effect against breast cancer; however, a tumorigenic role for DHEA has been described. For instance, the long-term administration and a high concentration of DHEA can produce hepatocellular carcinomas in rats.Citation45,46 On the other hand, DHEA can induce the proliferation of prostate cancer cells LNCaP.Citation47 Therefore, more studies both in vivo and in vitro are needed to clarify the mechanisms of the protective effect of DHEA on breast tumor cells.
In conclusion, our results showed that DHEA, at supra-phisiological concentrations, is able to inhibit several steps of the carcinogenesis process in breast cancer cells (). This effect was more evident in MCF-7 cells, the cell line with a low migration and invasion potential, indicating that DHEA could be useful alone or as an adjuvant in the treatment of some subtypes of breast cancer.
Figure 6. The metastatic process. During the initial steps of metastasis, tumor cells proliferate and loss the adhesion to neighbor cells detaching from the primary tumor (1). Individual tumor cells migrate and invade adjacent tissues and the basement membrane (2), reaching blood vessels or lymphatic channels, process called intravasation (3). Tumor cells are carried to distant organs, they extravasate (4) and proliferate to form a secondary tumor. DHEA inhibited some events related with the metastatic process (indicated with the red symbol) such as proliferation, migration and invasion, and induced a decrease of inflammatory molecules secretion in MCF-7 and MDA-MB-231 cells.
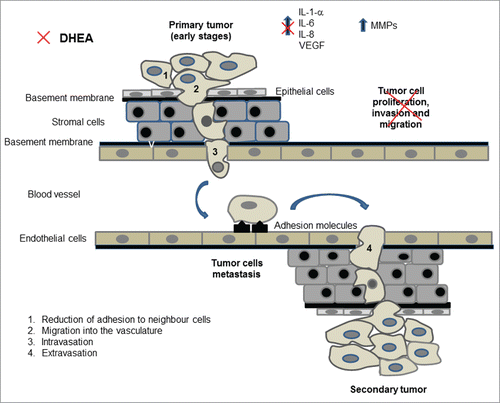
Materials and methods
Materials
RPMI medium, trypsin, antibiotics and fetal bovine serum (FBS) were purchased from GIBCO/BRL (Grand Island, NY, USA). Sterile plastic material for tissue culture was from Costar (Corning, NY, USA). Transwells and matrigel invasion chambers of 8 μm pore size were purchased from Becton Dickinson (Bedford, MA, USA). HCYTOMAG-60K | MILLIPLEX MAP Human Cytokine/Chemokine Magnetic Bead Panel-Immunology Multiplex Assay was purchased from Merck Millipore (Billerica, MA, USA). CytoSelect Cell Transformation Assay was from Cell Biolabs, INC (San Diego, CA, USA). Human matrix metalloproteinase antibody array C1 was from RayBiotech, Inc. (Norcross, GA, USA). DHEA (trans-dehydroandrosterone, minimum 99%) and the rest of the chemicals were purchased from Sigma Aldrich (St. Louis, MO, USA).
Cell culture
MCF-7, MDA-MB-231 and ZR-75-30 cell lines were purchased from ATCC (American Type Culture Collection, Manassas, VA, USA). Some characteristics of cell lines are showed in . All cells were grown in RPMI medium supplemented with 10% FBS, L-glutamine (2 mM) and 1% antibiotics (penicillin and streptomycin), and incubated at 37˚C in a 5% CO2-95% air environment.
Production of conditioned media (secretome)
Breast cancer cell lines were grown to confluence in 175 cm2 flasks. Then cell monolayer was washed 6 times with HEPES buffer (150 mM NaCl, 4.4 mM KCl, 10.9 mM Hepes, 12.2 mM glucose, pH 7.4) in order to remove serum components. Next, cultures were incubated in 20 ml of serum free RPMI medium without phenol red for 48 h. Conditioned media were collected, centrifuged and lyophilized. The resulting powders were dissolved in HEPES buffer (1/10 of the original volume) and dialyzed using a PM-12 ultra-filtration membrane (Millipore, Bedford, MA). To sterilize, the media were filtered using a 0.22 µm filter.
Migration assay
Two.5 × 105 /well cells were seeded onto 8 μm pore-size transwell filters in 200 μl of FBS free medium. To the lower chamber, 500 μl of medium with 10% FBS, and DHEA (100 μM) or vehicle (ethanol) was added. After 18, 24, and 48 h of treatment, cells on the topside of the filter were removed by scrubbing twice with a tipped swab. The migration of cells to the lower side of the filter was evaluated by crystal violet staining.
Crystal violet staining
The cells that migrated to the lower side of the filter were fixed with glutaraldehyde (1.1% in HEPES buffer) for 10 min. After this, cells were washed with de-ionized water, air-dried and stained with a 0.1 % crystal violet solution (in 200 mM phosphoric acid buffer, pH 6) for 20 min. After, cells were washed with de-ionized water to discard the no-incorporated crystal violet. The bound dye was solubilized with a 10% acetic acid solution. The optical density of each well was measured at 590 nm in a microplate spectrophotometer (Benchmark Plus, BioRad, Hercules, CA, USA).
Invasion in matrigel
Cell invasion was evaluated using matrigel, which is a basement membrane-like extracellular matrix extract. Cells were seeded onto 8 μm pore-size transwells membranes coated with matrigel at a concentration of 2.5 × 105 cells/well in 200 μl of FBS free medium. To the lower chamber, 500 μl of medium with 10% FBS, and DHEA (100 μM) or vehicle was added. After 24 and 48 h of treatment, the non-invading cells on the topside of the filter were removed by scrubbing twice with tipped swab. The invading cells on the lower surface of the membrane were evaluated by crystal violet staining.
Anchorage-independent growth assay
To test the effect of DHEA on anchorage-independent colony growth the CytoSelect Cell Transformation Assay was used according to the instructions of the manufacturer. Briefly, a base agar matrix layer was prepared in 2X RPMI medium supplemented with 20% FBS, and set in 96-well plates. Five thousands cells were resuspended in 2X RPMI medium supplemented with 20% FBS and mixed with agar matrix solution. The cell suspension was then dispensed into the wells already containing the base agar matrix layer. Fifty μl of medium containing 1, 10, and 100 μM DHEA were added to the wells. Plates were incubated at 37°C and 5% CO2 for 7 d. Colony formation was examined under a light microscope. For the quantification of anchorage-independent growth, the colonies were recovered after the solubilization of the agar matrix, and then incubated with MTT solution for 2 h at 37°C and 5% CO2. After incubation with detergent solution for 2 h, the absorbance at 570 nm was measured in a microtiter plate reader ELx800 (Bio Tek Instruments, Winooski, VT).
Spheroids culture
Breast cancer cells were cultured using the liquid overlay assay as was described by Yuhas et al.Citation48 Briefly, 1 × 106 cells were grown as a monolayer and seeded in petri dishes coated with a thin layer of 25% agarose for one week and then placed in a rotatory incubator at 37°C with Leibovitz L-15 medium, which was replaced every 2 d to eliminate malformed spheroids. Tumor spheroid growth was assessed by diameter measurement using a calibrated eye-piece graticule.
Secretion of molecules in the secretome
In order to evaluate the constituents from media, a MILLIPLEX MAP Human Cytokine/Chemokine Magnetic Bead Panel-Immunology Multiplex Assay was used. This is a microsphere-based immunoassay, which utilizes Luminex™ beads coupled to specific antibodies, as an analyte capture platform. In total, 25 μl of each secretome were used for detecting the secreted factors. Samples were added in duplicate to 96-well plates containing polystyrene beads from the 20-plex assay kit, and the beads were filter-washed twice with Bio-Plex wash buffer using a vacuum manifold (Millipore, Bedford, MA). Human cytokine standards were added to the antibody-conjugated beads, and incubated in the dark on a platform shaker for 30 min. Following incubation, samples and standards were removed by vacuum, and the beads were filter-washed 3 times with Bio-Plex wash buffer. Afterwards, a 1:50 dilution of biotinylated detection antibody was added to the beads, followed by incubation in the dark on a platform shaker for 30 min. The beads were washed 3 times and reacted with a 1:100 dilution of streptavidin-phycoerythrin (PE) for 10 min. The beads were washed 3 times as described above, resuspended in Bio-Plex assay buffer, and analyzed on a Bio-Plex plate reader.
MMPs and TIMPs expression
The expression of MMPs and TIMPs in the conditioned media derived from breast cancer cell lines, was determined with the human matrix metalloproteinase antibody array C1 according to the instructions of the manufacturer. Membranes containing the antibody arrays were blocked with 2 ml of blocking buffer for 30 min at room temperature. The blocking buffer was aspirated and 1 ml of each conditioned medium was added and incubated overnight at 4°C. The medium was discarded and membranes were washed 3 times with 2 ml of wash buffer for 5 min. After, membranes were incubated with 1 ml of biotinylated antibody cocktail overnight at 4°C. The cocktail was aspirated, membranes were washed twice with 2 ml of wash buffer and incubated with 2 ml of 1X HRP-streptavidin for 2 h at room temperature. After this, membranes were washed twice again with 2 ml of wash buffer for 5 min. Finally, membranes were incubated with detection buffer for 2 min and the luminescence was analyzed in a ChemiDocTM MP Imaging System (BIO-RAD, Hercules, CA, USA) with the software Image Lab 5.0.
Statistical analysis
All experiments were performed in 3 independent trials. The majority of results are expressed as means ± standard error of the mean (SEM), and were analyzed using one-way analysis of variance (ANOVA-test) and a Tukey post hoc performed with the GraphPad Prism software version 5.01. A P-value of <0 .01 was considered statistically significant.
Disclosure of potential conflicts of interest
No potential conflicts of interest were disclosed.
Authors contribution
RLM, designed the project and wrote the manuscript; EZG, performed the migration and invasion assays; LRZ and CA, evaluated the growth of cells in soft agar; MEC and JMZ, performed the spheroids assay; NMC, determined the expression of proteins in the secretome; CHG and RGA, determined the secretion of MMPs and TIMPs; EOGG, obtained the secretomes.
Funding
This investigation was financially supported by CONACyT (grant 182341).
References
- Watson RR, Huls A, Araghinikuam M, Chung S. Dehydroepiandrosterone and diseases of aging. Drugs Aging 1996; 9:274-91; PMID:8894525; http://dx.doi.org/10.2165/00002512-199609040-00005
- Tchernof A, Labrie F. Dehydroepiandrosterone, obesity and cardiovascular disease risk: a review of human studies. Eur J Endocrinol 2004; 151:1-14; PMID:15248817; http://dx.doi.org/10.1530/eje.0.1510001
- Gutiérrez G, Mendoza C, Zapata E, Montiel A, Reyes E, Montaño LF, López-Marure R. Dehydroepiandrosterone inhibits the TNF-α-induced inflammatory response in human umbilical vein endothelial cells. Atherosclerosis 2007; 190:90-9; PMID:16574124; http://dx.doi.org/10.1016/j.atherosclerosis.2006.02.031
- Wang L, Hao Q, Wang YD, Wang WJ, Li DJ. Protective effects of dehydroepiandrosterone on atherosclerosis in ovariectomized rabbits via alleviating inflammatory injury in endothelial cells. Atherosclerosis 2011; 214:47-57; PMID:21071029; http://dx.doi.org/10.1016/j.atherosclerosis.2010.07.043
- Schwartz AG, Pashko L, Whitcomb JM. Inhibition of tumor development by dehydroepiandrosterone and related steroids. Toxicol Pathol 1986; 14:357-62; PMID:3024302; http://dx.doi.org/10.1177/019262338601400312
- Gayosso V, Montano LF, López-Marure R. DHEA-induced antiproliferative effect in MCF-7 cells is androgen- and estrogen receptor-independent. Cancer J 2006; 12:160-5; PMID:16630408
- López-Marure R, Contreras PG, Dillon JS. Effects of dehydroepiandrosterone on proliferation, migration, and death of breast cancer cells. Eur J Pharmacol 2011; 660:268-74; PMID:21497598; http://dx.doi.org/10.1016/j.ejphar.2011.03.040
- Ortega-Calderón YN, López-Marure R. Dehydroepiandrosterone inhibits proliferation and suppresses migration of human cervical cancer cell lines. Anticancer Res 2014; 34:4039-44; PMID:25075027
- Knaul FM, Nigenda G, Lozano R, Arreola-Ornelas H, Langer A, Frenk J. Breast cancer in México: an urgent priority. Salud Publica Mex 2009; 51(2):s335-44; PMID:19967291; http://dx.doi.org/10.1590/S0036-36342009000800026
- Klevesath MB, Pantel K, Agbaje O, Provenzano E, Wishart GC, Gough P, Pinder SE, Duffy S, Purushotham AD. Patterns of metastatic spread in early breast cancer. Breast 2013; 22:449-54; PMID:23726130; http://dx.doi.org/10.1016/j.breast.2013.04.017
- Talmadge JE, Fidler IJ. AACR centennial series: The biology of cancer metastasis: historical perspective. Cancer Res 2010; 70:5649-69; PMID:20610625; http://dx.doi.org/10.1158/0008-5472.CAN-10-1040
- Li DM, Feng YM. Signaling mechanism of cell adhesion molecules in breast cancer metastasis: potential therapeutic targets. Breast Cancer Res Treat 2011; 128:7-21; PMID:21499686; http://dx.doi.org/10.1007/s10549-011-1499-x
- Egeblad M, Werb Z. New functions for the matrix metalloproteinases in cancer progression. Nat Rev Cancer 2002; 2:161-74; PMID:11990853; http://dx.doi.org/10.1038/nrc745
- Dano K, Behrendt N, Hoyer-Hansen G, Johnsen M, Lund LR, Ploug M, Romer J. Plasminogen activation and cancer. Thromb Haemost 2005; 93:676-81; PMID:15841311
- Stetler-Stevenson WG. Tissue inhibitors of metalloproteinases in cell signaling: metalloproteinase-independent biological activities. Sci Signal 2008; 1:re6; PMID:18612141; http://dx.doi.org/10.1126/scisignal.127re6
- Takawale A, Sakamuri SS, Kassiri Z. Extracellular matrix communication and turnover in cardiac physiology and pathology. Compr Physiol 2015; 5:687-719; PMID:25880510; http://dx.doi.org/10.1002/cphy.c140045
- Hanahan D, Weinberg RA. “Hallmarks of cancer: the next generation.” Cell 2011; 144:646-74; PMID:21376230; http://dx.doi.org/10.1016/j.cell.2011.02.013
- Yadav L, Puri N, Rastogi V, Satpute P, Ahmad R, Kaur G. Matrix metalloproteinases and cancer – roles in threat and therapy. Asian Pac J Cancer Prev 2014; 15:1085-91; PMID:24606423; http://dx.doi.org/10.7314/APJCP.2014.15.3.1085
- Guadamillas MC, Cerezo A, Del Pozo MA. Overcoming anoikis-pathways to anchorage-independent growth in cancer. J Cell Sci 2011; 124:3189-97; PMID:21940791; http://dx.doi.org/10.1242/jcs.072165
- Sutherland RM, McCredie JA, Inch WR. Growth of multicell spheroids in tissue culture as a model of nodular carcinomas. J Natl Cancer Inst 1971; 46:113-20; PMID:5101993
- Kunz-Schughart LA, Kreutz M, Knuechel R. Multicellular spheroids: a three-dimensional in vitro culture system to study tumour biology. Int J Exp Pathol 1998; 79:1-23; PMID:9614346; http://dx.doi.org/10.1046/j.1365-2613.1998.00051.x
- Hughes L, Malone C, Chumsri S, Burger AM, McDonnell S. Characterization of breast cancer cell lines and establishment of a novel isogenic subclone to study migration, invasion and tumourigenicity. Clin Exp Metastasis 2008; 25:549-57; PMID:18386134; http://dx.doi.org/10.1007/s10585-008-9169-z
- Yang C, Chen H, Yu L, Shan L, Xie L, Hu J, Chen T, Tan Y. Inhibition of FOXM1 transcription factor suppresses cell proliferation and tumor growth of breast cancer. Cancer Gene Ther 2013; 20:117-24; PMID:23306612; http://dx.doi.org/10.1038/cgt.2012.94
- Xia TS, Wang GZ, Ding Q, Liu XA, Zhou WB, Zhang YF, Zha XM, Du Q, Ni XJ, Wang J, et al. Bone metastasis in a novel breast cancer mouse model containing human breast and human bone. Breast Cancer Res Treat 2012; 132:471-86; PMID:21638054; http://dx.doi.org/10.1007/s10549-011-1496-0
- Zhong Y, Ji B. Impact of cell shape on cell migration behavior on elastic substrate. Biofabrication 2013; 5:15011-20; PMID:23302223; http://dx.doi.org/10.1088/1758-5082/5/1/015011
- Friedl P, Wolf K. Tube travel: the role of proteases in individual and collective cancer cell invasion. Cancer Res 2008; 68:7247-9; PMID:18794108; http://dx.doi.org/10.1158/0008-5472.CAN-08-0784
- Mierke CT, Rosel D, Fabry B, Brabek J. Contractile forces in tumor cell migration. Eur J Cell Biol 2008; 87:669-76; PMID:18295931; http://dx.doi.org/10.1016/j.ejcb.2008.01.002
- Kleinman HK, Martin GR. Matrigel: basement membrane matrix with biological activity. Semin Cancer Biol 2005; 15:378-86; PMID:15975825; http://dx.doi.org/10.1016/j.semcancer.2005.05.004
- Benton G, Arnaoutova I, George J, Kleinman HK, Koblinski J. Matrigel: from discovery and ECM mimicry to assays and models for cancer research. Adv Drug Deliv Rev 2014; 79-80:3-18; PMID:24997339; http://dx.doi.org/10.1016/j.addr.2014.06.005
- Kim YS, Kim SH, Kang JG, Ko JH. Expression level and glycan dynamics determine the net effects of TIMP-1 on cancer progression. BMB Rep 2012; 45:623-8; PMID:23187000; http://dx.doi.org/10.5483/BMBRep.2012.45.11.233
- Boccuzzi G, Brignardello E, di Monaco M, Forte C, Leonardi L, Pizzini A. Influence of dehydroepiandrosterone and 5-en-androstene-3 β, 17 β-diol on the growth of MCF-7 human breast cancer cells induced by 17 β-estradiol. Anticancer Res 1992; 12:799-803; PMID:1535770
- Slamon DJ, Clark GM, Wong SG, Levin WJ, Ullrich A, McGuire WL. Human breast cancer: correlation of relapse and survival with amplification of the HER-2/neu oncogene. Science 1987; 235:177-82; PMID:3798106; http://dx.doi.org/10.1126/science.3798106
- Dillon JS. Dehydroepiandrosterone, dehydroepiandrosterone sulfate and related steroids: their role in inflammatory, allergic and immunological disorders. Curr Drug Targets Inflamm Allergy 2005; 4:377-85; PMID:16101547; http://dx.doi.org/10.2174/1568010054022079
- Singer CF, Hudelist G, Gschwantler-Kaulich D, Fink-Retter A, Mueller R, Walter I, Czerwenka K, Kubista E. Interleukin-1alpha protein secretion in breast cancer is associated with poor differentiation and estrogen receptor α negativity. Int J Gynecol Cancer 2006; 16(2):556-9; PMID:17010072; http://dx.doi.org/10.1111/j.1525-1438.2006.00695.x
- Heikkilä K, Ebrahim S, Lawlor DA. Systematic review of the association between circulating interleukin-6 (IL-6) and cancer. Eur J Cancer 2008; 44:937-45; PMID:18387296; http://dx.doi.org/10.1016/j.ejca.2008.02.047
- Ning Y, Manegold PC, Hong YK, Zhang W, Pohl A, Lurje G, Winder T, Yang D, LaBonte MJ, Wilson PM, et al. Interleukin-8is associated with proliferation, migration, angiogenesis and chemosensitivity in vitro and in vivo in colon cancer cell line models. Inter J Cancer 2011; 128:2038-49; PMID:20648559; http://dx.doi.org/10.1002/ijc.25562
- Clendenen TV, Koenig KL, Arslan AA, Lukanova A, Berrino F, Gu Y, Hallmans G, Idahl A, Krogh V, Lokshin AE, et al. Factors associated with inflammation markers, a cross-sectional analysis. Cytokine 2011; 56:769-78; PMID:22015105; http://dx.doi.org/10.1016/j.cyto.2011.09.013
- Fujisaki K, Fujimoto H, Sangai T, Nagashima T, Sakakibara M, Shiina N, Kuroda M, Aoyagi Y, Miyazaki M. Cancer-mediated adipose reversion promotes cancer cell migration via IL-6 and MCP-1. Breast Cancer Res Treat 2015; 150:255-63; PMID:25721605; http://dx.doi.org/10.1007/s10549-015-3318-2
- Hicklin DJ, Ellis LM. Role of the vascular endothelial growth factor pathway in tumor growth and angiogenesis. J Clin Oncol 2005; 23:1011-27; PMID:15585754; http://dx.doi.org/10.1200/JCO.2005.06.081
- Shin SI, Freedman VH, Risser R, Pollack R. Tumorigenicity of virus-transformed cells in nude mice is correlated specifically with anchorage independent growth in vitro. Proc Natl Acad Sci USA 1975; 72:4435-9; PMID:172908; http://dx.doi.org/10.1073/pnas.72.11.4435
- Schwachoefer JH, Crooijmans RP, Hoogenhout J, Kal HB, Theeuwes AG. Sublethal damage repair in multicellular spheroids from a human melanoma line: Relationship to the size at irradiation. Cancer Ther Contr 1991; 1:293-308; PMID:1871510
- Sutherland RM. Cell and environment interactions in tumor microregions: the multicell spheroid model. Science 1988; 240:177-84; PMID:2451290; http://dx.doi.org/10.1126/science.2451290
- Span PN, Bussink J. Biology of hypoxia. Semin Nucl Med 2015; 45:101-9; PMID:25704383; http://dx.doi.org/10.1053/j.semnuclmed.2014.10.002
- Shweiki D, Neemant M, Itin A, Keshet E. Induction of vascular endothelial growth factor expression by hypoxia and by glucose deficiency in multicell spheroids: Implications for tumor angiogenesis. Proc Natl Acad Sci USA 1995; 92:768-72; PMID:7531342; http://dx.doi.org/10.1073/pnas.92.3.768
- Rao MS, Subbarao V, Yeldandi AV, Reddy JK. Hepatocarcinogenicity of dehydroepiandrosterone in the rat. Cancer Res 1992; 52:2977-9; PMID:1316232
- Metzger C, Bannasch P, Mayer D. Enhancement and phenotypic modulation of N-nitrosomorpholine-induced hepatocarcinogenesis by dehydroepiandrosterone. Cancer Lett 1997; 121:125-31; PMID:9570349; http://dx.doi.org/10.1016/S0304-3835(97)00341-8
- Arnold JT, Le H, McFann KK, Blackman MR. Comparative effects of DHEA vs. testosterone, dihydrotestosterone, and estradiol on proliferation and gene expression in human LNCaP prostate cancer cells. Am J Physiol Endocrinol Metab 2005; 288:E573-84; PMID:15536203; http://dx.doi.org/10.1152/ajpendo.00454.2004
- Yuhas JM, Tarleton AE, Molzen KB. Multicellular tumor spheroid formation by breast cancer cells isolated from different sites. Cancer Res 1978; 38:2486-91; PMID:667844
- Subik K, Lee JF, Baxter L, Strzepek T, Costello D, Crowley P, Xing L, Hung MC, Bonfiglio T, Hicks DG, et al. The Expression Patterns of ER, PR, HER2, CK5/6, EGFR, Ki-67 and AR by immunohistochemical analysis in breast cancer cell lines. Breast Cancer (Auckl) 2010; 4:35-41; PMID:20697531
- Marzec KA, Lin MZ, Martin JL, Baxter RC. Involvement of p53 in insulin-like growth factor binding protein-3 regulation in the breast cancer cell response to DNA damage. Oncotarget 2015; 6:26583-98; PMID:26378048; http://dx.doi.org/10.18632/oncotarget.5612
- Geng QQ, Dong DF, Chen NZ, Wu YY, Li EX, Wang J, Wang SM. Induction of p53 expression and apoptosis by a recombinant dual-target MDM2/MDMX inhibitory protein in wild-type p53 breast cancer cells. Int J Oncol 2013; 43:1935-42; PMID:24126697
- Yaacob NS, Nik Mohamed Kamal NN, Wong KK, Norazmi MN. Cell Cycle Modulation of MCF-7 and MDA-MB-231 by a Sub- Fraction of Strobilanthes crispus and its combination with Tamoxifen. Asian Pac J Cancer Prev 2015; 16:8135-40; PMID:26745050; http://dx.doi.org/10.7314/APJCP.2015.16.18.8135
- Charafe-Jauffret E, Ginestier C, Monville F, Finetti P, Adélaïde J, Cervera N, Fekairi S, Xerri L, Jacquemier J, Birnbaum D, et al. Gene expression profiling of breast cell lines identifies potential new basal markers. Oncogene 2006; 25:2273-84; PMID:16288205; http://dx.doi.org/10.1038/sj.onc.1209254
- Engel LW, Young NA, Tralka TS, Lippman ME, O'Brien SJ, Joyce MJ. Establishment and characterization of three new continuous cell lines derived from human breast carcinomas. Cancer Res 1978; 38:3352-64; PMID:688225
- Conley SJ, Bosco EE, Tice DA, Hollingsworth RE, Herbst R, Xiao Z. HER2 drives Mucin-like 1 to control proliferation in breast cancer cells. Oncogene 2016; 1-10