ABSTRACT
Glioblastoma multiforme (GBM) exhibits high resistance to the standard treatment of temozolomide (TMZ) combined with radiotherapy, due to its remarkable cell heterogeneity. Accordingly, there is a need to target alternative molecules enhancing specific GBM autocrine or paracrine mechanisms and amplifying the effect of standard treatment. Sphingosine 1-phosphate (S1P) is such a lipid target molecule with an important role in cell invasion and proliferation. Sphingosine kinase inhibitors (SKI) prevent S1P formation and induce increased production of reactive oxygen species (ROS), which may potentiate radiation cytotoxicity. We analyzed the effect of SKI singular versus combined treatments with TMZ and radiation on 2 human GBM cell lines characterized by a lack of MGMT expression and low or high expression of the anti-oxidant enzyme, glutathione peroxidase 1 (GPx1). Effects were drug concentration-, cell line-dependent and partly ROS-mediated. Clonogenic survival assay demonstrates that SKI was more effective than TMZ in increasing the sensitivity of U87 cells, which express low GPx1 amount, to a 2 Gy X-ray dose. Addition of both SKI and TMZ drastically decreased U87 cells survival compared with the combination temozolomide/radiation. SKI less effectively than TMZ sensitized LN229 cells to the 2 Gy X-ray dose. Its combination to TMZ in absence of irradiation was as efficient as TMZ combination with X-ray. We provide first evidence for SKI as an alternative or complementary treatment to TMZ, and for efficient combinations of low doses of drugs and X-ray. These may help as novel bi-modal and tri-modal therapies to contend with GBM heterogeneity.
Introduction
Glioblastoma multiforme (GBM) is the most common malignant primary brain tumor characterized by high intra- and inter-tumor heterogeneity.Citation1-3 This heterogeneity is the hallmark of GBM resistance to the standard multi-modal treatment that encompasses surgery, radiation and chemotherapy with temozolomide.Citation4,5 The invasive nature of GBM cells and their diffusion in the brain parenchyma makes a total surgical rejection of the tumor impossible.Citation6 A known resistance factor to radiation therapy is the hypoxic microenvironment, a characteristic feature of GBM.Citation7 The existence of oxygen gradients within the tumor tissue and differences in oxygenation status among patients,Citation8 are common causes for planned therapy failure and poor patient prognosis.Citation9 For those reasons, it is important for treatment planning to assess tissue hypoxia and resistance mechanisms induced by hypoxia (e.g. induction of stemness and invasion and migration).Citation10,11 It has recently been shown that cells exposed to chronic cyclic hypoxia became tolerant hence resistant to ROS-inducing treatments such as ionizing radiation via the upregulation of their anti-oxidant capacity.Citation12 We have observed that one of the main enzymes involved in ROS detoxification, the glutathione peroxidase 1 (GPx1), was an essential component of the resistance of GBM cells to oxidative stress and we postulated its critical role in the regulation of the oxidative stress response in GBM.Citation13
TMZ is an imidazotetrazine alkylating agent that crosses the blood brain barrier. The cytotoxicity of TMZ is the result of the formation of O6-methylguanine (O6 MeG) in the DNA which causes mispairing during DNA replication and thus DNA damage.Citation14 However, tumor cells can circumvent the effect of TMZ when expressing O6MeG DNA methyltransferase (MGMT) that demethylates the O-6 position of the substrate guanine in the DNA strand.Citation15,16 In view of these various resistance mechanisms, it is not surprising that the median survival of GBM patients is 15–25 months after diagnosis and treatment.Citation17 Considering the poor prognostics for patients, the pressing need for novel therapeutic approaches is thus evident.Citation18
Sphingosine-1-phosphate (S1P) is a bioactive lipid involved in glioblastoma proliferation, invasion and survival.Citation19 Neurons and astrocytes produce S1P as well as GBM cells. S1P production actually increases in GBM and has been reported to correlate with the malignancy grade.Citation20 S1P results from the phosphorylation of sphingosine by 2 intracellular isoenzymes: sphingosine kinases 1 and 2 (SphK1/2)Citation21 and is translocated extracellularly. S1P binds to extracellular S1P receptorsCitation22 which in turn induce autocrine signaling cascadesCitation23 and promote GBM cells survival and migration.Citation24-26 Inhibitors of SphK thus represent promising anti-cancer agentsCitation27,28 that may act at different levels. Besides impacting on S1P production and its functional consequences, inhibition of SphK1 has been reported to lead to death of glioblastoma cellsCitation29 and to sensitize cells to chemotoxic drugs.Citation30 Moreover, it might have a radiosensitizing potential. SphK inhibition was indeed reported to induce formation of reactive oxygen species (ROS) in carcinoma cells.Citation31 We have shown that the pharmacological inhibition of SphK by a low dose (10 µM) of the SphK inhibitor (SKI) SKI-IICitation32 induces an endoplasmic reticulum stress and an oxidative stress in a human GBM cell line.Citation33 We furthermore demonstrated that combination of such low doses of SKI-II and non-lethal doses of TMZ induces cell death in various human GBM cell lines. This effect was specifically directed at GBM cells and no other cell types.Citation33 Based on these observations, we decided to address the potential of SKI to interact with ionizing radiation by using the clonogenic assays.Citation34 We examined the radiosensitivity of the 2 human glioblastoma cell lines U87 and LN229 after mono SKI treatment and after its combination with TMZ. Our findings show a clear increase in radiosensitivity for both cell lines pretreated with sub-lethal concentrations of SKI and TMZ before application of low X-ray doses.
Results
Effect of sphingosine kinase inhibitor and temozolomide on cell survival
To evaluate the effect of the sphingosine kinase inhibitor (SKI-II, referred thereafter as SKI) on glioblastoma cell survival, U87 and LN229 cells were treated with increasing SKI doses (, left panel), chosen on the basis of our previous observations.Citation29,33 The results of the clonogenic survival demonstrate a clear, dose-dependent cytotoxic effect of SKI on both cell lines. This effect was comparable in efficacy to the known cytotoxic effect of temozolomide (TMZ; , right panel). However, the cell lines displayed different sensitivities. The cytotoxic effect of TMZ was significantly more pronounced in LN229 cells, whereas SKI more strongly affected the survival of U87 cells.
Figure 1. Clonogenic survival of U87 and LN229 cells after treatment with different concentrations of sphingosine kinase inhibitor (SKI, left panel) and temozolomide (TMZ, right panel). Data points represent the means and standard deviations. Statistical significance for the differences in survival between U87 and LN229 cells is calculated using 2-tailed Student's t-test (*p < 0.05, n = 3).
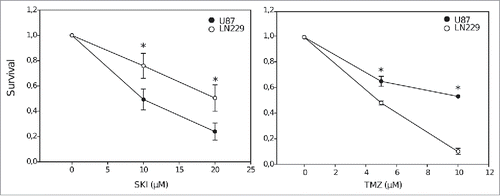
Comparison of clonogenic survival after irradiation and drug treatment
We then assessed the potential of singular and combined treatment to increase the cells sensitivity to radiation. SKI and TMZ were applied at low (5 µM TMZ, 10 µM SKI) and at higher (10 µM TZM, 20 µM SKI) concentrations before irradiation with a low, clinically relevant X-ray fraction dose (2 Gy). As shown in , a mono-treatment of U87 cells with SKI was as effective (at the low concentration) as, and more effective (at the higher concentration) than the applied dose of X-ray. SKI effect was not affected by its combination to TMZ at any dose. Low concentrations of SKI, TMZ and the combination of both had similar effects on cells sensitivity to the clinically relevant X-ray dose. Increase in drug concentrations led to increased radiation cytotoxicity after treatment with SKI, which was more efficient than TMZ. The increased radiosensitivity triggered by SKI resulted in abrogation of survival when SKI was combined with TMZ, indicating the strong cytotoxic efficacy of this tri-modal treatment. shows that LN229 cells were not as sensitive as the U87 cells to treatment with the low concentrations of drugs. Their response profile is however similar to that of the U87 cells, except that the effects observed for SKI in U87 cells are those observed for TMZ in LN229 cells, reflecting the higher sensitivity of LN229 cells to TMZ (, left). Mono-treatment of LN229 cells with increased concentrations of SKI or TMZ strongly enhanced their radiosensitivity. The combination of the drugs at those higher concentrations was very cytotoxic per se with no remaining surviving colonies and, as expected, addition of X-ray did not amplify this effect (, right).
Figure 2. Effect of irradiation, sphingosine kinase inhibitor and temozolomide on clonogenic cell survival. Data are presented as means and standard deviations of clonogenic survival of U87 (A) and LN229 (B) cells after treatment with different concentrations of sphingosine kinase inhibitor (SKI) and/or temozolomide (TMZ) with or without X-ray irradiation (2 Gy). **p < 0.01; ***p < 0.005; ****p < 0.0001 (n = 3, Student's t-test). Significant differences not indicated in the graphs for the clarity are in : **p (low SKI vs low TMZ), ***p (low TMZ vs low SKI+TMZ; higher TMZ+2Gy vs higher SKI+2Gy), ****p (higher SKI vs higher TMZ; higher TMZ vs higher SKI+TMZ); in : *p (low SKI vs low TMZ), **p (higher SKI vs higher TMZ), ****p (higher SKI vs higher SKI+TMZ). Differences in conditions not depicted with a p value on the graphs and in the legend are non-significant.
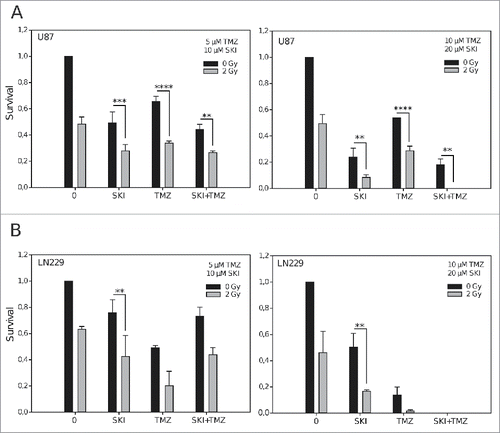
Combination of different irradiation doses with SKI and TMZ
We next tested the effects of increasing the X-ray doses on cells pretreated with the fixed low and higher concentrations of SKI and TMZ. Increase in irradiation led to the clear enhancement of cytotoxicity, when combined with low concentrations of SKI and TMZ, both in U87 and LN229 cells (, ). As reported in , the combination of higher concentrations of SKI and TMZ was per se very cytotoxic, namely for the LN229 cells. An additional effect of irradiation was not observed in case of the LN229 cells, whereas addition of sub-lethal X-ray irradiation doses (1 and 2 Gy) sufficed to decrease U87 cells survival below 10%.
Role of reactive oxygen species in cell survival after irradiation and drug treatment
The previous results indicate a clear difference between the cell lines response to the cytotoxic effects of single or combined treatment. LN229 cells, which similarly to U87 cells lack a detectable expression of MGMTCitation35 (, right), however were more sensitive to TMZ cytotoxicity (). U87 cells, which in contrary to LN229 cells express lower levels of the anti-oxidant glutathione peroxidase 1 (GPx1) (, left) were more sensitive to SKI toxicity (). This prompted us to investigate the possible role of reactive oxygen species (ROS) in the cell death induced by the drug and irradiation treatment. For this purpose, cell death rescue experiments were performed with the ROS scavenger N-acetylcysteine (NAC).Citation35 As shown in , NAC per se was not cytotoxic to the cells. Addition of NAC to any drug and irradiation condition did not affect the survival of LN229 cells significantly. It however significantly increased the survival of U87 cells to SKI or TMZ treatment, to irradiation and to the combination of irradiation with either drug. These results suggest that ROS induced by SKI or irradiation contribute to a larger extent to cell death in the GPx1 deficient U87 cells than in the GPx1 proficient LN229 cells.
Figure 4. (A) Western blot analysis for glutathione peroxidase 1 (GPx1, left) and O-6-methylguanine-DNA methyltransferase (MGMT, right) expressions in U87 and LN229 cells. LN18 cell lysates were used as a positive control for MGMT expression. (B) Cell death rescue experiment: clonogenic survival of U87 and LN229 cells after treatment with or without NAC in presence or absence of SKI (20 µM), TMZ (10 µM) and with or without X-ray irradiation (2 Gy). Non-treated cells were used as a control (CTRL). Data are presented as means and standard deviations. Values were normalized to the respective drug (SKI or TMZ) without irradiation. Statistical significance for differences between samples with and without NAC was calculated using 2-way ANOVA and Bonferroni post-tests. *p < 0.05; **p < 0.01; (n = 3).
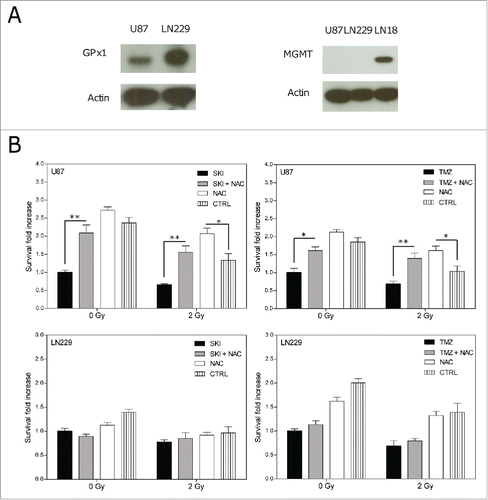
Discussion
Since the first clinical trials with dual TMZ and irradiation therapy that showed improved GBM patient prognosis,Citation36 TMZ has become part of the standard therapy for GBM patients. Even though the combination of TMZ and radiotherapy showed certain improvement for GBM patients, the recurrence rate is still high and median survival is unsatisfactory. Currently, different experimental strategies and clinical trials are evaluating potential radiosensitizers for GBM treatment.Citation37,38 Only few studies have analyzed the radiosensitizing potential of SKI. This has been shown up to now in the case of prostate and head and neck cancer cells.Citation39,40 To our knowledge, the study we report here demonstrates for the first time the capacity of SKI to strongly increase the radiosensitivity of 2 human GBM cell lines, thus supporting its radiosensitizing potential. This effect is even higher than that of TMZ in U87 cells, a cell line expressing low level of GPx1, hence lacking an efficient anti-oxidant response. Moreover, this study indicates the efficacy in combining oxidative stress-inducing drugs such as SKI and TMZ with irradiation, putting forward a basis for alternative tri-modal therapies.
Characterization of the sensitivity of the 2 cell lines to SKI and TMZ revealed an interesting correlation between a high sensitivity to SKI and low GPx1 expression. The low GPx1 expression is expected to negatively affect the overall capacity of the cells to scavenge ROS. As a consequence, accumulation of ROS generated through SphK inhibition should results in low cell survival. We therefore tested this hypothesis with a cell death rescue experiment. Utilization of the ROS scavenger NAC successfully altered SKI cytotoxicity in U87 cells. This result, in agreement with our previous demonstration for another GBM cell line,Citation13,33 indicates the contribution of ROS to the SKI-induced cell death. These current observations thus emphasize the therapeutic value of GPx1 as an indicator for the cell sensitivity to oxidative stress, by irradiation (note the protective effect of NAC to irradiation cytotoxicity in U87 cells) or by ROS inducing compounds such as SKI. NAC also protected the U87 cells against the cytotoxicity of 10 µM TMZ, suggesting a possible contribution of ROS to TMZ effects, as previously reported.Citation41 Sensitivity of the 2 cell lines to TMZ inversely correlated with their sensitivity to SKI. Difference in TMZ sensitivity could not be assessed to a difference in their expression of MGMT, which both lacked. However, TMZ resistance is not only linked to MGMT expression and a plausible explanation for the higher sensitivity of LN229 cells to TMZ might lie in their lack of alternative DNA repair mechanisms.Citation42
At low concentrations, the effects of TMZ or SKI to potentiate cell sensitivity to radiation were comparable, in each cell line. The cytotoxic effect of this bi-modal treatment increased with higher concentrations of each drug and correlated with the sensitivity of the cell line to either SKI or TMZ. The efficacy of combining both drugs in absence of irradiation was concentration-dependent and varied according to the sensitivity of the cell line to SKI or TMZ. Pretreatment with low concentrations of SKI combined to TMZ drastically increased the radiosensitivity of both cell lines. The strong improvement in cytotoxicity indicates the strong beneficial effect of such a tri-modal treatment over the singular irradiation or singular drug combination treatment.
Considering the cellular heterogeneity of GBM, it is conceivable that cells resembling U87 and LN229 cells, i.e. with various capacities for anti-oxidant response or DNA repair functions, might co-exist in the same tumor. Our present data illustrate the therapeutic relevance of tri-modal options in such conditions. We would like to point out that we used low concentrations of SKI (10 and 20 µM) that we showed to be non-lethal for non tumoral cells, such as astrocytes and neurons.Citation29,33 We used as well concentrations of TMZ (5 and 10 µM), which are much lower than those usually applied for in vitro assays (100 µM). Singular or combined uses of these low concentrations however were very effective in potentiating the radiation cytotoxicity in the cell lines we tested.
Altogether, our data strengthen the therapeutic relevance of SKI as a radiosensitisizer. They confirm the therapeutic value of GPx1 and that of oxidative status as relevant parameters for treatment strategies. Further studies to demonstrate the clinical relevance of such tri-modal options to these highly heterogeneous tumors are warranted.
Material and methods
Cell lines and culture conditions
The human glioblastoma cell lines U87 and LN229 were obtained from the American Type Culture Collection (ATCC). Cells were grown in complete growth medium (cDMEM), consisting of Dulbecco's Modified Eagle Medium (DMEM, Sigma) supplemented with 10% heat-inactivated Fetal Calf Serum (FCS, PAA), 2 mM glutamine (Invitrogen) and 50 µg/ml Gentamycin (Invitrogen) at 37° C and 5% CO2 atmosphere.
Cell treatment
Cells were seeded in triplicates in 6-well plates containing cDMEM in numbers necessary for forming individual colonies (300 to 2500 cells depending on the cell line and condition). After 1 to 2 h attachment to the plates, cells were mock treated (DMSO 0.1%) or with temozolomide (5 µM or 10 µM, Sigma) and/or with the sphingosine kinase inhibitor SKI-II (10 µM or 20 µM; Sigma) for 2 h at 37°C, 5% CO2. Both temozolomide and SKI-II were dissolved in DMSO and diluted in cDMEM. Cells were thereafter left non-irradiated or irradiated at different X-ray doses. After irradiation, cells were incubated for an additional 2 h in presence or absence of the drugs. At the end of this 4 h treatment, supernatants were removed by aspiration, cells were washed with PBS, fresh cDMEM was added and the plates were returned to the incubator for colony formation. Non-treated cells and non-irradiated cells were used as a control.
For cell death rescue experiments, cells were seeded, treated and incubated for colonies formation as described above, with the following modifications: 200 LN229 and 400 U87 cells per well were seeded in triplicates; 5 mM N-Acetylcystein (NAC, Sigma; dissolved in DMSO and diluted in cDMEM) was added at the time of the treatment with 20 µM SKI or 10 µM TMZ. Non-treated cells and non-irradiated cells were used as a control.
Irradiation set-up
Cells were irradiated with 1, 2, 4, 6 gray (Gy) using X-RAD 320 cabinet X-ray irradiator (Precision X-ray) at 320 keV and a dose rate of 112 cGy/min. Filter comprised 0.75 mm tin + 0.25 mm copper + 1.5 mm aluminum (half value layer = 3.7 mm copper).
Clonogenic survival assay
Clonogenic survival assay was modified from a described previously protocol.Citation34 After colony formation, cells were fixed with 3.7% paraformaldehyde for 5 min and labeled with 0.05% crystal violet for 15 min. Colonies containing more than 50 cells were counted as survivors. Curve fitting for surviving fraction was done using linear quadratic model as described.Citation43
Protein expression analysis
U87 and LN299 cells were lysed and western blot performed as described.Citation13 Membranes were incubated with primary antibodies in the blocking buffer (PBS containing 5% milk and 0.1% Tween-20) overnight at 4° C. The primary antibody against human glutathione peroxidase 1 (GPx1, Abcam) was used at the dilution of 1:800. The primary antibody against O-6-methylguanine-DNA methyltransferase (MGMT; Cell Signaling) was used at the dilution of 1:1000. The loading control primary antibody against actin (MP Biomedical) was used at the dilution of 1:2000.
Statistical analysis
Data are presented as means and standard deviations (SD). Statistical significance was determined using unequal variance t-test (2 tailed) or 2 way ANOVA. The asterisks represent significantly different values. *: p < 0.05; **: p < 0.01; ***: p < 0.005; ****: p < 0.0001. Experiments were performed 3 times.
Disclosure of potential conflicts of interest
No potential conflicts of interest were disclosed.
Acknowledgment
We gratefully acknowledge the support of Dr. Andrea Mairani.
Funding
This work was partly supported by German Research Council (DFG-KFO214), Deutsche Krebshilfe (Max-Eder 108876) and intramural grants from National Center for Tumor diseases (NCT/DKFZ-DKTK, Heidelberg, Germany).
References
- Cloughesy TF, Cavenee WK, Mischel PS. Glioblastoma: From molecular pathology to targeted treatment. Annu Rev Pathol 2014; 9:1-25; PMID:23937436; https://doi.org/10.1146/annurev-pathol-011110-130324
- Inda M-D-M, Bonavia R, Seoane J. Glioblastoma multiforme: A look inside its heterogeneous nature. Cancers (Basel) 2014; 6(1):226-39; PMID:24473088; https://doi.org/10.3390/cancers6010226
- Karsy M, Gelbman M, Shah P, Balumbu O, Moy F, Arslan E. Established and emerging variants of glioblastoma multiforme: Review of morphological and molecular features. Folia Neuropathol 2012; 50(4):301-21; PMID:23319187; https://doi.org/10.5114/fn.2012.32361
- Gilbert MR, Wang M, Aldape KD, Stupp R, Hegi ME, Jaeckle KA, Armstrong TS, Wefel JS, Won M, Blumenthal DT, et al. Dose-dense temozolomide for newly diagnosed glioblastoma: A randomized phase III clinical trial. J Clin Oncol 2013; 31(32):4085-91; PMID:24101040; https://doi.org/10.1200/JCO.2013.49.6968
- Nanegrungsunk D, Onchan W, Chattipakorn N, Chattipakorn SC. Current evidence of temozolomide and bevacizumab in treatment of gliomas. Neurol Res 2015; 37(2):167-83; PMID:25033940; https://doi.org/10.1179/1743132814Y.0000000423
- Cuddapah VA, Robel S, Watkins S, Sontheimer H. A neurocentric perspective on glioma invasion. Nat Rev Neurosci 2014; 15(7):455-65; PMID:24946761; https://doi.org/10.1038/nrn3765
- Harris AL. Hypoxia — A key regulatory factor in tumour growth. Nat Rev Cancer 2002; 2(1):38-47; PMID:11902584; https://doi.org/10.1038/nrc704
- Vaupel P, Höckel M, Mayer A. Detection and characterization of tumor hypoxia using pO2 histography. Antioxid Redox Signal 2007; 9(8):1221-35; PMID:17536958; https://doi.org/10.1089/ars.2007.1628
- Combs SE, Schmid TE, Vaupel P, Multhoff G. Stress response leading to resistance in glioblastoma- The need for innovative radiotherapy (iRT) concepts. Cancers (Basel) 2016; 8(1):15; PMID:26771644; https://doi.org/10.3390/cancers8010015
- Li P, Zhou C, Xu L, Xiao H. Hypoxia enhances stemness of cancer stem cells in glioblastoma: An in vitro study. Int J Med Sci 2013; 10(4):399-407; PMID:23471193; https://doi.org/10.7150/ijms.5407 10.7150/ijms.5770 10.7150/ijms.5224
- Joseph J V, Conroy S, Pavlov K, Sontakke P, Tomar T, Eggens-Meijer E, Balasubramaniyan V, Wagemakers M, den Dunnen WF, Kruyt FA. Hypoxia enhances migration and invasion in glioblastoma by promoting a mesenchymal shift mediated by the HIFα -ZEB1 axis. Cancer Lett 2015; 359(1):107-16; PMID:25592037; https://doi.org/10.1016/j.canlet.2015.01.010
- Matschke J, Riffkin H, Klein D, Handrick R, Lüdemann L, Metzen E, Shlomi T, Stuschke M, Jendrossek V. Targeted inhibition of glutamine-dependent glutathione metabolism overcomes death resistance induced by chronic cycling hypoxia. Antioxid Redox Signal 2016; 25(2):89-107; PMID:27021152; https://doi.org/10.1089/ars.2015.6589
- Dokic I, Hartmann C, Herold-Mende C, Régnier-Vigouroux A. Glutathione peroxidase 1 activity dictates the sensitivity of glioblastoma cells to oxidative stress. Glia 2012; 60(11):1785-800; PMID:22951908; https://doi.org/10.1002/glia.22397
- Kanzawa T, Bedwell J, Kondo Y, Kondo S, Germano IM. Inhibition of DNA repair for sensitizing resistant glioma cells to temozolomide. J Neurosurg 2003; 99(6):1047-52; PMID:14705733; https://doi.org/10.3171/jns.2003.99.6.1047
- Jiang G, Li L-T, Xin Y, Zhang L, Liu Y-Q, Zheng J-N. Strategies to improve the killing of tumors using temozolomide: Targeting the DNA repair protein MGMT. Curr Med Chem 2012; 19(23):3886-92; PMID:22788764; https://doi.org/10.2174/092986712802002446
- van Nifterik KA, van den Berg J, van der Meide WF, Ameziane N, Wedekind LE, Steenbergen RDM, Leenstra S, Lafleur MV, Slotman BJ, Stalpers LJ, et al. Absence of the MGMT protein as well as methylation of the MGMT promoter predict the sensitivity for temozolomide. Br J Cancer 2010; 103(1):29-35; PMID:20517307; https://doi.org/10.1038/sj.bjc.6605712
- Karsy M, Neil JA, Guan J, Mark MA, Colman H, Jensen RL. A practical review of prognostic correlations of molecular biomarkers in glioblastoma. Neurosurg Focus 2015; 38(3):1-8; PMID:25727226; https://doi.org/10.3171/2015.1.FOCUS14755
- Desai R, Suryadevara CM, Batich KA, Farber SH, Sanchez-Perez L, Sampson JH. Emerging immunotherapies for glioblastoma. Expert Opin Emerg Drugs 2016; 21(2):133-45; PMID:27223671; https://doi.org/10.1080/14728214.2016.1186643
- Bien-möller S, Lange S, Holm T, Böhm A, Paland H, Küpper J, Herzog S, Weitmann K, Havemann C, Vogelgesang S, et al. Expression of S1P metabolizing enzymes and receptors correlate with survival time and regulate cell migration in glioblastoma multiforme. Oncotarget 2016; 7(11):13031-46; PMID:26887055; https://doi.org/10.18632/oncotarget.7366
- Abuhusain HJ, Matin A, Qiao Q, Shen H, Kain N, Day BW, Stringer BW, Daniels B, Laaksonen MA, Teo C, et al. A metabolic shift favoring sphingosine 1-phosphate at the expense of ceramide controls glioblastoma angiogenesis. J Biol Chem 2013; 288(52):37355-64; PMID:24265321; https://doi.org/10.1074/jbc.M113.494740
- Liu H, Chakravarty D, Maceyka M, Milstien S, Spiegel S. Sphingosine kinases: A novel family of lipid kinases. Prog Nucleic Acid Res Mol Biol 2002; 71:493-506; PMID:12102559; https://doi.org/10.1016/S0079-6603(02)71049-0
- Strub GM, Maceyka M, Hait NC, Milstien S, Spiegel S. Extracellular and intracellular actions of sphingosine-1-phosphate. Adv Exp Med Biol 2010; 688:141-55; PMID:20919652; https://doi.org/10.1007/978-1-4419-6741-1_10
- Rosen H, Goetzl EJ. Sphingosine 1-phosphate and its receptors: An autocrine and paracrine network. Nat Rev Immunol 2005;5(7):560-70; PMID:15999095; https://doi.org/10.1038/nri1650
- Quint K, Stiel N, Neureiter D, Schlicker HU, Nimsky C, Ocker M, Strik H, Kolodziej MA. The role of sphingosine kinase isoforms and receptors S1P1, S1P2, S1P3, and S1P5 in primary, secondary, and recurrent glioblastomas. Tumor Biol 2014; 35(9):8979-89; PMID:24903384; https://doi.org/10.1007/s13277-014-2172-x
- Anelli V, Gault CR, Cheng AB, Obeid LM. Sphingosine kinase 1 is up-regulated during hypoxia in U87MG glioma cells: Role of hypoxia-inducible factors 1 and 2. J Biol Chem 2008; 283(6):3365-75; PMID:18055454; https://doi.org/10.1074/jbc.M708241200
- Riccitelli E, Giussani P, Di Vito C, Condomitti G, Tringali C, Caroli M, Galli R, Viani P, Riboni L. Extracellular sphingosine-1-phosphate: A novel actor in human glioblastoma stem cell survival. PLoS One 2013; 8(6):1-11; PMID:23826381; https://doi.org/10.1371/journal.pone.0068229
- Kunkel GT, Maceyka M, Milstien S, Spiegel S. Targeting the sphingosine-1-phosphate axis in cancer, inflammation and beyond. Nat Rev Drug Discov 2013; 12(9):688-702; PMID:23954895; https://doi.org/10.1038/nrd4099
- Canals D, Hannun YA. Novel chemotherapeutic drugs in sphingolipid cancer research. Handb Exp Pharmacol 2013; (215):211-38; PMID:23579458; https://doi.org/10.1007/978-3-7091-1368-4_12
- Mora R, Dokic I, Kees T, Hüber CM, Keitel D, Geibig R, Brügge B, Zentgraf H, Brady NR, Régnier-Vigouroux A. Sphingolipid rheostat alterations related to transformation can be exploited for specific induction of lysosomal cell death in murine and human glioma. Glia 2010; 58(11):1364-83; PMID:20607862; https://doi.org/10.1002/glia.21013
- Gault CR, Obeid LM. Still benched on its way to the bedside: Sphingosine kinase 1 as an emerging target in cancer chemotherapy. Crit Rev Biochem Mol Biol 2011; 46(4):342-51; PMID:21787121; https://doi.org/10.3109/10409238.2011.597737
- Huwiler A, Kotelevets N, Xin C, Pastukhov O, Pfeilschifter J, Zangemeister-Wittke U. Loss of sphingosine kinase-1 in carcinoma cells increases formation of reactive oxygen species and sensitivity to doxorubicin-induced DNA damage. Br J Pharmacol 2011; 162(2):532-43; PMID:20883472; https://doi.org/10.1111/j.1476-5381.2010.01053.x
- French KJ, Schrecengost RS, Lee BD, Zhuang Y, Smith SN, Eberly JL, Yun JK, Smith CD. Discovery and evaluation of inhibitors of human sphingosine kinase. Cancer Res 2003; 63:5962-9; PMID:14522923
- Noack J, Choi J, Richter K, Kopp-Schneider A, Régnier-Vigouroux A. A sphingosine kinase inhibitor combined with temozolomide induces glioblastoma cell death through accumulation of dihydrosphingosine and dihydroceramide, endoplasmic reticulum stress and autophagy. Cell Death Dis 2014; 5:e1425; PMID:25255218; https://doi.org/10.1038/cddis.2014.384
- Franken NAP, Rodermond HM, Stap J, Haveman J, van Bree C. Clonogenic assay of cells in vitro. Nat Protoc 2006; 1(5):2315-9; PMID:17406473; https://doi.org/10.1038/nprot.2006.339
- Zafarullah M, Li WQ, Sylvester J, Ahmad M. Molecular mechanisms of N-acetylcysteine actions. Cell Mol Life Sci 2003; 60:6-20; PMID:12613655; https://doi.org/10.1007/s000180300001
- Stupp R, Mason WP, van den Bent MJ, Weller M, Fisher B, Taphoorn MJB, Belanger K, Brandes AA, Marosi C, Bogdahn U, et al. Radiotherapy plus concomitant and adjuvant temozolomide for glioblastoma. N Engl J Med 2005; 10(352):987-96; PMID:15758009; https://doi.org/10.1056/NEJMoa043330
- Diaz-Miqueli A, Saurez Martinez G. Nimotuzumab as a radiosensitizing agent in the treatment of high grade glioma: Challenges and opportunities. OncoTarget Ther 2013; 6:931-42; PMID:23926436; https://doi.org/10.2147/OTT.S33532
- Chinot OL, Wick W, Mason W, Henriksson R, Saran F, Nishikawa R, Carpentier AF, Hoang-Xuan K, Kavan P, Cernea D, et al. Bevacizumab plus radiotherapy-temozolomide for newly diagnosed glioblastoma. N Engl J Med 2014; 370(8):709-22; PMID:24552318; https://doi.org/10.1056/NEJMoa1308345
- Masood R, Roy I, Zu S, Hochstim C, Yong K-T, Law W-C, Ding H, Sinha UK, Prasad PN. Gold nanorod-sphingosine kinase siRNA nanocomplexes: A novel therapeutic tool for potent radiosensitization of head and neck cancer. Integr Biol (Camb) 2012; 4(2):132-41; PMID:22159374; https://doi.org/10.1039/C1IB00060H
- Pchejetski D, Böhler T, Stebbing J, Waxman J. Therapeutic potential of targeting sphingosine kinase 1 in prostate cancer. Nat Rev Urol 2011; 8(10):569-678; PMID:21912422; https://doi.org/10.1038/nrurol.2011.117
- Lin CJ, Lee CC, Shih YL, Lin TY, Wang SH, Lin YF, Shih CM. Resveratrol enhances the therapeutic effect of temozolomide against malignant glioma in vitro and in vivo by inhibiting autophagy. Free Radic Biol Med 2012; 52:377-91; PMID:22094224; https://doi.org/10.1016/j.freeradbiomed.2012.02.028 10.1016/j.freeradbiomed.2011.10.487
- Erasimus H, Gobin M, Niclou S, Van Dyck E. DNA repair mechanisms and their clinical impact in glioblastoma. Mutat Res Mutat Res 2016; 769:19-35; PMID:27543314; https://doi.org/10.1016/j.mrrev.2016.05.005
- Latz D, Fleckenstein K, Eble M, Blatter J, Wannenmacher M, Weber KJ. Radiosensitizing potential of gemcitabine (2′,2′-difluoro-2′ deoxycytidine) within the cell cycle in vitro. Int J Radiat Oncol Biol Phys 1998; 41(4):875-82; PMID:9652852; https://doi.org/10.1016/S0360-3016(98)00105-9