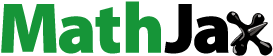
ABSTRACT
MicroRNAs had been proved to be pivotal regulators in nasopharyngeal carcinoma (NPC) by regulating a large amount of genes' expression. In our research, we aim to explore the functions of miR-9–3p on the metastases of NPC and figure out the potential mechanisms. First, we revealed downregulation of miR-9–3p and upregulation of fibronectin 1 (FN1), β1 integrin (ITGB1) and α5 integrin (ITGAV) expression in NPC tissues and cells compared with the normal using RNA-seq analysis, RT-qPCR, western blot and immunohistochemistry. By transfection of miR-9–3p mimics in CNE-1, CNE-2 and HONE-1 cells, we confirmed tumor-suppressing roles of miR-9–3p via suppressing EMT process by MTT, wound scratch, transwell assay and western blot. After constructing luciferase reporting plasmids and transient transfection in HEK 293T cells, we proved that FN1, ITGB1 and ITGAV were all targets of miR-9–3p. Then we manipulated the expression of miR-9–3p, FN1, ITGB1 and ITGAV in HONE-1 cells, verifying the tumor-promoting effect of FN1, ITGB1 and ITGAV on cell proliferation and metastases via facilitating EMT process of cells. Additionally, these functions of FN1, ITGB1 and ITGAV could be efficiently abrogated by overexpression of miR-9–3p. Taken together, we demonstrated that elevation of miR-9–3p suppresses the proliferation and metastases of NPC via downregulating FN1, ITGB1, ITGAV and inhibiting the EMT process, which provided a series of therapeutic targets for the treatment of NPC.
Introduction
Nasopharyngeal carcinoma (NPC) is regarded as the most malignant squamous-cell cancer of nasopharynx, which is prevalent especially in southern Asia.Citation1 Epstein-Barr virus infection, epigenetic factors and eating habits are widely recognized as main potential risks contributing to NPCCitation2 The standard first line treatment of NPC is radiotherapy combined with drug treatment or surgery in terms of its classification and stagingCitation3 However, the prognosis of patients having NPC is still poor due to the frequent local recurrence, potential radio-resistance and frequent distant metastasisCitation4 The most frequent metastasis location is the bone, followed by viscera and extra-cervical lymph nodes at low ratesCitation5 Therefore, the underlying mechanisms responsible for NPC metastases are urgently needed for searching novel therapeutic targets to improve the prognosis of NPC.
MicroRNAs (miRNAs), endogenous noncoding small RNAs of approximately 22 nucleotides, take part in the regulation of gene expression through translational repression or mRNA degradationCitation6 Emerging evidences indicate that several miRNAs execute functions in tumorigenesis and cancer progressionCitation7 Increasing researches have demonstrated that miRNAs such as miR-23b, miR-156a, miR-205, and miR-141 are dysregulated in NPC and contribute to the development of NPCCitation8-11 Although miR-9–3p is scarcely studied in NPC, several studies did find that it was downregulated and acted as a tumor suppressor in hepatocellular cancer and breast cancerCitation12,13 In our study, aberrant expression of miR-9–3p in NPC tissue was found and the potential effects were explored.
Epithelial-to-mesenchymal transition (EMT), an essential step in tumor progression including NPC, promotes the cancer cells to remove from the primary tumor and make distant metastasesCitation14 EMT process was usually associated with the loss of apical-basal cell polarity as well as cell–cell contacts, changes of cell-matrix adhesion, rearrangement of the cytoskeleton, degradation of extracellular matrix (ECM), variations of morphological, and enhancement of cell motility, which associated with downregulation of epithelial proteins such as E-cadherin and upregulation of mesenchymal proteins including vimentin, fibronectin, integrin and matrix metallopeptidaseCitation15-18 Fibronectin 1 (FN1), belonging to the ECM glycoprotein family, functions in ECM process and contributes to cellular adhesion, polarity, migration and tissue remodelingCitation19, 20 Besides, FN1 also executes its functions in infection resistances, and microvascular integrity maintenanceCitation21 High FN1 expression is associated with various human carcinomas, including prostatic,Citation22 pancreatic,Citation23 colorectal,Citation24 cervicalCitation25 cancers, glioma blastCitation26 and also NPCCitation27 As a family of heterodimeric extracellular matrix receptors, integrins act on cell-matrix adhesion, intracellular signaling and modulate cell proliferation, survival, motility and differentiationCitation28 High levels of β1 integrin (ITGB1) and α 5 integrin (ITGAV) were both required in EMT process and functionally correlated with the advanced metastases of tumors such as squamous cell carcinoma, breast cancer, hepatocellular carcinomas, melanoma and also NPCCitation13, 18,29,30 Intriguingly, overexpression of FN1, ITGB1 and ITGAV in NPC tissues was confirmed in our study, indicating the accelerated EMT process in NPC.
Given the correlations of upregulation of FN1, ITGB1, ITGAV and downregulation of miR-9–3p in NPC, we performed dual luciferase reporting assay and verified the target relationship. Furthermore, a series of in vitro assays were conducted to study the potential mechanism with respect to the anti-tumor role of miR-9–3p played in NPC cells. The evaluated EMT process and enhanced cell metastases were observed with overexpression of FN1, ITGB1 or ITGAV and attenuated with the co-transfection of miR-9–3p mimics. Collective data confirmed replenishment of miR-9–3p inhibited the metastases of NPC cells via downregulating FN1, ITGB1, ITGAV and inactivating EMT process, which provided novel targets for the clinical treatment of NPC.
Material and method
Tissue samples
Thirty paired NPC tissues and adjacent non-tumorous tissues were achieved from NPC patients without receiving preoperative chemotherapy or radiotherapy before biopsy sampling at Jining No.1 People's Hospital from February 2014 to February 2016. The samples were identified by pathology experts. Tissue samples were stored at -80°C. This research was approved and consented by the ethics committee and all the patients.
Cell culture
The human immortalized nasopharyngeal epithelial NP69 cell line and human NPC cell lines CNE-1, CNE-2 and HONE-1 (ATCC) were selected for this study and cultured in 10% FBS RPMI-1640 at a 37°C humidified circumstances with 5% CO2.
Microarray analysis and quantitative real time pcr (RT-qPCR)
Six samples of NPC tissues and 3 samples of adjacent tissues were selected randomly for further analysis. As described previouslyCitation31 Total RNA was extracted from frozen NPC tissues or cells by using RNA extraction Kit (Invitrogen, CA, USA). The Quant-iT RNA Assay Kit (Molecular Probes, Eugene, OR, USA) was used to detect the RNA concentration. Taqman Human MicroRNA Arrays V2.0 (Life Technology, Waltham, MA, USA) was used to measure the expression of microRNAs and U6 was regarded as internal control. For gene expression, the RNA was hybridized to Human Exon 1.0 ST Arrays (Affymetrix, Santa Clara, CA, USA) and analyzed as described previouslyCitation32
For RT-qPCR analysis, total RNAs extracted from frozen NPC tissues or cellsusing RNA extraction Kit (Invitrogen) were reverse-transcribed to cDNAs using RT Kit (Takara, Tokyo, Japan). The cDNA template was amplified using RT-qPCR kit (Qiagen, Venlo, Netherlands). The RT-qPCR experiment was conducted using ABI7500 quantitative PCR system (Applied Biosystems, Waltham, MA, USA), and the primers were showed in . U6 and GAPDH were served as the internal controls, and data were handled by themethod.
Table 1. The sequences of the primers used in qPCR.
Cell transfection
MiR-9–3p mimics, inhibitor, FN1 cDNA, ITGB1 cDNA, ITGAV cDNA and relative negative controls were all synthesized by Shanghai GenePharma Co. Ltd. FN1 cDNA, ITGB1 cDNA, ITGAV cDNA and relative negative controls were inserted into pCDNA3.1 before transfection and confirmed with sanger sequencing. MiR mimics, inhibitor, control sequence or plasmids were transfected into CNE-2 and hone-1 cells using Lepofectamine 2000 (Invitrogen) in accordance with the manufacturer's instrutions.
Western blot
Forty-eight hours after transfection, total proteins extracted by protein lysis solution were separated through SDS-PAGE, and transferred to PVDF membranes. Subsequently, the membrane was blocked for 1.5 h by 5% skim milk, and primary antibodies for FN1, ITGB1, ITGAV, E-cadherin, Vimentin, MMP1, MMP2, MMP9, COL IV or GAPDH (Abcam, Cambridge, MA, USA) were added to the membrane. After cultured overnight in 4°C, HPR-conjugated secondary antibodies (Abcam, Cambridge, MA, USA) were added. After incubated for 1 h, ECL reagents (Beyotime, Shanghai, China) were used to developing the film, and ImageJ software was used to calculate the gray value of each protein bands. GAPDH was served as an internal control.
Immunohistochemistry (IHC)
The 30 paired tissue sections of NPC were cut from paraffin-embedded blocks at 4-μm thickness. The slides were then deparaffinized, rehydrated, and heated at 95°C for retrieval of antigen epitopes in a 10 mM citrate buffer (pH 6) for 7 min. After quenching of endogenous peroxidase activity with 4% H2O2 and blocking with normal goat serum, the slides were incubated for 1 h with primary monoclonal antibodies targeting E-cadherin, vimentin, FN1, ITGB1 and ITGAV (Abcam, Cambridge, MA, USA) at 37°C. After washing, the slides were incubated with the secondary antibody (Abcam) for 30 min, developed with 3,3-diaminobenzidine for 5 min and then counterstained with hematoxylin. A mammary invasive ductal carcinoma known to express E-cadherin, FN1, ITGB1 and ITGAV was used for positive control. PBS was used to replace primary antibody as a negative control.
MTT assay
Forty-eight hours after transfection, cells (1 × 103 cells/well) were inoculated into a 96-well plate and 50μL MTT (Beyotime) per well was added. After the incubation of 2 h, 100μL DMSO were added into cells to dissolve the MTT. Cell viability was detected at 0, 24, 48 and 72 h. The OD value was assessed on a microplate reader at 570 nm.
Wound scratch assay
After incubated for 24 h, a 200μL sterile pipette was used to scratch the cell layers of each group. Then photographs were taken at 0 and 24 h, respectively. The intersection of the bottom line and the cell scratch line was considered as the observation point.
Transwell assay
Cells (105/well) were plated into the upper transwell chambers and 10% FBS DMEM was added to the lower chambers. After the incubation of 24 h, the membrane was fixed by 4% paraformaldehyde and stained by 0.1% crystal violet. Finally, the number of cells perforated across the membranes in 6 randomly selected different fields was counted.
Luciferase reporter assay
The 3′-UTR sequence of FN1 wild type, FN1 mut1-mut5, ITGB1 wild type, ITGB1 mut1-mut4, ITGAV wild type and TIGAV mut were synthesized by Shanghai GenePharma and cloned into pMIR-reporter plasmid (Promega, Madison, WI, USA). The wild type or the mutated type of 3′-UTRs were co-transfected into HEK 293T cells with miR-9–3p mimics or mimic controls using Lepofectamine 2000 (Invitrogen) in accordance with the manufacturer's instruction. After the transfection for 48 hours, the relative luciferase activity was detected using the luciferase reporter system (Promega).
Statistical analysis
SPSS 21.0 and GraphPad prism 6.0 were used to conduct statistical analyses. Measurement data recorded as the mean ± standard. The comparison between any 2 groups was analyzed using t test and that among more than 2 groups was analyzed using one-way ANOVA. P<0.05 means statistically significant.
Results
MiR-9–3p is downregulated in NPC tissues and correlates with accelerated EMT process
Microarray assay facilitated our research on miRNA and mRNA expression signatures in NPC (, ). Six NPC tissues and 3 adjacent normal tissues were analyzed. A total of 47 miRNAs and 243 mRNAs were aberrantly expressed in NPC tissues. To determine the functions of miRNAs, interactions between miRNAs and their target mRNAs were theoretically predicted by seed-matching sequence according to Targetscan database. Potentially targeting and suppressing multiple mRNAs such as FN1, ITGB1 and TIGAV, low level of miR-9–3p caused our attention and was selected for further research. Detected by RT-qPCR, miR-9–3p was downregulated while FN1, ITGB1 and ITGAV were significantly upregulated in BPC tissues (, P<0.05). Additionally, the expression of miR-9–3p was negatively correlated with FN1, ITGB1 and ITGAV expression in NPC tissues (, P<0.05). Given that FN1, ITGB1 and ITGAV were all associated with EMT process, western blot and IHC were performed to further study to measure the related protein level (, ). Obviously, upregulated protein level of Vimentin, FN1, ITGB1, ITGAV, MMP2 and downregulated level of E-cadherin and COL IV were confirmed in NPC tissues compared with the normal, suggesting an evaluated EMT process in NPC which would strengthen the metastases of NPC cells.
Figure 1. Expression patterns of miR-9–3p, FN1, ITGB1 and ITGAV. (A-B) Hierarchical clustering analysis of gene(A) and miRNA(B) that were differentially expressed among 6 NPC tissues and 3 adjacent tissues were shown (great than 2-fold difference in expression; P < 0.05). Expression values are represented in different colors. (C) RT-qPCR was performed to detect the expression of miR-9–3p, FN1 mRNA, ITGB1 mRNA and ITGAV mRNA. (D) The linear correlation among miR-3p, FN1 mRNA, ITGB1 mRNA and ITGAV mRNA was analyzed and negative correlations were shown.
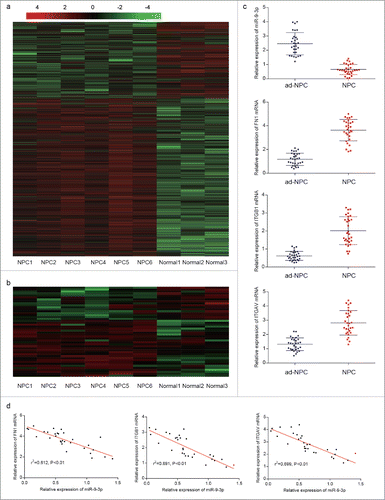
Figure 2. Expression of FN1,ITGB1, ITGAV and related protein expression in NPC tissues and adjacent tissues. (A) Immunohistochemistry was performed and one random paired tissue was shown. More positive area indicates high level of relative protein. (B) The protein level of FN1, ITGB1, ITGAV, E-cadherin, Vimentin, MMP2 and COL IV in NPC tissues and adjacent tissues were measured by western blot. Evaluated EMT process in NPC tissues was confirmed as upregulation of Vimentin and MMP2 and downregulation of E-cadherin and COL IV in adjacent tissues.
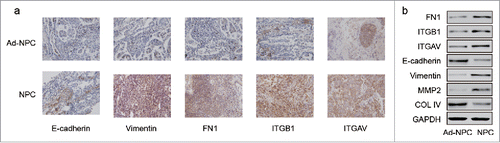
Replenishing of miR-9–3p suppresses the proliferation and metastases of NPC cells
Downregulation of miR-9–3p as well as upregulation of FN1, ITGB1 and ITGAV was verified in NPC cells lines including CNE-1, CNE-2 and HONE-1 compared with human immortalized nasopharyngeal epithelial NP69 cells (, P<0.05). To explore the role of miR-9–3p in NPC, we transfected miR-9–3p mimics (as mimics group) and control oligo (as NC group) into CNE-1, CNE-2 and HONE-1 cells respectively. RT-qPCR detecting miR-9–3p was first used to confirm the transfection efficiency (). Afterwards, MTT, wound scratch and transwell assay were performed and significantly attenuated proliferation, migration and invasion in mimics groups compared with relative NC groups were observed (). Measured with RT-qPCR and western blot, the expression of FN1, ITGB1 and ITGAV was suppressed in mimics groups together with Vimentin, MMP2 while E-cadherin and COL IV were upregulated (), indicating the anti-tumor effect of miR-9–3p via inhibiting the EMT process.
Figure 3. The anti-role of miR-9–3p in NPC tissues. (A) The expression of miR-9–3p, FN1, ITGB1 and ITGAV in NPC cells was measured by RT-qPCR. ** represents P<0.05 compared with NP69 cells. (B) Forty-eight hours after transfection, expression of miR-9–3p in CNE-1, CNE-2 and CNE-3 was detected by RT-qPCR. (C) MTT assay assessed the proliferation ability of transfected cells. (D-E) The migration ability of transfected cells was compared with wound scratch assay. (F-G) Transwell assay was used to examine the invasion ability of transfected cells and photo at 100 × magnification were taken. All data was presented as Mean ± SD from 3 independent experiments. **, ## and and& represents P < 0.05 compared with relative control group.
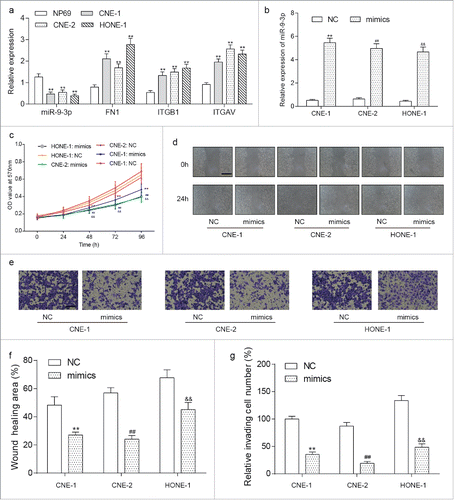
Figure 4. The downstream of miR-9–3p in NPC cells. (A-C) Measured by RT-qPCR, overexpression of miR-9–3p efficiently downregulated the The expression of FN1(A), ITGB1(B) and ITGAV(C) in transfected NPC cells. (D) Western blot analysis valued the protein expression of FN1, ITGB1, ITGAN, E-cadherin, Vimentin, MMP2 and COL IV, indicating an inactivated EMT process induced by upregulation of miR-9–3p. All data was presented as Mean ± SD from 3 independent experiments. **, ## and and& represents P < 0.05 compared with relative control group.
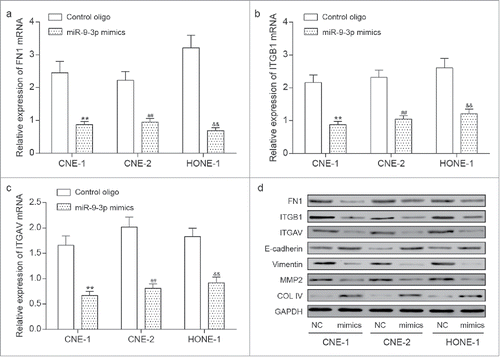
FN1, ITGB1 and ITGV5 are all targets of miR-9–3p
Predicted by Targetscan, the binding regions among miR-9–3p, FN1, ITGB1 and ITGV5 undergoes site directed mutagenesis. Given 4 target sites between miR-9–3p and FN1, 3 sites between miR-9–3p and ITGB1 and one site between ITGV5 were shown in Targetscan, we designed sequences with multiple or single site mutation for FN1, ITGB1 and ITGAV. These sequences were synthesized by Shanghai GenePharma, and cloned into pMIR reporter plasmid as shown in . The luciferase construct harboring those sequences were used for the transiently co-transfection with miR-9–3p in HEK 293T cells. FN1 mut1, FN1 mut2, FN1 mut4, FN1 wt, ITGB1 mut1, ITGB1 mut2, ITGB1 mut3, ITGB1 wt and ITGAV wt were all able to reduce the relative luciferases compared with the relative controls (). All these verified the target relationship among miR-9–3p, FN1, ITGB1 and ITGAV, which also explains the downregulation of FN1, ITGB1 and ITGV5 by miR-9–3p mimics at transcriptional level in NPC cells.
Figure 5. The target relationship among miR-9–3p, FN1, ITGB1 and ITGAV. (A) The reporting plasmids carrying wild type or mutant FN1, ITGB1 and ITGAV were designed according the predicted target sites provided by TargetScan database. Sequences under black lines were delated in mutant cDNAs. (B-D) Transient co-transfections were performed in HEK 293T cells with one of the plasmid and either miR-9–3p mimics or control oligo. The reduction on relative luciferase activity in wild type or poorly conserved sequence compared with the all-site-mutant group confirmed the target relationship among miR-9–3p, FN1, ITGB1 and ITGAV. All data was presented as Mean ± SD from 3 independent experiments. **, ## and and& represents P < 0.05 compared with relative control group.
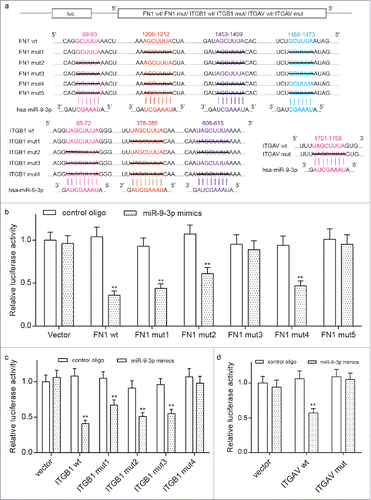
MiR-9–3p inhibits the EMT process by downregulating FN1, ITGB1 and ITGAV in HONE-1 cells
Plasmids overexpressing FN1, ITGB1 or ITGAV were constructed as well as a control vector. Co-transfection of HONE-1 cells was performed with one of plasmids and either miR-9–3p mimics or control oligo. Forty-eight hours after transfection, stably transfected cells were harvested and divided to 8 groups for further research: vector + oligo, vector + mimics, FN1 + oligo, FN1 + mimics, ITGB1 + oligo, ITGB1 + mimics, ITGAV + oligo and ITGAV + mimics. RT-qPCR results () confirmed an evaluated expression of miR-9–3p induced by mimics could not be affected by vector, FN1, ITGB1 or ITGAV. Moreover, expression of FN1, ITGB1, ITGAV was significantly enhanced in FN1 + oligo, ITGB1 + oligo and ITGAV + oligo groups respectively, which was abrogated by miR-9–3p mimics in FN1 + mimics, ITGB1 + mimics and ITGAV +mimics groups. Additionally, the transfection of FN1, ITGB1 or ITGAV has little effect on each other's expression at transcriptional level.
Figure 6. The anti-tumor role of miR-9–3p was via down-regulating FN1, ITGB1 and ITGAV. (A-D) Stable co-transfections with one of the plasmids carrying no sequence, FN1 cDNA, ITGB1 cDNA or ITGAV cDNA and either miR-9–3p mimics or control oligo were performed in HONE-1 cells. Forty-eight hours after transfection, expression of miR-9–3p (A), FN1 (B), ITGB1 (C) and ITGAV (D) was detected by RT-qPCR. (E) Proliferation ability of transfected cells was assessed using MTT assay. (F-G) Migration and invasion ability of transfected cells was measured by wound scratch and transwell assay respectively. All data was presented as Mean ± SD from 3 independent experiments. **represents P < 0.05 compared with vector + oligo group while ## represents P < 0.05 compared with relative control group.
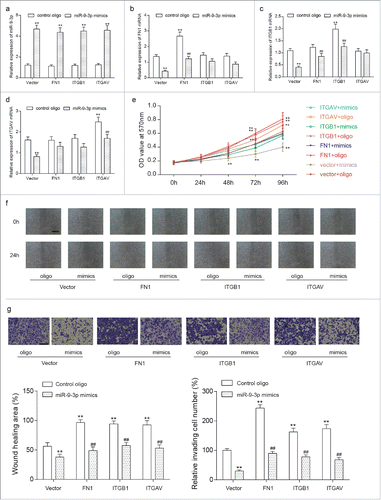
The proliferation, migration and invasion of cells were studied by the means of MTT, wound scratch and transwell assay. The results shown in , and demonstrated the enhanced proliferation and metastases of HONE-1 cells induced by the overexpression of FN1, ITGB1 or ITGAV. Still, co-transfection with miR mimics turned down the promoting effects and FN1 + mimics, ITGB1 + mimics and ITGAV + mimics showed the similar trends with vector + oligo group. The variation on EMT process related proteins were measured by western blot (), overexpression of FN1 mRNA, ITGB1 mRNA or ITGAV mRNA lead to an evaluated translational level respectively compared with vector + oligo group. Intriguingly, high level of FN1 contributed to the upregulation of ITGB1 and ITGAV level while the reverse relationship was not found. The level of E-cadherin and COL IV decreased and the level of Vimentin, MMP2 increased in FN1 + oligo, ITGB1 + oligo and ITGAV + oligo groups compared with vector + oligo group. Again, co-transfection with miR-9–3p mimics downregulated the evaluated protein level of FN1, ITGB1, ITGAV, Vimentin, MMP2 and upregulated the levels of E-cadherin and COL IV in FN1 + mimics, ITGB1 + mimics and ITGAV + mimics compared with FN1 + oligo, ITGB1 + oligo and ITGAV + oligo groups respectively. Taken together, miR-9–3p functions as an anti-tumor role via suppressing the expressions of FN1, ITGB1 and ITGAV.
Figure 7. The protein levels of transfected cells were detected with western blot. Upregulation of FN1, ITGB1 or ITGAV improved the expression of Vimentin, MMP2, downregulated the level of E-cadherin and COL IV and also facilitated the EMT process. Simultaneous overexpression reduced the FN1, ITGB1, ITGAV levels and abrogated the promoting effect of FN1, ITGB1 or ITGAV on EMT process.
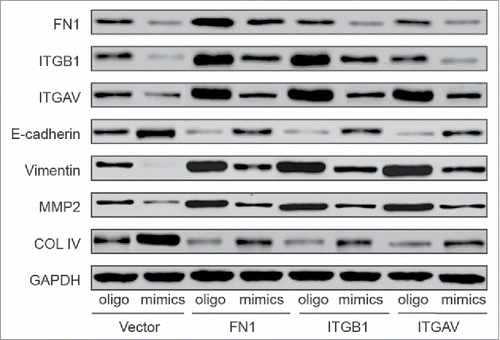
Discussion
As an uncommon epithelial cancer worldwide, NPC has high rate of occurrence and subsequence metastases especially in Southeast Asia33For the treatments of NPC, adjuvant radiotherapy or chemotherapy is often applied with drugs or surgery, whereas the development of radio-resistance or chemo-resistance remains a major limitationCitation34,35 Therefore, it is required to figure out the molecular mechanisms involved in the tumorigenesis and progression of NPC with the aim of acquiring molecular targets for the effective prevention and treatment of NPC.
It is well known that EMT is one of the most important manners contributing to cancer metastasesCitation36 The process involves tumor cell detachment and regulates the expression of adhesion molecules between cells and extracellular matrix such as E-cadherin, Vimentin, fibronectin, integrin, matrix metalloproteinase and collagenCitation37-39 Increasing evidences reveals that upregulation of FN1, ITGB1 and ITGAV facilitated the EMT process and accelerated the tumor metastasesCitation18,40,41 Moreover, these molecules also participate in various cellular procedures involving cell growth, differentiation and apoptosisCitation42,43 In our study, strengthened EMT process was characterized in NPC tissues by the upregulation of Vimentin, FN1, ITGB1, ITGAV, MMP2 and downregulation of E-cadherin and COL IV. In vitro experiments also demonstrated the tumor-promoting role of FN1, ITGB1 and ITGAV on proliferation, migration and invasion of HONE-1 cells as well as evaluated EMT process.
MiRNAs are reported as crucial regulators of physiologic processes involving neurogenesis, hematopoiesis, regeneration and homeostasis of stem cellsCitation44 Furthermore, miRNAs also contributed to the metastasis of numerous human carcinomas by transcriptional regulation of related genesCitation45-47 For example, MiR-105 promotes breast cancer cell metastases by targeting the junction protein ZO-145 MiR-374a directly targets CCND1 to inactivate PI3K/pAKT/c-JUN forming a negative back loop, subsequently suppresses downstream signals related to cell cycle progression and EMT process, finally contributes to the inhibition of NPC cell proliferation, invasion, metastasis and cisplatin resistance in vitro and in vivoCitation48 In this study, the expression of miR-9–3p was shown to be obviously downregulated in NPC tumor tissues in comparison with adjacent normal tissues. Replenishment of miR-9–3p suppressed proliferation, migration and invasion of NPC cells, indicating the anti-tumor role of miR-9–3p. Targeting ITGB1, miR-9–3p enhanced the AZD6244 (MEK1/2 inhibitor) induced extracellular signal regulated kinase inhibition and growth arrest in breast cancerCitation13 And in hepatocellular carcinoma, miR-9–3p targeted TAZ and inhibited cell proliferative ability via suppressing phosphorylations of ERK1/2 and AKTCitation12 To a degree, these studies gave support for our findings about the tumor-suppressing effect of miR-9–3p in NPC.
To further explore the potential mechanism of miR-9–3p, the expressions of miR-9–3p, FN1, ITGB1, and ITGAV in NPC tissues were first analyzed and a negative correlation was uncovered. According to the potential target sites provided by targetscan, we performed dual luciferase assay and confirmed the direct relationship among miR-9–3p, FN1, ITGB1, and ITGAV. By manipulating the expression of miR-9–3p, FN1, ITGB1 and ITGAV in NPC cells, we gave evidence that miR-9–3p suppressed the mRNA and protein level of FN1, ITGB1 and ITGAV. Additionally, the tumor-promoting effects of FN1, ITGB1 and ITGAV were efficiently attenuated by co-overexpression of miR-9–3p.
In summary, we first uncovered the anti-tumor role of miR-9–3p including suppressing cell proliferation, migration and invasion via downregulating FN1, ITGB1, ITGAV and inactivating EMT process. With multiple targets involving with the progression of NPC, miR-9–3p is of considerate interest to serve as a novel target in the clinic treatment of NPC.
Disclosure of potential conflicts of interest
No potential conflicts of interest were disclosed.
References
- Cao SM, Simons MJ, Qian CN. The prevalence and prevention of nasopharyngeal carcinoma in China. Chin J Cancer 2011; 30(2):114-9; PMID:21272443; https://doi.org/10.5732/cjc.010.10377
- Hildesheim A, Wang CP. Genetic predisposition factors and nasopharyngeal carcinoma risk: a review of epidemiological association studies, 2000–2011: Rosetta Stone for NPC: genetics, viral infection, and other environmental factors. Semin Cancer Biol 2012; 22(2):107-16; PMID:22300735; https://doi.org/10.1016/j.semcancer.2012.01.007
- Wu F, Wang R, Lu H, Wei B, Feng G, Li G, Liu M, Yan H, Zhu J, Zhang Y, et al. Concurrent chemoradiotherapy in locoregionally advanced nasopharyngeal carcinoma: treatment outcomes of a prospective, multicentric clinical study. Radiother Oncol 2014; 112(1):106-11; PMID:24933452; https://doi.org/10.1016/j.radonc.2014.05.005
- Sun X, Su S, Chen C, Han F, Zhao C, Xiao W, Deng X, Huang S, Lin C, Lu T. Long-term outcomes of intensity-modulated radiotherapy for 868 patients with nasopharyngeal carcinoma: an analysis of survival and treatment toxicities. Radiother Oncol 2014; 110(3):398-403; PMID:24231245; https://doi.org/10.1016/j.radonc.2013.10.020
- Bensouda Y, Kaikani W, Ahbeddou N, Rahhali R, Jabri M, Mrabti H, Boussen H, Errihani H. Treatment for metastatic nasopharyngeal carcinoma. Eur Ann Otorhinolaryngol Head Neck Dis 2011; 128(2):79-85; PMID:21177151; https://doi.org/10.1016/j.anorl.2010.10.003
- Shukla GC, Singh J, Barik S. MicroRNAs: Processing, Maturation, Target Recognition and Regulatory Functions. Mol Cell Pharmacol 2011; 3(3):83-92; PMID:22468167; https://doi.org/10.4255/mcpharmacol.11.13
- Nana-Sinkam SP, Croce CM. MicroRNA regulation of tumorigenesis, cancer progression and interpatient heterogeneity: towards clinical use. Genome Biol 2014; 15(9):445; PMID:25315999; https://doi.org/10.1186/s13059-014-0445-8
- Wang JY, Li XF, Li PZ, Zhang X, Xu Y, Jin X. MicroRNA-23b regulates nasopharyngeal carcinoma cell proliferation and metastasis by targeting E-cadherin. Mol Med Rep 2016; 14(1):537-43; PMID:27150436; https://doi.org/10.3892/mmr.2016.5206
- Tian Y, Cai L, Tu Y, Qiu H, Xie G, Huang D, Zheng R, Zhang W. miR156a Mimic Represses the Epithelial-Mesenchymal Transition of Human Nasopharyngeal Cancer Cells by Targeting Junctional Adhesion Molecule A. PLoS One 2016; 11(6):e0157686; PMID:27341697; https://doi.org/10.1371/journal.pone.0157686
- Mao Y, Wu S, Zhao R, Deng Q. MiR-205 promotes proliferation, migration and invasion of nasopharyngeal carcinoma cells by activation of AKT signalling. J Int Med Res 2016; 44(2):231-40; PMID:26880795; https://doi.org/10.1177/0300060515576556
- Liu Y, Zhao R, Wang H, Luo Y, Wang X, Niu W, Zhou Y, Wen Q, Fan S, Li X, et al. miR-141 is involved in BRD7-mediated cell proliferation and tumor formation through suppression of the PTEN/AKT pathway in nasopharyngeal carcinoma. Cell Death Dis 2016; 7:e2156; PMID:27010857; https://doi.org/10.1038/cddis.2016.64
- Higashi T, Hayashi H, Ishimoto T, Takeyama H, Kaida T, Arima K, Taki K, Sakamoto K, Kuroki H, Okabe H, et al. miR-9-3p plays a tumour-suppressor role by targeting TAZ (WWTR1) in hepatocellular carcinoma cells. Br J Cancer 2015; 113(2):252-8; PMID:26125451; https://doi.org/10.1038/bjc.2015.170
- Zawistowski JS, Nakamura K, Parker JS, Granger DA, Golitz BT, Johnson GL. MicroRNA 9-3p targets beta1 integrin to sensitize claudin-low breast cancer cells to MEK inhibition. Mol Cell Biol 2013; 33(11):2260-74; PMID:23530058; https://doi.org/10.1128/MCB.00269-13
- Zhao L, Lin L, Pan C, Shi M, Liao Y, Bin J, Liao W. Flotillin-2 promotes nasopharyngeal carcinoma metastasis and is necessary for the epithelial-mesenchymal transition induced by transforming growth factor-beta. Oncotarget 2015; 6(12):9781-93; PMID:25909165; https://doi.org/10.18632/oncotarget.3382
- Thiery JP, Sleeman JP. Complex networks orchestrate epithelial-mesenchymal transitions. Nat Rev Mol Cell Biol 2006; 7(2):131-42; PMID:16493418; https://doi.org/10.1038/nrm1835
- Zeisberg M, Neilson EG. Biomarkers for epithelial-mesenchymal transitions. J Clin Invest 2009; 119(6):1429-37; PMID:19487819; https://doi.org/10.1172/JCI36183
- Luo WR, Chen XY, Li SY, Wu AB, Yao KT. Neoplastic spindle cells in nasopharyngeal carcinoma show features of epithelial-mesenchymal transition. Histopathology 2012; 61(1):113-22; PMID:22486228; https://doi.org/10.1111/j.1365-2559.2012.04205.x
- Wasil LR, Shair KH. Epstein-Barr virus LMP1 induces focal adhesions and epithelial cell migration through effects on integrin-alpha5 and N-cadherin. Oncogenesis 2015; 4:e171; PMID:26479443; https://doi.org/10.1038/oncsis.2015.31
- Ma LJ, Lee SW, Lin LC, Chen TJ, Chang IW, Hsu HP, Chang KY, Huang HY, Li CF. Fibronectin overexpression is associated with latent membrane protein 1 expression and has independent prognostic value for nasopharyngeal carcinoma. Tumour Biol 2014; 35(2):1703-12; PMID:24081675; https://doi.org/10.1007/s13277-013-1235-8
- Hanahan D, Weinberg RA. Hallmarks of cancer: the next generation. Cell 2011; 144(5):646-74; PMID:21376230; https://doi.org/10.1016/j.cell.2011.02.013
- Mammoto A, Connor KM, Mammoto T, Yung CW, Huh D, Aderman CM, Mostoslavsky G, Smith LE, Ingber DE. A mechanosensitive transcriptional mechanism that controls angiogenesis. Nature 2009; 457(7233):1103-8; PMID:19242469; https://doi.org/10.1038/nature07765
- Ifon ET, Pang AL, Johnson W, Cashman K, Zimmerman S, Muralidhar S, Chan WY, Casey J, Rosenthal LJ. U94 alters FN1 and ANGPTL4 gene expression and inhibits tumorigenesis of prostate cancer cell line PC3. Cancer Cell Int 2005; 5:19; PMID:15972109; https://doi.org/10.1186/1475-2867-5-19
- Schwarz RE, Awasthi N, Konduri S, Caldwell L, Cafasso D, Schwarz MA. Antitumor effects of EMAP II against pancreatic cancer through inhibition of fibronectin-dependent proliferation. Cancer Biol Ther 2010; 9(8):632-9; PMID:20212356; https://dx.doi.org/10.4161/cbt.9.8.11265
- Ou J, Li J, Pan F, Xie G, Zhou Q, Huang H, Liang H. Endostatin suppresses colorectal tumor-induced lymphangiogenesis by inhibiting expression of fibronectin extra domain A and integrin alpha9. J Cell Biochem 2011; 112(8):2106-14; PMID:21465533; https://doi.org/10.1002/jcb.23130
- Yousif NG. Fibronectin promotes migration and invasion of ovarian cancer cells through up-regulation of FAK-PI3K/Akt pathway. Cell Biol Int 2014; 38(1):85-91; PMID:24115647; https://doi.org/10.1002/cbin.10184
- Lin ZX, Yang LJ, Huang Q, Fu J. Activated vascular endothelia regulate invasion of glioma cells through expression of fibronectin. Chin Med J (Engl) 2010; 123(13):1754-61; PMID:20819642; https://doi.org/10.3760/cma.j.issn.0366-6999.2010.13.026
- Kumar V, Gupta T, Jain S, Chanana B. Posterior sub-Tenon's triamcinolone in choroidal granuloma due to probable ocular sarcoidosis. Oman J Ophthalmol 2013; 6(2):127-8; PMID:24082675; https://doi.org/10.4103/0974-620X.116660
- Maschler S, Wirl G, Spring H, Bredow DV, Sordat I, Beug H, Reichmann E. Tumor cell invasiveness correlates with changes in integrin expression and localization. Oncogene 2005; 24(12):2032-41; PMID:15688013; https://doi.org/10.1038/sj.onc.1208423
- Jiang X, Wang J, Zhang K, Tang S, Ren C, Chen Y. The role of CD29-ILK-Akt signaling-mediated epithelial-mesenchymal transition of liver epithelial cells and chemoresistance and radioresistance in hepatocellular carcinoma cells. Med Oncol 2015; 32(5):141; PMID:25805567; https://doi.org/10.1007/s12032-015-0595-x
- Geng S, Guo Y, Wang Q, Li L, Wang J. Cancer stem-like cells enriched with CD29 and CD44 markers exhibit molecular characteristics with epithelial-mesenchymal transition in squamous cell carcinoma. Arch Dermatol Res 2013; 305(1):35-47; PMID:22740085; https://doi.org/10.1007/s00403-012-1260-2
- Navarro A, Pairet S, Alvarez-Larran A, Pons A, Ferrer G, Longaron R, Fernandez-Rodriguez C, Camacho L, Monzo M, Besses C, et al. miR-203 and miR-221 regulate SOCS1 and SOCS3 in essential thrombocythemia. Blood Cancer J 2016; 6:e406; PMID:26990535; https://doi.org/10.1038/bcj.2016.10
- Villadsen SB, Bramsen JB, Ostenfeld MS, Wiklund ED, Fristrup N, Gao S, Hansen TB, Jensen TI, Borre M, Orntoft TF, et al. The miR-143/-145 cluster regulates plasminogen activator inhibitor-1 in bladder cancer. Br J Cancer 2012; 106(2):366-74; PMID:22108519; https://doi.org/10.1038/bjc.2011.520
- Yu WM, Hussain SS. Incidence of nasopharyngeal carcinoma in Chinese immigrants, compared with Chinese in China and South East Asia: review. J Laryngol Otol 2009; 123(10):1067-74; PMID:19486543; https://doi.org/10.1017/S0022215109005623
- Zhang L, Zhao C, Ghimire B, Hong MH, Liu Q, Zhang Y, Guo Y, Huang YJ, Guan ZZ. The role of concurrent chemoradiotherapy in the treatment of locoregionally advanced nasopharyngeal carcinoma among endemic population: a meta-analysis of the phase III randomized trials. BMC Cancer 2010; 10:558; PMID:20950416; https://doi.org/10.1186/1471-2407-10-558
- Xiao WW, Huang SM, Han F, Wu SX, Lu LX, Lin CG, Deng XW, Lu TX, Cui NJ, Zhao C. Local control, survival, and late toxicities of locally advanced nasopharyngeal carcinoma treated by simultaneous modulated accelerated radiotherapy combined with cisplatin concurrent chemotherapy: long-term results of a phase 2 study. Cancer 2011; 117(9):1874-83; PMID:21509764; https://doi.org/10.1002/cncr.25754
- Yang J, Weinberg RA. Epithelial-mesenchymal transition: at the crossroads of development and tumor metastasis. Dev Cell 2008; 14(6):818-29; PMID:18539112; https://doi.org/10.1016/j.devcel.2008.05.009
- Falzone L, Candido S, Salemi R, Basile MS, Scalisi A, McCubrey JA, Torino F, Signorelli SS, Montella M, Libra M. Computational identification of microRNAs associated to both epithelial to mesenchymal transition and NGAL/MMP-9 pathways in bladder cancer. Oncotarget 2016; 7(45):72758-66; PMID:27602581; https://doi.org/10.18632/oncotarget.11805
- Jia D, Entersz I, Butler C, Foty RA. Fibronectin matrix-mediated cohesion suppresses invasion of prostate cancer cells. BMC Cancer 2012; 12:94; PMID:22433434; https://doi.org/10.1186/1471-2407-12-94
- Nair KS, Naidoo R, Chetty R. Expression of cell adhesion molecules in oesophageal carcinoma and its prognostic value. J Clin Pathol 2005; 58(4):343-51; PMID:15790695; https://doi.org/10.1136/jcp.2004.018036
- Yang J, Hou Y, Zhou M, Wen S, Zhou J, Xu L, Tang X, Du YE, Hu P, Liu M. Twist induces epithelial-mesenchymal transition and cell motility in breast cancer via ITGB1-FAK/ILK signaling axis and its associated downstream network. Int J Biochem Cell Biol 2016; 71:62-71; PMID:26693891; https://doi.org/10.1016/j.biocel.2015.12.004
- Morita Y, Hata K, Nakanishi M, Omata T, Morita N, Yura Y, Nishimura R, Yoneda T. Cellular fibronectin 1 promotes VEGF-C expression, lymphangiogenesis and lymph node metastasis associated with human oral squamous cell carcinoma. Clin Exp Metastasis 2015; 32(7):739-53; PMID:26319373; https://doi.org/10.1007/s10585-015-9741-2
- Yi W, Xiao E, Ding R, Luo P, Yang Y. High expression of fibronectin is associated with poor prognosis, cell proliferation and malignancy via the NF-kappaB/p53-apoptosis signaling pathway in colorectal cancer. Oncol Rep 2016; 36(6):3145-53; PMID:27748871; https://doi.org/10.3892/or.2016.5177
- Morandi EM, Verstappen R, Zwierzina ME, Geley S, Pierer G, Ploner C. ITGAV and ITGA5 diversely regulate proliferation and adipogenic differentiation of human adipose derived stem cells. Sci Rep 2016; 6:28889; PMID:27363302; https://doi.org/10.1038/srep28889
- Zhao Y, Srivastava D. A developmental view of microRNA function. Trends Biochem Sci 2007; 32(4):189-97; PMID:17350266; https://doi.org/10.1016/j.tibs.2007.02.006
- Zhou W, Fong MY, Min Y, Somlo G, Liu L, Palomares MR, Yu Y, Chow A, O'Connor ST, Chin AR, et al. Cancer-secreted miR-105 destroys vascular endothelial barriers to promote metastasis. Cancer Cell 2014; 25(4):501-15; PMID:24735924; https://doi.org/10.1016/j.ccr.2014.03.007
- Liu C, Kelnar K, Liu B, Chen X, Calhoun-Davis T, Li H, Patrawala L, Yan H, Jeter C, Honorio S, et al. The microRNA miR-34a inhibits prostate cancer stem cells and metastasis by directly repressing CD44. Nat Med 2011; 17(2):211-5; PMID:21240262; https://doi.org/10.1038/nm.2284
- Valastyan S, Weinberg RA. miR-31: a crucial overseer of tumor metastasis and other emerging roles. Cell Cycle 2010; 9(11):2124-9; PMID:20505365; https://doi.org/10.4161/cc.9.11.11843
- Zhen Y, Fang W, Zhao M, Luo R, Liu Y, Fu Q, Chen Y, Cheng C, Zhang Y, Liu Z. miR-374a-CCND1-pPI3K/AKT-c-JUN feedback loop modulated by PDCD4 suppresses cell growth, metastasis, and sensitizes nasopharyngeal carcinoma to cisplatin. Oncogene 2017; 36(2):275-85; PMID:27270423; https://doi.org/10.1038/onc.2016.201