ABSTRACT
Triple-negative breast cancer (TNBC) is the most aggressive form of breast cancer with poor prognosis due to lack of druggable targets such as hormone and growth factor receptors. Therefore, identification of targetable regulators such as miRNAs could provide new avenues for therapeutic applications. Here, we report that the expression of miR-4417 is suppressed during the progression of TNBC cells from non-malignant to the malignant stage. MiR-4417 is localized to chromosome 1p36, a region with high frequency of loss of heterozygosity in multiple cancers, and its biogenesis is DICER-dependent. Low expression of miR-4417 is significantly associated with worse prognosis in TNBC patients, while overexpression of miR-4417 is sufficient to inhibit migration and mammosphere formation of TNBC cells in vitro. Overall, our findings suggest miR-4417 exerts a tumor suppressive effect and thereby could serve as a prognostic biomarker and therapeutic tool against TNBC.
Introduction
Breast cancer is the most lethal non-smoking related cancer amongst women in the United States, with more than 40,000 deaths estimated to occur in 2018 .Citation1 Triple-negative breast cancer (TNBC), which by definition lacks the expression of estrogen receptor (ER), progesterone receptor (PR), and human epidermal growth factor receptor 2 (HER2), is resistant to therapies targeting ER and HER2.Citation2–Citation4 Accounting for 10–20% of breast cancers, TNBC is highly prevalent in young premenopausal African-American women.Citation5–Citation7 Despite 20%-30% discordance based on gene expression patterns between basal-like breast cancer (BLBC) and TNBC, due to lack of expression of ER, PR and the HER2 genes in these two definitions, both receive the same standard guide to personalized medicine.Citation8–Citation11 A minority (15%) of TNBC patients responds well to chemotherapy, however those with residual diseases are more likely to die from tumor recurrence and metastasis, partly due to the lack of effective therapies.Citation7,Citation12 Therefore, new therapeutic tools against metastatic TNBC are urgently needed to improve survival as well as to reduce the health outcome disparity between TNBC and non-TNBC.
Epithelial–mesenchymal transition (EMT) is a transcriptional program essential during embryonic development and for maintaining tissue homeostasis, where stationary cells acquire mobility to migrate and form new tissues.Citation13 It has been shown to play a pivotal role in the early stage of metastasis, where primary tumor cells hijack this program to invade local tissues and intravasate to the bloodstream, thereby initiating the progression of the disease from non-malignant to malignant stage. Moreover, EMT has been shown to confer tumor-initiating properties to tumor cells, which may contribute to resistance to therapy, recurrence, and metastasis. Importantly, incomplete eradication of residual tumor-initiating cells has been described as a major source of disease relapse.Citation14–Citation16 Hence, there is a critical need to dissect the molecular mechanisms of EMT program in TNBC cells and design targeted therapeutic strategies to prevent disease progression and recurrence.
MicroRNAs (miRNAs) are post-transcriptional regulators that inhibit the translation of one or more target mRNAs, thereby changing the proteome and exerting significant effect on cellular functions.Citation17 The mature ~22nt product of the small noncoding RNA species binds predominantly to the 3ʹUTR of their mRNA targets through a complementary seed region, sometimes leading to the degradation of the mRNA transcripts.Citation18 Due to their small size, tumor-suppressive miRNAs could potentially be delivered therapeutically to tumor cells to inhibit the expression of target oncogenes and suppress cancer progression.Citation19,Citation20
Here, we report that miR-4417 is a novel tumor-suppressive miRNA and its expression level decreases during progression of TNBC from nonmalignant to the malignant stage. miR-4417 is localized to a deletion hotspot on chromosome 1p36, which potentially harbors a tumor suppressor gene, and its expression is lost or low in mesenchymal TNBC cells compared to epithelial cells. Finally, overexpression of miR-4417 impairs migration and tumorigenicity of TNBC cells in vitro, suggesting the therapeutic benefit of miR-4417 as a tool against TNBC.
Materials and methods
Cell culture
MDA-MB-231-LM2 (LM2) and MDA-MB-231–BoM2 (BoM2) cells were a generous gift from Dr. Joan Massague. MDA-MB-231, MDAM-MB-543, UACC812, SUM149, and SUM159 cells were obtained from ATCC. MCF10A derivatives, MIII, MIV, and NeoT cells were cultured in DMEM/F12 medium supplemented with 5% Horse Serum (HS), 1% antibiotics, 10 mg/ml Insulin, 20ng/ml epidermal growth factor, 100ng/ml cholera toxin, and 500ng/ml hydrocortisone. MDA-MB-231, MDAM-MB-543, UACC812, LM2, and BoM2 cells were maintained in DMEM medium containing 10% FBS, while SUM149 and SUM159 cell lines were cultured in Ham’s F/12 containing 5% FBS, 10 mg/mL insulin, and 500 ng/mL hydrocortisone. Mammospheres were grown in MammoCult medium (Stem Cell Technologies) supplemented with 0.48 mg/mL hydrocortisone and in the presence of 1% methylcellulose. All cells were grown in the presence of 1% penicillin/streptomycin in a humidified incubator at 37°C with 5% CO2.
Kaplan-Meier survival analysis
A database of cancer patients was established as described previously.Citation21–Citation23 Survival curves were generated based on the transcript level of a candidate gene using the Cox proportional hazards regression analysis and plotting Kaplan-Meier plots as described. A p-value below 0.05 was accepted as a significant correlation between gene expression and survival.
shRNA cloning and stable gene knockdown
shRNA oligonucleotides were annealed and ligated to pLKO.1 lentiviral expression vector. The correct shRNA sequence was confirmed by sequencing. The pLKO.1 vector, psPAX2, and pMD2.G packaging vectors, and Xtreme-Gene transfection reagent (Roche) were then mixed in OptiMEM (Life Technologies) and added to HEK293 cells for 48 h. Viral medium was run through 0.45 μm filters and added to target cells for 24 h in the presence of 10 μg/ml polybrene. Cells were then fed with fresh media and selection of transduced cells was performed using 2 μg/ml of puromycin. Sequence of shRNA oligonucleotides ligated into pLKO.3G plasmid: shDICER: F: 5ʹAATTGGAAG AATCAGCCTCGCAACACTCGAGTGTTGCGAGGCTGATTCTTCCTTTTTTAT, R: 5ʹAAAAAAGGAAGAATCAGCCTCGCAACACTCGAGTGTTGCGAGGCTGATTCTTCC.
Reverse transcription-quantitative PCR (RT-qPCR)
Total RNA was isolated from cells using Trizol (Life Technologies) and Direct-zol RNA Miniprep kit (Zymo Research). For mRNA, total RNA was converted to cDNA using Super Script III Reverse Transcriptase (Life Technologies). The cDNA samples were then mixed with Power SYBR Green PCR Master Mix and qPCR primers, and run on 7900HT Real-Time PCR system to quantify expression level of target genes. Sequence of qPCR primers for target genes: SMAD2: F: 5ʹ AGAAGTCAGCTGGTGGGTCT, R: 5ʹ GAGTGGTGATGGCTTTCTCA; E-cadherin (CDH1): F: 5ʹ TTCTGCTGCTCTTG CTGTTT, R: TGGCTCAAGTCAAAGTCCTG; Vimentin: F: 5ʹ AGGTGGACCAGCTA ACCAAC, R: 5ʹ AGCATCTCCTCCTGCAATTT; β-ACTIN: F: 5ʹ CGAGCACAGAGC CTCGCCTTTGCC, R: 5ʹ TGTCGACGACGAGCGCGGCGATAT; DICER: F: 5ʹ CCG AGAGTTGCCTGATGGTA, R: 5ʹ CAGCCAATCGTACACAGCTC.
For miRNA, total RNA was first converted to cDNA using TaqMan™ MicroRNA Reverse Transcription Kit (Life Technologies). The cDNA samples were then mixed with TaqMan™ Universal PCR Master Mix II, no UNG (Life Technologies), and TaqMan™ Small RNA Assay (Life Technologies) and run on 7900HT Real-Time PCR system. RNU6 was used for normalization of the miRNA expression data.
Western blotting
Cells were lysed with RIPA buffer (Pierce) in the presence of protease and phosphatase inhibitors (Roche). Protein lysates were then scraped from the dish using cell lifters and centrifuged for 15 min at 14,000 g at 4°C. Protein supernatant was mixed with 4xLDS buffer and 10x reducing agent before being loaded onto NuPAGE 4–12% pre-cast gels and separated for 2 h at 100 V. Proteins were then transferred to a PVDF membrane overnight at 4°C. The membrane was blocked with 5% milk for 30 min at room temperature and then incubated with primary antibodies overnight at 4°C. The membrane was washed with TBS-T for 5 min three times and incubated with secondary antibodies for 1 h at room temperature. Membranes were washed with TBS-T for 5 min three times and incubated with enhanced chemiluminescence agent. Films were used for the visualization of protein bands of interest. Antibodies used were as follows: SMAD2, E-cadherin, vimentin, phosopho-p38, and total p38 from Cell Signaling Technology; ACTIN from Sigma-Aldrich.
Wound healing assay
Cells were grown to confluence and a scratch was introduced using a pipette tip. Cells were then allowed to migrate and close the wound for 20 h.
Microarray analysis
Total RNA was isolated from cells using Trizol (Life Technologies) and the miRNeasy Mini kit (Qiagen). The quality of RNA was determined using an Agilent Bioanalyzer 2100 and small RNA samples with an RNA Integrity Number (RIN) > 8 were hybridized to Affymetrix GeneChip miRNA v4.0 microarrays. Raw Affymetrix CEL files were normalized to produce probeset-level expression values for all human and control probesets using Affymetrix Expression Console (version 1.3.0.187), using the Robust Multiarray Average (RMA)Citation24 and Detection Above BackGround (DABG). Analysis was limited to the 2,578 human microRNAs interrogated by the array. Principal Component Analysis (PCA) was performed using the ‘prcomp’ R function with expression values that had been normalized across all samples to a mean of zero and a standard deviation of one. Pairwise differential microRNA expression was assessed using the moderated (empirical Bayesian) t- test implemented in the limma R package (version 3.14.4) (i.e., creating simple linear models with lmFit, followed by empirical Bayesian adjustment with eBayes). Correction for multiple hypothesis testing was accomplished using the Benjamini-Hochberg false discovery rate (FDR).Citation25 All statistical analyses were performed using the R environment for statistical computing (version 2.15.1).
Data availability
The raw and normalized miRNA microarray data can be accessed in Gene Expression Omnibus (GSE121657).
Transient transfection with miRNA mimics
MicroRNA mimics (Life Technologies) were mixed with Xtreme-Gene transfection reagent (Roche) in OptiMEM (Life Technologies) for 5 min at room temperature and then added drop-wise to target cells.
Transwell migration assay
Cells were serum-starved overnight, trypsinized, and resuspended in serum-free media. The cells were then seeded on transwell permeable supports with 8μm pores (Corning) on a 24-well plate. Cells were allowed to migrate towards serum-containing medium, which serves as a chemoattractant, for 24 h. Migrated cells were then dissociated from the basal side of the transwell using Hank’s-based Cell Dissociation Buffer (Life Technologies), stained with Calcein-AM (Life Technologies), and quantified using a plate reader.
Signaling pathway activity profiling
Cells seeded in 96-wells were fixed with 4% paraformaldehyde and the levels of target proteins spanning 20 pathways were determined using the ActivSignal IPAD platform (Immuno-Paired-Antibody Detection assay).
Statistical analysis
All statistical analyses were performed using the two-tailed student t-test. A p-value below 0.05 was considered statistically significant. Error bars represent standard deviation. All experiments were performed in triplicates.
Results
Microarray expression profiling of an EMT model of TNBC reveals miRNAs involved in suppressing malignant progression
To identify miRNAs that are likely to regulate tumor promotion/suppression in TNBC, we exploited a previously established model for transforming growth factor β (TGFβ)-induced EMT comprised of a pair of isogenic MIII cells with intact or deficient SMAD2 expression.Citation26 In silico Kaplan-Meier analyses revealed that the probability of distant metastasis-free survival (DMFS) and relapse-free survival (RFS) decreased significantly in patients with high levels of SMAD2 expression in BLBC, suggesting that SMAD2 is associated with a more aggressive phenotype in this disease subtype by maintaining intact TGFβ-SMAD signaling pathway ()).Citation21 Depletion of SMAD2 in MIII cells using shRNA led to a drastic increase in E-cadherin levels, with a corresponding decrease in vimentin levels, thereby changing the morphology of the cells from mesenchymal to more epithelial-like (). Importantly, knockdown of SMAD2 also diminished the migratory ability of MIII cells, confirming that intact TGFβ-SMAD2 signaling could play a role in acquiring tumorigenic properties by TNBC cells ()).
Figure 1. SMAD2 depletion reverses EMT and suppresses migration in TNBC cells. (a) In silico Kaplan-Meier analyses shows the correlation between SMAD2 expression and distant metastasis-free survival (DMFS, HR = 1.99 (1.17–3.37), logrank P = 0.0095), relapse-free survival (RFS, HR = 1.57 (1.22–2.03), logrank P = 0.00046), and overall survival (OS, HR = 1.51 (0.92–2.49), logrank P = 0.1) in patients with basal-like breast cancer. The analyses ran on a cohort of 232 (DMFS), 618 (RFS), and 241 (OS) patients, respectively. (b) Knockdown of SMAD2 in TNBC cells using shRNA and subsequent changes in E-cadherin, and vimentin levels was monitored by RT-qPCR (n = 3, *p < 0.05). (c) Western blotting shows the relative levels of SMAD2, E-cadherin, and vimentin in the indicated cell lines. (d) Representative images showing the closure of the wound in the indicated cell lines after 20 h of incubation.
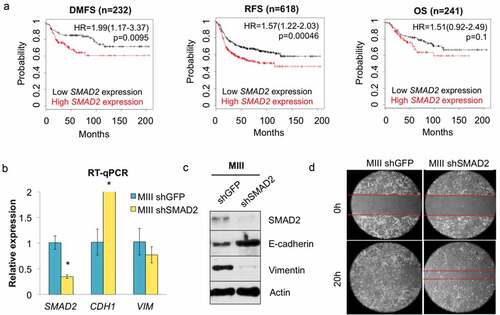
Next, we subjected MIIIshGFP and shSMAD2 cells to differential miRNA expression analysis and identified a list of miRNAs significantly suppressed in the malignant cells compared to the nonmalignant cells (). Among these miRNAs are miR-200c, miR-200b, and miR-141, which are well-established members of the miR-200 family known to suppress EMT, miR-205 (another EMT-suppressor), as well as miR-708 and miR-30a, which have been reported to play tumor-suppressive roles in breast cancer.Citation27–Citation32 Interestingly, the fold change of miR-4417 was the highest among these differentially expressed miRNAs, suggesting that it could play a critical role in suppressing the progression of TNBC cells.
Figure 2. SMAD2 depletion induces the expression of miRNAs that inhibit EMT and suppress progression in TNBC cells. (a) Unbiased hierarchical clustering of relative miRNA levels in MIIIshGFP (n = 3) and shSMAD2 (n = 3) cells. Legend shows the reported roles of the indicated miRNAs in breast cancer. (b) Principal component analysis across 2578 miRNAs between the two groups of samples (MIIIshGFP vs shSMAD2). (c) The change in expression levels of the indicated miRNAs was measured in isogenic mesenchymal (MIIIshGFP, LM2pQ) and epithelial cells (MIIIshSMAD2, LM2pQSDPR) using RT-qPCR (*p < 0.05).
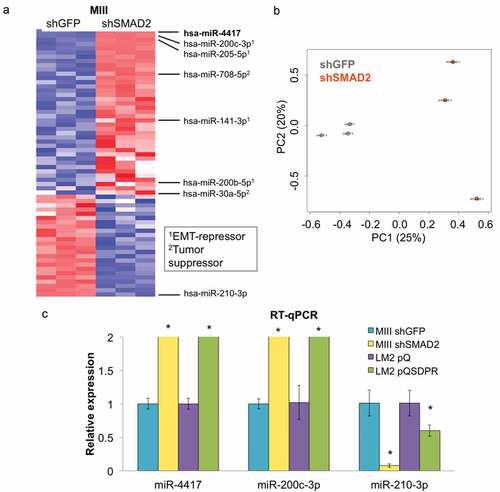
Mesenchymal TNBC cells exhibit low levels of miR-4417 compared to epithelial cells
To verify the changes in miRNA expression level observed from the microarray data, we performed RT-qPCR on selected miRNAs and confirmed that miR-4417 and miR-200c-3p were highly repressed in malignant MIIIshGFP cells compared to nonmalignant shSMAD2 cells. On the other hand, miR-210-3p was highly expressed in malignant MIIIshGFP cells. Moreover, the differential expression pattern of miR-4417, miR-200c-3p, and miR-210-3p was also observed in another established EMT model of TNBC comprised of isogenic LM2pQ (mesenchymal) and LM2pQSDPR (epithelial) cells ()).Citation33–Citation35 We proceeded to evaluate the expression of miR-4417 in a panel of breast cancer cell lines using RT-qPCR and again found that miR-4417 is more highly expressed in epithelial cells (NeoT, MIV, UACC812, MDA-MB-453) than in mesenchymal cells (SUM149, SUM159, MDA-MB-231, MDA-MB-231-BoM2), suggesting that low expression of miR-4417 may serve as a novel biomarker for malignant progression in breast cancer ()).
Figure 3. miR-4417 is suppressed in mesenchymal cells and is lost a subset of breast cancer. (a) The expression level of miR-4417 in a panel of breast cancer cell lines with epithelial or mesenchymal morphology was measured using RT-qPCR (*p < 0.05). (b) In silico Kaplan-Meier analysis showing the correlation between high miR-4417 expression and overall survival (OS, HR = 0.23 (0.08–0.79), logrank P = 0.0086) in TNBC patients. The analysis ran on a cohort of 97 patients. (c) In silico cBioPortal analysis shows the alteration frequency of miR-4417 across multiple breast cancer genomic profiling studies. (d) DICER depletion via shRNA in MIIIshSMAD2 cells was examined using RT-qPCR (*p < 0.05). (e) The levels of miR-4417 were measured using RT-qPCR upon knockdown of DICER in the indicated cell lines (*p < 0.05).
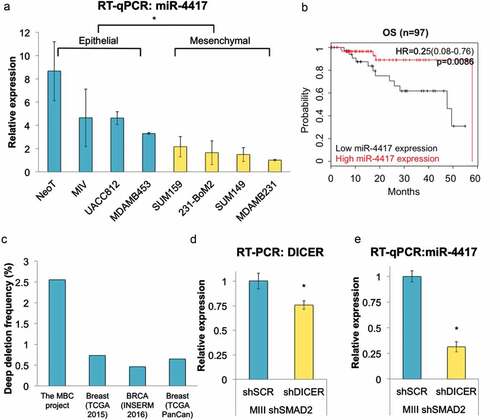
Low miR-4417 expression correlates with poor survival in TNBC patients
To determine if miR-4417 expression could predict prognosis in breast cancer, we performed Kaplan-Meier analysis using a recently established database.Citation22 We found that the probability of overall survival decreased significantly in TNBC patients with low levels of miR-4417, suggesting that miR-4417 is tumor-suppressive and could serve as a prognostic biomarker in this disease subtype ()). Interestingly, low levels of miR-4417 also correlate with worse prognosis in ER-negative, PR-negative, and HER2-positive breast cancer patients, indicating the potential applicability of miR-4417 as a potential prognostic biomarker in specific breast cancer subtypes as well as other cancer types (Suppl. Figs. 1 & 2).Citation23
Based on these observations, we hypothesized that miR-4417 acts as a tumor suppressor gene and may be lost or suppressed during breast cancer progression. Interestingly, miR-4417 is localized to chromosome 1p36, a genomic region associated with high frequency of loss of heterozygosity (LOH) in breast, cervical, colon, and thyroid cancers as well as melanoma.Citation36 In silico cBioPortal analysis revealed that miR-4417 is lost via deep deletion in a subset of breast cancer samples across multiple studies, most notably in the metastatic breast cancer (MBC) project, further strengthening the notion that the novel miRNA plays a tumor-suppressive role and may be targeted for inactivation to promote breast cancer progression ()).Citation37,Citation38
miR-4417 suppresses migration and mammosphere formation of TNBC cells in vitro
To validate that miR-4417 is a functional miRNA, we first knocked down DICER, a critical component in the miRNA biogenesis cascade, in TNBC cells ()). We observed that miR-4417 expression level diminished when DICER was depleted, indicating that the processing of miR-4417 is DICER-dependent ()). To determine if miR-4417 could suppress malignant phenotypes in TNBC, we transfected MIII cells with miR-4417 mimics and found that the migratory potential of the cells were significantly diminished (). Because EMT is known to confer tumor-initiating properties in cancer cells, we next assessed the ability of the TNBC cells to form three-dimensional mammospheres under serum-free conditions upon miR-4417 transfection.Citation39 As expected, TNBC cells overexpressing miR-4417 exhibited deficiency in growing as mammospheres, which are formed by tumor-initiating cells, further indicating that miR-4417 is tumor suppressive in TNBC ()). These patterns were similarly observed in SUM159 cells, suggesting that miR-4417 can be delivered as a therapeutic tool against TNBC ().
Figure 4. Overexpression of miR-4417 suppresses migration and tumorigenicity of TNBC cells in vitro. (a) MIII cells were transfected with negative control (NC) or miR-4417 mimics and the level of miR-4417 was monitored using RT-qPCR (*p < 0.05). (b) Transfected MIII cells were seeded on transwells for 24 h to assess their migratory ability (n = 3, *p < 0.05). (c) The number of transfected MIII cells growing as mammospheres under non-adherent conditions in serum-free medium was determined (n = 3, *p < 0.05). (d) The level of miR-4417 in transfected SUM159 cells was monitored using RT-qPCR (*p < 0.05). (e & f) Transfected SUM159 cells were assessed for their migratory and mammosphere formation abilities (n = 3, *p < 0.05).
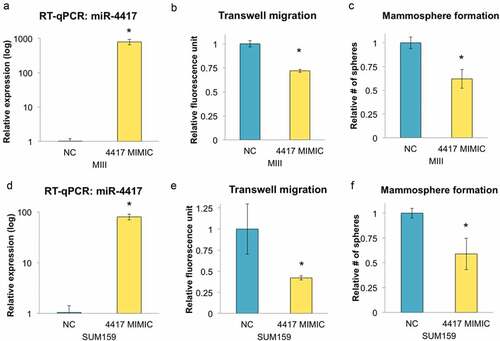
Discussion
Gene expression profiling studies have shown that miRNAs are globally downregulated in cancer when compared to normal tissues, in part through targeting of the miRNA biogenesis pathway, leading to induction of EMT to promote invasion, intravasation, and metastasis.Citation40–Citation42 The miR-200 family, consisting of miR-200a, miR-200b, miR-200c, miR-141, and miR-429, is one for the most well-characterized groups of EMT-suppressing miRNAs. The members in the family predominantly target ZEB1/ZEB2, which are transcriptional repressors of E-cadherin, a hallmark epithelial marker.Citation27 While these miRNAs were implicated in EMT suppression in many cancerous and non-cancerous cell types, specific tumor-suppressive miRNAs that inhibit progression of TNBC cells remain elusive.
Previously, we reported that sustained TGFβ-SMAD signaling pathway promotes EMT in breast cancer due to epigenetic regulation of gene expression via promoter DNA methylation and other mechanisms.Citation26 Here, we show that SMAD2 deficiency not only led to re-expression of E-cadherin but also impaired the migratory ability of these cells. We decided to exploit this EMT model to identify specific miRNAs that could inhibit TNBC. Differential expression analysis revealed a list of putative tumor-suppressive miRNAs significantly downregulated in malignant TNBC cells, including the novel miR-4417 with unknown function during TNBC progression. Evaluation of a panel of breast cancer cell lines further confirmed that miR-4417 is suppressed in cells with mesenchymal morphology when compared to those with epithelial morphology, suggesting that the miR-4417 is downregulated to promote cancer progression.
Because DICER has been reported to play a tumor suppressive role in breast cancer,Citation42 we sought to determine if the biogenesis of miR-4417 is dependent on this miRNA-processing protein. We found that the levels of miR-4417 decreased upon depletion of DICER, suggesting that miR-4417 processing is DICER-dependent and its expression may be indirectly suppressed when DICER is lost during TNBC progression. Interestingly, miR-4417 is localized to 1p36, an LOH hotspot in cancer potentially harboring a tumor suppressor gene. Furthermore, miR-4417 is deleted in a subset of breast cancer samples, suggesting that the miRNA loci could be directly inactivated to promote breast cancer progression.Citation38 Importantly, reduced expression of miR-4417 correlate with worse survival in TNBC patients, which is similarly observed with that of miR-200c, suggesting that these miRNAs could serve as prognostic biomarkers for TNBC ( & Supp. Figure 3).
Based on these observations, we hypothesized that miR-4417 plays a tumor suppressive role in TNBC. Delivery of miR-4417 mimics to TNBC cells significantly suppressed their migratory and self-renewal abilities, indicating that the miRNA exerts tumor-suppressive effects. Interestingly, signaling pathway activity profiling (ActivSignal) revealed that miR-4417 overexpression can significantly suppress the phosphorylation of proteins involved in cancer-regulating pathways, including those of p90RSK, p38 MAPK, and IGF-1R, suggesting that miR-4417 can serve as therapeutic tool to prevent malignant progression through interference with signaling pathways (Suppl. Figure 4). However, follow-up experiments should be performed to explore the interaction between miR-4417 and these pathways, particularly the p38 MAPK pathway, which has been reported to activate NF-κB and promote drug resistance and EMT in breast cancer.Citation43,Citation44
In conclusion, our findings suggest that miR-4417 expression is suppressed in TNBC cells undergoing EMT, which is a critical event during the early stage of metastasis. In addition, low expression of miR-4417 correlates with poor survival in patients with TNBC as well as other disease subtypes, suggesting the value of miR-4417 as a prognostic biomarker. Finally, we propose that miR-4417 plays a tumor suppressive role in TNBC, as shown by the impaired migratory and tumor-initiating properties in TNBC cells upon introduction of miR-4417 mimics, indicating its potential utility for development as a therapeutic agent in the future.
Disclosure of conflicts of interest
No potential conflicts of interest were disclosed.
Supplemental Material
Download Zip (870.1 KB)Acknowledgments
This work was supported by a grant from NIH/NCI (CA165707), grants funded by the Boston University Clinical & Translational Science Institute (NIH/NCATS award 1UL1TR001430 and CTSA award UL1TR000157), a seed grant from the Boston University Genome Science Institute to S.T. C.K.W is a recipient of the Boston University Cross-Disciplinary Training in Nanotechnology for Cancer (XTNC), BUnano, and Susan G. Komen Mentoring and Training in Cancer Health Disparities (MATCH) fellowships. We thank Dr Joan Massague for generously providing cell lines. We would like to thank the support from the Boston University School of Medicine Biomedical Genetics Section, the Boston University School of Medicine Microarray and Sequencing Resource, the Boston University School of Medicine Molecular Genetics Core Facility, and the Boston University Analytical Instrumentation Core Facility. We also thank Drs. Herbert T. Cohen, Shoumita Dasgupta, Hui Feng, and Marc Lenburg for their valuable suggestions for the research project.
Supplementary material
Supplemental data for this article can be accessed on the publisher’s website.
Additional information
Funding
References
- Siegel RL, Miller KD, Jemal A. Cancer statistics. CA Cancer J Clin. 2018;68:7–30. doi:10.3322/caac.21442.
- Turner NC, Neven P, Loibl S, Andre F. Advances in the treatment of advanced oestrogen-receptor-positive breast cancer. Lancet. 2017;389:2403–2414.
- Loibl S, Gianni L. HER2-positive breast cancer. Lancet. 2017;389:2415–2429.
- Denkert C, Liedtke C, Tutt A, von Minckwitz G. Molecular alterations in triple-negative breast cancer-the road to new treatment strategies. Lancet. 2017;389:2430–2442.
- Carey LA, Perou CM, Livasy CA, Dressler LG, Cowan D, Conway K. Race, breast cancer subtypes, and survival in the Carolina breast cancer study. JAMA. 2006;295:2492–2502.
- Bauer KR, Brown M, Cress RD, Parise CA, Caggiano V. Descriptive analysis of estrogen receptor (ER)-negative, progesterone receptor (PR)-negative, and HER2-negative invasive breast cancer, the so-called triple-negative phenotype: a population-based study from the California cancer registry. Cancer. 2007;109:1721–1728.
- Dent R, Trudeau M, Pritchard KI, Hanna WM, Kahn HK, Sawka CA. Triple-negative breast cancer: clinical features and patterns of recurrence. Clin Cancer Res. 2007;13:4429–4434.
- Perou CM, Sorlie T, Eisen MB, van de Rijn M, Jeffrey SS, Rees CA. Molecular portraits of human breast tumours. Nature. 2000;406:747–752.
- Livasy CA, Karaca G, Nanda R, Tretiakova MS, Olopade OI, Moore DT. Phenotypic evaluation of the basal-like subtype of invasive breast carcinoma. Mod Pathol. 2006;19:264–271.
- Badve S, Dabbs DJ, Schnitt SJ, Baehner FL, Decker T, Eusebi V, Fox SB, Ichihara S, Jacquemier J, Lakhani SR, et al. Basal-like and triple-negative breast cancers: a critical review with an emphasis on the implications for pathologists and oncologists. Mod Pathol. 2011;24:157–167. doi:10.1038/modpathol.2010.200.
- Prat A, Adamo B, Cheang MCU, Anders CK, Carey LA, Perou CM. Molecular characterization of basal-like and non-basal-like triple-negative breast cancer. Oncologist. 2013;18:123–133. doi:10.1634/theoncologist.2012-0397.
- Liedtke C, Mazouni C, Hess KR, André F, Tordai A, Mejia JA, Symmans WF, Gonzalez-Angulo AM, Hennessy B, Green M, et al. Response to neoadjuvant therapy and long-term survival in patients with triple-negative breast cancer. J Clin Oncol. 2008;26(8):1275–1281. doi: 10.1200/JCO.2007.14.4147.
- Thiery JP, Acloque H, Huang RY, Nieto MA. Epithelial-mesenchymal transitions in development and disease. Cell. 2009;139(5):871–890. doi: 10.1016/j.cell.2009.11.007.
- Mani SA, Guo W, Liao MJ, Eaton EN, Ayyanan A, Zhou AY, Brooks M, Reinhard F, Zhang CC, Shipitsin M, et al. The epithelial-mesenchymal transition generates cells with properties of stem cells. Cell. 2008;133(4):704–715. doi:10.1016/j.cell.2008.03.027.
- Polyak K, Weinberg RA. Transitions between epithelial and mesenchymal states: acquisition of malignant and stem cell traits. Nat Rev Cancer. 2009;9(4):265–273. doi:10.1038/nrc2620.
- May CD, Sphyris N, Evans KW, Werden SJ, Guo W, Mani SA. Epithelial-mesenchymal transition and cancer stem cells: a dangerously dynamic duo in breast cancer progression. Breast Cancer Res. 2011;13(1):202. doi:10.1186/bcr2789.
- Winter J, Jung S, Keller S, Gregory R, Diederichs S. Many roads to maturity: microRNA biogenesis pathways and their regulation. Nat Cell Biol. 2009;11(3):228–234. doi:10.1038/ncb0309-228.
- Lim LP, Lau NC, Garrett-Engele P, Grimson A, Schelter JM, Castle J, Bartel DP, Linsley PS, Johnson JM. Microarray analysis shows that some microRNAs downregulate large numbers of target mRNAs. Nature. 2005;433(7027):769–773.
- Rupaimoole R, Slack FJ. MicroRNA therapeutics: towards a new era for the management of cancer and other diseases. Nat Rev Drug Discov. 2017;16(3):203–222. doi:10.1038/nrd.2016.246.
- Chakraborty C, Sharma AR, Sharma G, Doss CGP, Lee SS. Therapeutic miRNA and siRNA: moving from bench to clinic as next generation medicine. Mol Ther Nucleic Acids. 2017;8:132–143. doi:10.1016/j.omtn.2017.06.005.
- Györffy B, Lanczky A, Eklund AC, Denkert C, Budczies J, Li Q, Szallasi Z. An online survival analysis tool to rapidly assess the effect of 22,277 genes on breast cancer prognosis using microarray data of 1,809 patients. Breast Cancer Res Treat. 2010;123(3):725–731. doi:10.1007/s10549-009-0674-9.
- Lanczky A, Nagy A, Bottai G, Munkacsy G, Paladini L, Szabo A, Santarpia L, Győrffy B. miRpower: a web-tool to validate survival-associated miRNAs utilizing expression data from 2,178 breast cancer patients. Breast Cancer Res Treat. 2016;160(3):439–446. doi:10.1007/s10549-016-4013-7.
- Á N, Lánczky A, Menyhárt O, Győrffy B. Validation of miRNA prognostic power in hepatocellular carcinoma using expression data of independent datasets. Sci Rep. 2018;8(1):9227. doi:10.1038/s41598-018-27521-y.
- Irizarry RA, Hobbs B, Collin F, Beazer-Barclay YD, Antonellis KJ, Scherf U, Speed TP. Exploration, normalization, and summaries of high density oligonucleotide array probe level data. Biostatistics. 2003;4(2):249–264.
- Benjamini Y, Hochberg Y. Controlling the false discovery rate: a practical and powerful approach to multiple testing. J Roy Statist Soc Ser B. 1995;57(1):289–300.
- Papageorgis P, Lambert AW, Ozturk S, Gao F, Pan H, Manne U, Alekseyev YO, Thiagalingam A, Abdolmaleky HM, Lenburg M, et al. Smad signaling is required to maintain epigenetic silencing during breast cancer progression. Cancer Res. 2010;70(3):968–978. doi:10.1158/0008-5472.CAN-09-1872.
- Park SM, Gaur AB, Lengyel E, Peter ME. The miR-200 family determines the epithelial phenotype of cancer cells by targeting the E-cadherin repressors ZEB1 and ZEB2. Genes Dev. 2008;22(7):894–907. doi:10.1101/gad.1640608.
- Gregory PA, Bert AG, Paterson EL, Barry SC, Tsykin A, Farshid G, Vadas MA, Khew-Goodall Y, Goodall GJ. The miR-200 family and miR-205 regulate epithelial to mesenchymal transition by targeting ZEB1 and SIP1. Nat Cell Biol. 2008;10(5):593–601. doi:10.1038/ncb1722.
- Lee JW, Guan W, Han S, Hong DK, Kim LS, Kim H. MicroRNA-708-3p mediates metastasis and chemoresistance through inhibition of epithelial-to-mesenchymal transition in breast cancer. Cancer Sci. 2018;109(5):1404–1413. doi:10.1111/cas.13588.
- Ryu S, McDonnell K, Choi H, Gao D, Hahn M, Joshi N, Park SM, Catena R, Do Y, Brazin J, et al. Suppression of miRNA-708 by polycomb group promotes metastases by calcium-induced cell migration. Cancer Cell. 2013 Jan 14;23(1):63–76. doi:10.1016/j.ccr.2012.11.019.
- Fu J, Xu X, Kang L, Zhou L, Wang S, Lu J, Cheng L, Fan Z, Yuan B, Tian P, et al. miR-30a suppresses breast cancer cell proliferation and migration by targeting eya2. Biochem Biophys Res Commun. 2014;445(2):314–319. doi:10.1016/j.bbrc.2014.01.174.
- Li L, Kang L, Zhao W, Feng Y, Liu W, Wang T, Mai H, Huang J, Chen S, Liang Y, et al. miR-30a-5p suppresses breast tumor growth and metastasis through inhibition of LDHA-mediated warburg effect. Cancer Lett. 2017;400:89–98. doi:10.1016/j.canlet.2017.04.034.
- Ozturk S, Papageorgis P, Wong CK, Lambert AW, Abdolmaleky HM, Thiagalingam A, Cohen HT, Thiagalingam S. SDPR functions as a metastasis suppressor in breast cancer by promoting apoptosis. Proc Natl Acad Sci USA. 2016;113(3):638–643. doi:10.1073/pnas.1514663113.
- Camps C, Buffa FM, Colella S, Moore J, Sotiriou C, Sheldon H, Harris AL, Gleadle JM, Ragoussis J. hsa-miR-210 is induced by hypoxia and is an independent prognostic factor in breast cancer. Clin Cancer Res. 2008;14(5):1340–1348. doi:10.1158/1078-0432.CCR-07-1755.
- Rothé F, Ignatiadis M, Chaboteaux C, Haibe-Kains B, Kheddoumi N, Majjaj S, Badran B, Fayyad-Kazan H, Desmedt C, Harris AL, et al. Global microRNA expression profiling identifies MiR-210 associated with tumor proliferation, invasion and poor clinical outcome in breast cancer. PLoS One. 2011;6(6):e20980. doi:10.1371/journal.pone.0020980.
- Bagchi A, Mills AA. The quest for the 1p36 tumor suppressor. Cancer Res. 2008;68(8):2551–2556. doi:10.1158/0008-5472.CAN-07-2095.
- Cerami E, Gao J, Dogrusoz U, Gross BE, Sumer SO, Aksoy BA, Jacobsen A, Byrne CJ, Heuer ML, Larsson E, et al. The cBio cancer genomics portal: an open platform for exploring multidimensional cancer genomics data. Cancer Discov. 2012;2(5):401–404. doi:10.1158/2159-8290.CD-12-0095.
- Gao J, Aksoy BA, Dogrusoz U, Dresdner G, Gross B, Sumer SO, Sun Y, Jacobsen A, Sinha R, Larsson E, et al. Integrative analysis of complex cancer genomics and clinical profiles using the cBioPortal. Sci Signal. 2013;6(269):pl1. doi:10.1126/scisignal.2004088.
- Lambert AW, Wong CK, Ozturk S, Papageorgis P, Raghunathan R, Alekseyev Y, Gower AC, Reinhard BM, Abdolmaleky HM, Thiagalingam S. Tumor cell-derived periostin regulates cytokines that maintain breast cancer stem cells. Mol Cancer Res. 2016 Jan;14(1):103–113. doi:10.1158/1541-7786.MCR-15-0079.
- Lu J, Getz G, Miska EA, Alvarez-Saavedra E, Lamb J, Peck D, Sweet-Cordero A, Ebert BL, Mak RH, Ferrando AA, et al. MicroRNA expression profiles classify human cancers. Nature. 2005;435(7043):834–838.
- Kumar MS, Lu J, Mercer KL, Golub TR, Jacks T. Impaired microRNA processing enhances cellular transformation and tumorigenesis. Nat Genet. 2007;39(5):673–677.
- Martello G, Rosato A, Ferrari F, Manfrin A, Cordenonsi M, Dupont S, Enzo E, Guzzardo V, Rondina M, Spruce T, et al. A MicroRNA targeting dicer for metastasis control. Cell. 2010;141(7):1195–1207. doi:10.1016/j.cell.2010.05.017.
- Madrid LV, Mayo MW, Reuther JY, Baldwin AS Jr. Akt stimulates the transactivation potential of the RelA/p65 subunit of NF-kappa B through utilization of the Ikappa B kinase and activation of the mitogen-activated protein kinase p38. J Biol Chem. 2001;276(22):18934–18940.
- Antoon JW, Nitzchke AM, Martin EC, Rhodes LV, Nam S, Wadsworth S, Salvo VA, Elliott S, Collins-Burow B, Nephew KP, et al. Inhibition of p38 mitogen-activated protein kinase alters microRNA expression and reverses epithelial-to-mesenchymal transition. Int J Oncol. 2013 Apr;42(4):1139–1150. doi:10.3892/ijo.2013.1814.