ABSTRACT
Emerging evidence had highlighted that exosomes could mediate cell-cell communication in human cancerous development via transferring the various molecular cargos, including long non-coding RNA (lncRNA). Taurine up-regulated 1 (TUG1) was previously reported as an oncogenic lncRNA in cervical cancer (CC) via facilitating cell proliferation and other vital biological behaviors. Nevertheless, the presence of TUG1 in exosomes and the functional regulation of exosomal TUG1 in CC are still elusive. The current study aimed at the communication between CC cell lines and endothelial cell-mediated by exosomes, as well as the roles of exosomes derived from CC cells and exosomal TUG1 in affecting angiogenesis. Initially, it was found that TUG1 expression was upregulated in both CC cells and their secreted exosomes. TUG1 was transferred from CC cells to recipient human umbilical vein endothelial cells (HUVECs) in the exosomes way. Interestingly, TUG1 depletion impaired the exosomes-mediated proangiogenic potential of HUVECs by modulating certain key angiogenesis-related genes. In addition, exosomal TUG1 contributed to HUVECs proliferation through suppressing caspase-3 activity and impacting apoptosis-related proteins. Collectively, we identified a new exosomes-mediated molecular mechanism by which CC cells transferred TUG1 via exosomes to recipient HUVECs, thus promoting angiogenesis, providing a promising target for early diagnosis of CC.
Introduction
Cervical cancer (CC), one of the most prevalent gynecological malignancies, is featured with poor prognosis, seriously threatening female health.Citation1,Citation2 However, predominant therapeutic schemes for CC encompassing surgery, chemotherapy, and radiotherapy were failed to improve this situation.Citation3 The unsatisfactory prognosis is mainly attributed to strong distant metastasis ability of CC.Citation4 Consequently, novel biomarkers for early diagnosis and better prognosis of CC are urgently needed to promote survival rate.
Advances with the development of sequencing technologies reveal that the human genome is transcribed pervasively to a multitude of new non-coding RNAs (ncRNAs). Long noncoding RNAs (lncRNAs) are emerging as RNA transcripts >200 nucleotides in length, distinct from other classes of small and structural RNAs.Citation5 Recent data suggested that dysregulated lncRNAs were strongly related to the cellular functions defined as the hallmark cancerous processes, as detected either in cancer tissues and/or in biological fluids.Citation6,Citation7 Growing evidence supports the involvement of lncRNA in CC progression. LncRNA taurine up-regulated 1 (TUG1), located at chromosome 22q12, was identified to be upregulated in CC tissues and cells, in close relation to the advanced clinical characteristic and poor overall survival.Citation8 TUG1 can promote CC progression via promoting cell proliferation and inhibiting cell apoptosis.Citation9 TUG1 thus functioned as an oncogene in CC and might represent a novel therapeutic target.
Exosomes are small membranous vesicles (30–150 nm), especially cup-shaped vesicles with diameters of 40–100 nm. They exist in all biological fluids encompassing cervical secretion.Citation10–Citation12 Exosomes can transfer molecules cargo, such as proteins and genetic material (DNA, lncRNA, miRNA, and mRNA), which disclose the genetic information of their parent cells.Citation13 In this manner, exosomes mediate the cell-cell communication and exert diverse biological functions in human tumors. It has recently reported the enrichment of lncRNAs in secreted exosomes from CC cells,Citation14 implying that exosomal lncRNAs can be developed into the valuable biomarkers for the early detection, diagnosis, and prognosis of CC.
This study confirmed the presence of TUG1 in exosomes from CC cells and focused on the value of exosomal TUG1 in CC progression. We disclosed the fact that CC cells could transfer TUG1 via exosomes into recipient HUVECs, promoting angiogenesis and HUVECs proliferation. Our findings might shed new light on the identification of new markers for CC.
Materials and methods
Cell lines
Human cervical epithelial cells (CerEpiC) and two accepted CC cell lines (HeLa, CaSki) were utilized in our study, from American Type Culture Collection (ATCC, Manassas, VA, USA), as well as human umbilical vein endothelial cells (HUVECs). Roswell Park Memorial Institute-1640 medium (RPMI-1640; Sigma Aldrich, Saint-Louis, Missouri, USA) was used for culturing cell with supplements containing 10% fetal bovine serum (FBS; Gibco, Carlsbad, CA, USA), penicillin (100 U/mL) and streptomycin (100 mg/mL). HUVECs were cultured with 6 µg/ml endothelial cell growth supplement (ECGS, BD Bioscience), 10 units/ml heparin. Routine culture was conducted at 37°C under 5% CO2/95% air.
Quantitative real-time polymerase chain reaction (qRT-PCR)
Extraction of total cellular RNA was performed as demanded by the supplier of TRIzol Reagent (Invitrogen, Carlsbad, CA, USA). Using PrimeScript II 1st Strand cDNA Synthesis Kit from Thermo Fisher Scientific (Waltham, MA, USA), RNA was transcribed reversely into first-strand complementary DNA (cDNA). SYBR Green (Takara, Tokyo, Japan) was then applied on ABI 7500 Real-time PCR System (Applied Biosystems, Foster City, CA, USA). GAPDH served as an endogenous normalizer. Relative gene expression was determined by 2−ΔΔCt method. Primer sequences were shown in .
Table 1. Related primer sequences were presented.
Antibody and reagent
Commercially available antibodies were acquired from Abcam (Cambridge, MA, USA), including primary antibodies against CD63 (ab59479), CD9 (ab2215), TSG101 (ab83), HSP90 (ab13495), GM130 (ab32337), Bcl-2 (ab692), Bcl-xl (ab2568), Bak (ab32371), Bax (ab53154), GAPDH (ab22555), and appropriate secondary horseradish peroxidase-conjugated antibody. GW4869, N-SMase2 chemical inhibitor, was bought from Santa Cruz Biotechnology (Santa Cruz, CA, USA) to block exosomes release. Actinomycin D from Sigma-Aldrich, RNA polymerase II inhibitor, was applied for blocking mRNA synthesis. Z-DEVD-FMK, the caspase-3 inhibitor was purchased from Selleck Chemicals (Houston, TX, USA).
Exosomes isolation and purification
After ultracentrifugation, cells were grown routinely in medium without FBS. Confluent cells were washed thrice in phosphate-buffered saline (PBS) and cultivated in the medium for 48 h. 1 × 109 exosomes were isolated from CerEpiC, HeLa, or CaSki cell supernatant (termed CerEpiC-Exo, HeLa-Exo or CaSki-Exo) using Total Exosomes Isolation Reagent from Thermo Fisher Scientific as per strictly the direction and purified for further analysis.
Nanoparticle tracking analysis (NTA)
Exosome samples resuspended in PBS were treated with a syringe filter (Millipore, Billerica, MA, USA) prior to dilution to track individual nanoparticles. The size distribution of the exosomes was examined by the use of NanoSight NS300 instrument from Malvern Instruments Ltd. (Worcestershire, UK).
Western blotting
Cell extracts were prepared in RIPA lysis buffer, then 25 µg of cellular protein samples were collected for SDS-PAGE. Following transferring samples onto nitrocellulose (NC) membrane (Millipore), 1% bovine serum albumin (BSA) was utilized to block membranes at room temperature for 1 h. Samples on membranes were cultured with primary antibodies at 4°C all night and then with secondary antibody at 37°C for 1 h prior to observation with enhanced chemiluminescence reagents (Millipore).
Tube formation assay
Growth factor-reduced Matrigel was bought from BD Biosciences (San Jose, CA, USA) and put into 48-well plates. HUVECs in serum-free medium were cultivated for 12 h and placed into 48-well plates which were pre-coated with Matrigel for 10 h. Tube formation was tested in pictures taken under a microplate. The angiogenic potential was assessed by total branching points, total tube length, and total loops.
Plasmid transfection
After cell density reached 50–80%, cells in 6-well culture plates were transfected with three specific short hairpin RNAs (shRNAs) to TUG1 and nonspecific shRNA as a negative control in accordance with the protocol of Lipofectamine2000 kit (Invitrogen). Interference sequences were shown as follows: sh-TUG1#1 CTACCAACCAACACCCTAAAGATGAAAC; sh-TUG1#2 AAACAGACGCGGAGTCAAAGGAGGAGAC; sh-TUG1#3 GTACCACGACGGTCGGGTCGATTAATTA. 48 h later, cells were reaped for further analysis.
Cell counting kit-8 (CCK-8) assay
After 48 h of transfection, cells were collected in 96-well plates at 6 × 103 cells/well. Culture medium was changed to fresh medium containing 10% (v/v) CCK-8 solution (Dojindo, Kumamoto, Japan) at indicated time points. Absorbance at 450 nm of each well was tested on a microplate reader.
Colony formation assay
Cells were plated into 6-well plates (500 cells per well) for 2 weeks. Fixed cells were stained in 0.1% crystal violet. After taking pictures, the number of colonies containing more than 50 cells were counted and recorded.
5-ethynyl-20-deoxyuridine (EdU) incorporation assay
EdU incorporation assay kit was bought from Ribobio (Guangzhou, Guangdong, China) for cell proliferation assay. Cells were treated and planted into 96-well plates, followed by the addition of 100 μL of 50 μM EdU diluent. After 3 h, cells were fixed in 4% paraformaldehyde, cultivated with 100 μL of 0.5% Troxin X-100 and mixed with 100 μL of 1 × Apollo® 488 fluorescent staining solution. Cell nucleus was finally subjected to DAPI staining in the dark.
Measurement of caspase-3 activity
Colorimetric caspase-3 assay kit from Abcam was utilized as per the user manual. 5 × 106 cultured cells in chilled cell lysis buffer were reaped for isolating cell supernatant. After assessing protein concentration, each sample was cultivated with 2 × Reaction Buffer and DEVD-p-NA substrate for 2 h. Absorbance at a wavelength of 405 nm was determined by a microplate reader.
Cytokine analysis
Culture medium was collected 24 h after the treatment with or without exosomes. The concentrations of all cell cytokines in supernatants were measured using ELISA and Signosis-Catalog Number EA-4012 kits (BD Technologies).
Labeling of RNA and transfer into cells
The details of this experiment were presented in this reference.Citation15
Statistical analyses
Experimental data were exhibited as mean ± standard deviation (S.D.), with each sample run in triplicate. SPSS v.19.0 (SPSS, Chicago, IL, USA) and GraphPad Prism v.7.0 (GraphPad, San Diego, CA, USA), which are both for Windows, were applied for data analysis and graph generation. Significant differences were determined by Student’s t-test or ANOVA, with the p-value threshold set as 0.05.
Results
The obvious expression level of TUG1 was observed in CC cell lines and their secreted exosomes
Previous researchesCitation8,Citation9 have showed that TUG1 was abundantly expressed in CC cell lines, especially in HeLa and CaSki. To verify the conclusion, qRT-PCR was performed and demonstrated that TUG1 expression was upregulated in HeLa and CaSki cell lines comparing with normal cell line CerEpiC, which were consistent with the previous researches ()). As exosomes are the pivotal mediator of intercellular communication to affect cancerous biological behaviors, the exosomes-based mechanism was explored in this study. We isolated and characterized the exosomes derived from HeLa and CaSki, with CerEpiC as a negative control. Experimental result of nanoparticle tracking analysis (NTA) showed that there was no obvious significant difference among the size distribution profiles of CerEpiC-Exo, HeLa-Exo, and CaSki-Exo, with the peaks of the particle size ~100 nm, which was within the expected size of exosomes ()). Western blotting was then conducted to confirm the presence of four acknowledged exosomal markers (CD63, CD9, TSG101, and HSP90) in exosomes. As shown in ), both HeLa-Exo and CaSki-Exo were sufficiently expressed in exosomal markers, indicating that exosomes isolation was successful. Subsequently, the expression level of TUG1 in CerEpiC-Exo, HeLa-Exo, and CaSki-Exo was determined by qRT-PCR analysis. Result revealed that TUG1 level was dramatically increased not only in HeLa and CaSki cells, but also in respective secreted exosomes in comparison with CerEpiC and CerEpiC-Exo ()). Intriguingly, the upregulated tendency of TUG1 in exosomes was more evident than that in their parental cells, suggesting TUG1 might be not only an intracellular lncRNA but more an exosomal lncRNA in CC.
Figure 1. The obvious expression level of TUG1 was observed in CC cell lines and their secreted exosomes. (a). qRT-PCR examined the expression of TUG1 expression in CC cell lines (HeLa, CaSki) and human cervical epithelial cells (CerEpiC). (b). Nanoparticle tracking analysis was applied for the size distribution of HeLa-Exo, CaSki-Exo and CerEpiC-Exo (the cells were cultivated in the medium for 48 h. 1 × 109 exosomes were isolated from CerEpiC, HeLa, or CaSki cell supernatant). (c). Western blot analysis illustrated the expression situation of exosomal markers in exosomes derived from HeLa and CaSki. GM130, the total cellular protein marker, served as control. (d). The expression of TUG1 in CC cell lines and their secreted exosomes was detected by qRT-PCR. Data are exhibited as mean ± standard deviation (S.D.; n ≥ 3), **P < .01.
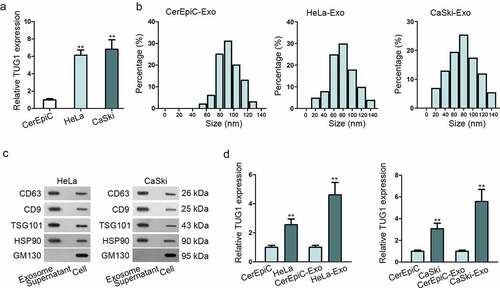
Exosomes derived from CC cells facilitated angiogenesis of HUVECs and transferred TUG1 to HUVECs
Tumor angiogenesis occupies an indispensable position in cancerous progression, so we then probed into the function of HeLa-Exo and CaSki-Exo in regulating the tube formation of HUVECs for determining the impact of exosomes derived from CC cells on angiogenesis. In the meantime, HUVECs were co-cultured with CC cells after transfecting Cy3-labeled TUG1 in CC cells, the location of TUG1 in Supplementary Figure 1(a) demonstrates that the successful delivering of TUG1 to the recipient cell. As presented in ), all indicators of tube formation ability, including the total branching points, total tube length, and total loops, were markedly enhanced in HUVECs incubated with HeLa or CaSki cell lines compared with the control group. However, treatment with GW4869 which could inhibit exosome secretion abolished partly this proangiogenic effect. Above data manifested the proangiogenic potential of HeLa-Exo and CaSki-Exo. Given that exosomes must be internalized by recipient cells to release their cargo and achieve their function, we confirmed that HeLa-Exo and CaSki-Exo could be internalized by HUVECs, thus exerting regulatory role in HUVECs angiogenesis. For cargo RNAs in exosomes are responsible for the exosomal function, we then speculated that TUG1 in exosomes could contribute to this proangiogenic capacity. Furthermore, it’s necessary to confirm Whether TUG1 could be transferred from HeLa and CaSki to HUVECs in the exosomes manner. Not surprisingly, it was observed that TUG1 level was distinctly strengthened in HUVECs treated with both HeLa-Exo and CaSki-Exo in comparison with that in untreated HUVECs, and this increase was not hindered by Actinomycin D (RNA polymerase II inhibitor for inhibiting mRNA synthesis), consistently with an existing reportCitation16 ()). Additionally, in Supplementary Figure 2A, Signosis-Catalog Number EA-4012 kit was applied to detect ELISA-based cytokine array, further proving that exosomal TUG1 promoted angiogenesis. In the whole, TUG1 was delivered from HeLa and CaSki cells to HUVECs by means of exosomes, which might play a role of exosomal lncRNA.
Figure 2. Exosomes derived from CC cells facilitated the angiogenesis of HUVECs and transferred TUG1 to HUVECs. (a). Tube formation assay in HUVECs uncovered the changes of angiogenesis after co-culture with HeLa or CaSki cell line, as well as with GW4869 treatment. (b). The expression of TUG1 was detected by qRT-PCR in HUVECs treated with HeLa-Exo or CaSki-Exo under Actinomycin D supplementation. Data are exhibited as mean ± standard deviation (S.D.; n ≥ 3), **P < .01.
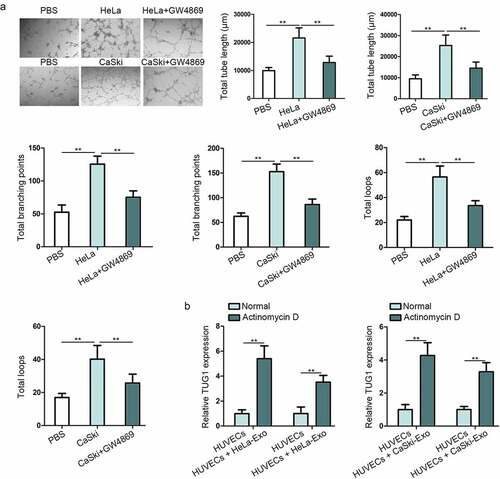
Exosomal TUG1 promoted angiogenesis of HUVECs
Based on the previous works cited above, TUG1 could promote the metastatic ability of CC cells. We thus hypothesized that the angiogenesis in CC might be modulated by exosomal TUG1 in some way. We initially silenced TUG1 expression to confirm whether the proangiogenic ability of HeLa-Exo and CaSki-Exo was dependent on exosomal TUG1. Result presented in ) indicated that sh-TUG1#2 more efficiently reduced TUG1 expression not only in HeLa and CaSki but in their secreted exosomes and the recipient HUVECs. In addition, TUG1 depletion weakened the proangiogenic potential of HeLa-Exo and CaSki-Exo in HUVECs ()). To probe into the possible mechanism by which exosomal TUG1 contributed to HUVECs angiogenesis, the expression levels of certain angiogenesis-associated genes were tested by qRT-PCR method after knocking down TUG1. As shown in ), vascular endothelial growth factor (VEGF-A), matrix metallopeptidase 9 (MMP-9), transforming growth factor-β (TGF-β), interleukin (IL-8) and basic fibroblast growth factor (bFGF) were possible downstream mediators of exosomal TUG1 activity. These observations proved that exosomal TUG1 modulated angiogenesis at least partly via VEGF-A, MMP-9, TGF-β, IL-8, and bFGF in CC.
Figure 3. Exosomal TUG1 promoted the angiogenesis of HUVECs. (a). qRT-PCR measured TUG1 expression in shRNAs transfected HeLa and CaSki cell lines as well as their secreted exosomes and the recipient HUVECs. (b). Tube formation assay was conducted in HUVECs co-cultured with PBS (blank group) or transfected HeLa-Exo and CaSki-Exo. (c). The expression levels of certain angiogenesis-associated genes were examined by qRT-pCR. Data are exhibited as mean ± standard deviation (S.D.; n ≥ 3), *P < .05, **P < .01.
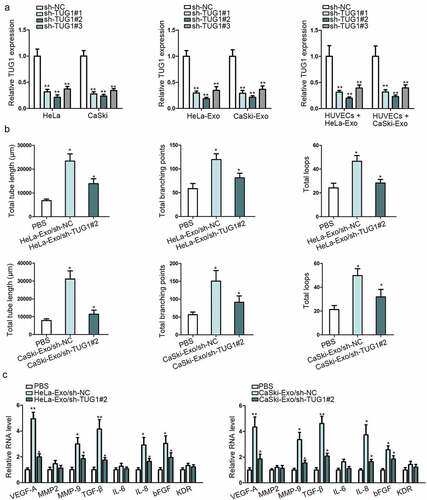
Exosomal TUG1 positively regulated the proliferative potential of HUVECs
Further investigation about the effects of exosomal TUG1 on HUVECs proliferation was achieved by CCK-8, colony formation, and EdU incorporation assays. Their results implied that the cell viability, colonies numbers, and EdU-positive cells of HUVECs were increased by culturing with HeLa-Exo or CaSki-Exo, but was decreased by TUG1 knockdown, inferring the positive regulation of exosomal TUG1 on HUVECs proliferation (–c)). Furthermore, the inhibition of apoptotic HUVECs caused by HeLa/CaSki-Exo was reversed through silencing TUG1, but Z-DEVD-FMK treatment offset the rescuing role of silencing TUG1 ()). Result of western blotting suggested that the level of pro-apoptotic proteins (Bak and Bax) was suppressed by exosomes derived from HeLa and CaSki cells but restored partly via TUG1 depletion, while the strengthened level of anti-apoptotic proteins (Bcl-2 and Bcl-xl) after incubating with HeLa-Exo or CaSki-Exo was abolished partially through silencing TUG1 expression ()). This opposite finding implied the negative modulation of TUG1 on HUVECs apoptosis. In sum, we can conclude that exosomal TUG1 boosted HUVECs proliferation by reducing apoptotic cells.
Figure 4. Exosomal TUG1 positively regulated the proliferative potential of HUVECs. (a–c). CCK-8, colony formation, and EdU assays were performed to test the proliferation ability of HUVECs in different transfected groups. (d). The caspase-3 activity was evaluated in different transfected groups. (e). Western blotting measured the levels of Bcl-2, Bcl-xl, Bak, and Bax in HeLa/CaSki-Exo-mediated HUVECs. Data are exhibited as mean ± standard deviation (S.D.; n ≥ 3), *P < .05, **P < .01.
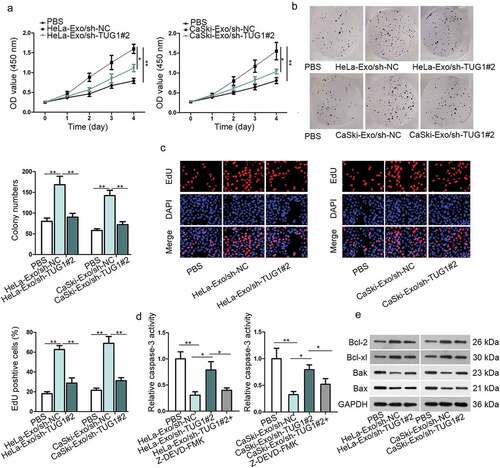
Discussion
Despite that well-run screening project in high-risk groups contributes to the sharp reduction in the incidence and mortality of CC, CC is still the dominating cause of cancer-associated deaths among females around the world.Citation17–Citation19 Hence, more valuable biomarkers are strongly indispensable for improving the early diagnosis and prognosis of patients with CC.
Much attention has been paid into research about the involvement of exosomes in human tumor progression in recent years. Long non-coding RNA (LncRNA) has attracted increasing interests among the cargo components of exosomes. Growing evidence has emphasized that lncRNA holds a significant place in multiple aspects of human tumor development, such as cell proliferative ability and angiogenesis.Citation20,Citation21 Angiogenesis is involved in providing nutrients and oxygen to tumors for the continuous tumor growth. As the shared feature of all human tumors, angiogenesis is known as the prerequisite to tumor metastasis, due to its participation in facilitating tumor cells to leave primary sites for distant organs or circulatory system.Citation22–Citation27 Therefore, it has become a hot topic to explore the potential mechanisms of angiogenesis for developing new therapeutic strategies of human malignancies. A quintessential example should be cited that epithelial ovarian cancer (EOC) cells could accelerate angiogenesis through the regulation of exosomes-mediated transfer of lncRNA metastasis-associated lung adenocarcinoma transcript 1 (MALAT1) to HUVECs.Citation28 Qiu JJ et al. stated that the obvious upregulation of lncRNA MALAT1 could be found in the exosomes of serum acquired from patients with EOC. Moreover, they concluded that serum exosomal MALAT1 might serve as a prognostic biomarker for EOC. Currently, TUG1, a well-known lncRNA, is the major research object. TUG1 was previously reported to exert crucial roles in regulating diverse biological behaviors of other human malignancies, including small cell lung cancer, hepatocellular carcinoma, gastric cancer, and so on.Citation29–Citation31 Notably, the research finding of Cai H et al. suggested that TUG1 could strengthen the tumor-induced angiogenesis and VEGF expression in human glioblastoma.Citation32 In the present work, we firstly identified TUG1 as an exosomal lncRNA in CC. All our findings proved that the functional oncogenic lncRNA TUG1 could be delivered from the two CC cell lines HeLa and CaSki to the endothelial cell line HUVECs in the exosomes-transferring manner. Remarkably, exosomal TUG1 promoted angiogenesis and endothelial cell proliferation in CC via stimulating expression of angiogenesis-related genes and inhibiting cell apoptosis, facilitating the tumor-promoting phenotype.
Jointly, our research data provide a novel mechanism by which CC cells can transfer lncRNA TUG1 mediated by exosomes to recipient HUVECs, thus enhancing the proliferative and angiogenetic capacities of HUVECs, as shown in . This study is the first to validate the presence of TUG1 in exosomes derived from CC cells and focus on the impact of exosomal TUG1 on the angiogenesis of endothelial cells in CC. But the prognostic value of exosomal TUG1 for CC patients remains to be elucidated in the future research.
Disclosure of Potential Conflicts of Interest
Authors have no commercial or other relationships that could pose a conflict of interest.
Authors’ contributions
Lei Lei: manuscript editing, review, and data analysis
Lei Lei, Qinwei Mou: data collection and experiments
Qinwei Mou: figures preparation
Supplemental Material
Download Zip (876.4 KB)Acknowledgments
Authors deeply appreciated all lab members’ support.
Supplementary material
Supplemental data for this article can be accessed on the publisher’s website.
References
- Jemal A, Bray F, Center MM, Ferlay J, Ward E, Forman D. Global cancer statistics. CA Cancer J Clin. 2011;61:69–90. doi:10.3322/caac.20107.
- Torre LA, Bray F, Siegel RL, Ferlay J, Lortet-Tieulent J, Jemal A. Global cancer statistics, 2012. CA Cancer J Clin. 2015;65:87–108. doi:10.3322/caac.21262.
- Vaccarella S, Lortet-Tieulent J, Plummer M, Franceschi S, Bray F. Worldwide trends in cervical cancer incidence: impact of screening against changes in disease risk factors. Eur J Cancer (Oxford, England: 1990). 2013;49:3262–3273. doi:10.1016/j.ejca.2013.04.024.
- Waggoner SE. Cervical cancer. Lancet (London, England). 2003;361:2217–2225. doi:10.1016/S0140-6736(03)13778-6.
- Spizzo R, Almeida MI, Colombatti A, Calin GA. Long non-coding RNAs and cancer: a new frontier of translational research? Oncogene. 2012;31:4577–4587. doi:10.1038/onc.2011.621.
- Gupta RA, Shah N, Wang KC, Kim J, Horlings HM, Wong DJ, Tsai MC, Hung T, Argani P, Rinn JL, et al. Long non-coding RNA HOTAIR reprograms chromatin state to promote cancer metastasis. Nature. 2010;464:1071–1076. doi:10.1038/nature08975.
- Hirata H, Hinoda Y, Shahryari V, Deng G, Nakajima K, Tabatabai ZL, Ishii N, Dahiya R. Long noncoding RNA MALAT1 promotes aggressive renal cell carcinoma through Ezh2 and interacts with miR-205. Cancer Res. 2015;75:1322–1331. doi:10.1158/0008-5472.CAN-14-2931.
- Zhu J, Shi H, Liu H, Wang X, Li F. Long non-coding RNA TUG1 promotes cervical cancer progression by regulating the miR-138-5p-SIRT1 axis. Oncotarget. 2017;8:65253–65264. doi:10.18632/oncotarget.18224.
- Hu Y, Sun X, Mao C, Guo G, Ye S, Xu J, Zou R, Chen J, Wang L, Duan P, et al. Upregulation of long noncoding RNA TUG1 promotes cervical cancer cell proliferation and migration. Cancer Med. 2017;6(2):471–482. doi:10.1002/cam4.994.
- Skog J, Wurdinger T, van Rijn S, Meijer DH, Gainche L, Sena-Esteves M, Curry WT Jr., Carter BS, Krichevsky AM, Breakefield XO. Glioblastoma microvesicles transport RNA and proteins that promote tumour growth and provide diagnostic biomarkers. Nat Cell Biol. 2008;10:1470–1476. doi:10.1038/ncb1800.
- Pisitkun T, Shen RF, Knepper MA. Identification and proteomic profiling of exosomes in human urine. Proc Natl Acad Sci U S A. 2004;101:13368–13373. doi:10.1073/pnas.0403453101.
- Lasser C, Alikhani VS, Ekstrom K, Eldh M, Paredes PT, Bossios A, Sjostrand M, Gabrielsson S, Lotvall J, Valadi H. Human saliva, plasma and breast milk exosomes contain RNA: uptake by macrophages. J Transl Med. 2011;9:9. doi:10.1186/1479-5876-9-9.
- Xiao D, Ohlendorf J, Chen Y, Taylor DD, Rai SN, Waigel S, Zacharias W, Hao H, McMasters KM. Identifying mRNA, microRNA and protein profiles of melanoma exosomes. PLoS One. 2012;7:e46874. doi:10.1371/journal.pone.0046874.
- Gezer U, Ozgur E, Cetinkaya M, Isin M, Dalay N. Long non-coding RNAs with low expression levels in cells are enriched in secreted exosomes. Cell Biol Int. 2014;38:1076–1079. doi:10.1002/cbin.10301.
- Diken M, Kreiter S, Selmi A, Britten CM, Huber C, Tureci O, Sahin U. Selective uptake of naked vaccine RNA by dendritic cells is driven by macropinocytosis and abrogated upon DC maturation. Gene Ther. 2011;18:702–708. doi:10.1038/gt.2011.17.
- Hsu YL, Hung JY, Chang WA, Lin YS, Pan YC, Tsai PH, Wu CY, Kuo PL. Hypoxic lung cancer-secreted exosomal miR-23a increased angiogenesis and vascular permeability by targeting prolyl hydroxylase and tight junction protein ZO-1. Oncogene. 2017;36:4929–4942. doi:10.1038/onc.2017.105.
- Appleby P, Beral V, Berrington de Gonzalez A, Colin D, Franceschi S, Goodhill A, Green J, Peto J, Plummer M, Sweetland S. Cervical cancer and hormonal contraceptives: collaborative reanalysis of individual data for 16,573 women with cervical cancer and 35,509 women without cervical cancer from 24 epidemiological studies. Lancet (London, England). 2007;370:1609–1621.
- Ding X, Jia X, Wang C, Xu J, Gao SJ, Lu C. A DHX9-lncRNA-MDM2 interaction regulates cell invasion and angiogenesis of cervical cancer. Cell Death Differ. 2018;6:1750-1765. doi:10.1038/s41418-018-0242-0.
- Li H, Jia Y, Cheng J, Liu G, Song F. LncRNA NCK1-AS1 promotes proliferation and induces cell cycle progression by crosstalk NCK1-AS1/miR-6857/CDK1 pathway. Cell Death Dis. 2018;9:198. doi:10.1038/s41419-017-0249-3.
- Guo P, Zhang G, Meng J, He Q, Li Z, Guan Y. Upregulation of long noncoding RNA TUG1 promotes bladder cancer cell proliferation, migration, and invasion by inhibiting miR-29c. Oncol Res. 2018;26:1083–1091. doi:10.3727/096504018X15152085755247.
- Jiang X, Yan Y, Hu M, Chen X, Wang Y, Dai Y, Wu D, Wang Y, Zhuang Z, Xia H. Increased level of H19 long noncoding RNA promotes invasion, angiogenesis, and stemness of glioblastoma cells. J Neurosurg. 2016;2016:129–136. doi:10.3171/2014.12.JNS1426.test.
- Rivera LB, Bergers G. Tumor Angiogenesis, from Foe to Friend. Science (New York, NY). 2015;349:694–695. doi:10.1126/science.aad0862.
- Weis SM, Cheresh DA. Tumor angiogenesis: molecular pathways and therapeutic targets. Nat Med. 2011;17:1359–1370. doi:10.1038/nm.2537.
- Gordon MS, Mendelson DS, Kato G. Tumor angiogenesis and novel antiangiogenic strategies. International Journal of Cancer. 2010;126:1777–1787. doi:10.1002/ijc.25026.
- Xue G, Yan HL, Zhang Y, Hao LQ, Zhu XT, Mei Q, Sun SH. c-Myc-mediated repression of miR-15-16 in hypoxia is induced by increased HIF-2alpha and promotes tumor angiogenesis and metastasis by upregulating FGF2. Oncogene. 2015;34:1393–1406. doi:10.1038/onc.2014.82.
- Tate MC, Aghi MK. Biology of angiogenesis and invasion in glioma. Neurotherapeutics. 2009;6:447–457. doi:10.1016/j.nurt.2009.04.001.
- Liu Y, Carson-Walter EB, Cooper A, Winans BN, Johnson MD, Walter KA. Vascular gene expression patterns are conserved in primary and metastatic brain tumors. J Neurooncol. 2010;99:13–24. doi:10.1007/s11060-009-0105-0.
- Qiu JJ, Lin XJ, Tang XY, Zheng TT, Lin YY, Hua KQ. Exosomal metastasis‑associated lung adenocarcinoma transcript 1 promotes angiogenesis and predicts poor prognosis in epithelial ovarian cancer. Int J Biol Sci. 2018;14:1960–1973. doi:10.7150/ijbs.28048.
- Niu Y, Ma F, Huang W, Fang S, Li M, Wei T, Guo L. Long non-coding RNA TUG1 is involved in cell growth and chemoresistance of small cell lung cancer by regulating LIMK2b via EZH2. Mol Cancer. 2017;16:5. doi:10.1186/s12943-016-0575-6.
- Huang MD, Chen WM, Qi FZ, Sun M, Xu TP, Ma P, Shu YQ. Long non-coding RNA TUG1 is up-regulated in hepatocellular carcinoma and promotes cell growth and apoptosis by epigenetically silencing of KLF2. Mol Cancer. 2015;14:165. doi:10.1186/s12943-015-0431-0.
- Zhang E, He X, Yin D, Han L, Qiu M, Xu T, Xia R, Xu L, Yin R, De W. Increased expression of long noncoding RNA TUG1 predicts a poor prognosis of gastric cancer and regulates cell proliferation by epigenetically silencing of p57. Cell Death Dis. 2016;7:e2109. doi:10.1038/cddis.2015.356.
- Cai H, Liu X, Zheng J, Xue Y, Ma J, Li Z, Xi Z, Li Z, Bao M, Liu Y. Long non-coding RNA taurine upregulated 1 enhances tumor-induced angiogenesis through inhibiting microRNA-299 in human glioblastoma. Oncogene. 2016;36:318-331. doi:10.1038/onc.2016.212.