ABSTRACT
Cyclin-dependent kinase (Cdk) in complex with a corresponding cyclin plays a pivotal role in neurogenic differentiation. In particular, Cdk4 activity acts as a signaling switch to direct human mesenchymal stem cells (MSCs) to neural transdifferentiation. However, the molecular evidence of how Cdk4 activity converts MSCs to neurogenic lineage remains unknown. Here, we found that Cdk4 inhibition in human MSCs enriches the populations of neural stem and progenitor pools rather than differentiated glial and neuronal cell pools. Interestingly, Cdk4 inhibition directly inactivates Smads and subsequently STAT3 signaling by hypophosphorylation, and both Cdk4 and Smads levels are linked during the processes of neural transdifferentiation and differentiation. In summary, our results provide novel molecular evidence in which Cdk4 inhibition leads to directing human MSCs to a multipotent neurogenic fate by inactivating Smads-STAT3 signaling.
Introduction
The differentiation fate of stem cells is tightly tied to cell cycle regulation, which is mediated by a cyclin-dependent kinase (Cdk) in a complex with its corresponding cyclin. During neurogenesis, both Cdk and cyclin play a pivotal role in the initial phases of neuronal development when neural stem and progenitor cells commit to neuronal fate and withdraw from the cell cycle and begin to differentiate.Citation1-4 Interestingly, recent studies have provided evidence that cell cycle length is itself a regulator of the balance between progenitor maintenance and differentiation,Citation2,5 although the mechanism for this regulation is unknown. For example, Cdk2 and Cdk4 double knock-out NSCs displayed a reduction of the S phase length and were more prone to increase the tendency to neurogenic differentiation.Citation2 In contrast, the overexpression of Cdk4 and cyclinD1 in NSCs shortens G1 phase length and delays neurogenesis, but promotes the generation and expansion of progenitor pools.Citation1 In addition, the higher proportion of neurogenic divisions in the cortical regions is characterized by progenitors with a longer G1 population of cell cycle.Citation1,6 Similarly, it has been reported that neurogenic progenitors are characterized by a longer G1 than the other coexisting proliferative progenitors,Citation1,7 indicating a tight correlation between regulation of cell cycle arrest and neurogenesis.
Several forms of evidence indicate that human mesenchymal stem cells (hMSCs) can differentiate into mesodermal, ectodermal, and endodermal cell types.Citation8-10 Evidence also suggests that hMSCs have the potential to become neural cells, but commitment to these lineages depends on the culture conditions.Citation8,10,11 In addition to neural morphologic differentiation, selected neural induction media functionally convert hMSCs to typical neuronal and glial cells,Citation11,12 suggesting the possibility of controlled neural differentiation of human MSCs as a source of cells for cell therapy of neurodegenerative diseases. We have recently reported that the activity of Cdk4 was required for syngenic differentiation, and the loss of Cdk4 activity or expression selectively led to reversible neural trans-differentiation.Citation13 This study indicated that the regulation of Cdk4 activity may be a key signaling device to confer hMSCs for neural trans-differentiation and reversible de-differentiation, although the molecular details on how the inhibition of Cdk4 activity converts hMSCs to neurogenic lineage remains unknown.
Developmentally, activation of Smad1/5/9 (also known as Smad8) and STAT3 signaling are important for determining distinct cell fates in the stem cells plate.Citation14-16 Smad1/5/9 signaling actively instructs the development and differentiation of distinct neural lineages from human pluripotent stem cells and neural stem cells at the border between the epidermis and the neural plate.Citation15,17 In addition, differentiation into a neural lineage can be induced by the IL-6 family of cytokines [e.g., leukemia inhibitory factor (LIF) and ciliary neurotrophic factor and bone morphogenic protein (BMP) family cytokines (e.g., BMP2 and BMP4)], which activate distinct downstream transcription factors such as STAT3 and Smad1.Citation14,16,18,19 The receptor kinases, such as TGF-β and BMP, activate Smad1 and STAT3 signaling by phosphorylation as phosphorylation plays a central role in their transcriptional activation bridged by p300.Citation16,20 Interestingly, the inhibition of tyrosine phosphorylation of STAT3 (at Y705 residue) is mediated by the Smad4-dependent TGF-β signaling.Citation21,22 Phosphorylation of Smad3 is also decreased by the inhibition of JAK2/STAT3 signaling, and thus establishing an additional functional link between STAT and Smad proteins.Citation21,22
Although activation of Smads and STAT3 signaling seems to be critical for fate decision of stem cells, it is important to identify whether this signaling is also fate determining in transdifferentiation of hMSCs. In the present study, our systemic fluorescence-activated cell sorting (FACS) and biochemical analyses unexpectedly revealed that Cdk4 inhibition allowed for changing of hMSCs into a multipotent neural stem and progenitor lineage rather than inducing differentiation into distinct neuronal or glial cells. Also Cdk4 inhibition inactivated Smads-STAT3 signaling by inducing hypophosphorylation. Together, this study indicates that Cdk4 inhibition appears to be cell fate determining with transdifferentiation of hMSCs through Smads-STAT3 signaling.
Result
Cdk4 inhibition results in commitment of human mesenchymal stem cells into a multipotent neural lineage
To specify the neurogenic processes that are induced by Cdk4 inhibition-mediated transdifferentiation, human adipose-derived mesenchymal stem cells (hereafter hMSCs) were characterized by specific surface antigen expressions. Previously, treatment of selective Cdk4 inhibitor efficiently led to the transdifferentiation of hMSCs to neural cells morphologically, as indicated by the cell body condensing and becoming increasingly spherical and retractile ().Citation13 We have observed similar neural transdifferentiation of hMSCs by treatment with 2 different Cdk4 inhibitors (Fig. S1A and S1B). Here, we unexpectedly found that cells resulting from Cdk4 inhibition of hMSCs still had surface markers representing multipotency, such as CD44, CD90, CD105, and CD73 (90.8%, 58.1%, 76.4%, and 88.6% cell surface expression compared with original cells, respectively).Citation23,24 The comparisons used isotype antibody controls, Cdk4 inhibition slightly reduced the relative levels of these surface marker expressions compare to the DMSO control (CD44 96.1%, CD90 95.2%, CD105 96.4% and CD73 96.2%, respectively) ( and 1C). These data raise an important possibility that transdifferentiation process of hMSCs by Cdk4 inhibition results in shifting to a neurogenic lineage rather than a terminal differentiation.
Figure 1. The expression pattern of multipotent MSC markers following Cdk4 inhibition. (A) Phase contrast image of human adipose-derived MSCs (hMSCs) were treated with 10 μM CDK4i or DMSO for 24 h. (B) hMSCs were labeled with antibodies specific for CD44, CD73, CD90 and CD105 antibodies or immunoglobulin isotype control. The surface phenotype was analyzed by FACS. Gray-colored dot histograms illustrate the control immunoglobulin and black-colored open histograms illustrate the specific antibodies for DMSO control, as indicated. Red-colored open histograms represent the staining against each specified antibodies of the Cdk4 inhibitor treated hMSCs. (C) The graph shows that percentages of positive cells in each cell subset shown in B.
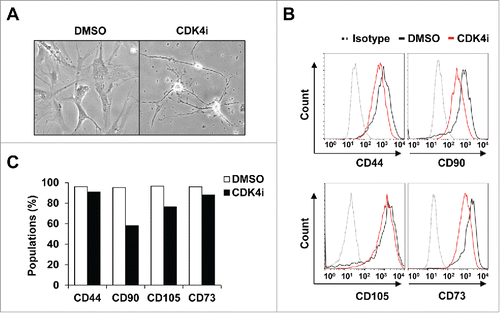
Cdk4 inhibition shifts hMSCs to neural lineage by producing a progenitor pool
To further characterize the stages of neural lineage switch by inhibiting Cdk4 activity, we examined the representative marker expressions of stem and neural cells, Nanog, Oct3/4, Sox2 and CD15 (SSEA-1) for neural stem cells, Nestin for neural stem/progenitor cells, and DCX for neuronal progenitor and immature cells, βIII tubulin, MAP2b and NeuroD for immature/mature neuron cells, GFAP for neural stem/glial cells, and PCNA and Ki-67 for proliferating cells (). Interestingly, we found that neurogenic hMSCs by Cdk4 inhibition still showed the expression of marker portfolio which related to the stages of neural stem and progenitor pools, such as Nanog, Oct3/4, Sox2, CD15, SSEA-3, TRA-1-81 and Nestin (). Cdk4 inhibition also led to induction of GFAP expression, which is considered as both a neural stem/progenitor and a glial marker (). In addition, Cdk4 inhibition increased MAP2b and NeuroD expression but not DCX and βIII tubulin among immature and mature neuronal marker proteins.
Figure 2. Cdk4 inhibition is enriching neural stem and progenitor pool. (A) Characterized marker proteins of neural stem and progenitor, immature/mature neuron and glial cells were categorized according to the various stages of neural development. GFAP (underlined) are used as both early stem/progenitor and glial marker. (B) Surface and intracellular markers were stained with specific antibodies and analyzed by flow cytometry. Black-colored open histograms illustrate the specific antibodies for DMSO control, as indicated. Red-colored open histograms represent the staining against each specified antibodies of the Cdk4 inhibitor-treated hMSCs and gray-colored dot histograms illustrate the control immunoglobulin. (C) The graph shows that percentages of neural lineage markers positive cells treated with Cdk4 inhibitor compared to the control DMSO-treated cells in B. (D) Relative expressions of mRNA transcripts were amplified by qRT-PCR. mRNA expression of each gene was normalized to 18S mRNA.
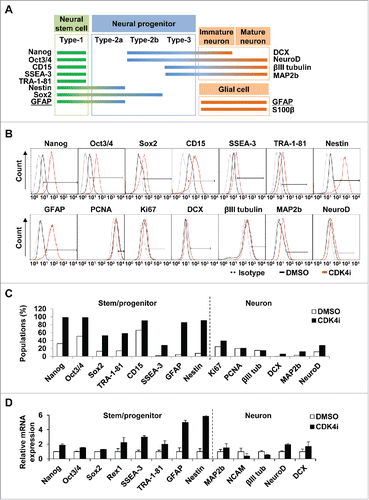
The relative percentages of neural marker expression by Cdk4 inhibition was measured and compared to the isotype antibody control (). Additional qRT-PCR analysis showed similar results in that Cdk4 inhibition increased the levels of neural stem and progenitor markers but not immature and mature neuronal markers (). Together, these results indicate that Cdk4 inhibition seems to generate the populations of neural stem and progenitor cells rather than terminally differentiated neuronal or glial cells.
Cdk4 inhibition of hMSCs leads to dominance of astroglial progenitors
To further specify the distinct stages of neurogenic pools induced by Cdk4 inhibition, we examined the populations of cell phenotypes with CD184+/CD271−/CD44−/CD24+-gated cells (a marker for NSCs), CD184+/CD44+-gated cells (a marker for glia cells) or CD184−/CD44−/CD15Low/CD24+-gated cells (a marker for neuronal cells) phenotype ( and 3B). We have to note that CD44 expression is also used as a universal marker for multipotent MSCs.Citation24 Interestingly, our FACS screening analysis revealed that Cdk4 inhibition in hMSCs promoted the expansion of CD184+/CD271−/CD44−/CD24+-gated cells (NSCs, 16.88%) and CD184+/CD44+-gated cells (glia, 24.5%) compared to the control DMSO treatment, whereas CD184−/CD44−/CD15Low/CD24+-gated cells (neuron, 0.42%) remained unexpanded (). Also hMSCs treated with Cdk4 inhibitor or DMSO controls were negative for CD271 expression (data not shown). Thus, Cdk4 inhibition appears to cause a shift of hMSCs into neural stem and progenitor pools by increasing the population of CD24+CD184+-gated cells.
Figure 3. Astroglial lineage commitment of hMSC by Cdk4 inhibition. (A) Categorization of neural lineage based on cell surface maker expressions. (B and C) Cells monitored on a flow cytometer using human neural lineage antibody set, CD184, CD.271, CD44 and CD24. As differentiation progressed, cells expressed CD184 high and CD44 high (universal MSC markers, ), but not expressed CD271. CD24 positive cells were co-expressed with CD184 only (B). Bar graph showing the percentage of cells that were expressed CD184 and/or CD24 cell surface marker (C). (D to I) Immunophenotypic characterization by flow cytometry to quantify marker expressions followed antibodies double staining of GFAP/Nestin antibodies (D), A2B5/Nestin antibodies (F), and A2B5/GFAP antibodies (H). First column shows isotype control gated cells, the second column shows DMSO gated cells, and third column shows Cdk4 activity-inhibited hMSCs. (E, G and I) Graphic represent cell percentage of gated population in D, F, and H respectively. (J and K) Cells were stained with CD140α antibody and control immunoglobulin and analyzed by flow cytometry.
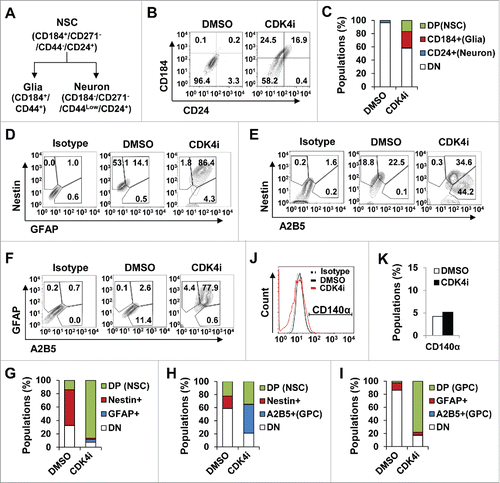
To further define the specific neural progenitor lineage, we examined expression of glial progenitor marker A2B5, oligodendrocyte progenitor marker CD140α, astrocyte marker GFAP, and typical neural stem/progenitor marker Nestin by flow cytometry ().Citation25 GFAP and Nestin double positive populations (neural stem and progenitor cells) were significantly increased by Cdk4 inhibition ( and Fig. S1C). However, the populations of Nestin and A2B5 double positive cells (neural stem cells) were relatively less than those of A2B5 single positive cells ( and 3G). Moreover, most of the GFAP-expressing cells also were positive for A2B5 expression in the Cdk4 inhibited hMSCs ( and 3I). Interestingly, the populations of CD140α-gated cells were not significantly changed by Cdk4 inhibition ( and 3K). Together, these results suggest that Cdk4 inhibition bring about a switch in hMSCs to neural stem and progenitor cells that have a differentiating tendency into astrocyte lineage.
Neural fate change of hMSCs is determined by Cdk4 inhibition-mediated hypophosphorylation of Smads and STAT3
Cytokines and growth factors play critical roles in the cell fate specification of embryonic stem cells and neural stem cells. Particularly, it has been proposed that cross talk between Smad and STAT signaling pathways play a critical role in glial and neuronal development.Citation15,16 In addition, the STAT3-mediated activation of Smad signaling and vice versa by BMPs and IL-6 family cytokines seems to drive neural stem cells into the astrogliogenic fate,Citation14,26 indicating that the activation of Smads-STAT3 signaling may be a critical process in deciding the ultimate fate of embryonic and neural stem cells.
To examine the molecular mechanism of which induces the multipotent neurogenic fate of hMSCs by Cdk4 inhibition, extracts from cells treated with control DMSO or selective Cdk4 inhibitor in the presence of serum were separated into cytoplasmic and nuclear fractions. These fractions were then analyzed by immunoblotting with anti-Smad2, anti-p-Smad2 S465/467 (phosphorylated on residues S465 and S467), anti-Smad5, anti-p-Smad1/5/9 (phosphorylated on Smad1 S463 and S465, Smad5 S463 and S465, and Smad9 S465 and S467), anti-STAT3, anti-p-STAT3 (phosphorylated on residue Y705), anti-GAPDH (a marker for cytoplasmic fraction), and anti-lamin B1 (a marker for nuclear fraction). As shown in , we unexpectedly found that the inhibition of Cdk4 activity showed a marked reduction of phosphorylated STAT3 levels rather than increasing these levels, whereas the levels of phosphorylated Smad2 and Smad1/5/9 were unchanged compared to control treatment.
Figure 4. Cdk4 inhibition results in the hypophosphorylations of Smads and subsequently STAT3. (A) Extracts from hMSCs treated with control DMSO or selective Cdk4 inhibitor in the presence of serum were separated into cytoplasmic and nuclear fractions. Each fraction was analyzed by SDS-PAGE and immunoblotted with anti-Smad2, anti-p-Smad2 S465/467 (phosphorylated on residues S465 and S467), anti-Smad5, anti-p-Smad1/5/9 (phosphorylated on Smad1 S463 and S465, Smad5 S463 and S465, and Smad9 S465 and S467), anti-STAT3, anti-p-STAT3 (phosphorylated on residue Y705), anti-GAPDH (a marker for cytoplasmic fraction), and anti-lamin B1 (a marker for nuclear fraction) antibodies. (B and C) hAd-MSCs were grown in serum-starved condition for 24 h and then treated with 10 μM Cdk4 inhibitor or a control DMSO in combination with dose-dependently increasing amounts of BMP4 (0, 1, 5, 10, 20, 100 ng/ml) for additional 24 h incubation. Lysates were immunoblotted with anti-Smad5 and anti-p-Smad1/5/9 (phosphorylated on Smad1 S463 and S465, Smad5 S463 and S465, and Smad9 S465 and S467) antibody. (D) Extracts from glioblastoma cell lines, U87, U251 and U373, human neural stem cells (NSC), human MSCs originated from umbilical cord blood (UBC), bone marrow (BM) and adipose tissue (Ad), and hAd-MSCs treated with Cdk4 inhibitor (CDK4i) or forskolin/bFGF (F/F) were analyzed by immunoblotting using anti-Cdk4, anti-p-Smad1/5/9, anti-Smad3, and anti-actin antibodies. (E and F) Purified GST-Smad proteins or GST-STAT3 were incubated with Cdk4/cyclin D1 complex in the presence of [γ-32P] ATP. *represent nonspecific band. Lines indicate Cdk4/cyclin D1 recombinant proteins.
![Figure 4. Cdk4 inhibition results in the hypophosphorylations of Smads and subsequently STAT3. (A) Extracts from hMSCs treated with control DMSO or selective Cdk4 inhibitor in the presence of serum were separated into cytoplasmic and nuclear fractions. Each fraction was analyzed by SDS-PAGE and immunoblotted with anti-Smad2, anti-p-Smad2 S465/467 (phosphorylated on residues S465 and S467), anti-Smad5, anti-p-Smad1/5/9 (phosphorylated on Smad1 S463 and S465, Smad5 S463 and S465, and Smad9 S465 and S467), anti-STAT3, anti-p-STAT3 (phosphorylated on residue Y705), anti-GAPDH (a marker for cytoplasmic fraction), and anti-lamin B1 (a marker for nuclear fraction) antibodies. (B and C) hAd-MSCs were grown in serum-starved condition for 24 h and then treated with 10 μM Cdk4 inhibitor or a control DMSO in combination with dose-dependently increasing amounts of BMP4 (0, 1, 5, 10, 20, 100 ng/ml) for additional 24 h incubation. Lysates were immunoblotted with anti-Smad5 and anti-p-Smad1/5/9 (phosphorylated on Smad1 S463 and S465, Smad5 S463 and S465, and Smad9 S465 and S467) antibody. (D) Extracts from glioblastoma cell lines, U87, U251 and U373, human neural stem cells (NSC), human MSCs originated from umbilical cord blood (UBC), bone marrow (BM) and adipose tissue (Ad), and hAd-MSCs treated with Cdk4 inhibitor (CDK4i) or forskolin/bFGF (F/F) were analyzed by immunoblotting using anti-Cdk4, anti-p-Smad1/5/9, anti-Smad3, and anti-actin antibodies. (E and F) Purified GST-Smad proteins or GST-STAT3 were incubated with Cdk4/cyclin D1 complex in the presence of [γ-32P] ATP. *represent nonspecific band. Lines indicate Cdk4/cyclin D1 recombinant proteins.](/cms/asset/320d46d0-25cc-4194-a11a-92981bd5abf5/kccy_a_1188230_f0004_b.gif)
Next, to clarify the effect of Cdk4 inhibition on the hypophosphorylation of Smads in response to BMP4, which activates downstream STAT3 and Smads signaling,Citation14 hMSCs were treated with BMP4 in combination with Cdk4 inhibitor (), and separated into cytoplasmic (Cyt) and nuclear (Nuc) fractions (). hMSCs were treated with increasing amounts of BMP4 (0, 1, 5, 10, 20, 100 ng/ml) and cultured in serum-starved condition. The control DMSO treatment gradually increased the phosphorylation levels of Smad1/5/9, but the Cdk4 inhibitor treatment drastically reduced the levels of Smad1/5/9 phosphorylation, which was triggered by BMP4 treatment. Furthermore, immunoblotting showed that Cdk4 inhibition clearly reduced levels of phosphorylated Smad1/5/9 in both cytoplasmic and nuclear fractions, whereas Cdk4 inhibition did not affect to the overall levels of Smad1/5/9 proteins. However, in the same experiment condition, we were unable to detect the level of STAT3 phosphorylation at Y705 residue (Fig. S2). Thus, our results indicates that Cdk4 inhibition inactivated Smads-STAT3 signaling by hypophosphorylation, and thus provide a novel regulatory mechanism in which Cdk4 inhibition confers hMSCs self-renewal or neurogenic switching with gliogenic tendency.
We also tested the Smad phosphorylation changes in other cell types as well; these included other types of hMSCs, neural stem cells (NSCs), glioblastoma cells, and hMSCs in presence of Cdk4 inhibitor or forskolin/bFGF (F/F), which induces neuron-like cell differentiation ().Citation27 The levels of endogenous Cdk4 were very low in NSCs (lane 4) but markedly high in all glioblastoma cells tested (lanes 1-3), and we found a strong positive correlation between Cdk4 level and Smad1/5/9 phosphorylation in these cells. In all three different types of hMSCs, there were high levels of Cdk4 with concomitant Smad1/5/9 phosphorylations (). The treatment with Cdk4 inhibitor on all three types of hMSCs significantly reduced the levels of phosphorylated Smad1/5/9, whereas those with F/F treatment had no impact (compare lanes 9 to 7 and 8). Together, these results suggest that Cdk4 activity is correlated with the phosphorylation state of Smad family, and the sequential regulation of Cdk4 activity seems to be a critical event for the neurogenic fate decision of hMSCs.
Next, we examined whether Cdk4 directly phosphorylates Smad proteins. To do so, 293T cells were transfected with Myc epitope-tagged Smad family expression plasmids (Myc-Smad1, -Smad3, -Smad4, -Smad5 and -Smad9), and lysates were immunoprecipitated and immunoblotted as indicated (Fig. S3A). From these experiments, Cdk4 seems to complex with Smad3 and Smad4 (weakly) and Smad9 (strongly). Next, we generated GST-fused Smad family (G-Smad1, -Smad2, -Smad3, -Smad4, -Smad5 and -Smad9) and incubated it with recombinant human Cdk4/cyclin D1 complex (, Fig. S3B). In vitro kinase assay revealed that Smad1, SMad3, Smad5 and Smad9 were efficiently phosphorylated by Cdk4/cyclin D1, whereas Smad2 and Smad4 were very weakly phosphorylated (). Additionally, we found that Cdk4/cyclin D1 phosphorylated GST-fused STAT3 but not a control GST in condition that the phosphorylation of GST-fused Rb by Cdk4/cyclin D1 (a positive control) was sharply reduced in presence of Cdk4 inhibitor (Fig. S3D). Although we failed to see a clear interaction between Cdk4 and Smad5, Smad5 was strongly phosphorylated by Cdk4/cyclin D1. Together, Cdk4 inhibition directly leads to hypophosphorylation of Smad and subsequently STAT3, and promotes the differentiation pathway of hMSCs to a multipotent neural lineage.
Discussion
The temporal and spatial controls of cell cycle regulate the balance between expansion, symmetric and asymmetric division, and changes in the pools of neural stem and precursor cells. In particular, Cdks and cyclins play a pivotal role in the neurogenic differentiation of a variety of stem cells. Cells withdraw from the cell cycle by inhibition of Cdks and cyclins. In stem cells, inhibition of Cdks and cyclins leads to differentiation to distinct neurogenic cell fates.Citation1-4 There is an also role for Smad-STAT3 as their phosphorylation and activation has been known as a key signaling process of syngenic neural differentiation.
In the present study, we unexpectedly found that Cdk4 inhibition during transdifferentiation of hMSCs led to a marked reduction of phosphorylations of Smads and STAT3 transcription factors probably from onset of hypophosphorylations of Smad1, Smad3, Smad5, Smad9 and STAT3. We found that this led to a switch of hMSCs to a multipotent neural stem and progenitor lineage. We additionally found that Cdk4 is maintained at high levels in mesenchymal stem cells and by comparison it is at low levels in differentiated neural cells (shown by Cdk4 inhibitor treatments and using F/F treatment as a control), indicating that the regulation of Cdk4 level seems to be a critical determinant for MSCs committing to either self-renewal or multipotent neurogenic transdifferentiation. In addition, we saw that Cdk4 activity/level appears to be periodically regulated during neurogenesis: NSCs had very low levels of Cdk4, but glial differentiation seemed to have reactivation and/or recovery of Cdk4. The mechanism on how the Cdk4 activity/level is regulated during differentiation and transdifferentiation may need to be explored further. This study provides novel evidence that the sequential regulation of Cdk4 activity along with Smads-STAT3 signaling leads to determination of neurogenic fate of hMSCs during transdifferentiation.
It has been known that Cdk4-mediated phosphorylation of Rb and Smad proteins are important in regulating their antiproliferating and differentiating signals.Citation28-30 Dysregulation by genetic or epigenetic activation of core pathway components of Smad-STAT signaling results in increased cell proliferation and decreased apoptosis and differentiation, which may ultimately lead to many disorders. Smad1 and STAT3 signaling play important roles in directing proliferation and fate decisions for stem cells. In particular, canonical Smad1 and STAT3 pathway regulates the expression of different sets of target genes, which are involved in directing stemness potency and/or cell fate, such as Nanog and Nestin.Citation14,31-33 However, our results clearly showed that fate shifting of hMSCs to neural lineage is accompanied by inactivation of Smads-STAT3 signaling. How these two opposite functions of Smads-STAT3 signaling are integrated with differentiation and transdifferentiation, respectively? One possibility is that the transcriptional activation of downstream target genes by Smads-STAT3 signaling may produce specific subsets of genes, which are essential for inducing neural differentiation pathways, such as Nanog, NeuroD, Nestin, Math1, and GFAP. However, inactivation of Smads-STAT3 signaling may exclusively affect maintenance of multipotency and self-renewal of MSCs by avoiding Smads-STAT3-mediated transcriptional activation. Indeed, a recent study has suggested that the transcriptional activation of Smads-Oct4 complex prevents differentiation by suppressing expression of mesoderm markers, and thus maintaining pluripotency of mouse ES cells.Citation34 Otherwise, it is likely that Cdk4 inhibition may affect another signaling pathway to activate the set of genes required for neural fate shifting and inducing Nanog, Nestin, Sox2 and GFAP.
There is increasing evidence that stem cells continuously integrate intrinsic and extrinsic signals to make fate decisions regarding specific differentiation lineages and self-renewal.Citation26 These fate choice mechanisms have increasing importance for understanding development as well as designing novel therapeutic treatments concerning stem cells for various ailments. Therefore, Cdk signaling, in particular Cdk4 signaling, will offer new insight into how human MSCs can be directed into a neural cell lineage and may provide for a new target for the therapeutic application of neurologic diseases. Our data further provide for a model of how hMSCs utilize Smad-STAT signaling in 2 different contexts: for mesenchymal stem cell maintenance and for transdifferentiation and selecting cell fates. However, further studies are required regarding on how Smads-STAT3 signaling is manipulated with respect to Cdks, particularly Cdk4, during differentiation and transdifferentiation.
In summary, this study explores how mesenchymal stem cells regulate their stemness and cell fate with respect to neuroectoderm lineage via affecting Smads-STAT3 via Cdk4 kinase activity. From our study, selective Cdk4 inhibition of human MSCs may be a new pathway for supplying neural progenitor pools and may have applications for novel therapeutics for neurogenic diseases.
Materials and methods
Cell culture
This study was approved by the Institutional Review Board (IRB) at Kangbuk Samsung Hospital of the Sungkyunkwan University School of Medicine. For all liposuctioned tissues used herein, we obtained written informed consent from all donors prior to surgery. hAd-MSCs were isolated from adipose tissue using methods previously described,Citation35 with minor modifications.Citation13 Human MSCs from bone marrow (hBM-MSCs) were isolated from human bone marrow aspirates using methods previously described.Citation23 Human MSCs from umbilical cord matrix (hUCB-MSCs) were purchased from PromoCell. hMSCs were grown in low glucose DMEM, 10% FBS and 1% Pen/Strep. 293T, U87, U251 and U373 cells were grown in high glucose DMEM, 10% FBS and 1% Pen/Strep. Human neural stem cells (NSC) (H9 derived, Gibco) were grown on CELLstart (Gibco) coated dish with StemPro NSC SFM (KnockOut DMEM/F12, StemPro neural supplement, hEGF, hFGFb, Glutamax and 1% Pen/Strep) medium according to the manufacturer's protocol.
Inhibition of Cdk4 activity was obtained by treatment of CDK4 inhibitor (CDK4i, Otava, #7070707035), CDK4 inhibitor III (CDK4i III, Santa Cruz, #sc-202988), or CDK4/6 inhibitor IV (CDK4/6i IV, Santa Cruz, #sc-203874).
Immunoblotting and immunoprecipitation
Cells for the immunoblots were lysed in RIPA buffer (50 mM Tris-HCl pH 8.0, 150 mM NaCl, 1 mM EDTA, 1% Triton X-100, 0.1% SDS, 1% NP-40, 1% Sodium deoxycholate, 1 mM DTT, 1 mM PMSF and protease inhibitor cocktail), and the lysates were clarified by centrifugation at 13,200 rpm for 30 min. Equal amounts of protein were resolved by SDS-PAGE, transferred to nitrocellulose filters, blocked, and analyzed with antibodies.
For immunoprecipitation from total cell extracts, cells were resuspended in RIPA buffer, incubated at 4°C for 30 min, sonicated and centrifuged at 14,000 rpm for 30 min. The supernatants were obtained from 1 mg of lysates and incubated with antibodies overnight at 4°C.
FACS analyses
For surface staining, cells were pre-incubated with BD Human Fc Block (BD biosciences) for 10 min and for surface staining with the listed antibodies in FACS buffer (0.1% BSA and 0.1% sodium azide in PBS) for 30 min on ice. For intracellular staining, cells were fixed using BD Fixation buffer (or 4% paraformaldehyde) for 20 min at room temperature, and were washed with FACS buffer. The cells were then permeabilized using BD Perm buffer III for 30 min on ice. After washing with FACS buffer, cells were incubated with the listed antibodies. Primary antibodies used were for Nanog, Oct3/4, SSEA-1, SSEA-3, TRA-4-71, Ki-67, Doublecortin (DCX), Sox-1, Sox-2, Nestin, GFAP, CD24, CD271, CD15, CD184, A2B5, NeuroD1, PCNA, βIII Tub, MAP2b, CD57 CD140a, CD90, CD44, CD73, and CD105. The MSC negative cocktail were for CD34, CD11b, CD19, CD45, HLA-DR. Secondary antibodies used Streptavidin-PE. All the antibodies were from BD Biosciences, San Jose CA, USA. The cells were suspended with FACS buffer and analyzed on FACS Aria III SORP (BD Biosciences). Analyses were done using FlowJo (http://flowjo.com).
Polymerase chain reaction (PCR)
Total RNA was extracted with the RNeasy Mini Kit (Qiagen) and quantified by UV spectroscopy. To prepare RNA for PCR analysis, 1.2 μg of RNA were converted to cDNA using the ImProm-II Reverse Transcription System (Promega). The qRT-PCR reactions were performed with the SYBR Green PCR Master Mix on a MicroAmp optical 96-well reaction plate (Applied Biosystems). The mRNA expression of candidate genes was determined by real-time quantitative PCR using the AMI PRISM 7000 SDS (v1.1) instrument, according to the manufacturer's instructions (Applied Biosystems). The following specific primers were used for real-time PCR: 18s rRNA (5′-TCA ACT TTC GAT GGT AGT CGC CGT-3′/5′-TCC TTG GAT GTG GTA GCC GTT TCT-3′); NeuroD1 (5′-AGC CAC GGA TCA ATC TTC TC-3′/5′-ACT GTA AGC ACA GTG GGT TC-3′); DCX (5′-GGA AGG GGA AAG CTA TGT CTG-3′/5′-TTG CTG CTA GCC AAG GAC TG-3′); Nestin (5′-CGC TGG CGG GAG AAG C-3′/5′-GCC ACG CGC TCC TGG TA-3′); NCAM (5′-AGG ATC TCA TCT GGA CTT TG-3′/5′-CCA TCC AGA GTC TTT TCT TC-3′); GFAP (5′-TCG CTG GAG GAG GAG ATC C-3′/5′-GCG ATC TCG ATG TCC AGG G-3′); MAP2 (5′-TCA GAG GCA ATG ACC TTA CC-3′/5′-GTG GTA GGC TCT TGG TCT TT-3′); βIII Tub (5′-CTA CGA CAT CTG CTT CCG CA-3′/5′-CTT GCG CCG GAA CT GG-3′); Oct4 (5′-TCG AGA ACC GAG TGA GAG GC-3′/5′-CAC ACT CGG ACC ACA TCC TTC-3′); Sox2 (5′-CAC ACT GCC CCT CTC ACA CAT-3′/5′-CT TTC CCT CGT TTT TCT TTG AA-3′); Nanog (5′-CCA ACA TCC TGA ACC TCA GCT AC-3′/5′-GCC TTC TGC GTC ACA CCA TT-3′); Rex1 (5′-GCT GAC CAC CAG CAC ACT AGG C-3′/5′-TTT CTG GTG TCT TGT CTT TGC CCG-3′); Cdk4 (5′-CAT GTA GAC CAG GAC CTA AGC-3′/5′-AAC TGG CGC ATC AGA TCC TAG-3′); cyclinD1 (5′-TGG TGA ACA AGC TCA AGT GG-3′/5′-TGA CTC CAG CAG GGC TTC GA-3′); Bmp2 (5′-CCA GAA ACG AGT GGG AAA AC-3′/5′-AAG TCC ACG TAC AAA GGG TG-3′); Bmp4 (5′-ACT GGT CCA CCA CAA TGT GAC ACG-3′/5′-GCT GAA GTC CAC ATA GAG CGA GTG-3′).
Glutathione-S-transferase (GST) fusion proteins
The PCR fragments encoding the Smad genes were subcloned into pGEX-KG vector to generate GST-tagged fusion constructs; GST and GST-fusion proteins were generated in E. coli BL21 or Rosetta cells. Cells were resuspended in STE buffer (10 mM Tris-HCl pH 8.0, 150mM NaCl, 2% Triton X-100, 1 mM EDTA, 1mM PMSF, 2 mM DTT, 100 μg/ml lysozyme) and lysed by sonication. Lysates were centrifuged at 14,000 rpm for 30 min at 4°C, and the obtained supernatants were incubated with glutathione sepharose 4B beads (Incospharm) to purify the GST and GST-fusion proteins.
In vitro kinase assay
For the in vitro kinase assay, purified GST-fused proteins were washed with kinase buffer (25 mM Tris-HCl pH 7.5, 25 mM MgCl2, 2 mM DTT, 1 mM Na3VO4). The recombinant human Cdk4/cyclin D1 protein (Abcam) was used to phosphorylate substrates in 20 µl kinase buffer reactions with [γ-32P] ATP for 50 min at 30°C. The reaction was stopped by the addition of the SDS sample buffer and resolved by SDS–PAGE.
Disclosure of Potential Conflicts of Interest
No potential conflicts of interest were disclosed.
1188230_Supplemental_Material.zip
Download Zip (87.2 MB)Acknowledgments
Flow cytometric analysis and optical imaging system were performed in the Research Core Facility, Samsung Biomedical Research Institute.
Funding
This work was supported by the internal research grant of Center for Molecular Medicine, Samsung Biomedical Research Institute.
References
- Lange C, Huttner WB, Calegari F. Cdk4/cyclinD1 overexpression in neural stem cells shortens G1, delays neurogenesis, and promotes the generation and expansion of basal progenitors. Cell Stem Cell 2009; 5:320-31; PMID:19733543; http://dx.doi.org/10.1016/j.stem.2009.05.026
- Lim S, Kaldis P. Loss of Cdk2 and Cdk4 induces a switch from proliferation to differentiation in neural stem cells. Stem Cells 2012; 30:1509-20; PMID:22532528; http://dx.doi.org/10.1002/stem.1114
- Jablonska B, Aguirre A, Vandenbosch R, Belachew S, Berthet C, Kaldis P, Gallo V. Cdk2 is critical for proliferation and self-renewal of neural progenitor cells in the adult subventricular zone. J Cell Biol 2007; 179:1231-45; PMID:18086919; http://dx.doi.org/10.1083/jcb.200702031
- Artegiani B, Lindemann D, Calegari F. Overexpression of cdk4 and cyclinD1 triggers greater expansion of neural stem cells in the adult mouse brain. J Exp Med 2011; 208:937-48; PMID:21482697; http://dx.doi.org/10.1084/jem.20102167
- Ali F, Hindley C, McDowell G, Deibler R, Jones A, Kirschner M, Guillemot F, Philpott A. Cell cycle-regulated multi-site phosphorylation of Neurogenin 2 coordinates cell cycling with differentiation during neurogenesis. Development 2011; 138:4267-77; PMID:21852393; http://dx.doi.org/10.1242/dev.067900
- Lukaszewicz A, Savatier P, Cortay V, Giroud P, Huissoud C, Berland M, Kennedy H, Dehay C. G1 phase regulation, area-specific cell cycle control, and cytoarchitectonics in the primate cortex. Neuron 2005; 47:353-64; PMID:16055060; http://dx.doi.org/10.1016/j.neuron.2005.06.032
- Calegari F, Haubensak W, Haffner C, Huttner WB. Selective lengthening of the cell cycle in the neurogenic subpopulation of neural progenitor cells during mouse brain development. J Neurosci 2005; 25:6533-8; PMID:16014714; http://dx.doi.org/10.1523/JNEUROSCI.0778-05.2005
- Krabbe C, Zimmer J, Meyer M. Neural transdifferentiation of mesenchymal stem cells–a critical review. APMIS 2005; 113:831-44; PMID:16480453; http://dx.doi.org/10.1111/j.1600-0463.2005.apm_3061.x
- Lindvall O, Kokaia Z, Martinez-Serrano A. Stem cell therapy for human neurodegenerative disorders-how to make it work. Nat Med 2004; 10:S42-50; PMID:15272269; http://dx.doi.org/10.1038/nm1064
- Montzka K, Lassonczyk N, Tschoke B, Neuss S, Fuhrmann T, Franzen R, Smeets R, Brook GA, Woltje M. Neural differentiation potential of human bone marrow-derived mesenchymal stromal cells: misleading marker gene expression. BMC Neurosci 2009; 10:16; PMID:19257891; http://dx.doi.org/10.1186/1471-2202-10-16
- Munoz-Elias G, Woodbury D, Black IB. Marrow stromal cells, mitosis, and neuronal differentiation: stem cell and precursor functions. Stem Cells 2003; 21:437-48; PMID:12832697; http://dx.doi.org/10.1634/stemcells.21-4-437
- Nishino J, Yamashita K, Hashiguchi H, Fujii H, Shimazaki T, Hamada H. Meteorin: a secreted protein that regulates glial cell differentiation and promotes axonal extension. EMBO J 2004; 23:1998-2008; PMID:15085178; http://dx.doi.org/10.1038/sj.emboj.7600202
- Lee J, Baek JH, Choi KS, Kim HS, Park HY, Ha GH, Park H, Lee KW, Lee CG, Yang DY, et al. cyclin-dependent kinase 4 signaling acts as a molecular switch between syngenic differentiation and neural transdifferentiation in human mesenchymal stem cells. Cell Cycle 2013; 12:442-51; PMID:23324348; http://dx.doi.org/10.4161/cc.23308
- Fukuda S, Abematsu M, Mori H, Yanagisawa M, Kagawa T, Nakashima K, Yoshimura A, Taga T. Potentiation of astrogliogenesis by STAT3-mediated activation of bone morphogenetic protein-Smad signaling in neural stem cells. Mol Cell Biol 2007; 27:4931-7; PMID:17452461; http://dx.doi.org/10.1128/MCB.02435-06
- Hegarty SV, O'Keeffe GW, Sullivan AM. BMP-Smad 1/5/8 signalling in the development of the nervous system. Progress Neurobiol 2013; 109:28-41; ; http://dx.doi.org/10.1016/j.pneurobio.2013.07.002
- MuhChyi C, Juliandi B, Matsuda T, Nakashima K. Epigenetic regulation of neural stem cell fate during corticogenesis. Int J Dev Neurosci 2013; 31:424-33; PMID:23466416; http://dx.doi.org/10.1016/j.ijdevneu.2013.02.006
- Singh AM, Reynolds D, Cliff T, Ohtsuka S, Mattheyses AL, Sun Y, Menendez L, Kulik M, Dalton S. Signaling network crosstalk in human pluripotent cells: a Smad2/3-regulated switch that controls the balance between self-renewal and differentiation. Cell Stem Cell 2012; 10:312-26; PMID:22385658; http://dx.doi.org/10.1016/j.stem.2012.01.014
- Barnabe-Heider F, Wasylnka JA, Fernandes KJ, Porsche C, Sendtner M, Kaplan DR, Miller FD. Evidence that embryonic neurons regulate the onset of cortical gliogenesis via cardiotrophin-1. Neuron 2005; 48:253-65; PMID:16242406; http://dx.doi.org/10.1016/j.neuron.2005.08.037
- He F, Ge W, Martinowich K, Becker-Catania S, Coskun V, Zhu W, Wu H, Castro D, Guillemot F, Fan G, et al. A positive autoregulatory loop of Jak-STAT signaling controls the onset of astrogliogenesis. Nat Neurosci 2005; 8:616-25; PMID:15852015; http://dx.doi.org/10.1038/nn1440
- Alarcon C, Zaromytidou AI, Xi Q, Gao S, Yu J, Fujisawa S, Barlas A, Miller AN, Manova-Todorova K, Macias MJ, et al. Nuclear CDKs drive Smad transcriptional activation and turnover in BMP and TGF-β pathways. Cell 2009; 139:757-69; PMID:19914168; http://dx.doi.org/10.1016/j.cell.2009.09.035
- Zhao S, Venkatasubbarao K, Lazor JW, Sperry J, Jin C, Cao L, Freeman JW. Inhibition of STAT3 Tyr705 phosphorylation by Smad4 suppresses transforming growth factor β-mediated invasion and metastasis in pancreatic cancer cells. Cancer Res 2008; 68:4221-8; PMID:18519681; http://dx.doi.org/10.1158/0008-5472.CAN-07-5123
- Wrighton KH, Lin X, Feng XH. Phospho-control of TGF-β superfamily signaling. Cell Res 2009; 19:8-20; PMID:19114991; http://dx.doi.org/10.1038/cr.2008.327
- Majumdar MK, Keane-Moore M, Buyaner D, Hardy WB, Moorman MA, McIntosh KR, Mosca JD. Characterization and functionality of cell surface molecules on human mesenchymal stem cells. J Biomedical Sci 2003; 10:228-41; http://dx.doi.org/10.1007/BF02256058
- Pittenger MF, Mackay AM, Beck SC, Jaiswal RK, Douglas R, Mosca JD, Moorman MA, Simonetti DW, Craig S, Marshak DR. Multilineage potential of adult human mesenchymal stem cells. Science 1999; 284:143-7; PMID:10102814; http://dx.doi.org/10.1126/science.284.5411.143
- Sim FJ, McClain CR, Schanz SJ, Protack TL, Windrem MS, Goldman SA. CD140a identifies a population of highly myelinogenic, migration-competent and efficiently engrafting human oligodendrocyte progenitor cells. Nat Biotechnol 2011; 29:934-41; PMID:21947029; http://dx.doi.org/10.1038/nbt.1972
- Rajan P, Panchision DM, Newell LF, McKay RD. BMPs signal alternately through a SMAD or FRAP-STAT pathway to regulate fate choice in CNS stem cells. J Cell Biol 2003; 161:911-21; PMID:12796477; http://dx.doi.org/10.1083/jcb.200211021
- Jang S, Cho HH, Cho YB, Park JS, Jeong HS. Functional neural differentiation of human adipose tissue-derived stem cells using bFGF and forskolin. BMC Cell Biol 2010; 11:25; PMID:20398362; http://dx.doi.org/10.1186/1471-2121-11-25
- Kato J, Matsushime H, Hiebert SW, Ewen ME, Sherr CJ. Direct binding of cyclin D to the retinoblastoma gene product (pRb) and pRb phosphorylation by the cyclin D-dependent kinase CDK4. Genes Dev 1993; 7:331-42; PMID:8449399; http://dx.doi.org/10.1101/gad.7.3.331
- Zelivianski S, Cooley A, Kall R, Jeruss JS. cyclin-dependent kinase 4-mediated phosphorylation inhibits Smad3 activity in cyclin D-overexpressing breast cancer cells. Mol Cancer Res 2010; 8:1375-87; PMID:20736297; http://dx.doi.org/10.1158/1541-7786.MCR-09-0537
- Matsuura I, Denissova NG, Wang G, He D, Long J, Liu F. cyclin-dependent kinases regulate the antiproliferative function of Smads. Nature 2004; 430:226-31; PMID:15241418; http://dx.doi.org/10.1038/nature02650
- Do DV, Ueda J, Messerschmidt DM, Lorthongpanich C, Zhou Y, Feng B, Guo G, Lin PJ, Hossain MZ, Zhang W, et al. A genetic and developmental pathway from STAT3 to the OCT4-NANOG circuit is essential for maintenance of ICM lineages in vivo. Genes Dev 2013; 27:1378-90; PMID:23788624; http://dx.doi.org/10.1101/gad.221176.113
- Huang G, Yan H, Ye S, Tong C, Ying QL. STAT3 phosphorylation at tyrosine 705 and serine 727 differentially regulates mouse ESC fates. Stem Cells 2014; 32:1149-60; PMID:24302476; http://dx.doi.org/10.1002/stem.1609
- Stuart HT, van Oosten AL, Radzisheuskaya A, Martello G, Miller A, Dietmann S, Nichols J, Silva JC. NANOG amplifies STAT3 activation and they synergistically induce the naive pluripotent program. Curr Biol 2014; 24:340-6; PMID:24462001; http://dx.doi.org/10.1016/j.cub.2013.12.04sss0
- Beyer TA, Weiss A, Khomchuk Y, Huang K, Ogunjimi AA, Varelas X, Wrana JL. Switch enhancers interpret TGF-β and Hippo signaling to control cell fate in human embryonic stem cells. Cell Reports 2013; 5:1611-24; PMID:24332857; http://dx.doi.org/10.1016/j.celrep.2013.11.021
- Schaffler A, Buchler C. Concise review: adipose tissue-derived stromal cells–basic and clinical implications for novel cell-based therapies. Stem Cells 2007; 25:818-27; PMID:17420225; http://dx.doi.org/10.1634/stemcells.2006-0589