ABSTRACT
HIV-1 infected macrophages play a significant role in the neuropathogenesis of AIDS. HIV-1 viral protein R (Vpr) not only facilitates HIV-1 infection but also contribute to long-lived persistence in macrophages. Our previous studies using SILAC-based proteomic analysis showed that the expression of critical metabolic enzymes in the glycolytic pathway and tricarboxylic acid (TCA) cycle were altered in response to Vpr expression in macrophages. We hypothesized that Vpr-induced modulation of glycolysis and TCA cycle regulates glutamate metabolism and release in HIV-1 infected macrophages.
We assessed the amount of specific metabolites induced by Vpr and HIV-1 in macrophages at the intracellular and extracellular level in a time-dependent manner utilizing multiple reaction monitoring (MRM) targeted metabolomics. In addition, stable isotope-labeled glucose and an MRM targeted metabolomics assay were used to evaluate the de novo synthesis and release of glutamate in Vpr overexpressing macrophages and HIV-1 infected macrophages, throughout the metabolic flux of glycolytic pathway and TCA cycle activation.
The metabolic flux studies demonstrated an increase in glucose uptake, glutamate release and accumulation of α-ketoglutarate (α-KG) and glutamine in the extracellular milieu in Vpr expressing and HIV-1 infected macrophages. Interestingly, glutamate pools and other intracellular intermediates (glucose-6-phosphate (G6P), fructose-6-phosphate (F6P), citrate, malate, α-KG, and glutamine) showed a decreased trend except for fumarate, in contrast to the glutamine accumulation observed in the extracellular space in Vpr overexpressing macrophages.
Our studies demonstrate that dysregulation of mitochondrial glutamate metabolism induced by Vpr in HIV-1 infected macrophages commonly seen, may contribute to neurodegeneration via excitotoxic mechanisms in the context of NeuroAIDS.
Introduction
A significant number of patients infected with human immunodeficiency virus-1 (HIV-1) have cognitive impairment ranging from mild to severe HIV-associated dementia (HAD). HIV-1 infection of macrophages leads to long-lived survival and low levels of constitutive virus production, generating macrophage viral reservoirs,Citation1 which contribute to HIV-1 associated neurocognitive diseases (HAND).Citation2 HIV-1 viral protein R (Vpr) mediates many processes that promote HIV-1 infection, allows evasion of the immune system, and facilitates persistence in the host cells.Citation3 Vpr is known to promote viral replication in monocytes and differentiated macrophages.Citation4 It is becoming increasingly apparent that changes occur in the metabolic profile of the cell upon viral infection since metabolites are essential for viral replication.Citation5-8 Studies suggest that alterations in cellular metabolism can alter macrophage function, and also macrophage plasticity is dependent on the ability of the macrophage to reprogram or alter cellular metabolism.Citation9 Furthermore, there is growing evidence supporting the role of cell cycle regulators such as cyclins and cyclin-dependent kinases in the regulation of metabolism.Citation10 Vpr is known to induce cell cycle arrest in G2/M phase.Citation11,12 Vpr-mediated pathogenesis in macrophages has been extensively investigated,Citation13-15 but its effects on the global molecular mechanism associated with metabolic pathways have not been characterized. Our recent SILAC-based proteomics study showed that HIV-1 Vpr overexpression in macrophages induces changes at the protein level in many of the enzymes involved in cellular metabolism.Citation16 HAD has been linked to macrophage toxin production including glutamate.Citation17-20 Increased glutamate levels are also seen in the plasma, and cerebrospinal fluid (CSF) of HIV-1 infected patients.Citation18,21 In addition, the observation that soluble Vpr is detected in the CSF of HIV-1 infected individualsCitation22 suggests that Vpr can have bystander effects on uninfected cells and on neuronal cellsCitation23 and contribute to glutamate-mediated neurodegeneration.
We hypothesize that glutamate metabolism in macrophages is regulated by HIV-1 Vpr as a result of activation of TCA cycle, one of the altered pathways identified in our earlier proteomic study.Citation16 The development of a metabolomics platform to evaluate the final products of the metabolic pathways induced in macrophages overexpressing Vpr can offer a better understanding of mechanisms responsible not only for viral reservoir persistence in macrophages but also for the pathogenesis of HAD. Such biomarker(s) will help in early detection and classification of neurocognitive impairment.Citation24 Furthermore, multiple reaction monitoring (MRM)Citation25 can also be applied to drug discovery assays in the context of HIV-1 infection of macrophages and can be used for lead selection, lead optimization, in vivo efficacy screening, and early in vivo toxicological testing. This targeted metabolomics platform can be utilized in the future as a high-throughput screening tool for identification of new biomarker(s) in plasma and CSF of HIV-1 infected individuals. In this study, we aim to elucidate the role of Vpr in the macrophage-mediated modulation of extracellular glutamate, glutamine, and α-KG metabolism in macrophages.
Results
Earlier studies have demonstrated an increase in extracellular levels of glutamate from HIV-1 infected macrophages predominantly derived from glutamine regulated by mitochondrial glutaminase.Citation17,20 We hypothesize that the observed increase in extracellular levels of glutamate in the context of HIV-1 infection of macrophages is mediated by Vpr induced glycolysis, and TCA cycle activation in HIV-1 infected macrophages. To test this hypothesis we employed U937 cells activated by phorbol-12-myristate-13-acetate (PMA), to promote cell cycle arrest and induction of a differentiation program into macrophages, characterized by phenotypic and functional changes.Citation26 We have developed a state-of-the-art mass spectrometry-based targeted 13C-metabolic flux profiling of glucose to measure de novo synthesis and release of glutamate upon activation of the glycolytic-TCA pathways to evaluate the role of HIV-1 Vpr in orchestrating changes in macrophage glutamate metabolism ().
Figure 1. Schematic representation of Glucose-dependent Glutamate Flux in macrophages. The red circles indicate the position of stable isotope 13C label in 13C6-Glucose and the subsequent intermediate metabolites during glutamate synthesis during activation of the glycolytic and TCA cycle in macrophages. Bold arrows in tandem mean several intermediate process before next metabolite.
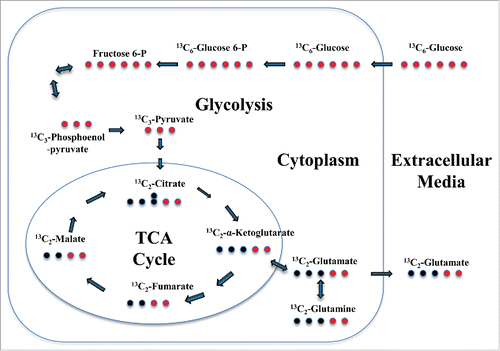
Optimization of multiple reaction monitoring (MRM) method/assay
The MRM methodCitation25 optimization for analysis of targeted 13C-metabolic flux profiling of glucose and its intermediate metabolites was performed using standard aqueous stock solutions of selected metabolites, namely G6P, F6P, citrate, α-KG, fumarate, malic acid, glutamic acid and glutamine ( and ). MRM parameters for the transitions (parent ions) detection, as well as the production of compound-specific fragment ions (daughter ions), were optimized including the cone voltages, collision energies, and ion mode detection; all these aspects varied to obtain the optimal fragmentation of each metabolite (). The MRM analysis is based on matching 3 unique characteristics of each metabolite: a) the retention time, b) the masses of the precursor ion and c) the mass of at least one intense fragment ion and a second confirmatory ion ( and ).
Figure 2. MRM targeted metabolomics assays to measure glutamate and 13C6-glucose uptake. (A) Glutamate MRM spectrum profile of the daughter ions (83.996 and 129.962 m/z) identified for the fragmentation of the parent ion (147.904 m/z) (left panel). The area under the peak of the most abundant daughter ion (147.904 – 83.996 m/z) was used to perform a standard curve with a serial dilution of the glutamate standard (right panel). (B) 13C6-glucose MRM spectrum profile of the daughter ions (168.969 and 88.903 m/z) identified for the fragmentation of the parent ion (204.15 m/z).
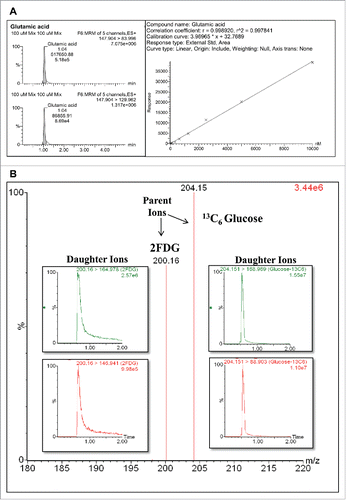
Table 1. Optimization for the parent and daughters ions identification of the target metabolites in the MRM Method.
The MRM for glutamic acid and glucose (both 2-FDG and 13C6-glucose) are shown as examples (). The plot in illustrates the glutamate MRM spectrum profile of the daughter ions (83.996 and 129.962 m/z) identified after the fragmentation of the parent ion (147.904 m/z). The area under the peak of the most abundant daughter ion (147.904→83.996 m/z) was used to perform a standard curve with a serial dilution of the glutamate standard (Panel A, right panel). Furthermore, this analysis allowed us to establish that 5 nM is the lower limit of detection that we can measure using MRM assay. The 2-FDG and 13C6-glucose MRM spectrum profiles are shown in : the FDG MRM spectrum profile of the daughter ions (164.978 and 146.941 m/z) identified after the fragmentation of the parent ion (200.16 m/z) and the 13C6-glucose MRM spectrum profile of the daughter ions (168.969 and 88.903 m/z) identified after the fragmentation of the parent ion (204.15 m/z). The values of the parent and daughter ions of all the selected metabolites analyzed using the MRM assay are shown in . Finally, 2 h after 13C-glucose addition, the derived metabolites 13C2-ketoglutarate, 13C2-glutamine and 13C2-glutamic acid were detected in the culture media by adding 2 atomic mass units to the initial values of parents and daughters ions in the MRM identification assay.
Changes in levels of intracellular and extracellular metabolites of glycolysis and TCA cycle induced by HIV-1 Vpr in macrophages
We first assessed glutamate flux by measuring the intracellular, and extracellular metabolites pools of the glycolytic and TCA pathways altered in response to HIV-1 Vpr overexpression in U937 macrophages in comparison to the control Ad-null transduced macrophages (). MRM analysis of intracellular G6P levels demonstrated a significant decrease on day 7 compared to control cells in the glycolytic pathway. MRM analysis of intracellular metabolites of the TCA cycle demonstrated a significant increase in fumarate levels in Vpr transduced macrophages. However, we observed a significant decrease in levels of α-KG and malate in Vpr transduced macrophages (). In contrast, we observed a significant increase in levels of α-KG and glutamine in the extracellular media of macrophages transduced with Vpr on day 7 in comparison to the Ad-Null transduced macrophages (). Increase in glutamate levels in the extracellular media of macrophages transduced with Vpr was observed on day 3 only, in comparison to the Ad-Null transduced macrophages ().
Figure 3. Changes in metabolite levels in response to HIV-1 Vpr protein in U937 macrophages. MRM analysis of glutamate pools and other intracellular intermediates of glycolytic and TCA cycle pathways including G6P, F6P, citrate, malate, α-KG, fumarate, and glutamine were measured in U937 derived macrophages overexpressing Ad-Vpr or Ad-Null in a time-dependent manner. Glutamate, glutamine, and α-ketoglutarate were also detected and measured in the culture media at the indicated time. All data are expressed as relative intensity of metabolites (means ± SD) of triplicates and are a representative of at least 2 independent experiments. Significant p-values are shown as *< 0.05 and **< 0.01.
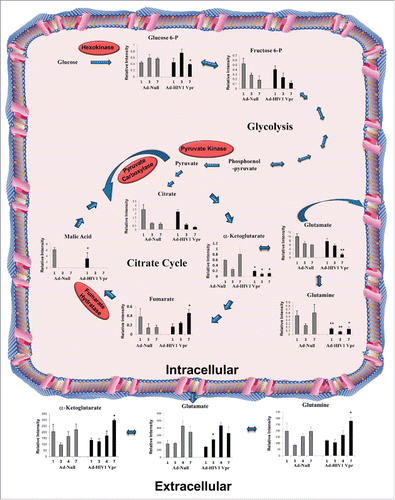
Changes in expression levels of selected enzymes in TCA cycle and glutamate metabolism is modulated by HIV-1 Vpr in macrophages
Our earlier studies have demonstrated the upregulation of numerous enzymes in the glycolytic pathway in response to HIV-1 Vpr overexpression in U937 macrophages in comparison to the control Ad-null transduced macrophages.Citation16 To corroborate changes in the intracellular and extracellular metabolite levels we assessed the expression levels of selected enzymes in the TCA cycle in response to HIV-1 Vpr overexpression in U937 macrophages in comparison to the control Ad-null transduced macrophages on day 1, 3 and 7 (). Our results show that the expression of malate dehydrogenase 2 (MDH2), a member of the TCA cycle is upregulated by Vpr by ∼2.2-fold on day 1 and downregulated on day 3 and 7 respectively (). Our studies also show that expression level of fumarase, a member of the TCA cycle is upregulated by Vpr by ∼2.2-fold and 1.3-fold on day 1 and day 3 respectively, which was downregulated on day 7 (). Significant changes in levels of enzymes involved in glutamate metabolism are observed in response to HIV-1 Vpr. Among them, we observed a modest increase in the expression of the mitochondrial glutaminase (GLS), a phosphate-activated amidohydrolase that catalyzes the hydrolysis of glutamine to glutamate and ammonia by ∼1.2-fold on day 1 and 3. However, the levels of GLS declined on day 7 in Vpr transduced cells (). We observed no change in expression levels of mitochondrial glutamate dehydrogenase 2 (GLUD2) that catalyzes the reversible oxidative deamination of glutamate to α-KG. However, there was no change in the expression level of glutamine synthetase (GS) that catalyzes the ATP-dependent condensation of glutamate with ammonia to yield glutamine on day 1. However, GS expression was downregulated in Vpr treated cells on day 3 and 7 ().
Figure 4. Changes in levels of enzymes in the TCA cycle and glutamate metabolism in response to HIV-1 Vpr protein in U937 macrophages. (A) Western blot of proteins in total cell lysates of U937 macrophages transduced with Ad-Null (Null) or Ad-Vpr (Vpr) on day 1, 3 and 7. GLUD1/2: glutamate dehydrogenase 1/2, GS: glutamine synthetase, GLS: glutaminase, MDH2: malate dehydrogenase 2, FH: fumarate hydratase, tubulin, and Vpr: viral protein R. (B-D) Densitometric analysis of expression levels for GLUD 1/2, GLS, GS, MDH2, and FH, normalized to tubulin levels shown in panel A plotted as fold change wherein the levels of expression in Ad-Null transduced cells is set at 1 on day 1, 3, 7 after transduction, respectively. (E) Western blot of GLS and GLUD2 in the culture supernatant. A representative Western blot of 3 independent experiment is shown. Significant p-values are shown as *< 0.05 and **< 0.01.
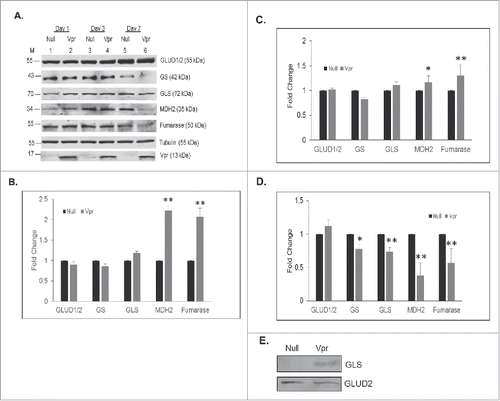
Since we observed a modest increase or no change in intracellular GLS and GLUD2 expression levels on day 3 in Vpr transduced macrophages we assessed extracellular levels of GLS and GLUD2 on day 3 post-transduction with Ad-Null or Ad-Vpr. This study shows a significant increase in the extracellular levels of mitochondrial GLS in Ad-Vpr transduced macrophages in comparison to the Ad-Null transduced macrophages (), however, we observed no change in levels of GLUD2 ().
Increase in glutamate release in macrophages transduced with HIV-1 Vpr is mediated by an increase in glucose uptake and glucose flux
Glutamate flux was measured to demonstrate that the observed increase in glutamate release in Vpr overexpressing macrophages is indeed glucose-dependent and not as a consequence of the conversion of media glutamine to glutamate. U937 derived macrophages were transduced with Ad-Vpr or Ad-Null then pulse-labeled with 13C-labeled glucose containing media for 2 hr and followed by 2-FDG treatment for 30 mins on day 1, 3 and 7 post-infection. We observed a significant increase (∼4-fold) in 13C6-glucose uptake on day 3 and 7 (). Interestingly, we also observed significant increase in 13C2-glutamate release on day 3 (∼2.5-fold) and on day 7 (∼1.5-fold) () and accumulation of 13C2-glutamine and 13C2-α-ketoglutarate in the extracellular media of cells overexpressing HIV-1 Vpr in comparison to the null transduced macrophages on day 3 and 7 ().
Figure 5. HIV-1 Vpr induces increased glucose uptake, and glutamate release in U937 derived macrophages. (A) The relative intensity of glucose uptake in U937 derived macrophages overexpressing Ad-Vpr in comparison to Ad-Null transduced cells on day 1, 3 and 7 post-transduction; the intracellular amount of stable heavy isotope 13C6-glucose was measured by a specific MRM method to measure the glucose accumulation after blocking the glycolytic pathway with FDG. (B, C, and D) The relative intensity of heavy isotope of 13C2-glutamate, 13C2-glutamine, and 13C6-α-ketoglutarate accumulation in the culture media supernatant 2 h after 13C6-glucose incubation measured by MRM method in U937 derived macrophages transduced with Ad-Vpr or Ad-Null. All data are expressed as means ± SD of triplicates and are a representative of at least 2 independent experiments. Significant p-values are shown as *< 0.05 and **< 0.01.
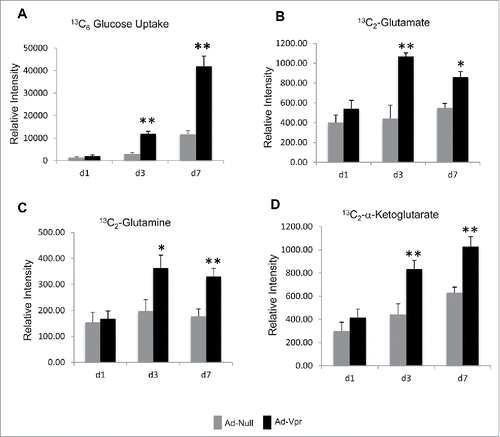
HIV-1 infection of macrophages induces extracellular glutamate levels
We first assessed glutamate flux by measuring the extracellular metabolites pools of glutamate in response to HIV-1 infection of MDMs in comparison to the mock-infected MDMs grown in regular cell culture medium (). MRM analysis of extracellular levels of glutamate, glutamine, and α-KG on day 7 and day 14 post infection demonstrated that there was a significant increase in glutamate levels in the extracellular media on day 14 post infection of MDMs with HIV-1 in comparison to mock-infected MDMs (). In contrast, we observed no alterations in levels of glutamine and α-KG in the extracellular media of macrophages infected with HIV-1 in comparison to mock infected MDMs ().
Figure 6. HIV-1 infection of MDMs induces enhanced extracellular glutamate release. (A) MRM method to measure glutamate, glutamine, and α-ketoglutarate accumulation in the culture media on day 7 and day 14 post infection in HIV-1- and mock infected MDMs. The levels of HIV-1 in the infected cell supernatant was 265.25 pg/ml on day 14 post infection. (A-C) The relative intensity of glutamate, glutamine, and α-ketoglutarate accumulation in the culture media supernatant. All data are expressed as means ± SD of triplicates and are a representative of at least 2 independent experiments. Significant p-values shown as *< 0.05.
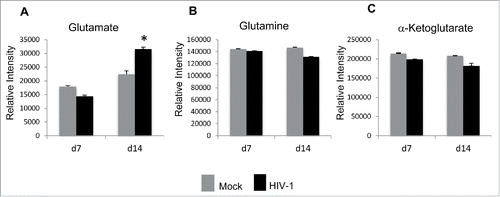
Increase in glucose uptake and α-KG in intracellular compartment of HIV-1 infected macrophages
Since Vpr overexpression increased glucose uptake and glucose flux, we asked the question whether HIV-1 expression in MDMs also increases glucose uptake. To characterize glycolytic flux, we switched the regular culture media of HIV-1 infected MDMs, as well as their mock-infected counterpart on day 14 post-infection to 13C-labeled glucose containing media for 2 hr followed by 2-FDG treatment for 30 mins. Targeted MRM method was used to monitor uptake of labeled glucose and secretion of intracellular metabolized labeled glutamate into the extracellular media. Increase in glucose-dependent glutamate flux in HIV-1 infected macrophages was observed, as evidenced by an increase in uptake of stable heavy isotope 13C6-glucose by 3-fold (). On the contrary, we observed a significant decrease in 13C2-glutamate accumulation (), with no major change in levels of 13C2-glutamine in the extracellular media (), while there was a significant increase in 13C2-α-ketoglutarate levels in the extracellular media in HIV-1 infected scenario (). These observations suggests for unidirectional enzymatic conversion of 13C2-glutamine and 13C2-glutamate into 13C2-α-ketoglutarate in the extracellular milieu.
Figure 7. Increase in glucose uptake and glutamate metabolite secretion in HIV-1 infected macrophages. (A) Glucose uptake in HIV-1 infected and mock infected MDMs on day 14 after infection. The intracellular levels of heavy isotope 13C6-glucose were measured by a specific MRM method after inhibition of the glycolytic pathway with 2FDG. The relative intensity of 13C6-glucose levels is shown in mock and HIV-1 infected MDMs. (B, C, and D) Stable heavy isotope of 13C2-glutamate, 13C2-glutamine and 13C2-α-Ketoglutarate were detected and measured in the culture media supernatant 2 h after 13C6-glucose incubation by using a specific MRM method in HIV-1 infected MDMs 14 d after infection. All data are expressed as means ± SD. Significant p-values are noted as * for less than 0.05 and ** for less than 0.01.
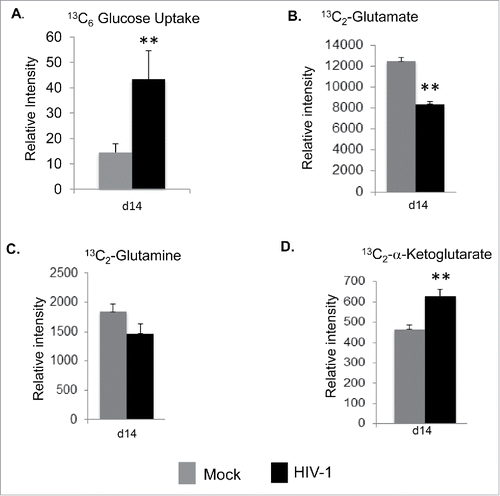
Discussion
HIV-1 infected macrophages play a major role in the neuropathogenesis of AIDS and mechanisms responsible for HIV-1 mediated neurotoxicity, including increased production of glutamate.Citation18,19 In this context, HIV-1 Vpr mediates many processes that facilitate HIV-1 infection and promote persistence in the host cells.Citation3,13 Our earlier studies using SILAC-based proteomics analysis demonstrated that numerous enzymes in the glycolytic pathway and TCA cycle were altered in response to HIV-1 Vpr in macrophages.Citation16 In this study, we evaluated alteration in metabolic profile in response to HIV-1 Vpr in macrophages to correlate with the observed changes in expression profile of critical enzymes in the glycolytic pathway and TCA cycle. In fact, metabolomics has provided sensitive, fast, and robust tools for analyzing metabolite levels during viral infections.Citation7 Isotope-assisted metabolomics is applicable for certain classes of metabolites or simplified metabolite mixtures that allow measurement and interpretation of endogenous and released metabolites flux in cells.Citation27
The MRM analysis of glycolytic pathway and TCA intermediate metabolites, such as G6P, α-KG, and malate, demonstrated a significant decrease in HIV-1 Vpr transduced macrophages compared to control cells. This suggests an increase in the enzymatic activity of the respective enzyme that consumes the intermediate metabolite and is in agreement with our earlier observation that HIV-1 Vpr induced the protein levels of the glycolytic pathway and TCA cycle.Citation16 The significant decline in levels of malic acid () corroborates with the increase in MDH2 levels by 1.6-fold in Vpr transduced macrophages (). Of interest, we observed significant accumulation of fumarate in the macrophages overexpressing HIV-1 Vpr protein, in contrast, to decrease in other metabolites such as citrate and α-KG. This observation suggests that Vpr most likely inhibits fumarase activity since no significant difference in expression levels of fumarase is observed in macrophages transduced with Ad-Null or Ad-Vpr (). Interestingly, fumarate can induce a hypoxic gene expression profile by inhibiting the activity of all 3 prolyl 4-hydroxylases.Citation28 Furthermore, fumarate also is a competitive inhibitor of α-KG or 2-oxoglutarate-dependent 10-11 translocation enzymes (TETs) for DNA demethylation and many JmjC-type of histone demethylases.Citation29
In this report, we demonstrate for the first time that active glutamate production and release in Vpr transduced macrophages is mediated by glucose-dependent metabolic flux following activation of glycolytic and TCA cycle (). Furthermore, the altered profiles of intracellular and extracellular metabolites reflect modulation of expression and activity of enzymes in the glycolytic and TCA cycle pathways in Vpr transduced macrophages. The observed increase in extracellular levels of glutamate, α-KG and glutamine in the Vpr transduced macrophages suggest that most likely deamination of glutamine also known as glutaminolysis by GLS to glutamate and ammonia with subsequent conversion of glutamate to α-KG and its accumulation. The increase α-KG levels suggest that α-KG, a TCA cycle intermediate can result in increased production of both ATP and anabolic carbons for the synthesis of lipids, amino acids, and nucleotides. We have shown earlier that Vpr induces oxidative stress in an hypoxia-inducible factor 1 α (HIF-1α) dependent manner,Citation30 and in turn HIF-1α is induced by Vpr.Citation16 Glutaminolysis also favors production of antioxidants such as glutathione (GSH) and NADPH.Citation31,32 Furthermore, α-KG a key intermediate in glutamate metabolism is known to stabilize reactive oxygen species (ROS) homeostasis in cells and thereby mitigate oxidative stress-mediated damage to proteins, lipids and DNA.Citation33,34 Therefore, maintenance of ROS homeostasis in infected HIV-1 macrophages can promote long-term survival and persistence.
Earlier studies have shown that glutamate production is enhanced following HIV-1 infection of cultured macrophages by up-regulation of the glutaminase isoform GAC.Citation17 A recent study also demonstrated that recombinant Vpr increases production of glutaminase C, a regulator of glutamate synthesis in murine macrophages.Citation35 Our results are in agreement with previous findings where the HIV-1 infection of human macrophages leads to increase in glutamate production mediated by the enzyme glutaminase,Citation17,36. Increase in glutamate levels has been reported to be glutamine-dependent, and the glutamate-mediated neurotoxicity has been shown to be augmented by the mitochondrial glutaminase release.Citation17,20 Similar to these reports, we have observed a significant increase in the levels of GLS in the media by HIV-1 Vpr overexpression in macrophages () resulting in increased extracellular release of glutamate. Our findings in HIV-1 infected MDMs are novel as we demonstrate for the first time a transient increase in α-KG levels in the extracellular media () which is derived as a consequence of increased glucose flux () through the glycolytic and TCA cycle pathway.
These results indicate that HIV-1 infection of macrophages and HIV-1 Vpr overexpression induces relevant changes in multiple metabolic pathways with the consequent increase in glutamate production and release upon activation of glycolysis and TCA cycle.
Materials and methods
Chemical reagents
All components of cell culture were obtained from Life Technologies (CA, USA) and protease inhibitor cocktail were obtained from Sigma-Aldrich (St. Louis, MO, USA). All the chemicals were HPLC-grade unless specifically mentioned. Stable heavy isotope glucose (13C6Glucose), 2-Fluoro-deoxy-2-d-glucose (FDG), G6P, F6P, citrate, fumarate, malate, α-ketoglutarate, glutamate, and glutamine were obtained from Sigma-Aldrich. Primary antibodies were used against fumarase (Cell Signaling Technology, Danvers, MA), malate dehydrogenase 2 (MDH2) (Santa Cruz Biotechnology, Dallas, TX, USA), glutaminase (GLS) (Abgent, San Diego, CA, USA), glutamine synthetase (GS) (Thermo Fisher Scientific, USA), glutamate dehydrogenase 2 (GLUD2) (R&D Systems, Minneapolis, MN, USA) and Tubulin (Sigma-Aldrich, St. Louis, MO, USA). IR-dye conjugated secondary antibodies were obtained from LI-COR (Lincoln, NE, USA).
Cell culture
The human monocytic cell line U937 was obtained from American Type Culture Collection (Manassas, VA, USA) and grown in complete DMEM medium at 37°C in humidified atmosphere with 5% CO2.
Construction of recombinant adenoviruses
The recombinant adenoviral vector harboring HIV-1 Vpr was constructed by excision of Vpr cDNA (288 bp) from pcDNA3-Vpr and subsequent cloning into the EcoRI and NheI sites of the shuttle plasmid pDC515 harboring the murine cytomegalovirus promoter (Microbix Inc., Ontario, Canada). Adeno-Vpr recombinant shuttle vector was transfected into HEK-293 cells with pBHGfrt (del) E1, 3FLP, and a plasmid that provides adenovirus type 5 genome deleted in E1 and E3 genes.Citation30 Plaques of recombinant adenovirus were isolated, grown, and purified by cesium chloride density centrifugation as described previously.Citation37 pDC515 was used to construct control adenoviral vector (Adeno-null, a virus without a transgene).
Adenoviral transduction
PMA differentiated U937 cells (5 × 106/well) were plated in 6-well tissue culture dish and transduced with an adenoviral stock of Ad-Null (empty vector) or Ad-Vpr at a multiplicity of infection (MOI) of 5 pfu (plaque forming units) per cell.Citation16 Cells were harvested at 1, 3 and 7 day post transduction.
Preparation of monocyte-derived macrophages and HIV-1 infection
Buffy coats were provided by the Comprehensive NeuroAIDS center (CNAC) under the approval of the Temple University Institutional Review Board (IRB). No informed consent was obtained as the study was classified as exempt (category 4) by the IRB.
PBMCs were isolated from buffy coat using the standard ficoll gradient centrifugation method and resuspended in DMEM containing 10% FBS and 10 mg/ml gentamicin (Life Technologies). Isolated PBMCs were allowed to adhere to tissue culture flask overnight, and adherent monocytes were differentiated in DMEM (Life Technologies) media containing 10% FBS, and 100 ng/ml M-CSF (R&D Systems) for 7 d. Fresh complete media containing M-CSF was added after removal of one-third of the medium every third day. MDMs were then infected with 10000 TCID50/106 cells (MOI ∼0.01) of HIV-1 89.6 overnight in a 37°C incubator with 5% CO2. The next day, cells were washed thrice with 1 X PBS and grown in DMEM containing 10% FBS and 10 mg/ml gentamicin. An aliquot of cell culture supernatant was collected on day 14 to quantify viral load by p24 ELISA (Advanced Bioscience Laboratories, Cat #5421). HIV-1 infected MDMs were used on days 7 and 14 for the studies.
Glucose uptake and metabolic flux in macrophages
Two million cells were used in triplicate for each condition. After transduction with Adeno-Vpr or Ad-Null for specific duration, cells were washed with PBS, followed by addition of 10 mM of 13C6 Glucose in a glucose free DMEM medium containing dialyzed 10% FBS and incubated for 2 hours at 37°C, followed by an additional 30 min incubation with 2-FDG at a final concentration of 5 mM, 2-FDG is an inhibitor of glucose metabolism thus preventing the glucose breakdown and allowing its accumulation to furher be measure and quantified. The supernatant cell media was collected for further fluxing metabolites evaluation and cells were harvested, washed thrice with 1X PBS and the cell pellet was lysed in 50 μl of M-PER buffer for extraction of metabolites and further analysis.
Metabolite extraction
Proteins from the supernatant cell media or from the cell lysates were precipitated with 3 volumes of acetonitrile, and the liquid phase was concentrated in a speed-vacuum.Citation38 The dry pellet containing the metabolites was resuspended in buffer A (20 mM ammonium hydroxide and 20 mM ammonium acetate) and used to measure the metabolites.
Multiple Reaction Monitoring (MRM)
The detection of the 13C6 glucose, G6P, F6P, citrate, fumarate, malate, glutamine, α-ketoglutarate and glutamate, as well as, the heavy forms of these metabolites, products of heavy 13C6 glucose metabolism was carried out using a Waters XevoTM triple quadrupole tandem mass spectrometer (Waters Corp., Manchester, UK). IntelliStart software was used for method development of each metabolite and the optimization of the mass spectrometer parameters for best metabolite transitions detection conditions were performed including an automated checking of cone voltage (range: 2–100), collision energy (range 2-80), lowest fragment mass detection of 40.00 m/z and the identification up to 5 daughters -fragmentation ions- in both positive and negative electrospray ionization mode. During this optimization, each metabolite was dissolved in 20 mM ammonium hydroxide 50% acetonitrile, at a final concentration of 10 µM and directly inject into the mass spectrometer.
UPLC/MS/MS method for the detection of all metabolites was developed for a complete separation and identification of the metabolic extracts for in less than 6 min per sample including a rapid elution of compounds and subsequent MRM. This method was confirmed using the metabolite standards in an ACQUITY UPLC® (100 mm × 2.1 mm, 1.7 μm) BEH Amide C18 column heated to 40°C employing a flow rate of 0.4 mL/min and mobile phases A and B, 20 mM ammonium hydroxide and 20 mM ammonium acetate in acetonitrile, respectively, and with the following gradient: 0% B, 0-1 min; 0% B-50% B, 1-2.5 min; 50% B-90% B, 2.5-3.2 min; 90% B to 0% B, 3.2-4 min; total run time 5 min. The MRM method development was performed using a standard aqueous stock solution of each of the metabolites to be analyzed at 30 μM in 20 mM ammonium hydroxide, based on unique product matching 3 unique aspects characteristics of each metabolite: the retention time, the masses of the precursor ion and the mass of at least one intense fragment ion. Chromatographic and mass spectrum data were collected and analyzed with Waters MassLynx v4.1 software. The quantification was obtained for each metabolite using linear regression analysis of the peak area ratio analyte versus concentration. The ratios of the precursor and fragment ions allow an accurate quantification of all the target metabolites. Confirmation was performed using a standard calibration curves for each metabolite at concentrations of 0.01, 0.05, 0.1, 0.5, 1, 5, 10, 50, and 100µM.
Western blot analysis
Protein samples (40 μg) from cell lysates and protein samples (10 μg) from TCA precipitated conditioned media were separated by 10% gradient SDS-PAGE and then transferred to a PVDF membrane in a blotting chamber (BioRad) at 100 V for 30 min. The membrane was blocked with 5% powdered milk in Tris-buffer saline solution (pH 7.6) containing 0.05% Tween-20 (TBS/T) then probed with antibodies against MDH2 (1:200), FH (1:1000), GLS (1:500), GLUD1/2, GS (1:5000), Vpr (1:1000) and tubulin (1:1000). Membranes were incubated with primary antibodies overnight at 4°C, washed, and then incubated with appropriate secondary antibodies conjugated to IR-Dye at room temperature for 1 h. Images were captured using Odyssey CLx imager (LI-COR, Lincoln, NE). GelQuant.net software (BiochemLabSolutions.com) was used for densitometric quantification of changes in expression levels of proteins.
Statistical analysis
All data were expressed as means ± SD. Values between control and HIV-1 and Ad-Null and Ad-Vpr studies were compared by unpaired 2-tailed Student's t-test using GraphPad software (GraphPad Software Inc., LaJolla, CA, USA). p-value < 0.05 were considered significant.
Disclosure of potential conflicts of interest
No potential conflicts of interest were disclosed.
Funding
This study was supported in part by R01 DA033213 to PKD and 1P01DA037830-01A1 to PKD, KK, and by the Comprehensive NeuroAIDS Center grant (P30MH0921777) to KK, a seed grant by the Developmental Core of CNAC to CB.
References
- Kilareski EM, Shah S, Nonnemacher MR, Wigdahl B. Regulation of HIV-1 transcription in cells of the monocyte-macrophage lineage. Retrovirology 2009; 6:118; PMID:20030845; http://dx.doi.org/10.1186/1742-4690-6-118
- Brew BJ, Crowe SM, Landay A, Cysique LA, Guillemin G. Neurodegeneration and ageing in the HAART era. J Neuroimmune Pharmacol 2009; 4:163-74; PMID:19067177; http://dx.doi.org/10.1007/s11481-008-9143-1
- Kogan M, Rappaport J. HIV-1 accessory protein Vpr: relevance in the pathogenesis of HIV and potential for therapeutic intervention. Retrovirology 2011; 8:25; PMID:21489275; http://dx.doi.org/10.1186/1742-4690-8-25
- Connor RI, Chen BK, Choe S, Landau NR. Vpr is required for efficient replication of human immunodeficiency virus type-1 in mononuclear phagocytes. Virology 1995; 206:935-44; PMID:7531918; http://dx.doi.org/10.1006/viro.1995.1016
- Bukrinsky M, Sviridov D. Human immunodeficiency virus infection and macrophage cholesterol metabolism. J Leukoc Biol 2006; 80:1044-51; PMID:17056763; http://dx.doi.org/10.1189/jlb.0206113
- Hollenbaugh JA, Munger J, Kim B. Metabolite profiles of human immunodeficiency virus infected CD4+ T cells and macrophages using LC-MS/MS analysis. Virology 2011; 415:153-9; PMID:21565377; http://dx.doi.org/10.1016/j.virol.2011.04.007
- Noto A, Dessi A, Puddu M, Mussap M, Fanos V. Metabolomics technology and their application to the study of the viral infection. J Matern Fetal Neonatal Med 2014; 27 Suppl 2:53-7; PMID:25284178; http://dx.doi.org/10.3109/14767058.2014.955963
- Sen S, Kaminski R, Deshmane S, Langford D, Khalili K, Amini S, Datta PK. Role of hexokinase-1 in the survival of HIV-1-infected macrophages. Cell Cycle 2015; 14:980-9; PMID:25602755; http://dx.doi.org/10.1080/15384101.2015.1006971
- El Kasmi KC, Stenmark KR. Contribution of metabolic reprogramming to macrophage plasticity and function. Semin Immunol 2015; 7:S1044-5323
- Kaplon J, van Dam L, Peeper D. Two-way communication between the metabolic and cell cycle machineries: the molecular basis. Cell Cycle 2015; 14:2022-32; PMID:26038996; http://dx.doi.org/10.1080/15384101.2015.1044172
- Andersen JL, Le Rouzic E, Planelles V. HIV-1 Vpr: mechanisms of G2 arrest and apoptosis. Exp Mol Pathol 2008; 85:2-10; PMID:18514189; http://dx.doi.org/10.1016/j.yexmp.2008.03.015
- Guenzel CA, Hérate C, Benichou S. HIV-1 Vpr-a still “enigmatic multitasker.” Front Microbiol 2014; 5:127; PMID:24744753; http://dx.doi.org/10.3389/fmicb.2014.00127
- Chen R, Le Rouzic E, Kearney JA, Mansky LM, Benichou S. Vpr-mediated incorporation of UNG2 into HIV-1 particles is required to modulate the virus mutation rate and for replication in macrophages. J Biol Chem 2004; 279:28419-25; PMID:15096517; http://dx.doi.org/10.1074/jbc.M403875200
- Jacquot G, Le Rouzic E, David A, Mazzolini J, Bouchet J, Bouaziz S, Niedergang F, Pancino G, Benichou S. Localization of HIV-1 Vpr to the nuclear envelope: impact on Vpr functions and virus replication in macrophages. Retrovirology 2007; 4:84; PMID:18039376; http://dx.doi.org/10.1186/1742-4690-4-84
- Sherman MP, De Noronha CM, Williams SA, Greene WC. Insights into the biology of HIV-1 viral protein R. DNA Cell Biol 2002; 21:679-88; PMID:12396611; http://dx.doi.org/10.1089/104454902760330228
- Barrero CA, Datta PK, Sen S, Deshmane S, Amini S, Khalili K, Merali S. HIV-1 Vpr modulates macrophage metabolic pathways: a SILAC-based quantitative analysis. PLoS One 2013; 8(7):e68376; PMID:23874603; http://dx.doi.org/10.1371/journal.pone.0068376
- Erdmann N, Tian C, Huang Y, Zhao J, Herek S, Curthoys N, Zheng J. In vitro glutaminase regulation and mechanisms of glutamate generation in HIV-1-infected macrophage. J Neurochem 2009; 109:551-61; PMID:19222703; http://dx.doi.org/10.1111/j.1471-4159.2009.05989.x
- Ferrarese C, Aliprandi A, Tremolizzo L, Stanzani L, De Micheli A, Dolara A, Frattola L. Increased glutamate in CSF and plasma of patients with HIV dementia. Neurology 2001; 57:671-5; PMID:11524477; http://dx.doi.org/10.1212/WNL.57.4.671
- Jiang ZG, Piggee C, Heyes MP, Murphy C, Quearry B, Bauer M, Zheng J, Gendelman HE, Markey SP. Glutamate is a mediator of neurotoxicity in secretions of activated HIV-1-infected macrophages. J Neuroimmunol 2001; 117:97-107; PMID:11431009; http://dx.doi.org/10.1016/S0165-5728(01)00315-0
- Tian C, Sun L, Jia B, Ma K, Curthoys N, Ding J, Zheng J. Mitochondrial glutaminase release contributes to glutamate-mediated neurotoxicity during human immunodeficiency virus-1 infection. J Neuroimmune Pharmacol 2012; 7:619-28; PMID:22527635; http://dx.doi.org/10.1007/s11481-012-9364-1
- Ollenschlager G, Jansen S, Schindler J, Rasokat H, Schrappe-Bacher M, Roth E. Plasma amino acid pattern of patients with HIV infection. Clin Chem 1988; 34:1787-9; PMID:2901300
- Levy DN, Refaeli Y, MacGregor RR, Weiner DB. Serum Vpr regulates productive infection and latency of human immunodeficiency virus type 1. Proc Natl Acad Sci U S A 1999; 91:10873-7; http://dx.doi.org/10.1073/pnas.91.23.10873
- Jones GJ, Barsby NL, Cohen EA, Holden J, Harris K, Dickie P, Jhamandas J, Power C. HIV-1 Vpr causes neuronal apoptosis and in vivo neurodegeneration. J Neurosci 2007; 27:3703-11; PMID:17409234; http://dx.doi.org/10.1523/JNEUROSCI.5522-06.2007
- Pendyala G, Want EJ, Webb W, Siuzdak G, Fox HS. Biomarkers for neuroAIDS: the widening scope of metabolomics. J Neuroimmune Pharmacol 2007; 2:72-80; PMID:18040828; http://dx.doi.org/10.1007/s11481-006-9041-3
- Guo B, Chen B, Liu A, Zhu W, Yao S. Liquid chromatography-mass spectrometric multiple reaction monitoring-based strategies for expanding targeted profiling towards quantitative metabolomics. Curr Drug Metab 2012; 13:1226-43; PMID:22519369; http://dx.doi.org/10.2174/138920012803341401
- Hass R, Brach M, Kharbanda S, Giese G, Traub P, Kufe D. Inhibition of phorbol ester-induced monocytic differentiation by dexamethasone is associated with down-regulation of c-fos and c-jun (AP-1). J Cell Physiol 1991; 149:125-31; PMID:1939341; http://dx.doi.org/10.1002/jcp.1041490116
- You L, Zhang B, Tang YJ. Application of stable isotope-assisted metabolomics for cell metabolism studies. Metabolites 2014; 42:142-65; http://dx.doi.org/10.3390/metabo4020142
- Koivunen P, Hirsila M, Remes AM, Hassinen IE, Kivirikko KI, Myllyharju J. Inhibition of hypoxia-inducible factor (HIF) hydroxylases by citric acid cycle intermediates: possible links between cell metabolism and stabilization of HIF. J Biol Chem 2007; 282:4524-32; PMID:17182618; http://dx.doi.org/10.1074/jbc.M610415200
- Xiao M, Yang H, Xu W, Ma S, Lin H, Zhu H, Liu L, Liu Y, Yang C, Xu Y, et al. Inhibition of α-KG-dependent histone and DNA demethylases by fumarate and succinate that are accumulated in mutations of FH and SDH tumor suppressors. Genes Dev 2012; 26:1326-38; PMID:22677546; http://dx.doi.org/10.1101/gad.191056.112
- Deshmane SL, Mukerjee R, Fan S, Del Valle L, Michiels C, Sweet T, Rom I, Khalili K, Rappaport J, Amini S, Sawaya BE. Activation of the oxidative stress pathway by HIV-1 Vpr leads to induction of hypoxia-inducible factor 1alpha expression. J Biol Chem 2009; 284:11364-73; PMID:19204000; http://dx.doi.org/10.1074/jbc.M809266200
- DeBerardinis RJ, Cheng T. Q's next: the diverse functions of glutamine in metabolism, cell biology and cancer. Oncogene 2010; 29:313-24; PMID:19881548; http://dx.doi.org/10.1038/onc.2009.358
- Wise DR, Thompson CB. Glutamine addiction: a new therapeutic target in cancer. Trends Biochem Sci 2010; 35:427-33; PMID:20570523; http://dx.doi.org/10.1016/j.tibs.2010.05.003
- Niemiec T, Sikorska J, Harrison A, Szmidt M, Sawosz E, Wirth-Dzieciolowska E, Wilczak J, Pierzynowski S. Alpha-ketoglutarate stabilizes redox homeostasis and improves arterial elasticity in aged mice. J Physiol Pharmacol 2011; 62:37-43; PMID:21451208
- Perry G, Nunomura A, Hirai K, Takeda A, Aliev G, Smith MA. Oxidative damage in Alzheimer disease: the metabolic dimension. J Neuropathol Exp Neurol 2001; 60:759-67; PMID:11487050; http://dx.doi.org/10.1093/jnen/60.10.994
- Doi A, Iijima K, Kano S, Ishizaka Y. Viral protein R of human immunodeficiency virus type-1 induces retrotransposition and upregulates glutamate synthesis by the signal transducer and activator of transcription 1 signaling pathway. Microbiol Immunol 2015; 59:398-409; PMID:25990091; http://dx.doi.org/10.1111/1348-0421.12266
- Erdmann N, Zhao J, Lopez AL, Herek S, Curthoys N, Hexum TD, Tsukamoto T, Ferraris D, Zheng J. Glutamate production by HIV-1 infected human macrophage is blocked by the inhibition of glutaminase. J Neurochem 2007; 102:539-49; PMID:17596215; http://dx.doi.org/10.1111/j.1471-4159.2007.04594.x
- Zolotukhin S, Byrne BJ, Mason E, Zolotukhin I, Potter M, Chesnut K, Summerford C, Samulski RJ, Muzyczka N. Recombinant adeno-associated virus purification using novel methods improves infectious titer and yield. Gene Ther 1999; 6:973-85; PMID:10455399; http://dx.doi.org/10.1038/sj.gt.3300938
- Dietmair S, Timmins NE, Gray PP, Nielsen LK, Kromer JO. Towards quantitative metabolomics of mammalian cells: development of a metabolite extraction protocol. Anal Biochem 2010; 404:155-64; PMID:20435011; http://dx.doi.org/10.1016/j.ab.2010.04.031