ABSTRACT
Cyclin A2 is an essential gene for development and in haematopoietic stem cells and therefore its functions in definitive erythropoiesis have not been investigated. We have ablated cyclin A2 in committed erythroid progenitors in vivo using erythropoietin receptor promoter-driven Cre, which revealed its critical role in regulating erythrocyte morphology and numbers. Erythroid-specific cyclin A2 knockout mice are viable but displayed increased mean erythrocyte volume and reduced erythrocyte counts, as well as increased frequency of erythrocytes containing Howell-Jolly bodies. Erythroblasts lacking cyclin A2 displayed defective enucleation, resulting in reduced production of enucleated erythrocytes and increased frequencies of erythrocytes containing nuclear remnants. Deletion of the Cdk inhibitor p27Kip1 but not Cdk2, ameliorated the erythroid defects resulting from deficiency of cyclin A2, confirming the critical role of cyclin A2/Cdk activity in erythroid development. Loss of cyclin A2 in bone marrow cells in semisolid culture prevented the formation of BFU-E but not CFU-E colonies, uncovering its essential role in BFU-E function. Our data unveils the critical functions of cyclin A2 in regulating mammalian erythropoiesis.
Introduction
The erythroid lineage has the highest cell output in the human body, with about 2 million erythrocytes dying every second and an equal number being produced at the same rate to tightly regulate erythrocyte numbers in the body. In humans, the erythrocyte size (known as the mean corpuscular volume or MCV), like the erythrocyte numbers, displays little variation under normal conditions (80–95 fL) but can vary from individual to invidual. This requires a tight regulation of proliferation and differentiation of the committed erythroid progenitors, BFU-E (Burst-forming unit-erythroid) and CFU-E (Colony-forming unit-erythroid).Citation1 Several cell cycle regulators such as cyclin-dependent kinases (Cdks),Citation2 cyclins,Citation3,4 and Cdk inhibitorsCitation5-9 have been implicated in controlling the division of haematopoietic stem cells (HSCs), which are predominantly quiescent in adults. In contrast, regulators of the cell cycle in committed progenitors of the rapidly turning over erythroid lineage are poorly understood. The reason for this is that targeted mutations in mouse models that affect the functioning of the HSC compartment may arrest haematopoietic development before specific phenotypes in the downstream progenitors such as BFU-Es and CFU-Es can be studied.
Cyclin A2 is expressed in all proliferating mammalian cells and is critical for the onset of both DNA replication (S phase) as well as mitosis by binding to and activating Cdk2 and Cdk1.Citation10,11 Cyclin A2 knockout mice die 5.5 d post implantation,Citation12 highlighting its essential role in mammalian development. Studies in conditional cyclin A2 knockout mice revealed that cyclin A2 is essential for HSCs and embryonic stem cells (ESCs) but is dispensable for proliferation in mouse embryonic fibroblasts (MEFs),Citation4 revealing cell type-specific differences in the requirement for cyclin A2 in cell division. In particular, conditional ablation of cyclin A2 specifically in the HSCs resulted in lethality due to severe decrease in peripheral blood counts of erythrocytes, leukocytes, as well as platelets,Citation4 revealing its critical role in HSC proliferation. Nevertheless, the roles of cyclin A2 in progenitors downstream of HSCs remain to be investigated. Recently, genome-wide association studies (GWAS) of human red blood cell phenotypes identified a genetic locus at 4q27 near the cyclin A2 gene (CCNA2) that reached genome-wide significance for association with erythrocyte size (Mean Corpuscular Volume or MCV).Citation13,14 One of these identified SNPs (rs77666063)Citation13 lies within the coding sequence of cyclin A2, and is predicted to result in a truncated protein lacking the Cdk binding domains.Citation15 These findings necessitated loss of function studies of cyclin A2 in primary erythroid progenitors in vivo to elucidate its potentially critical role in mammalian erythropoiesis. In addition, a recent study reported that silencing of cyclin A2 in fetal liver erythroblasts in culture results in enlarged erythrocytes due to defects in cytokinesis in an in vitro model of the terminal erythropoiesis.Citation16 However, the in vivo effects of cyclin A2 loss on terminal erythropoiesis remain to be studied.
In this study, we investigated the role of cyclin A2 in the committed erythroid progenitors using a conditional cyclin A2 knockout mouse model in combination with an erythroid-specific Cre-recombinase driven by the erythropoietin receptor promoter (ErGFPcre)Citation17 or a 4-hydroxytamoxifen (4OHT) inducible Cre (Rosa26-CreERT2).Citation18 Our data reveals a differential requirement for cyclin A2 in BFU-Es and CFU-Es that is critical for regulating erythrocyte morphology and numbers.
Materials and methods
Mouse generation and analysis
All mouse work was carried out with approved Institutional Animal Care and Use Committee protocols at the Biological Resource Centre mouse facility at Biopolis, Singapore. The generation of cyclin A2fl/fl Citation19 and Cdk2fl/fl Citation20 mice has been previously described. Cyclin A2fl/fl mice were crossed to Rosa26-CreERT2Citation18 mice to obtain 4OHT inducible conditional knockouts, or to ErGFPcreCitation17 mice to obtain erythroid-specific cyclin A2 knockout mice (cyclin A2fl/fl ErGFPcre). p27+/− miceCitation21 were crossed to cyclin A2fl/fl ErGFPcre mice to obtain cyclin A2fl/fl p27−/− ErGFPcre mice. Loss of cyclin A2 was induced in cultures of primary cells obtained from cyclin A2fl/fl Rosa26-CreERT2 mice by the addition of 100 nM 4-hydroxytamoxifen (4OHT). Peripheral blood collected from the submandibular vein was used for analysis of complete blood counts using a Hemavet 950 FS hematology analyzer (Drew Scientific, CDC-9950-005). To evaluate proliferation in spleen, histological sections were stained using Ki-67 antibodies (Leica Microsystems, NCL-Ki67p).
Stress hematopoiesis studies
To study haematopoietic recovery after stress, Phenylhydrazine (Sigma, 114715) was dissolved at 6 mg/ml in sterile PBS and injected intraperitoneally at 60 mg/kg on 2 consecutive days (D0 and D1). Peripheral blood was collected from the submandibular vein on D0, D3, D7, D10, and D14 to analyze the hematocrit recovery kinetics using a Hematology analyzer (Nihon Kohden, MEK-6318K).
Colony formation assays
Freshly harvested bone marrow cells were plated in MethoCult 3234 containing 2 units/ml erythropoietin, 20 ng/ml recombinant mouse Interleukin-3, 20 ng/ml recombinant mouse Interleukin-6, and 50 ng/ml recombinant mouse stem cell factor (SCF), at the concentration of 200,000 cells/ml. CFU-Es were scored at day 3, and BFU-Es were scored at day 8–9 in the same plates. After the BFU-E counts, the colonies from each plate were harvested for genotyping by diluting the methylcellulose in 5 times the volume of PBS and centrifugation, followed by washing with PBS 2 times.
Purification and culture of Lin− bone marrow erythroid progenitors
Lineage-negative (Lin) progenitors were purified from whole bone marrow using the Lineage cell depletion kit (Miltenyi Biotec, 130-090-858). The purified Lin− erythroid progenitors were cultured in erythroid differentiation medium consisting of Iscove's modified Dulbecco medium (Gibco, 12440-053) containing 15% FBS (StemCell Technologies, 06200), 1% detoxified bovine serum albumin (StemCell Technologies, 09300), 200μg/mL holo-transferrin (Sigma, T0665), 10μg/mL recombinant human insulin (Sigma, 91077C), 2 mM L-glutamine (Invitrogen), 10−4M β-mercaptoethanol (Invitrogen), and 2 U/mL erythropoietin (R&D systems). Measurement of cell counts and size were performed using a Vi-CELL XR Beckman Coulter cell counter. FACS analysis of erythroid differentiation and enucleation was performed at 48 hours in culture, as previously described.Citation22
Immunostaining and flow cytometry analysis
Freshly harvested bone marrow cells were fixed using BD cytofix/cytoperm kit (BD Biosciences, 555028), followed by immunostaining with the appropriate antibodies: Phospho-gamma histone H2AX [Ser139] (Cell Signaling, 9718), AlexaFluor 647-conjugated phospho-histone H3 [Ser10] (Cell Signaling, 9716), phycoerythrin-conjugated TER119 antibody (BD, 553673), fluorescein isothiocyanate-conjugated CD71 antibody (BD, 553266).
High content screening
Peripheral blood was stained with phycoerythrin-conjugated TER119 antibody, and 100μL of a 105 cells/ml suspension of cells in PBS was added to each well of a 96-well flat bottom plate. The 96-well plate was centrifuged at 440 g for 5 minutes to settle down the cells at the bottom of the well. Images were acquired by the automated Arrayscan VTI high content screening reader (Cellomics) using a 20X objective lens from 16 different fields in each well. The images were analyzed by Cellomics Target Activation BioApplication software to measure the mean cell area of TER119+ cells.
Imaging processing method
The acquired images were processed automatically using a custom program written in Matlab. First, the images were smoothed using a Gaussian kernel to reduce the noise. Then we applied the Otsu threshold to convert them to a binary image. Since the red blood cells are of regular shape, we calculated the distance function. The center of each cell, known as seeds, was detected based on the local maxima in the distance function. Seeds-controlled watershed approach was applied to segment the cells and then the cell size is calculated. A2KO HJ cells contain a blue dot (the Howell-Jolly body) as shown in , so we extracted the 3rd channel in the image, i.e. blue channel. We identified the A2KO HJ cells based on the total brightness of blue signal within each cell. The output was the Probability Density Function (PDF) of cell size. The value of a given curve, y-axis, provides the probability of observing the cell with given size, i.e., x-axis (see ).
Figure 1. Ablation of cyclin A2 in erythroid cells in vivo using erythropoietin receptor promoter-driven Cre leads to defective erythropoiesis. (A) Complete blood count data from cyclin A2fl/fl ErGFPcre mice (A2 KO, red dots, n = 11) and littermate controls (Control, blue dots, n = 9) at 3 months of age. (B-C) May-Grünwald Giemsa stained blood smears. Scale bar = 10µm. Arrows indicate erythrocytes with Howell-Jolly bodies. (D) 3D reconstruction of erythrocyte volume using z-stacks from confocal microscopy of blood smears stained with fluorescence-labeled PKH26. Representative wild-type erythrocytes (left panel, blue, inset density diagram in the middle); A2 KO (middle panel, red, inset density diagram in the middle [yellow plane indicates the cut of the inset]; and merge (right panel, inset representing 3D-view); indicating the difference in volume between wild-type and A2 KO erythrocytes. (E-F) Representative flow cytometry analysis plots of peripheral blood from age-matched control (top) and A2 KO (bottom) mice stained with antibodies against TER119 and CD71, and Hoechst33342 (n = 3). The percentage of TER119+ erythrocytes with nuclear remnants (Howell-Jolly bodies, HJ) is indicated in panel E. CD71 versus Hoechst plots for TER119+-gated peripheral blood cells with the frequencies of CD71+Hoechst− reticulocytes (RET) and CD71−Hoechst+ erythrocytes with Howell-Jolly bodies (HJ) are shown in panel F. (G) Bar graphs representing flow cytometry quantification of the percentage of erythrocytes containing Howell-Jolly bodies (HJ) in peripheral blood gated as shown in panel E, and the percentage of reticulocytes (RET) in TER119+-gated peripheral blood cells gated as shown in panel F (n = 3). (H) The mean forward scatter values of TER119+Hoechst−CD71 erythrocytes (RBC, red bars), TER119+Hoechst−CD71+ reticulocytes (RET, green bars), and TER119+Hoechst+CD71 Howell-Jolly bodies (HJ, blue bar) in the peripheral blood, gated as shown in panel F (n = 3). The forward scatter histogram overlays are shown in Figure S1C. Error bars represent standard deviation. Two-tailed t-test results are indicated by asterisks. *, p < 0.05; **, p < 0.01; ***, p < 0.001.
![Figure 1. Ablation of cyclin A2 in erythroid cells in vivo using erythropoietin receptor promoter-driven Cre leads to defective erythropoiesis. (A) Complete blood count data from cyclin A2fl/fl ErGFPcre mice (A2 KO, red dots, n = 11) and littermate controls (Control, blue dots, n = 9) at 3 months of age. (B-C) May-Grünwald Giemsa stained blood smears. Scale bar = 10µm. Arrows indicate erythrocytes with Howell-Jolly bodies. (D) 3D reconstruction of erythrocyte volume using z-stacks from confocal microscopy of blood smears stained with fluorescence-labeled PKH26. Representative wild-type erythrocytes (left panel, blue, inset density diagram in the middle); A2 KO (middle panel, red, inset density diagram in the middle [yellow plane indicates the cut of the inset]; and merge (right panel, inset representing 3D-view); indicating the difference in volume between wild-type and A2 KO erythrocytes. (E-F) Representative flow cytometry analysis plots of peripheral blood from age-matched control (top) and A2 KO (bottom) mice stained with antibodies against TER119 and CD71, and Hoechst33342 (n = 3). The percentage of TER119+ erythrocytes with nuclear remnants (Howell-Jolly bodies, HJ) is indicated in panel E. CD71 versus Hoechst plots for TER119+-gated peripheral blood cells with the frequencies of CD71+Hoechst− reticulocytes (RET) and CD71−Hoechst+ erythrocytes with Howell-Jolly bodies (HJ) are shown in panel F. (G) Bar graphs representing flow cytometry quantification of the percentage of erythrocytes containing Howell-Jolly bodies (HJ) in peripheral blood gated as shown in panel E, and the percentage of reticulocytes (RET) in TER119+-gated peripheral blood cells gated as shown in panel F (n = 3). (H) The mean forward scatter values of TER119+Hoechst−CD71 erythrocytes (RBC, red bars), TER119+Hoechst−CD71+ reticulocytes (RET, green bars), and TER119+Hoechst+CD71 Howell-Jolly bodies (HJ, blue bar) in the peripheral blood, gated as shown in panel F (n = 3). The forward scatter histogram overlays are shown in Figure S1C. Error bars represent standard deviation. Two-tailed t-test results are indicated by asterisks. *, p < 0.05; **, p < 0.01; ***, p < 0.001.](/cms/asset/1d230bb1-caa8-4212-83a0-1469f61ed987/kccy_a_1234546_f0001_c.gif)
Cumulative BrdU labeling for measurement of cell cycle length
Mice were injected intraperitonially with 100 µl of 10 mg/ml BrdU in PBS. The mice were sacrificed at the indicated time points after injection and bone marrow was harvested, followed by fixing and staining for the detection of BrdU incorporation by flow cytometry using APC BrdU Flow Kit (BD PharMingen, 552598). The cells were additionally immunostained with fluorescein isothiocyanate-conjugated CD71 antibody to specifically gate the CD71+ erythroblasts during flow cytometry analysis of BrdU labeling. The cell cycle time (Tc) and length of S-phase (Ts) was calculated from the cumulative labeling index plot as described previously.Citation23,24
Quantitative Real-time PCR analysis of cyclin A2 expression in BFU-Es and CFU-Es
Fetal liver was harvested from E13.5 C57BL/6 embryos and BFU-E and CFU-E containing fractions were purified by flow cytometry as previously described.Citation25 Total RNA for each sample was reverse-transcribed using the High Capacity cDNA Archive kit (Applied Biosystems). Relative transcript levels of cyclin A2 was quantified by SYBR Green real-time PCR using 7900HT Fast real time PCR detection system 2.2 (Applied Biosystems) and analyzed using SDS 2.2.2 software. The data was normalized to beta-actin expression. The primers used are: cyclin A2: 5′-CAACCCCGAAAAACT-GGCGC-3′ and 5′-AAGAGGAGCAACCCGTCGAG-3′; Beta-actin: 5′-ACGGCTCCGGCATGTGCAAA-3′ and 5′-TTCCC-ACCATCACACCCTGG-3′.
Western blots
Cell pellets were lysed in Laemmli buffer (60 mM Tris-HCl pH6.8; 10% glycerol; 100 mM DTT; 2% SDS) completed with Protease inhibitors (Chymostatin, Leupeptin and Pepstatin 10µg/ml), 50 mM ß-glycerophosphate, 4 mM NaF and 0.1 mM sodium orthovanadate. Lysates were homogenized using a plastic pestle and boiled for 5 minutes. 25µg of whole lysates were resolved by SDS-PAGE, transferred to PVDF membranes and blotted using the following antibodies: cyclin A (Santa Cruz, sc-596), Cdk2 affinity purified antibodies have been described previously,Citation26 Cdk1 (Santa Cruz, sc-954), phospho-Rb (BD PharMingen, 554136), phospho-Rb pT821 (Biosource-Invitrogen, 44-582G), phospho-Rb pS807/811 (Cell Signaling, 9308), cyclin D1 (NeoMarkers, Rb-010-P), cyclin E (eBioscience, 14-6714), p27 (BD Transduction, 610242), and HSP90 (BD Transduction, 610419).
Statistical analysis
Student's t test was used to determine the significance of differences between treated samples and controls. Statistical analysis was performed using Microsoft Office Excel 2007. In some cases as , we used 2-way ANOVA analysis to determine whether the variability is due to differences between experiments of Controls vs KO. We set alpha = 5.000% and the graphs show the mean with 95% confidence interval.
Results
Ablation of cyclin A2 in erythroid cells in vivo using erythropoietin receptor promoter-driven Cre leads to defective erythropoiesis
Since cyclin A2 was suggested in recent GWAS studies to be linked with erythrocyte size,Citation13,14 we investigated the role of cyclin A2 in terminal erythroid maturation in vivo by crossing cyclin A2fl/fl Citation19 mice to ErGFPcre miceCitation17 in which Cre mediated recombination of floxed alleles occurs only in late stage erythroid progenitors that express the erythropoietin receptor. Cyclin A2fl/fl ErGFPcre (A2 KO) mice were born at expected Mendelian frequencies (data not shown) and appeared overtly normal. Complete blood counts of A2 KO mice revealed increased erythrocyte size of approximately 7% (MCV or Mean Corpuscular Volume, 59.8 ± 1.0 fL in A2 KO vs 55.7 ± 1.0 fL in controls) and Mean Corpuscular Hemoglobin of 7% (MCH, 15.3 ± 0.4 pg in A2 KO vs 14.3 ± 0.4 pg in controls) but decreased erythrocyte counts of 14% (RBC, 7.9 ± 0.4 × 106/µl in A2 KO vs 9 ± 0.4 × 106/µl in controls) compared to littermate controls (). The A2 KO erythrocytes were well hemoglobinized, as indicated by their unperturbed Mean Corpuscular Hemoglobin Content (MCHC, 25.6 ± 0.5 g/dl in A2 KO vs 25.7 ± 0.8 g/dl in controls). The hemoglobin content (HGB, 12.1 ± 0.5 g/dl in A2 KO vs 12.8 ± 0.6 g/dl in controls) and hematocrit (HCT, 47.1 ± 1.9% in A2 KO vs 49.9 ± 1.9% in controls) of the A2 KO mice were slightly lower compared to littermate controls, indicating that these mice are mildly anemic. The erythrocyte distribution width (RDW, 17.9 ± 0.7% in A2 KO vs 16.3 ± 0.6% in controls) was increased by 10%, indicating greater variation in A2 KO erythrocyte size (). Microscopic examination of A2 KO peripheral blood smears () revealed an increased occurrence of erythrocytes containing Howell-Jolly (HJ) bodies, which are inclusion bodies consisting of nuclear remnants left behind as a result of defective nuclear extrusion during final stages of terminal erythroid differentiation.Citation27 Similar proportions of HJ were also found in sorted bone marrow cell populations (data not shown). Microscopic measurement using 3D-reconstruction of the cell volume revealed that the size of the A2 KO erythrocytes was increased by 7–10% in peripheral blood compared to controls (). We next quantified the erythrocytes containing Howell-Jolly bodies and reticulocytes in peripheral blood by flow cytometry analysis and found that very few control cells contained HJ [≈0.2%] (). In A2 KO peripheral blood 5–6% erythrocytes contained Howell-Jolly bodies (), which were predominantly CD71-negative (indicated as HJ in ), as well as a 2-fold increase in CD71+ reticulocytes [RET] (). The increase in reticulocytes in peripheral blood of A2 KO mice was confirmed by flow cytometry using thiazole orange (Fig. S1A-B). To confirm our erythrocyte volume measurements, we analyzed the flow cytometry forward scatter histograms (Figure S1C) and the mean forward scatter values () of the normal erythrocytes (RBC), reticulocytes (RET), and erythrocytes containing Howell-Jolly bodies (HJ). Consistent with the findings from microscopic measurement of cell volume (), the forward scatter data indicated a small cell size increase in erythrocytes of the A2 KO mice compared to wild-type controls ( and S1C). The reticulocytes and erythrocytes containing Howell-Jolly bodies displayed significantly increased mean forward scatter () compared to normal erythrocytes as expected. In summary, A2 KO mice displayed increased erythrocyte MCV and reduced erythrocyte numbers, as well as a significant increase in the number of erythrocytes with Howell-Jolly bodies. The increased erythrocyte MCV in the absence of cyclin A2 was due to a modest increase in the size of erythrocytes with a contribution of increased frequency of erythrocytes containing Howell-Jolly bodies and reticulocytes in the peripheral blood.
Analysis of erythropoiesis in cyclin A2fl/fl ErGFPcre mice
In order to identify the cause of the erythrocyte defects, we investigated erythropoiesis in adult A2 KO mice. The bone marrow cellularity of A2 KO mice appeared comparable to wild type controls (). Staining of spleen sections with antibodies against Ki-67 indicated a mild increase in proliferation in the red pulp (). Flow cytometry analysis of TER119+ spleen cells indicated an increase in the percentage of Ki-67+ cells (), but this increase did not reach statistical significance (2-tailed t-test p-value= 0.061, n = 3). The spleen size of the A2 KO mice was slightly increased () suggesting increased splenic erythropoiesis to compensate for the mild anemia observed in the A2 KO mice. The A2 KO mice recovered their hematocrit normally after a Phenylhydrazine-induced hemolytic anemia (), indicating that the loss of cyclin A2 in erythroblasts did not impair stress hematopoiesis. We next checked the relative proportions of various erythroblast subpopulations in the bone marrow and spleen ( and S2A–B). While the proportions of various erythroblast subpopulations were unperturbed in the A2 KO bone marrow (), a slight increase in TER119hiCD71loFSClo orthochromatic erythroblasts was observed in the A2 KO spleen (). Hence A2 KO mice displayed signs of increased erythropoiesis in the spleen, most likely a compensatory response to the reduced erythrocytes in circulation ().
Figure 2. Analysis of erythropoiesis in cyclin A2fl/fl ErGFPcre mice. (A-B) Hematoxylin and Eosin stained sections of control (A) and A2 KO (B) bone marrow from 12 weeks old mice. Scale bar = 100µm. (C) Absolute cell counts per femur for 12 weeks old mice (n = 3). (D-E) Staining of spleen sections from 12 weeks old control (C) and A2 KO (D) mice with antibodies against Ki-67. Scale bar = 1 mm. (F) The percentage of TER119+Ki-67+ cells in the spleen of 10 weeks old mice measured by flow cytometry (n = 3). (G) Weight of spleen of 12 weeks old A2 KO mice (n = 7) and their littermate controls (n = 6). (H) Hematocrit recovery kinetics after PHZ treatments for A2 KO mice (n = 7) and their littermate controls (n = 6). (I-J) Frequency of CD71hiTER119lo pro-erythroblasts [pro-E], TER119hiCD71hiFSChi basophilic erythroblast (EryB), TER119hiCD71hiFSClo polychromatic erythroblast (EryP) and TER119hiCD71loFSClo orthochromatic erythroblast (EryO) in bone marrow (I) and spleen (J) (n = 3). (K) Western blot analysis of total fetal liver cells isolated from A2 KO or littermate control E13.5 mouse embryos using the indicated antibodies. Hsp90 served as loading control. (L-O) Flow cytometry analysis of E13.5 fetal liver erythropoiesis. (L) Representative flow cytometry analysis plots showing the percentage of enucleated erythroid cells (ENU) and TER119+ cells containing Howell-Jolly bodies (HJ) in E13.5 fetal livers (n = 4). (M) The cell size distribution of enucleated E13.5 fetal liver erythroblasts (ENU, gated as shown in panel L) is indicated by the forward scatter histogram overlays (n = 4). (N) Bar graphs representing the percentage of enucleated erythroblasts (ENU) in E13.5 fetal livers (n = 4), gated as shown in panel L. (O) Bar graphs representing the percentage of erythroblasts containing Howell-Jolly bodies (HJ) in E13.5 fetal livers (n = 4), gated as shown in panel L. (P) The percentage of BrdU+ cells among CD71+TER119+ erythroblasts in E13.5 fetal livers (n = 4). (Q) Purified TER119-negative fetal liver erythroblasts were labeled with PKH26 dye and cultured for 48 hours. Representative PKH26 staining histogram overlays at 0 h (D0 PKH26) and 48 h (D2 PKH26) in culture are shown (n = 3). Error bars represent standard deviation. Two-tailed t-test results are indicated by asterisks. *, p < 0.05; **, p < 0.01; ***, p < 0.001.
![Figure 2. Analysis of erythropoiesis in cyclin A2fl/fl ErGFPcre mice. (A-B) Hematoxylin and Eosin stained sections of control (A) and A2 KO (B) bone marrow from 12 weeks old mice. Scale bar = 100µm. (C) Absolute cell counts per femur for 12 weeks old mice (n = 3). (D-E) Staining of spleen sections from 12 weeks old control (C) and A2 KO (D) mice with antibodies against Ki-67. Scale bar = 1 mm. (F) The percentage of TER119+Ki-67+ cells in the spleen of 10 weeks old mice measured by flow cytometry (n = 3). (G) Weight of spleen of 12 weeks old A2 KO mice (n = 7) and their littermate controls (n = 6). (H) Hematocrit recovery kinetics after PHZ treatments for A2 KO mice (n = 7) and their littermate controls (n = 6). (I-J) Frequency of CD71hiTER119lo pro-erythroblasts [pro-E], TER119hiCD71hiFSChi basophilic erythroblast (EryB), TER119hiCD71hiFSClo polychromatic erythroblast (EryP) and TER119hiCD71loFSClo orthochromatic erythroblast (EryO) in bone marrow (I) and spleen (J) (n = 3). (K) Western blot analysis of total fetal liver cells isolated from A2 KO or littermate control E13.5 mouse embryos using the indicated antibodies. Hsp90 served as loading control. (L-O) Flow cytometry analysis of E13.5 fetal liver erythropoiesis. (L) Representative flow cytometry analysis plots showing the percentage of enucleated erythroid cells (ENU) and TER119+ cells containing Howell-Jolly bodies (HJ) in E13.5 fetal livers (n = 4). (M) The cell size distribution of enucleated E13.5 fetal liver erythroblasts (ENU, gated as shown in panel L) is indicated by the forward scatter histogram overlays (n = 4). (N) Bar graphs representing the percentage of enucleated erythroblasts (ENU) in E13.5 fetal livers (n = 4), gated as shown in panel L. (O) Bar graphs representing the percentage of erythroblasts containing Howell-Jolly bodies (HJ) in E13.5 fetal livers (n = 4), gated as shown in panel L. (P) The percentage of BrdU+ cells among CD71+TER119+ erythroblasts in E13.5 fetal livers (n = 4). (Q) Purified TER119-negative fetal liver erythroblasts were labeled with PKH26 dye and cultured for 48 hours. Representative PKH26 staining histogram overlays at 0 h (D0 PKH26) and 48 h (D2 PKH26) in culture are shown (n = 3). Error bars represent standard deviation. Two-tailed t-test results are indicated by asterisks. *, p < 0.05; **, p < 0.01; ***, p < 0.001.](/cms/asset/e7b6322c-380c-412e-aa5c-a7b40371619c/kccy_a_1234546_f0002_c.gif)
In order to investigate the effect of cyclin A2 ablation on erythroblasts, we performed Western blot analysis of E13.5 A2 KO fetal liver cells, which are primarily composed of erythroid cellsCitation28 unlike adult bone marrow and spleen. Western blotting confirmed the efficient reduction but not complete ablation of cyclin A2 protein levels in the A2 KO erythroblasts (), most likely due to early erythroblasts continuing to express cyclin A2 until recombination by ErGFPcre is complete. The expression levels of its cognate partners Cdk2 and Cdk1, as well as phosphorylation of the retinoblastoma protein (Rb), were comparable to wild type controls (). Cyclin D1, cyclin E, and the Cdk inhibitor p27Kip1, which is highly expressed in late stage erythroblasts,Citation29 did not show significant changes in expression (). Cyclin E has been shown to compensate for cyclin A2 loss in MEFs but not in HSCs since cyclin E is expressed at higher levels in MEFs compared to HSCs.Citation4 Indeed, cyclin E is expressed at high levels in the E13.5 mice fetal liver,Citation30 suggesting that cyclin A2 is dispensable for erythroblasts, similar to MEFs, possibly due to the high cyclin E expression.
We investigated erythropoiesis in the fetal liver of E13.5 A2 KO embryos. The different stages of erythroid differentiation were assayed using flow cytometry analysis of CD71 and TER119 expression.Citation28 The A2 KO fetal livers displayed normal erythroid differentiation (Fig. S2C-D). The enucleated population was reduced in A2 KO fetal livers and there was a concomitant 3-fold increase in erythrocytes containing Howell-Jolly bodies (), suggesting defects in erythroid enucleation. The cell size distribution of enucleated population in the A2 KO fetal liver indicated a mild increase in the frequency of larger cells while majority of the cells displayed a near normal size distribution (). In order to detect any proliferation defects in vivo, BrdU was injected into pregnant females one hour before sacrificing and harvesting embryos. The BrdU labeling percentage among CD71+TER119+ fetal liver erythroblasts was similar between A2 KO and littermate control embryos (), indicating comparable rates of proliferation. To detect any changes in the number of erythroblasts cell divisions, purified TER119-negative fetal liver erythroid progenitors were stained with the cell membrane dye PKH26, and cultured in vitro for 48 hours. The loss of intensity of PKH26 due to erythroblast proliferation over 2 days was comparable between A2 KO and control erythroblasts (), indicating that the number of cell divisions undergone by A2 KO fetal liver erythroblasts is normal. Hence, A2 KO fetal liver erythroblasts display defects in enucleation, although proliferation and differentiation appear to be in the normal range.
Defective nuclear extrusion in cyclin A2fl/fl ErGFPcre bone marrow erythroblasts
During terminal differentiation, erythroblasts undergo approximately 4–5 rapid cell divisions accompanied by a progressive decrease in cell size, followed by exit from the cell cycle and enucleation.Citation31 Since the A2 KO mice displayed increased erythrocyte MCV associated with increased frequency of erythrocytes containing Howell-Jolly bodies in their peripheral blood (), we investigated the A2 KO bone marrow for defects in erythroid enucleation in vivo using flow cytometry. While the total frequency of CD71+TER119+ and CD71−TER119+ erythroblast subpopulations in the A2 KO bone marrow were normal (), further resolving these erythroblast subpopulations based on Hoechst staining intensities revealed the underlying enucleation defects (). The A2 KO CD71+TER119+ erythroblasts displayed a ≈10% decrease in the enucleated fraction and a corresponding increase in the nucleated fraction, as well as a 4-fold increased frequency of cells with nuclear remnants [identified as Hoechst+ cells with a Hoechst signal intensity lower than the clearly discernible nucleated cell population] (, G-I). The increase in the fraction of nucleated cells as well as cells with nuclear remnants were also displayed by the CD71−TER119+ erythroblast subpopulation (, G-I), which are normally predominantly enucleated erythrocytes resulting from maturation of CD71+TER119+ erythroblasts. Therefore, impaired erythroblast enucleation in the bone marrow leads to decreased production of enucleated erythrocytes and an increased frequency of erythrocytes containing nuclear remnants, which could be the reason for the phenotypes observed in the peripheral blood (). The cell size distribution of TER119+ erythroblasts in the A2 KO bone marrow indicated a mild increase in the frequency of larger cells ().
Figure 3. Defective nuclear extrusion in cyclin A2fl/fl ErGFPcre bone marrow erythroblasts. Freshly isolated bone marrow cells from A2 KO (n = 4) and littermate control mice (n = 3) were stained with antibodies against CD71 and TER119, as well as Hoechst33342, and analyzed by flow cytometry. Representative flow cytometry plots are shown. (A) The gating and frequencies of CD71+TER119+ and CD71−TER119+ erythroblast subpopulations are shown. (B) Gated CD71+TER119+ erythroblasts were resolved into enucleated, nucleated, and nuclear remnants-containing populations based on Hoechst staining, and their respective frequencies are shown. (C) Gated CD71−TER119+ erythroblasts were resolved into enucleated, nucleated, and nuclear remnants-containing populations based on Hoechst staining, and their respective frequencies are shown. (D) The cell size distribution of TER119+ bone marrow erythroblasts is indicated by the forward scatter histogram. (E) The cell size distribution of enucleated TER119+ bone marrow erythroblasts is indicated by the forward scatter histogram. (F) Bar graphs representing the percentages of the CD71+TER119+ and CD71−TER119+ erythroblast subpopulations in the bone marrow, gated as shown in panel A. (G) Bar graphs representing the percentages of the CD71+TER119+ and CD71−TER119+ erythroblast subpopulations that contain nuclear remnants, gated as shown in panels B and C. (H) Bar graphs representing the percentages of the CD71+TER119+ and CD71−TER119+ erythroblast subpopulations that are nucleated, gated as shown in panels B and C. (I) Bar graphs representing the percentages of the CD71+TER119+ and CD71−TER119+ erythroblast subpopulations that are enucleated, gated as shown in panels B and C. Error bars represent standard deviation. Two-tailed t-test results are indicated by asterisks. *, p < 0.05; **, p < 0.01; ***, p < 0.001.
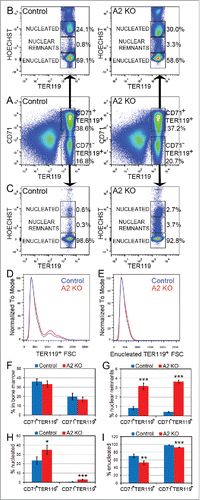
Analysis of DNA damage and cell cycle in cyclin A2fl/fl ErGFPcre bone marrow erythroblasts
Since cyclin A2 is involved in both S phase and the G2/M transition of the cell cycle through its association with Cdk2 and Cdk1,Citation10,11 we next investigated DNA damage and cell cycle progression in erythroblasts lacking cyclin A2. The A2 KO CD71+TER119+ erythroblasts displayed a 8-fold increase of phosphorylated gamma-H2AX staining (p-γH2AX, 3.3 ± 1.2% in A2 KO vs 0.4 ± 0.2% in controls, 2-tailed t-test p-value = 0.000046, n = 7) that marks DNA double strand breaks, potentially indicating defective DNA replication in the absence of cyclin A2 (). The percentage of mitotic cells in A2 KO CD71+TER119+ erythroblasts, measured by phospho-histone-H3 staining (p-H3high, 2.7 ± 0.4% in A2 KO vs 2.3 ± 0.6% in controls, 2-tailed t-test p-value = 0.25, n = 5), was similar to that of wild-type controls (). To investigate the length of various cell cycle phases in A2 KO bone marrow erythroblasts in vivo, we performed cumulative BrdU labeling (). The cell cycle time (Tc) and the length of S-phase (Ts) were calculated using the Nowakowski et al. method.Citation24 The A2 KO bone marrow CD71+ erythroblasts displayed a mild increase in total cell cycle time (10 hours in A2 KO versus 9.4 hours in control) as well as the length of S phase (3.3 hours in A2 KO vs. 3.2 hours in control), indicating a minimal reduction in erythroblast proliferation in A2 KO bone marrow. Taken together, our data indicates that most likely increased DNA damage in the absence of cyclin A2 underlie the erythroid abnormalities displayed by the A2 KO mice.
Figure 4. Analysis of DNA damage and cell cycle in cyclin A2fl/fl ErGFPcre bone marrow erythroblasts. (A) The percentage of phospho-gamma-H2AX-positive cells in the gated CD71+TER119+ erythroblasts in bone marrow from A2 KO and age-matched control mice, indicated as average ± standard deviation (n = 7). (B) The percentage of mitotic (phospho-histone-H3; p-H3high) cells in the gated CD71+TER119+ erythroblasts in bone marrow from A2 KO and age-matched control mice, indicated as average ± standard deviation (n = 5). (C) The plot of BrdU labeling index of CD71+ bone marrow erythroblasts vs. the cumulative BrdU labeling time are shown for A2 KO mice and age-matched wild-type control mice (n = 3 mice per time point). Error bars represent standard deviation. Two-tailed t-test results are indicated by asterisks. *, p < 0.05; **, p < 0.01; ***, p < 0.001.
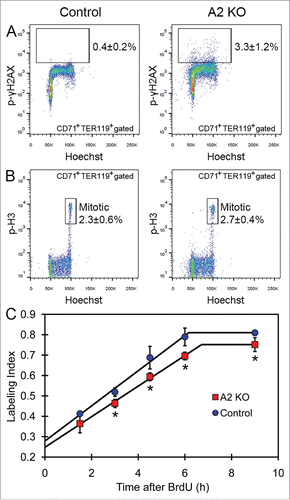
Loss of cyclin A2 in erythroid progenitors in culture
Analysis of cyclin A2fl/fl ErGFPcre (A2 KO) mice indicated DNA damage and enucleation of erythroblasts lacking cyclin A2 in vivo. In order to confirm these findings, we aimed to induce loss of cyclin A2 in primary bone marrow erythroblasts in culture using a different genetic model system. To this end, we crossed cyclin A2fl/fl to Rosa26-CreERT2Citation18 mice. Loss of cyclin A2 was induced in cultures of primary whole bone marrow cells obtained from cyclin A2fl/fl Rosa26-CreERT2 mice by the addition of 4-hydroxytamoxifen (4OHT), which leads to the recombination of the floxed cyclin A2 locus by the nuclear translocation of CreERT2.Citation18 Lineage-negative (Lin−) bone marrow erythroid progenitors were cultured in erythropoietin-containing medium for 48 hours to allow for terminal differentiation and proliferation. Ablation of cyclin A2 in cyclin A2fl/fl Rosa26-CreERT2 erythroblasts by addition of 4OHT did not affect erythroid differentiation measured by the percentage of CD71+TER119+ cells (), but resulted in a 3-fold increase in reticulocytes containing nuclear remnants () as well a significant reduction in enucleated cells (). Ablation of cyclin A2 also resulted in a small reduction in total cell counts at 48 hours for an equal starting cell number at 0 hours in culture (). Hence, the loss of cyclin A2 in cyclin A2fl/fl Rosa26-CreERT2 erythroblasts in culture confirmed enucleation defects observed in vivo in cyclin A2fl/fl ErGFPcre mice (see ).
Figure 5. Induction of cyclin A2 loss in erythroid progenitors in culture. (A-F) Whole bone marrow cells were isolated from cyclin A2fl/fl Rosa26-CreERT2 mice, or wild-type control mice, followed by lineage-depletion of the differentiated cell types. The Lin− bone marrow erythroblasts were cultured for 48 hours in erythropoietin-containing medium with 100 nM 4-hydroxytamoxifen (4OHT) or empty vehicle control (EtOH), followed by cell counting and FACS analysis. (A) Flow cytometry analysis of erythroid differentiation at 48 hours in culture by quantifying the CD71+TER119+ population, which represents the late stage erythroid cells. (B-C) Flow cytometry analysis of reticulocytes with nuclear remnants (HJ, B) and enucleated reticulocytes (Enu, C) at 48 hours in culture. (D) Total cell counts at 48 hours in culture for an equal starting cell number (10Citation5) of Lin− bone marrow erythroblasts. (E-F) Whole bone marrow cells were isolated from cyclin A2fl/flRosa26-CreERT2 mice (n = 5), or control mice (n = 4) consisting of wild type or cyclin A2+/fl Rosa26-CreERT2 mice, and 100,000 cells were plated in colony formation medium with 100 nM 4-hydroxytamoxifen (4OHT) or empty vehicle control (EtOH). CFU-E (E) and BFU-E (F) colony counts are shown. (G) Genotyping PCR performed on colonies pooled from entire plates after colony counts as shown in panels E and F. (H) Quantitative real-time PCR analysis of cyclin A2 mRNA expression on populations enriched for BFU-E (c-kit+CD71low) and CFU-E (c-kit+CD71high) purified from wild-type mice bone marrow (n = 3). Error bars represent standard deviation. Paired two-tailed t-test results are indicated by asterisks. *, p < 0.05; **, p < 0.01; ***, p < 0.001; ****, p < 0.0001. In B-C, we used a one-way ANOVA with alpha = 5.000% and the graphs show the mean with 95% confidence interval.
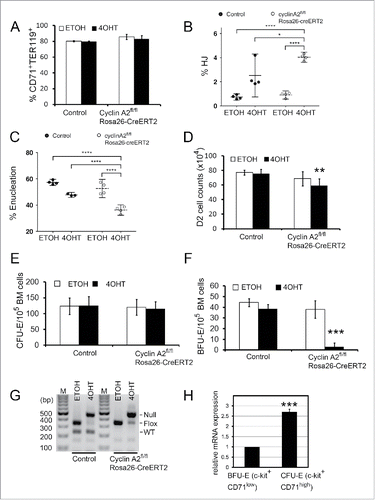
After investigating the role of cyclin A2 in late stage erythroid progenitors (CFU-Es), we aimed to test the requirement of cyclin A2 in committed early erythroid progenitors (BFU-E) using the cyclin A2fl/fl Rosa26-CreERT2 system because this was not possible using cyclin A2fl/fl ErGFPCre mice. Both BFU-E and CFU-E colonies formed readily upon 4OHT addition to control bone marrow ( and S3). In contrast, cyclin A2fl/fl Rosa26-CreERT2 bone marrow formed CFU-E colonies normally () but failed to form BFU-E colonies () upon loss of cyclin A2 after 4OHT addition, indicating that cyclin A2 is essential for BFU-E function. The combined colonies from each methylcellulose plate were genotyped to confirm the efficient recombination of the floxed cyclin A2 locus upon the addition of 4OHT (). Our data indicates that BFU-Es behave similar to HSCsCitation4 in their essential requirement for cyclin A2, while CFU-Es can broadly function in the absence of cyclin A2. This was surprising given that cyclin A2 mRNA is expressed at higher levels in CFU-Es compared to BFU-Es () as assayed in enriched cells populations of CFU-E and BFU-E.Citation25 We conclude that cyclin A2 is essential for BFU-E but not CFU-E.
Cdk2fl/fl cyclin A2fl/fl ErGFPcre mice display erythroid phenotypes similar to cyclin A2fl/fl ErGFPcre mice
Since cyclin A2 was dispensable for terminal erythroid differentiation, we aimed to check whether Cdk2 activity is essential for compensating the loss of cyclin A2 because Cdk2 can also form active complexes with cyclin E. To this end, we generated Cdk2fl/fl cyclin A2fl/fl ErGFPcre double knockout mice (A2 K2 DKO). The blood of A2 K2 DKO mice was analyzed and displayed increased erythrocyte MCV, reduced erythrocyte counts, and signs of mild anemia, similar to A2 KO mice () and Cdk2KO mice (Fig. S4). The proportion of various erythroblasts populations were unperturbed in A2 K2 DKO mice, except a significant decrease in the orthochromatic erythroblasts in the bone marrow and a significant increase in proerythroblasts in the spleen (). The A2 K2 DKO mice recovered their hematocrit normally after a Phenylhydrazine-induced hemolytic anemia (). Hence, A2 K2 DKO mice display erythroid phenotypes similar to that of A2 KO mice, indicating that the Cdk2 activity is dispensable for terminal erythropoiesis. This also indicates that the erythroid defects in the absence of cyclin A2 may be mediated through a Cdk1-dependent pathway.
Figure 6. Cdk2fl/fl cyclin A2fl/fl ErGFPcre mice display erythroid phenotypes similar to cyclin A2fl/fl ErGFPcre mice. (A) Complete blood count data from Cdk2fl/fl cyclin A2fl/fl ErGFPcre mice (A2 K2 DKO, red dots, n = 7) and aged matched wild-type control mice (Control, blue dots, n = 6). (B-C) Frequency of CD71hiTER119lo pro-erythroblasts [pro-E], TER119hiCD71hiFSChi basophilic erythroblast (EryB), TER119hiCD71hiFSClo polychromatic erythroblast (EryP) and TER119hiCD71loFSClo orthochromatic erythroblast (EryO) in bone marrow (B) and spleen (C) (n = 3). (D) Hematocrit recovery kinetics after PHZ treatments for A2 K2 DKO mice (n = 8) and their littermate controls (n = 8). Error bars represent standard deviation. Two-tailed t-test results are indicated by asterisks. *, p < 0.05; **, p < 0.01; ***, p < 0.001.
![Figure 6. Cdk2fl/fl cyclin A2fl/fl ErGFPcre mice display erythroid phenotypes similar to cyclin A2fl/fl ErGFPcre mice. (A) Complete blood count data from Cdk2fl/fl cyclin A2fl/fl ErGFPcre mice (A2 K2 DKO, red dots, n = 7) and aged matched wild-type control mice (Control, blue dots, n = 6). (B-C) Frequency of CD71hiTER119lo pro-erythroblasts [pro-E], TER119hiCD71hiFSChi basophilic erythroblast (EryB), TER119hiCD71hiFSClo polychromatic erythroblast (EryP) and TER119hiCD71loFSClo orthochromatic erythroblast (EryO) in bone marrow (B) and spleen (C) (n = 3). (D) Hematocrit recovery kinetics after PHZ treatments for A2 K2 DKO mice (n = 8) and their littermate controls (n = 8). Error bars represent standard deviation. Two-tailed t-test results are indicated by asterisks. *, p < 0.05; **, p < 0.01; ***, p < 0.001.](/cms/asset/7aeb9b7a-0490-4236-b3ed-29b2499f96b7/kccy_a_1234546_f0006_c.gif)
Loss of p27 ameliorates the defects in cyclin A2fl/fl ErGFPcre mice
Cyclin A2 functions by binding to and activating Cdk2 and Cdk1Citation10,11 with loss of cyclin A2 adversely affecting the activity of both these Cdks. The cell cycle inhibitor p27Kip1, on the other hand, inhibits the activity of Cdk2 and Cdk1 complexes.Citation32,33 If the defects resulting from the loss of cyclin A2 were mediated through impaired Cdk1 rather than Cdk2 activity, enhancing Cdk1 activity through deletion of p27 would be expected to ameliorate the defects in A2 KO mice. Therefore, we checked whether genetic ablation of p27 rescued the erythroid defects caused by the lack of cyclin A2 and generated double knockout mice. Indeed, the increased erythrocyte MCV displayed by cyclin A2fl/fl ErGFPcre (A2 KO) mice was partially rescued in A2fl/fl p27−/−ErGFPcre (DKO) mice, while the erythrocyte counts and other blood parameters were comparable between A2 KO and DKO mice (). The frequency of erythrocytes containing Howell-Jolly bodies was also slightly reduced in DKO mice compared to A2 KO mice (). Hence loss of p27 only partially rescued the erythroid defects in A2 KO mice, consistent with antagonistic roles of cyclin A2 and p27 in regulating the mammalian cell cycle. Our data reveals the regulation of fidelity of erythroid enucleation by cell cycle proteins in addition to their role in controlling the cell cycle and disruption of this regulation can result in abnormal and inefficient erythropoiesis.
Figure 7. Loss of p27 ameliorates the defects in cyclin A2fl/fl ErGFPcre mice. (A) Complete blood count data from age-matched cyclin A2fl/fl p27−/− ErGFPcre mice (DKO, green dots, n = 4), cyclin A2fl/fl ErGFPcre mice (A2 KO, red dots, n = 4), p27−/− mice (p27 KO, black dots, n = 4), and wild-type control mice (Control, blue dots, n = 4). (B-C) Flow cytometry analysis of Howell-Jolly bodies in peripheral blood of mice with the indicated genotypes (n = 3). The representative FACS plots (B) and the Bar graphs (C) are shown. Error bars represent standard deviation. Two-tailed t-test results are indicated by asterisks. *, p < 0.05; **, p < 0.01; ***, p < 0.001.
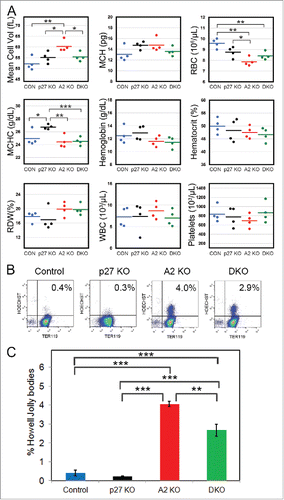
Discussion
Recent GWAS studies suggested that the cyclin A2 locus is linked to increased erythrocyte size.Citation13,14 Here, we demonstrate using erythroid-specific cyclin A2 knockout mice that impaired erythroblast enucleation lead to mildly increased erythrocyte MCV and reduced erythrocyte counts in the absence of cyclin A2. The increased erythrocyte MCV in the absence of cyclin A2 was due to a small increase in the size of erythrocytes in addition to increased frequency of erythrocytes containing Howell-Jolly bodies and reticulocytes entering the circulation. Furthermore, we identified cyclin A2 as the first cell cycle protein with a differential requirement during various stages of terminal erythropoiesis, with it being essential for BFU-E but not CFU-E.
Erythroblasts undergo a progressive decrease in cell size during terminal maturation cell divisions due to an alteration or loss of cell size control at the G1-S restriction point.Citation34 Consistent with this theory, several cell cycle proteins that control the G1-S transition, such as E2F4,Citation35-37 Cdk4/Cdk6,Citation2 cyclin D3,Citation38 and Cdk2/Cdk4,Citation20 play critical roles in the regulation of erythrocyte size and numbers. Erythroblasts lacking cyclin D3 underwent reduced number of cell divisions during terminal differentiation resulting in a dramatic 40% increase in erythrocyte MCV and 38% decrease in erythrocyte counts in the peripheral blood of cyclin D3−/− mice.Citation38 In comparison, our mouse model of erythroblast-specific deletion of cyclin A2 using ErGFPcre (A2 KO mice) displayed a modest 7% increase in erythrocyte MCV and a 12% decrease in erythrocyte counts, resulting from defects in bone marrow erythroblast enucleation. The increase in MCV in A2 KO mice was the result of an increased frequency of erythrocytes containing Howell-Jolly bodies and CD71+ reticulocytes entering the circulation in addition to an increase in size of the general erythrocyte population in all 3 dimensions. The defects in enucleation in erythroblasts deficient for cyclin A2 were independently confirmed by a recent in vitro studyCitation16 using fetal liver erythroblast culture system. The knockdown of cyclin A2 in fetal liver erythroblasts in culture using short hairpin RNAs led to significant enucleation defects,Citation16 similar to phenotypes displayed by A2 KO fetal liver erythroblasts and bone marrow in our study. Nevertheless, while they observed proliferation defects, in our study there are only mild or no effects on proliferation. These differences in the response of erythroblasts to loss of cyclin A2 are likely a result of the timing of cyclin A2 ablation and alternative compensatory mechanisms. Our erythroid-specific cyclin A2 knockout mouse model allowed us to elucidate the in vivo manifestation of cyclin A2 loss on terminal erythropoiesis including specific defects such as increased frequency of erythrocyte and bone marrow cells containing Howell-Jolly bodies. Since biochemical analysis of underlying mechanisms were complicated by the fact that loss of cyclin A2 affected only a small subpopulation of erythroblasts (∼5%), we undertook a genetics-based approach. Erythroid-specific double knockout of Cdk2 and cyclin A2 displayed phenotypes similar to A2 KO mice, indicating that the erythroid defects resulting from lack of cyclin A2 are more likely to be mediated through a Cdk1-dependent pathway. We have shown previously that Cdk1 activity can be enhanced by the loss of p27,Citation32 and therefore, we tested whether deletion of p27 can rescue some of the defects in A2 KO mice. Deletion of p27 rescued the increased MCV displayed by A2 KO erythrocytes, and also partially reduced the frequency of erythrocytes containing Howell-Jolly bodies in circulation. This indicates that erythroid defects resulting from lack of cyclin A2 are most likely mediated through Cdk1 and not Cdk2.
Enucleation of late erythroblasts in the bone marrow is impaired in the absence of cyclin A2, resulting in the reduced production of enucleated erythrocytes and an increased frequency of erythrocytes containing Howell-Jolly bodies. Since erythrocytes containing nuclear remnants form at the expense of normal erythrocytes and rarely exit bone marrow into circulation except under pathological conditions,Citation39 the net amount of normal erythrocytes entering circulation is reduced. These factors contribute to lower erythrocyte counts in peripheral blood and mild anemia in the A2 KO mice. While the mechanistic basis for enucleation defects observed in a small fraction of A2 KO erythroblasts is unclear, the increased frequency of erythroblasts with positive phospho-γH2AX staining hints at disruption of normal chromatin condensation, a process critical for erythroid enucleation.Citation22,31,40 Nevertheless, other causes of impaired enucleation such as disruption of the cytoskeletal machinery responsible for extrusion of the condensed nucleus cannot be ruled out.Citation31,41
Understanding the regulation of erythrocyte counts and size is essential for accurate identification and treatment of several hematological disorders including but not limited to macrocytic and microcytic anemia. Macrocytosis, a condition where circulating erythrocytes are larger than normal, is a relatively common finding in clinical complete blood counts with a frequency of more than 1.7%, and is presented in about 60% of cases without associated anemia.Citation42-44 It is not considered a significant complication unless accompanied by other symptoms indicative of an underlying diseased state.Citation44 While it is well established that macrocytosis is often a result of disruption of normal DNA synthesis, such as deficiency of vitamins (e.g. folate and B12) or treatment with certain drugs and chemotherapeutic agents,Citation42-44 the mechanisms remain unclear. The effects of impaired DNA synthesis are thought to be mediated mainly by impeding proliferation of rapidly dividing erythroid progenitors resulting in the production of large and immature red blood cells. Indeed, mouse knockouts of cell cycle regulators such as E2F4,Citation35-37 Cdk4/6,Citation2 and cyclin D3Citation38 display macrocytic anemia attributed to reduced number of erythroblast cell divisions during terminal erythroid differentiation. Ablation of cyclin A2 in erythroblasts results in increased DNA damage and impaired enucleation, thereby affecting erythrocyte morphology and counts. Hence, our data implies defective enucleation as an important mediator of defects in erythrocyte numbers and MCV, in addition to other factors such as DNA damage or block of differentiation.
BFU-Es differ from CFU-Es in terms of their limited but significant self renewal capacity, lower proliferation rates, and different growth factor requirements,Citation1 alluding to different regulation of their respective cell cycles. In this study, we demonstrate the differential requirement of cyclin A2 in the proliferation of BFU-Es and CFU-Es. CFU-Es resemble MEFs in being able to proliferate normally in the absence of cyclin A2.Citation4 BFU-Es, on the other hand, are similar to HSCs and embryonic stem cells in their requirement of cyclin A2,Citation4 suggesting that cyclin A2 dependency may be a feature of cells with significant self-renewal potential and a need to switch between periods of relative quiescence and active proliferation. Therefore, cyclin A2 appears be at the center of the molecular differences between proliferation of BFU-Es and CFU-Es. These findings have significant implications for therapy development for anemia that involves targeting DNA replication and cell cycle regulation.
Disclosure of potential conflicts of interest
No potential conflicts of interest were disclosed.
Author contributions
S.R.J. and P.K. designed research; S.R.J., H.A.Y-K., C.Q.W., X.B., G.X.X., and M.J.C. performed research and collected data; S.R.J., H.A.Y-K., C.Q.W., W.Y., V.T., M.O., B.L. and P.K. analyzed and interpreted data; S.R.J. and P.K. wrote the manuscript.
Supplementary File
Download MS Word (1.2 MB)Acknowledgments
The authors would like to thank Zakiah Talib and Vithya Anantaraja for animal care; Biopolis shared facilities staff for flow cytometry services, Jingxian Zhang for bioinformatics support, and the Kaldis lab for support and discussions. We acknowledge the technical expertise provided by the Advanced Molecular Pathology Laboratory at IMCB. We thank Jos Jonkers and Anton Berns for providing the Rosa26-CreERT2 mice, Ursula Klingmüller for the ErGFPcre-mice, and Padmakumar VC, Vincenzo Coppola, and Lino Tessarollo for generating the cyclin A2flox mice.
Funding
This work was supported by the Biomedical Research Council of A*STAR (Agency for Science, Technology and Research), Singapore.
References
- Lodish H, Flygare J, Chou S. From stem cell to erythroblast: regulation of red cell production at multiple levels by multiple hormones. IUBMB Life 2010; 62:492-6; PMID:20306512; http://dx.doi.org/10.1002/iub.322
- Malumbres M, Sotillo R, Santamaria D, Galan J, Cerezo A, Ortega S, Dubus P, Barbacid M. Mammalian cells cycle without the D-type cyclin-dependent kinases Cdk4 and Cdk6. Cell 2004; 118:493-504; PMID:15315761; http://dx.doi.org/10.1016/j.cell.2004.08.002
- Kozar K, Ciemerych MA, Rebel VI, Shigematsu H, Zagozdzon A, Sicinska E, Geng Y, Yu Q, Bhattacharya S, Bronson RT, et al. Mouse development and cell proliferation in the absence of D-cyclins. Cell 2004; 118:477-91; PMID:15315760; http://dx.doi.org/10.1016/j.cell.2004.07.025
- Kalaszczynska I, Geng Y, Iino T, Mizuno S, Choi Y, Kondratiuk I, Silver DP, Wolgemuth DJ, Akashi K, Sicinski P. Cyclin A is redundant in fibroblasts but essential in hematopoietic and embryonic stem cells. Cell 2009; 138:352-65; PMID:19592082; http://dx.doi.org/10.1016/j.cell.2009.04.062
- Cheng T, Rodrigues N, Shen H, Yang Y, Dombkowski D, Sykes M, Scadden DT. Hematopoietic stem cell quiescence maintained by p21cip1/waf1. Science 2000; 287:1804-8; PMID:10710306; http://dx.doi.org/10.1126/science.287.5459.1804
- Cheng T, Rodrigues N, Dombkowski D, Stier S, Scadden DT. Stem cell repopulation efficiency but not pool size is governed by p27Kip1. Nat Med 2000; 6:1235-40; PMID:11062534; http://dx.doi.org/10.1038/81335
- Zou P, Yoshihara H, Hosokawa K, Tai I, Shinmyozu K, Tsukahara F, Maru Y, Nakayama K, Nakayama KI, Suda T. p57Kip2 and p27Kip1 cooperate to maintain hematopoietic stem cell quiescence through interactions with Hsc70. Cell Stem Cell 2011; 9:247-61; PMID:21885020; http://dx.doi.org/10.1016/j.stem.2011.07.003
- Yuan Y, Shen H, Franklin DS, Scadden DT, Cheng T. In vivo self-renewing divisions of haematopoietic stem cells are increased in the absence of the early G1-phase inhibitor, p18INK4C. Nat Cell Biol 2004; 6:436-42; PMID:15122268; http://dx.doi.org/10.1038/ncb1126
- Janzen V, Forkert R, Fleming HE, Saito Y, Waring MT, Dombkowski DM, Cheng T, DePinho RA, Sharpless NE, Scadden DT. Stem-cell ageing modified by the cyclin-dependent kinase inhibitor p16INK4a. Nature 2006; 443:421-6; PMID:16957735
- Pagano M, Pepperkok R, Verde F, Ansorge W, Draetta G. Cyclin A is required at two points in the human cell cycle. EMBO J 1992; 11:961-71; PMID:1312467
- Yam CH, Fung TK, Poon RY. Cyclin A in cell cycle control and cancer. Cell Mol Life Sci 2002; 59:1317-26; PMID:12363035; http://dx.doi.org/10.1007/s00018-002-8510-y
- Murphy M, Stinnakre MG, Senamaud-Beaufort C, Winston NJ, Sweeney C, Kubelka M, Carrington M, Brechot C, Sobczak-Thepot J. Delayed early embryonic lethality following disruption of the murine cyclin A2 gene. Nat Genet 1997; 15:83-6; PMID:8988174; http://dx.doi.org/10.1038/ng0197-83
- Paul DS, Albers CA, Rendon A, Voss K, Stephens J, van der Harst P, Chambers JC, Soranzo N, Ouwehand WH, Deloukas P. Maps of open chromatin highlight cell type-restricted patterns of regulatory sequence variation at hematological trait loci. Genome Res 2013; 23:1130-41; PMID:23570689; http://dx.doi.org/10.1101/gr.155127.113
- van der Harst P, Zhang W, Mateo Leach I, Rendon A, Verweij N, Sehmi J, Paul DS, Elling U, Allayee H, Li X, et al. Seventy-five genetic loci influencing the human red blood cell. Nature 2012; 492:369-75; PMID:23222517; http://dx.doi.org/10.1038/nature11677
- Jeffrey PD, Russo AA, Polyak K, Gibbs E, Hurwitz J, Massague J, Pavletich NP. Mechanism of CDK activation revealed by the structure of a cyclinA-CDK2 complex. Nature 1995; 376:313-20; PMID:7630397; http://dx.doi.org/10.1038/376313a0
- Ludwig LS, Cho H, Wakabayashi A, Eng JC, Ulirsch JC, Fleming MD, Lodish HF, Sankaran VG. Genome-wide association study follow-up identifies cyclin A2 as a regulator of the transition through cytokinesis during terminal erythropoiesis. Am J Hematol 2015; 90:386-91; PMID:25615569; http://dx.doi.org/10.1002/ajh.23952
- Heinrich AC, Pelanda R, Klingmuller U. A mouse model for visualization and conditional mutations in the erythroid lineage. Blood 2004; 104:659-66; PMID:15090451; http://dx.doi.org/10.1182/blood-2003-05-1442
- Vooijs M, Jonkers J, Berns A. A highly efficient ligand-regulated Cre recombinase mouse line shows that LoxP recombination is position dependent. EMBO Rep 2001; 2:292-7; PMID:11306549; http://dx.doi.org/10.1093/embo-reports/kve064
- Gopinathan L, Tan SL, Padmakumar VC, Coppola V, Tessarollo L, Kaldis P. Loss of Cdk2 and cyclin A2 impairs cell proliferation and tumorigenesis. Cancer Res 2014; 74:3870-9; PMID:24802190; http://dx.doi.org/10.1158/0008-5472.CAN-13-3440
- Jayapal SR, Wang CQ, Bisteau X, Caldez MJ, Lim S, Tergaonkar V, Osato M, Kaldis P. Hematopoiesis specific loss of Cdk2 and Cdk4 results in increased erythrocyte size and delayed platelet recovery following stress. Haematologica 2015; 100:431-8; PMID:25616574; http://dx.doi.org/10.3324/haematol.2014.106468
- Kiyokawa H, Kineman RD, Manova-Todorova KO, Soares VC, Hoffman ES, Ono M, Khanam D, Hayday AC, Frohman LA, Koff A. Enhanced growth of mice lacking the cyclin-dependent kinase inhibitor function of p27Kip1. Cell 1996; 85:721-32; PMID:8646780; http://dx.doi.org/10.1016/S0092-8674(00)81238-6
- Jayapal SR, Lee KL, Ji P, Kaldis P, Lim B, Lodish HF. Down-regulation of Myc is essential for terminal erythroid maturation. J Biol Chem 2010; 285:40252-65; PMID:20940306; http://dx.doi.org/10.1074/jbc.M110.181073
- Lim S, Kaldis P. Loss of Cdk2 and Cdk4 induces a switch from proliferation to differentiation in neural stem cells. Stem Cells 2012; 30:1509-20; PMID:22532528; http://dx.doi.org/10.1002/stem.1114
- Nowakowski RS, Lewin SB, Miller MW. Bromodeoxyuridine immunohistochemical determination of the lengths of the cell cycle and the DNA-synthetic phase for an anatomically defined population. J Neurocytol 1989; 18:311-8; PMID:2746304; http://dx.doi.org/10.1007/BF01190834
- Flygare J, Rayon Estrada V, Shin C, Gupta S, Lodish HF. HIF1alpha synergizes with glucocorticoids to promote BFU-E progenitor self-renewal. Blood 2011; 117:3435-44; PMID:21177435; http://dx.doi.org/10.1182/blood-2010-07-295550
- Berthet C, Aleem E, Coppola V, Tessarollo L, Kaldis P. Cdk2 knockout mice are viable. Curr Biol 2003; 13:1775-85; PMID:14561402; http://dx.doi.org/10.1016/j.cub.2003.09.024
- Sears DA, Udden MM. Howell-Jolly bodies: a brief historical review. Am J Med Sci 2012; 343:407-9; PMID:21946828; http://dx.doi.org/10.1097/MAJ.0b013e31823020d1
- Zhang J, Socolovsky M, Gross AW, Lodish HF. Role of Ras signaling in erythroid differentiation of mouse fetal liver cells: functional analysis by a flow cytometry-based novel culture system. Blood 2003; 102:3938-46; PMID:12907435; http://dx.doi.org/10.1182/blood-2003-05-1479
- Hsieh FF, Barnett LA, Green WF, Freedman K, Matushansky I, Skoultchi AI, Kelley LL. Cell cycle exit during terminal erythroid differentiation is associated with accumulation of p27Kip1 and inactivation of cdk2 kinase. Blood 2000; 96:2746-54; PMID:11023508
- Geng Y, Yu Q, Whoriskey W, Dick F, Tsai KY, Ford HL, Biswas DK, Pardee AB, Amati B, Jacks T, et al. Expression of cyclins E1 and E2 during mouse development and in neoplasia. Proc Natl Acad Sci USA 2001; 98:13138-43; PMID:11687642; http://dx.doi.org/10.1073/pnas.231487798
- Ji P, Murata-Hori M, Lodish HF. Formation of mammalian erythrocytes: chromatin condensation and enucleation. Trends Cell Biol 2011; 21:409-15; PMID:21592797; http://dx.doi.org/10.1016/j.tcb.2011.04.003
- Aleem E, Kiyokawa H, Kaldis P. Cdc2-cyclin E complexes regulate the G1/S phase transition. Nat Cell Biol 2005; 7:831-6; PMID:16007079; http://dx.doi.org/10.1038/ncb1284
- Kaldis P. Another piece of the p27Kip1 puzzle. Cell 2007; 128:241-4; PMID:17254963; http://dx.doi.org/10.1016/j.cell.2007.01.006
- Dolznig H, Bartunek P, Nasmyth K, Mullner EW, Beug H. Terminal differentiation of normal chicken erythroid progenitors: shortening of G1 correlates with loss of D-cyclin/cdk4 expression and altered cell size control. Cell Growth Differ 1995; 6:1341-52; PMID:8562472
- Humbert PO, Rogers C, Ganiatsas S, Landsberg RL, Trimarchi JM, Dandapani S, Brugnara C, Erdman S, Schrenzel M, Bronson RT, et al. E2F4 is essential for normal erythrocyte maturation and neonatal viability. Mol Cell 2000; 6:281-91; PMID:10983976; http://dx.doi.org/10.1016/S1097-2765(00)00029-0
- Kinross KM, Clark AJ, Iazzolino RM, Humbert PO. E2f4 regulates fetal erythropoiesis through the promotion of cellular proliferation. Blood 2006; 108:886-95; PMID:16861343; http://dx.doi.org/10.1182/blood-2005-09-008656
- Rempel RE, Saenz-Robles MT, Storms R, Morham S, Ishida S, Engel A, Jakoi L, Melhem MF, Pipas JM, Smith C, et al. Loss of E2F4 activity leads to abnormal development of multiple cellular lineages. Mol Cell 2000; 6:293-306; PMID:10983977; http://dx.doi.org/10.1016/S1097-2765(00)00030-7
- Sankaran VG, Ludwig LS, Sicinska E, Xu J, Bauer DE, Eng JC, Patterson HC, Metcalf RA, Natkunam Y, Orkin SH, et al. Cyclin D3 coordinates the cell cycle during differentiation to regulate erythrocyte size and number. Genes Dev 2012; 26:2075-87; PMID:22929040; http://dx.doi.org/10.1101/gad.197020.112
- Schwartz SO, Stansbury F. Significance of nucleated red blood cells in peripheral blood; analysis of 1,496 cases. J Am Med Assoc 1954; 154:1339-40; PMID:13151849; http://dx.doi.org/10.1001/jama.1954.02940500019007
- Ji P, Yeh V, Ramirez T, Murata-Hori M, Lodish HF. Histone deacetylase 2 is required for chromatin condensation and subsequent enucleation of cultured mouse fetal erythroblasts. Haematologica 2010; 95:2013-21; PMID:20823130; http://dx.doi.org/10.3324/haematol.2010.029827
- Ji P, Jayapal SR, Lodish HF. Enucleation of cultured mouse fetal erythroblasts requires Rac GTPases and mDia2. Nat Cell Biol 2008; 10:314-21; PMID:18264091; http://dx.doi.org/10.1038/ncb1693
- Aslinia F, Mazza JJ, Yale SH. Megaloblastic anemia and other causes of macrocytosis. Clin Med Res 2006; 4:236-41; PMID:16988104; http://dx.doi.org/10.3121/cmr.4.3.236
- Colon-Otero G, Menke D, Hook CC. A practical approach to the differential diagnosis and evaluation of the adult patient with macrocytic anemia. Med Clin North Am 1992; 76:581-97; PMID:1578958; http://dx.doi.org/10.1016/S0025-7125(16)30341-8
- Kaferle J, Strzoda CE. Evaluation of macrocytosis. Am Fam Physician 2009; 79:203-8; PMID:19202968