ABSTRACT
Snail and Slug are zinc-finger transcription factors that play key roles in directing the epithelial-mesenchymal transition (EMT) programs associated with normal development as well as disease progression. More recent work suggests that these EMT-associated transcription factors also modulate the function of both embryonic and adult stem cells. Interestingly, YAP and TAZ, the co-transcriptional effectors of the Hippo pathway, likewise play an important role in stem cell self-renewal and lineage commitment. While direct intersections between the Snail/Slug and Hippo pathways have not been described previously, we recently described an unexpected cooperative interaction between Snail/Slug and YAP/TAZ that controls the self-renewal and differentiation properties of bone marrow-derived mesenchymal stem cells (MSCs), a cell population critical to bone development. Additional studies revealed that both Snail and Slug are able to form binary complexes with either YAP or TAZ that, together, control YAP/TAZ transcriptional activity and function throughout mouse development. Given the more recent observations that MSC-like cell populations are found in association throughout the vasculature where they participate in tissue regeneration, fibrosis and cancer, the Snail/Slug-YAP/TAZ axis is well-positioned to regulate global stem cell function in health and disease.
Mesenchymal stem/stromal cells (MSCs) are a specialized population of progenitor cells that are positioned throughout host tissues during embryogenesis and postnatal life.Citation1,2 Though the full multipotent range of these cells remains the subject of ongoing debate, bone marrow-derived MSCs (also referred to as skeletal stem cells) of either mesodermal or neural crest origin are able to differentiate into osteoblasts, chondrocytes or adipocytes as well as haematopoietic stem cell-supportive stroma.Citation2-4 Loss of stem cell function not only leads to developmental defects, but also contributes to postnatal osteoporosis with associated defects in fracture repair, haematopoietic potential and tissue homeostasis.Citation1,2 Despite their importance, however, the molecular mechanisms by which MSC self-renewal and function are maintained in the in vivo setting remain unclear.
The zinc-finger transcriptional repressors, Snail and Slug (alternatively termed, Snail1 and Snail2, respectively), are best known for their respective roles in orchestrating the epithelial-mesenchymal transition (EMT) programs normally associated with embryogenesis, early development and a growing list of pathologic states, ranging from cancer to fibrosis.Citation5-12 More recently, however, Snail and Slug functions have been expanded to include the control of fate determination in stem cell populations where expression of either transcription factor has been proposed to selectively modulate self-renewal, pluripotency, proliferative activity and senescence.Citation9,13-22 Nevertheless, the ability of endogenous Snail or Slug to regulate MSC function in the in vivo setting has, until recently, only been the subject of speculation.
In recent work, we discovered that Snail and Slug are co-expressed in bone marrow MSCs during both pre- and post- natal states in vivo.Citation23 Though effects of Snail/Slug on stem cell function are most frequently assigned to embryonic or epithelial cell populations,Citation5-7 these data demonstrated that MSCs – despite their mesenchymal origin – co-express both transcription factors, reinforcing the fact that these transcription factors assume functional roles outside of classic EMT-related programs.Citation24,25 However, following in vivo targeting of either MSC-derived Snail or Slug alone, we noted only subtle defects in bone development.Citation23 These results contrasted with earlier reports proposing roles for Snail alone in regulating osteoblastogenesis.Citation25-27 Likewise, whereas individual roles for either Snail or Slug have been linked to the control of chondrogenesis,Citation28,29 we were unable to document frank defects in cartilage development, a finding similar to that first reported by Gridley and colleagues.Citation23,30 Despite these negative results, spurred by recent reports documenting the compensatory regulation of Snail and Slug during early cartilage formation as well as craniofacial development,Citation30-32 we elected to target both MSC transcription factors in tandem. Remarkably, under these conditions, MSC self-renewal and differentiation were impaired strikingly with attendant defects in osteoprogenitor function that completely undermined bone formation in vivo as well as terminal osteoblast differentiation in vitro.Citation23 These results should not, however, be construed to suggest that Snail and Slug necessarily compensate for one another under all circumstances. For example, the tissue-specific deletion of Snail alone undermines developmental programs associated with vascular development,Citation33 intestinal homeostasis,Citation19 pancreatic acinar cell maintenanceCitation22 and neural maturation.Citation34 Likewise, Slug alone appears to regulate adipogenesis via a Snail-independent process while Snail can, in and of itself, modulate insulin-induced lipolysis.Citation35,36 Indeed, Snail and Slug function may even exert opposite effects in selected events, such as stem cell reprogramming.Citation37 Nevertheless, similar to defects observed in chondrocytes or neural crest stem cells,Citation30-32 only the dual loss of Snail and Slug unmasked the key roles played by these transcription factors in developing bone.Citation23
From a functional perspective, the ability of Snail and Slug to cooperatively regulate MSC proliferation as well as differentiation was unexpected, leaving us to conjecture as to how those transcription factors might control bone formation. We were, however, intrigued by the fact that the observed effects of Snail/Slug targeting on MSC self-renewal and lineage commitment dovetailed with those previously ascribed to the transcriptional co-activators, Yes-associated protein (YAP) and transcriptional co-activator with PDZ-binding motif (TAZ).Citation38-43 These molecules, through their direct binding interactions with the TEAD family of transcription factors, serve as key mediators of the Hippo pathway that regulates organ size, cell proliferation and cell fate determination.Citation38-43 Seminal work in the field had also established the fact that YAP and TAZ play critical roles in stem cell self-renewal, maintenance and expansion.Citation38-44 Indeed, with specific regard to bone marrow-derived MSCs, YAP/TAZ not only control stem cell fate, but also serve as downstream mediators of cell-extracellular matrix interactions.Citation45,46 Though the possibility that Snail and Slug coordinate MSC behavior by working in tandem with the YAP and TAZ axis had not been explored previously, we further noted that YAP and TAZ, like Snail/Slug, bind to 14.3.3, translocate from the cytosolic to nuclear compartment, undergo phosphorylation-dependent β-TRCP-mediated ubiquitination and proteasomal degradation, interface with the canonical Wnt pathway as well as TGFβ signaling, and are responsive to mechanical inputs.Citation6-8,38-43 Furthermore, like Snail and Slug, several recent reports have established compensatory roles for YAP and TAZ during development.Citation47-53 Indeed, these multiple, but potentially coincidental, similarities proved informative as we found that Snail and Slug control both the transcriptional and post-transcriptional regulation of YAP/TAZ in mouse as well as human MSCs.Citation23 That is, in the combined absence of both Snail and Slug, YAP/TAZ protein, but not mRNA, levels decreased markedly.Citation23 Likewise, Snail/Slug co-deletion inhibited expression of multiple YAP/TAZ-TEAD targets, including Ctgf, Ankrd1, Axl, Dkk1 and Cyr61 in vitro as well as in vivo.Citation23 Interestingly, effects on gene expression did not reflect global changes in MSC transcriptional program as Runx2 expression (both at the mRNA and protein levels), a master regulator of bone and cartilage development,Citation54-56 remained unaffected. Indeed, changes in stem cell-associated transcription factors, e.g., Nanog, Oct4 or Sox2, are not detected in Snail/Slug double-null MSCs relative to wild-type controls (). Hence, despite the combined absence of Snail/Slug, MSCs commit to the osteoprogenitor lineage in normal fashion, but are unable to complete the terminal steps of the osteoblastogenic program in vitro or in vivo.Citation23
Figure 1. Relative expression of stem cell markers in MSCs isolated from Snailf/f/Slugf/f mice and transduced with adenoviral- GFP or Cre expression vectors.
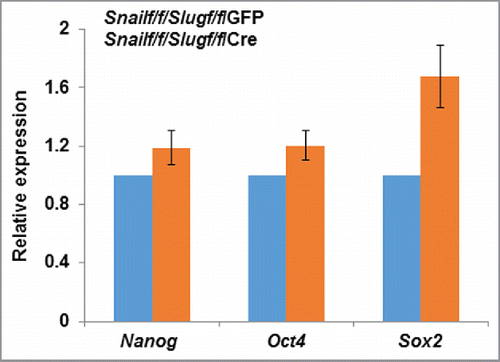
Under normal conditions, YAP/TAZ stability is regulated, in large part, by the Hippo cascade-associated kinases, MST1 and MST2 as well as the Lats kinases, LATS1 and LATS2; the latter kinases mediating YAP/TAZ phosphorylation, thereby controlling YAP/TAZ subcellular localization and proteosomal degradation.Citation38-43 However, Snail/Slug co-deletion did not affect MST or LATS activity in isolated MSCs.Citation23 Nevertheless, in the absence of Snail/Slug, the levels of phosphorylated YAP/TAZ were markedly increased with concomitant decreases in YAP/TAZ protein half-lives and steady-state levels.Citation23 As such, next considered the possibility that Snail/Slug might modulate YAP/TAZ function via direct binding interactions given recent precedents wherein i) the tyrosine phosphatase, SHP2, was reported to bind and modulate YAP/TAZ-TEAD-dependent transcriptional activity Citation57 ii) and the transcription factor, TXB5, was found to form function-modulating complexes with YAP and β-catenin.Citation58 Indeed, we found that biomolecular complexes were readily detected between Snail and either YAP or TAZ, or alternatively, between Slug and YAP or TAZ, both in vitro and in vivo.Citation23 Consistent with the fact that binding interactions between YAP/TAZ and nuclear transcription factors are mediated by their respective WW domains,Citation38,39 the formation of Snail/Slug-YAP/TAZ complexes in MSCs was likewise WW domain-dependent.Citation23 In turn, Snail/Slug binding interactions with the YAP/TAZ WW domains were dependent on their extended, N-terminal SNAG domains that also serve to recruit chromatin modifying enzymes critical for Snail/Slug-dependent transcriptional regulation.Citation5,6 WW domains classically interact with protein partners via embedded PPxY motifs,Citation38,39,59 but the extended SNAG domains of neither Snail nor Slug include this sequence.Citation5 Though additional molecules might be integrated into Snail/Slug-YAP/TAZ complexes that serve to bridge these proteins together, we find that bacterially-expressed Snail/Slug and YAP/TAZ are able to form binary complexes directly (). Interestingly, the extended SNAG domains of Snail and Slug contain a conserved PxY motif that potentially participates in YAP/TAZ binding,Citation5 but further studies are needed to identify the critical sequences involved in WW-Snail/Slug domain interactions and whether these interactions might apply to other members of the Snail Family.Citation60
Figure 2. Snail/Slug-YAP/TAZ binding interactions. GST-YAP, GST-TAZ or GST alone were bound to glutathione beads and incubated with recombinant Flag-tagged Snail or Slug proteins. Aliquots of Flag-tagged proteins and each bound fraction were subjected to SDS-PAGE and immunoblotted with the anti- Flag and GST antibodies.
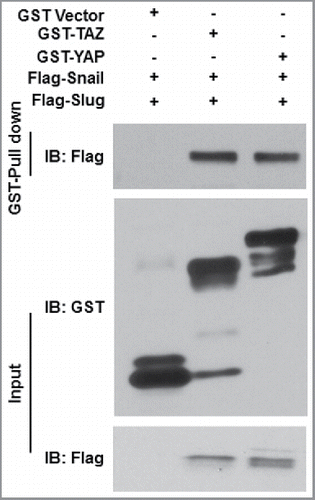
Given the identification of Snail/Slug-YAP/TAZ binding interactions, their potential functional significance was underlined following the detection of these complexes in the promoter regions of a series of YAP/TAZ-TEAD-targeted genes.Citation23 Likewise, confirming and extending earlier studies describing the ability of Runx2 to bind YAP or TAZ directly,Citation61,62 Runx2-YAP/TAZ-Snail/Slug complexes were likewise identified in the promoter region of multiple genes known to regulate osteoblast differentiation and function.Citation23 A requirement for Snail/Slug-YAP/TAZ complex formation in MSC differentiation programs was further reinforced by the ability of a dominant-negative Snail-deletion mutant that efficiently blocks binding interactions between endogenous Snail/Slug and YAP/TAZ, to completely inhibit osteoblastogenesis in a fashion that recapitulated the phenotype of Snail/Slug-null or YAP/TAZ hemizygote MSCs.Citation23 Hence, in the absence of Snail and Slug, YAP/TAZ alone is unable to support bone development while partial, but combined, deficiencies of YAP and TAZ undermine the ability of Snail and Slug to drive osteoblastogenesis.Citation23 Taken together, these data describe a model where, Snail, Slug, YAP and TAZ play critical roles in cooperatively regulating the transcriptional events downstream of Runx2 activation that play required roles in osteoblast differentiation and bone formation ().Citation23 Interestingly, whole-genome ChIP studies have identified both TEAD and Runx family members as YAP-binding transcription factors.Citation62-65 However, at least in the case of YAP/TEAD complexes, only a handful of target genes were identified whose expression was regulated by YAP-dependent binding in specific promoter regions.Citation62-64 Instead, transcriptional control was found to be exerted by YAP/TEAD complex-mediated action on distal enhancers that contacted target promoters through chromatin looping.Citation62-65 Even with this information in hand, the transcriptional targets regulated by these types of interactions in MSCs remains to be determined as DNA-binding sites for YAP-TEAD complexes, to date analyzed most frequently in cancer cells, are distinct when comparing transformed to non-transformed cell lines.Citation63
Until recently, bone marrow MSCs were often times treated as a homogenous population.Citation1,2 However, it is now appreciated that multiple and distinct MSC populations participate in early bone formation as opposed to postnatal skeletal homeostasis and fracture repair.Citation1-4,66-68 As such, these findings raise the question as to whether the functional roles defined for Snail/Slug-YAP/TAZ complexes are confined to early MSC development or extend into the adult setting.Citation66-68 We found, however, that MSCs recovered from adult mice continue to rely on Snail/Slug-YAP/TAZ activity to maintain self-renewal properties as well as undergo osteoblastogenesis.Citation23 Nevertheless, Snail/Slug-YAP/TAZ-dependent MSC function is unlikely to be maintained throughout the lifespan of normal mice. Indeed, we find that the well-known decrease in the size and function of MSC reserve pools that occurs during aging Citation69 coincides with marked losses in Snail, Slug, and YAP/TAZ expression levels (). Given complementary studies demonstrating important roles for Snail/Slug as well as YAP in chondrogenesis,Citation30,70 the identification of the factors that regulate MSC Snail/Slug and YAP/TAZ levels could have important ramifications for improving overall skeletal health in aging populations. Likewise, we presume that it will prove informative to identify the mediators that control the Snail/Slug-YAP/TAZ axis and the ability of MSCs to re-engage this pathway as a function of age following bone or cartilage damage.
Figure 4. Expression of Snail and Slug in young versus aged mice. (A) LacZ expression in 10 day-old vs. 6 month-old parietal bone. Scale bar: 50 µm. (B) LacZ expression in 2 day-old vs. 12 month-old mice femur. (C) LacZ expression in 10 day-old vs. 6 month-old mice femur. Scale bar: 100 µm.
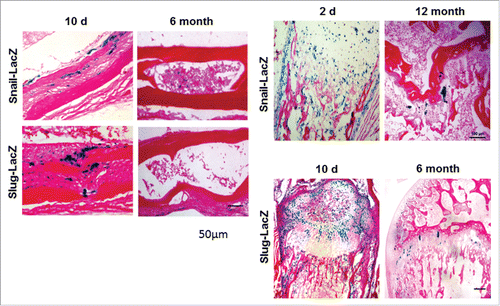
Figure 5. Expression of YAP and TAZ in young vs. aged mice. Immunostaining of YAP/TAZ in the femurs of 2 day-old vs. 8 month-old mice are shown. Scale bar: 100 µm.
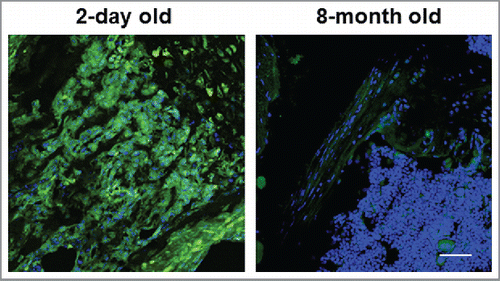
Studies performed to date have focused on the regulation of the Snail/Slug-YAP/TAZ axis in bone marrow-derived MSCs, but pericyte-like MSCs are now known to be associated with the vasculature throughout the body where they have likewise been linked to events ranging from tissue repair and cancer to fibrosis.Citation71-76 As Snail/Slug as well as YAP/TAZ can participate in all of these events,Citation7,42,43 we presume that the functional roles for the Snail/Slug-YAP/TAZ axis outlined herein may also extend to MSC function outside the confines of the bony skeleton, or even the vasculature.Citation77 Further, as cancer cells themselves can display stem cell-like characteristics,Citation8,9,13,16-18 and are known to express Snail/Slug as well as YAP/TAZ, functional roles for the Snail/Slug-YAP/TAZ axis in neoplastic processes bear further consideration. Finally, from the perspective of YAP/TAZ function, limiting such analyses to interactions with Snail/Slug alone is likely premature as complementary studies have recently established similar binding interactions between YAP and ZEB1, an additional, but structurally distinct, member of the family of EMT-associated zinc-finger transcription factors.Citation6,8,78 In this case, the interaction of ZEB1 and YAP switches ZEB1 from a transcriptional repressor to a transcriptional co-activator that promotes stemness, proliferation, metastatic activity and chemoresistance in breast carcinoma cells.Citation78 As such, and given the widespread expression of Snail/Slug as well as YAP/TAZ in both epithelial as well as mesenchymal cell populations in vivo, the interacting transcriptional networks we have outlined herein will likely prove pertinent to the regulation of stem cell-associated programs in a wide variety of both normal and neoplastic states.
Methods
Mice
Snailflox/flox and Snail+/LacZ mice were generated as described previously and maintained on a C57BL/6 genetic background.Citation15,24,33 YAPf/f/TAZf/f mice were previously developed and provided by E. Olsen.Citation47 Slug+/LacZ mice were obtained from T. Gridley and maintained on a C57BL/6 background.Citation79 All mouse work was performed with IACUC approval.
GST-pull down assay
Flag-Snail and Flag-Slug expression plasmids Citation23 were transfected into HEK-293 cells, and cell lysates were harvested 2 d after transfection. The Flag-Snail and Flag-Slug fusion proteins were isolated and purified with Anti-Flag M2 affinity resin (Sigma-Aldrich), and the purified proteins eluted with a Flag peptide (Sigma-Aldrich). GST-YAP and GST-TAZ (Addgene) fusion proteins were produced in bacteria and purified by glutathione-agarose affinity chromatography. Protein-protein interactions were determined using a GST-Pull Down assay kit according to the manufacturer's instructions (Thermo Scientific).
RT-PCR
Bone marrow MSCs have been isolated and cultured as described previously.Citation23 Total RNA was harvested from the cultured cells and RT-PCR performed according to the manufacturer's instructions (Power SYBR Green, Applied Biosystems). Primers for Nanog, forward: AGGGTCTGCTACTGAGATGCTCTG and reverse: CAACCACTGGTTTTTCTGCCACCG. Oct4, Forward: TGGAGACTTTGCAGCCTGAG and reverse: AAGCGACAGATGGTGGTCTG. Sox2, Forward: GAAGGGGAGAGATTTTCAAAG and the reverse: CCAGATCTATACATGGTCCGATTCCC.
LacZ staining and immunostaining
Mouse bone was harvested and the LacZ staining and immunostaining performed as described.Citation23
Disclosure of potential conflicts of Interest
The authors declare no conflict of interest.
Funding
This work is supported by the National Institutes of Health under grant number AR065524 (SJW) and the Breast Cancer Research Foundation (SJW).
References
- Kfoury Y, Scadden DT. Mesenchymal cell contributions to the stem cell niche. Cell Stem Cell 2015; 16:239-53; PMID: 25748931; http://dx.doi.org/10.1016/j.stem.2015.02.019
- Bianco P, Robey PG. Skeletal stem cells. Development 2015; 142:1023-7; PMID: 25758217; http://dx.doi.org/10.1242/dev.102210
- Isern J, Garcia-Garcia A, Martin AM, Arranz L, Martin-Perez D, Torroja C, Sanchez-Cabo F, Mendez-Ferrer S. The neural crest is a source of mesenchymal stem cells with specialized hematopoietic stem cell niche function. ELife 2014; 3:e03696; PMID: 25255216; http://dx.doi.org/10.7554/eLife.03696
- Ono N, Ono W, Nagasawa T, Kronenberg HM. A subset of chondrogenic cells provides early mesenchymal progenitors in growing bones. Nat Cell Biol 2014; 16:1157-67; PMID: 25419849; http://dx.doi.org/10.1038/ncb3067
- Barrallo-Gimeno A, Nieto MA. Evolutionary history of the Snail/Scratch superfamily. Trends Genet 2009; 25:248-52; PMID: 19427053; http://dx.doi.org/10.1016/j.tig.2009.04.001
- Nieto MA. Epithelial plasticity: a common theme in embryonic and cancer cells. Science 2013; 342:1234850; PMID: 24202173; http://dx.doi.org/10.1126/science.1234850
- Nieto MA, Huang RY, Jackson RA, Thiery JP. EMT: 2016. Cell 2016; 166:21-45; PMID: 27368099; http://dx.doi.org/10.1016/j.cell.2016.06.028
- Puisieux A, Brabletz T, Caramel J. Oncogenic roles of EMT-inducing transcription factors. Nat Cell Biol 2014; 16:488-94; PMID: 24875735; http://dx.doi.org/10.1038/ncb2976
- Ni T, Li XY, Lu N, An T, Liu ZP, Fu R, Lv WC, Zhang YW, Xu XJ, Grant Rowe R, et al. Snail1-dependent p53 repression regulates expansion and activity of tumour-initiating cells in breast cancer. Nat Cell Biol 2016; 18:1221-32; PMID: 27749822; http://dx.doi.org/10.1038/ncb3425
- Alba-Castellon L, Batlle R, Franci C, Fernandez-Acenero MJ, Mazzolini R, Pena R, Loubat J, Alameda F, Rodriguez R, Curto J, et al. Snail1 expression is required for sarcomagenesis. Neoplasia 2014; 16:413-21; PMID: 24947186; http://dx.doi.org/10.1016/j.neo.2014.05.002
- Grande MT, Sanchez-Laorden B, Lopez-Blau C, De Frutos CA, Boutet A, Arevalo M, Rowe RG, Weiss SJ, Lopez-Novoa JM, Nieto MA. Snail1-induced partial epithelial-to-mesenchymal transition drives renal fibrosis in mice and can be targeted to reverse established disease. Nat Med 2015; 21:989-97; PMID: 26236989; http://dx.doi.org/10.1038/nm.3901
- Lovisa S, LeBleu VS, Tampe B, Sugimoto H, Vadnagara K, Carstens JL, Wu CC, Hagos Y, Burckhardt BC, Pentcheva-Hoang T, et al. Epithelial-to-mesenchymal transition induces cell cycle arrest and parenchymal damage in renal fibrosis. Nat Med 2015; 21:998-1009; PMID: 26236991; http://dx.doi.org/10.1038/nm.3902
- Guo W, Keckesova Z, Donaher JL, Shibue T, Tischler V, Reinhardt F, Itzkovitz S, Noske A, Zurrer-Hardi U, Bell G, et al. Slug and Sox9 cooperatively determine the mammary stem cell state. Cell 2012; 148:1015-28; PMID: 22385965; http://dx.doi.org/10.1016/j.cell.2012.02.008
- Soleimani VD, Yin H, Jahani-Asl A, Ming H, Kockx CE, van Ijcken WF, Grosveld F, Rudnicki MA. Snail regulates MyoD binding-site occupancy to direct enhancer switching and differentiation-specific transcription in myogenesis. Mol Cell 2012; 47:457-68; PMID: 22771117; http://dx.doi.org/10.1016/j.molcel.2012.05.046
- Lin Y, Li XY, Willis AL, Liu C, Chen G, Weiss SJ. Snail1-dependent control of embryonic stem cell pluripotency and lineage commitment. Nat Commun 2014; 5:3070; PMID: 24401905
- Desgrosellier JS, Lesperance J, Seguin L, Gozo M, Kato S, Franovic A, Yebra M, Shattil SJ, Cheresh DA. Integrin alphavbeta3 drives Slug activation and stemness in the pregnant and neoplastic mammary gland. Dev Cell 2014; 30:295-308; PMID: 25117682; http://dx.doi.org/10.1016/j.devcel.2014.06.005
- Hwang WL, Jiang JK, Yang SH, Huang TS, Lan HY, Teng HW, Yang CY, Tsai YP, Lin CH, Wang HW, et al. MicroRNA-146a directs the symmetric division of Snail-dominant colorectal cancer stem cells. Nat Cell Biol 2014; 16:268-80; PMID: 24561623; http://dx.doi.org/10.1038/ncb2910
- Ye X, Tam WL, Shibue T, Kaygusuz Y, Reinhardt F, Ng Eaton E, Weinberg RA. Distinct EMT programs control normal mammary stem cells and tumour-initiating cells. Nature 2015; 525:256-60; PMID: 26331542; http://dx.doi.org/10.1038/nature14897
- Horvay K, Jarde T, Casagranda F, Perreau VM, Haigh K, Nefzger CM, Akhtar R, Gridley T, Berx G, Haigh JJ, et al. Snai1 regulates cell lineage allocation and stem cell maintenance in the mouse intestinal epithelium. EMBO J 2015; 34:1319-35; PMID: 25759216; http://dx.doi.org/10.15252/embj.201490881
- Goldman O, Valdes VJ, Ezhkova E, Gouon-Evans V. The mesenchymal transcription factor SNAI-1 instructs human liver specification. Stem Cell Res 2016; 17:62-8; PMID: 27240252; http://dx.doi.org/10.1016/j.scr.2016.05.007
- Javaid S, Zhang J, Anderssen E, Black JC, Wittner BS, Tajima K, Ting DT, Smolen GA, Zubrowski M, Desai R, et al. Dynamic chromatin modification sustains epithelial-mesenchymal transition following inducible expression of Snail-1. Cell Rep 2013; 5:1679-89; PMID: 24360956; http://dx.doi.org/10.1016/j.celrep.2013.11.034
- Loubat-Casanovas J, Pena R, Gonzalez N, Alba-Castellon L, Rosell S, Franci C, Navarro P, Garcia de Herreros A. Snail1 is required for the maintenance of the pancreatic acinar phenotype. Oncotarget 2016; 7:4468-82; PMID: 26735179
- Tang Y, Feinberg T, Keller ET, Li XY, Weiss SJ. Snail/Slug binding interactions with YAP/TAZ control skeletal stem cell self-renewal and differentiation. Nat Cell Biol 2016; 18:917-29; PMID: 27479603; http://dx.doi.org/10.1038/ncb3394
- Rowe RG, Li XY, Hu Y, Saunders TL, Virtanen I, Garcia de Herreros A, Becker KF, Ingvarsen S, Engelholm LH, Bommer GT, et al. Mesenchymal cells reactivate Snail1 expression to drive three-dimensional invasion programs. J Cell Biol 2009; 184:399-408; PMID: 19188491; http://dx.doi.org/10.1083/jcb.200810113
- Batlle R, Alba-Castellon L, Loubat-Casanovas J, Armenteros E, Franci C, Stanisavljevic J, Banderas R, Martin-Caballero J, Bonilla F, Baulida J, et al. Snail1 controls TGF-beta responsiveness and differentiation of mesenchymal stem cells. Oncogene 2013; 32:3381-9; PMID: 22869142; http://dx.doi.org/10.1038/onc.2012.342
- de Frutos CA, Dacquin R, Vega S, Jurdic P, Machuca-Gayet I, Nieto MA. Snail1 controls bone mass by regulating Runx2 and VDR expression during osteoblast differentiation. EMBO J 2009; 28:686-96; PMID: 19197242; http://dx.doi.org/10.1038/emboj.2009.23
- Park SJ, Jung SH, Jogeswar G, Ryoo HM, Yook JI, Choi HS, Rhee Y, Kim CH, Lim SK. The transcription factor snail regulates osteogenic differentiation by repressing Runx2 expression. Bone 2010; 46:1498-507; PMID: 20215006; http://dx.doi.org/10.1016/j.bone.2010.02.027
- de Frutos CA, Vega S, Manzanares M, Flores JM, Huertas H, Martinez-Frias ML, Nieto MA. Snail1 is a transcriptional effector of FGFR3 signaling during chondrogenesis and achondroplasias. Dev Cell 2007; 13:872-83; PMID: 18061568; http://dx.doi.org/10.1016/j.devcel.2007.09.016
- Lisignoli G, Manferdini C, Lambertini E, Zini N, Angelozzi M, Gabusi E, Gambari L, Penolazzi L, Lolli A, Facchini A, et al. Chondrogenic potential of Slug-depleted human mesenchymal stem cells. Tissue Eng Part A 2014; 20:2795-805; PMID: 24712489; http://dx.doi.org/10.1089/ten.tea.2013.0343
- Chen Y, Gridley T. Compensatory regulation of the Snai1 and Snai2 genes during chondrogenesis. J Bone Miner Res 2013; 28:1412-21; PMID: 23322385; http://dx.doi.org/10.1002/jbmr.1871
- Chen Y, Gridley T. The SNAI1 and SNAI2 proteins occupy their own and each other's promoter during chondrogenesis. Biochem Biophys Res Commun 2013; 435:356-60; PMID: 23665016; http://dx.doi.org/10.1016/j.bbrc.2013.04.086
- Murray SA, Oram KF, Gridley T. Multiple functions of Snail family genes during palate development in mice. Development 2007; 134:1789-97; PMID: 17376812; http://dx.doi.org/10.1242/dev.02837
- Wu ZQ, Rowe RG, Lim KC, Lin Y, Willis A, Tang Y, Li XY, Nor JE, Maillard I, Weiss SJ. A Snail1/Notch1 signalling axis controls embryonic vascular development. Nat Commun 2014; 5:3998; PMID: 24894949
- Zander MA, Cancino GI, Gridley T, Kaplan DR, Miller FD. The Snail transcription factor regulates the numbers of neural precursor cells and newborn neurons throughout mammalian life. PLoS One 2014; 9:e104767; PMID: 25136812; http://dx.doi.org/10.1371/journal.pone.0104767
- Perez-Mancera PA, Bermejo-Rodriguez C, Gonzalez-Herrero I, Herranz M, Flores T, Jimenez R, Sanchez-Garcia I. Adipose tissue mass is modulated by SLUG (SNAI2). Hum Mol Genet 2007; 16:2972-86; PMID: 17905753; http://dx.doi.org/10.1093/hmg/ddm278
- Sun C, Jiang L, Liu Y, Shen H, Weiss SJ, Zhou Y, Rui L. Adipose Snail1 regulates lipolysis and lipid partitioning by suppressing adipose triacylglycerol lipase expression. Cell Rep 2016; 17:2015-27; PMID: 27851965; http://dx.doi.org/10.1016/j.celrep.2016.10.070
- Gingold JA, Fidalgo M, Guallar D, Lau Z, Sun Z, Zhou H, Faiola F, Huang X, Lee DF, Waghray A, et al. A genome-wide RNAi screen identifies opposing functions of Snai1 and Snai2 on the Nanog dependency in reprogramming. Mol Cell 2014; 56:140-52; PMID: 25240402; http://dx.doi.org/10.1016/j.molcel.2014.08.014
- Piccolo S, Dupont S, Cordenonsi M. The biology of YAP/TAZ: Hippo signaling and beyond. Physiol Rev 2014; 94:1287-312; PMID: 25287865; http://dx.doi.org/10.1152/physrev.00005.2014
- Varelas X. The Hippo pathway effectors TAZ and YAP in development, homeostasis and disease. Development 2014; 141:1614-26; PMID: 24715453; http://dx.doi.org/10.1242/dev.102376
- Mo JS, Park HW, Guan KL. The Hippo signaling pathway in stem cell biology and cancer. EMBO Rep 2014; 15:642-56; PMID: 24825474
- Pan D. The Hippo signaling pathway in development and cancer. Dev Cell 2010; 19:491-505; PMID: 20951342; http://dx.doi.org/10.1016/j.devcel.2010.09.011
- Yu FX, Zhao B, Guan KL. Hippo pathway in organ size control, tissue homeostasis, and cancer. Cell 2015; 163:811-28; PMID: 26544935; http://dx.doi.org/10.1016/j.cell.2015.10.044
- Zanconato F, Cordenonsi M, Piccolo S. YAP/TAZ at the roots of cancer. Cancer Cell 2016; 29:783-803; PMID: 27300434; http://dx.doi.org/10.1016/j.ccell.2016.05.005
- Panciera T, Azzolin L, Fujimura A, Di Biagio D, Frasson C, Bresolin S, Soligo S, Basso G, Bicciato S, Rosato A, et al. Induction of expandable tissue-specific stem/progenitor cells through transient expression of YAP/TAZ. Cell Stem Cell 2016; 19(6):725-737; PMID: 27641305
- Dupont S, Morsut L, Aragona M, Enzo E, Giulitti S, Cordenonsi M, Zanconato F, Le Digabel J, Forcato M, Bicciato S, et al. Role of YAP/TAZ in mechanotransduction. Nature 2011; 474:179-83; PMID: 21654799; http://dx.doi.org/10.1038/nature10137
- Tang Y, Rowe RG, Botvinick EL, Kurup A, Putnam AJ, Seiki M, Weaver VM, Keller ET, Goldstein S, Dai J, et al. MT1-MMP-dependent control of skeletal stem cell commitment via a beta1-integrin/YAP/TAZ signaling axis. Dev Cell 2013; 25:402-16; PMID: 23685250; http://dx.doi.org/10.1016/j.devcel.2013.04.011
- Xin M, Kim Y, Sutherland LB, Murakami M, Qi X, McAnally J, Porrello ER, Mahmoud AI, Tan W, Shelton JM, et al. Hippo pathway effector Yap promotes cardiac regeneration. Proc Natl Acad Sci U S A 2013; 110:13839-44; PMID: 23918388; http://dx.doi.org/10.1073/pnas.1313192110
- Finch-Edmondson ML, Strauss RP, Passman AM, Sudol M, Yeoh GC, Callus BA. TAZ protein accumulation is negatively regulated by YAP abundance in mammalian cells. J Biol Chem 2015; 290:27928-38; PMID: 26432639
- Reginensi A, Hoshi M, Boualia SK, Bouchard M, Jain S, McNeill H. YAP and TAZ are required for Ret-dependent urinary tract morphogenesis. Development 2015; 142:2696-703; PMID: 26243870; http://dx.doi.org/10.1242/dev.122044
- Miesfeld JB, Gestri G, Clark BS, Flinn MA, Poole RJ, Bader JR, Besharse JC, Wilson SW, Link BA. YAP and TAZ regulate retinal pigment epithelial cell fate. Development 2015; 142:3021-32; PMID: 26209646; http://dx.doi.org/10.1242/dev.119008
- Elbediwy A, Vincent-Mistiaen ZI, Spencer-Dene B, Stone RK, Boeing S, Wculek SK, Cordero J, Tan EH, Ridgway R, Brunton VG, et al. Integrin signalling regulates YAP and TAZ to control skin homeostasis. Development 2016; 143:1674-87; PMID: 26989177; http://dx.doi.org/10.1242/dev.133728
- Poitelon Y, Lopez-Anido C, Catignas K, Berti C, Palmisano M, Williamson C, Ameroso D, Abiko K, Hwang Y, Gregorieff A, et al. YAP and TAZ control peripheral myelination and the expression of laminin receptors in Schwann cells. Nat Neurosci 2016; 19:879-87; PMID: 27273766; http://dx.doi.org/10.1038/nn.4316
- Wang J, Xiao Y, Hsu CW, Martinez-Traverso IM, Zhang M, Bai Y, Ishii M, Maxson RE, Olson EN, Dickinson ME, et al. YAP and TAZ play a crucial role in neural crest-derived craniofacial development. Development 2016; 143:504-15; PMID: 26718006; http://dx.doi.org/10.1242/dev.126920
- Karsenty G, Kronenberg HM, Settembre C. Genetic control of bone formation. Annu Rev Cell Dev Biol 2009; 25:629-48; PMID: 19575648; http://dx.doi.org/10.1146/annurev.cellbio.042308.113308
- Almalki SG, Agrawal DK. Key transcription factors in the differentiation of mesenchymal stem cells. Differentiation 2016; 92:41-51; PMID: 27012163; http://dx.doi.org/10.1016/j.diff.2016.02.005
- Meyer MB, Benkusky NA, Sen B, Rubin J, Pike JW. Epigenetic plasticity drives adipogenic and osteogenic differentiation of marrow-derived mesenchymal stem cells. J Biol Chem 2016; 291:17829-47; PMID: 27402842; http://dx.doi.org/10.1074/jbc.M116.736538
- Tsutsumi R, Masoudi M, Takahashi A, Fujii Y, Hayashi T, Kikuchi I, Satou Y, Taira M, Hatakeyama M. YAP and TAZ, Hippo signaling targets, act as a rheostat for nuclear SHP2 function. Dev Cell 2013; 26:658-65; PMID: 24035415; http://dx.doi.org/10.1016/j.devcel.2013.08.013
- Rosenbluh J, Nijhawan D, Cox AG, Li X, Neal JT, Schafer EJ, Zack TI, Wang X, Tsherniak A, Schinzel AC, et al. beta-Catenin-driven cancers require a YAP1 transcriptional complex for survival and tumorigenesis. Cell 2012; 151:1457-73; PMID: 23245941; http://dx.doi.org/10.1016/j.cell.2012.11.026
- Wang W, Li X, Huang J, Feng L, Dolinta KG, Chen J. Defining the protein-protein interaction network of the human hippo pathway. Mol Cell Proteomics 2014; 13:119-31; PMID: 24126142; http://dx.doi.org/10.1074/mcp.M113.030049
- Pioli PD, Chen X, Weis JJ, Weis JH. Fatal autoimmunity results from the conditional deletion of Snai2 and Snai3. Cell Immunol 2015; 295:1-18; PMID: 25732600; http://dx.doi.org/10.1016/j.cellimm.2015.02.009
- Hong JH, Hwang ES, McManus MT, Amsterdam A, Tian Y, Kalmukova R, Mueller E, Benjamin T, Spiegelman BM, Sharp PA, et al. TAZ, a transcriptional modulator of mesenchymal stem cell differentiation. Science 2005; 309:1074-8; PMID: 16099986; http://dx.doi.org/10.1126/science.1110955
- Zanconato F, Forcato M, Battilana G, Azzolin L, Quaranta E, Bodega B, Rosato A, Bicciato S, Cordenonsi M, Piccolo S. Genome-wide association between YAP/TAZ/TEAD and AP-1 at enhancers drives oncogenic growth. Nat Cell Biol 2015; 17:1218-27; PMID: 26258633; http://dx.doi.org/10.1038/ncb3216
- Stein C, Bardet AF, Roma G, Bergling S, Clay I, Ruchti A, Agarinis C, Schmelzle T, Bouwmeester T, Schubeler D, et al. YAP1 exerts its transcriptional control via TEAD-mediated activation of enhancers. PLoS Genet 2015; 11:e1005465; PMID: 26295846; http://dx.doi.org/10.1371/journal.pgen.1005465
- Galli GG, Carrara M, Yuan WC, Valdes-Quezada C, Gurung B, Pepe-Mooney B, Zhang T, Geeven G, Gray NS, de Laat W, et al. YAP drives growth by controlling transcriptional pause release from dynamic enhancers. Mol Cell 2015; 60:328-37; PMID: 26439301; http://dx.doi.org/10.1016/j.molcel.2015.09.001
- Liu X, Li H, Rajurkar M, Li Q, Cotton JL, Ou J, Zhu LJ, Goel HL, Mercurio AM, Park JS, et al. TEAD and AP1 coordinate transcription and motility. Cell Rep 2016; 14:1169-80; PMID: 26832411; http://dx.doi.org/10.1016/j.celrep.2015.12.104
- Chan CK, Seo EY, Chen JY, Lo D, McArdle A, Sinha R, Tevlin R, Seita J, Vincent-Tompkins J, Wearda T, et al. Identification and specification of the mouse skeletal stem cell. Cell 2015; 160:285-98; PMID: 25594184; http://dx.doi.org/10.1016/j.cell.2014.12.002
- Worthley DL, Churchill M, Compton JT, Tailor Y, Rao M, Si Y, Levin D, Schwartz MG, Uygur A, Hayakawa Y, et al. Gremlin 1 identifies a skeletal stem cell with bone, cartilage, and reticular stromal potential. Cell 2015; 160:269-84; PMID: 25594183; http://dx.doi.org/10.1016/j.cell.2014.11.042
- Yue R, Zhou BO, Shimada IS, Zhao Z, Morrison SJ. Leptin receptor promotes adipogenesis and reduces osteogenesis by regulating mesenchymal stromal cells in adult bone marrow. Cell Stem Cell 2016; 18:782-96; PMID: 27053299; http://dx.doi.org/10.1016/j.stem.2016.02.015
- Duscher D, Rennert RC, Januszyk M, Anghel E, Maan ZN, Whittam AJ, Perez MG, Kosaraju R, Hu MS, Walmsley GG, et al. Aging disrupts cell subpopulation dynamics and diminishes the function of mesenchymal stem cells. Sci Rep 2014; 4:7144; PMID: 25413454; http://dx.doi.org/10.1038/srep07144
- Deng Y, Wu A, Li P, Li G, Qin L, Song H, Mak KK. YAP1 regulates multiple steps of chondrocyte differentiation during skeletal development and bone repair. Cell Rep 2016; 14:2224-37; PMID: 26923596; http://dx.doi.org/10.1016/j.celrep.2016.02.021
- Crisan M, Yap S, Casteilla L, Chen CW, Corselli M, Park TS, Andriolo G, Sun B, Zheng B, Zhang L, et al. A perivascular origin for mesenchymal stem cells in multiple human organs. Cell Stem Cell 2008; 3:301-13; PMID: 18786417; http://dx.doi.org/10.1016/j.stem.2008.07.003
- Karnoub AE, Dash AB, Vo AP, Sullivan A, Brooks MW, Bell GW, Richardson AL, Polyak K, Tubo R, Weinberg RA. Mesenchymal stem cells within tumour stroma promote breast cancer metastasis. Nature 2007; 449:557-63; PMID: 17914389; http://dx.doi.org/10.1038/nature06188
- Kramann R, Schneider RK, DiRocco DP, Machado F, Fleig S, Bondzie PA, Henderson JM, Ebert BL, Humphreys BD. Perivascular Gli1+ progenitors are key contributors to injury-induced organ fibrosis. Cell Stem Cell 2015; 16:51-66; PMID: 25465115; http://dx.doi.org/10.1016/j.stem.2014.11.004
- Li HJ, Reinhardt F, Herschman HR, Weinberg RA. Cancer-stimulated mesenchymal stem cells create a carcinoma stem cell niche via prostaglandin E2 signaling. Cancer Discov 2012; 2:840-55; PMID: 22763855; http://dx.doi.org/10.1158/2159-8290.CD-12-0101
- Quante M, Tu SP, Tomita H, Gonda T, Wang SS, Takashi S, Baik GH, Shibata W, Diprete B, Betz KS, et al. Bone marrow-derived myofibroblasts contribute to the mesenchymal stem cell niche and promote tumor growth. Cancer Cell 2011; 19:257-72; PMID: 21316604; http://dx.doi.org/10.1016/j.ccr.2011.01.020
- Sacchetti B, Funari A, Remoli C, Giannicola G, Kogler G, Liedtke S, Cossu G, Serafini M, Sampaolesi M, Tagliafico E, et al. No identical “mesenchymal stem cells” at different times and sites: human committed progenitors of distinct origin and differentiation potential are incorporated as adventitial cells in microvessels. Stem Cell Reports 2016; 6:897-913; PMID: 27304917; http://dx.doi.org/10.1016/j.stemcr.2016.05.011
- Toma JG, Akhavan M, Fernandes KJ, Barnabe-Heider F, Sadikot A, Kaplan DR, Miller FD. Isolation of multipotent adult stem cells from the dermis of mammalian skin. Nat Cell Biol 2001; 3:778-84; PMID: 11533656; http://dx.doi.org/10.1038/ncb0901-778
- Lehmann W, Mossmann D, Kleemann J, Mock K, Meisinger C, Brummer T, Herr R, Brabletz S, Stemmler MP, Brabletz T. ZEB1 turns into a transcriptional activator by interacting with YAP1 in aggressive cancer types. Nat Commun 2016; 7:10498; PMID: 26876920; http://dx.doi.org/10.1038/ncomms10498
- Oram KF, Carver EA, Gridley T. Slug expression during organogenesis in mice. Anat Rec A Discov Mol Cell Evol Biol 2003; 271:189-91; PMID: 12552634; http://dx.doi.org/10.1002/ar.a.10027