ABSTRACT
The proliferative ability of oligodendrocyte progenitor cells (OPCs) varied markedly under different culture conditions. Astrocytes (ASTs) have been verified to play a major role in regulating the proliferation of OPCs through direct contact. However, the mechanisms have not been fully clarified. To investigate the effect and mechanism under AST and OPC co-culture conditions, we analyzed all connexins comprehensively in OPCs under OPC mono-culture, AST-secreted cell factor co-culture and AST-OPC direct-contact co-culture, and found that significantly differentially expressed Cx47 was the most significant. To assess whether Cx47 plays a role in proliferation, Cx47 siRNA were conducted. The result indicates that the cell cycle of OPCs was changed, and the cell proliferation was markedly inhibited. Kyoto Encyclopedia of Genes and Genomes (KEGG) predictive analysis suggested that Cx47 regulate cell cycle and proliferation by Ca2+ activation of ERK1/2. To verify the prediction, flow cytometry, confocal microscopy, 5-ethynyl-2′-deoxyuridine (EdU), polymerase chain reaction (RT-PCR) and western blot were used. The results show that interference of Cx47 led to decreased Ca2+ concentrations, lower p-ERK 1/2 levels, reduced transcription factor inhibitor of DNA binding 4 (Id4) expression, arrested cell cycle and reduced OPCs proliferative ability. Additionally, blocking ERK1/2 signaling caused decreased Id4 expression, arrested cell cycle in G1 phase, and reduced OPCs proliferative ability. In conclusion, ASTs can cause Ca2+ signaling activation, ERK1/2 phosphorylation, and Id4 expression stimulation in OPCs, inducing proliferation of these cells, mainly through Cx47.
Introduction
Oligodendrocytes (OLs), the only myelin-forming cells in the central nervous system, play an extremely important role in maintaining the structural integrity of myelin and facilitating the transmission of information. Many degenerative diseases of the central nervous system are associated with pathological demyelination and poor OLs development. Demyelination is induced by the death and breakdown of OLs and is the main feature of neurodegenerative diseases.Citation1 As discovered by Lopez et al., loss of OLs is a significant symptom of multiple sclerosis.Citation2 Although neural stem cells can form OLs,Citation3,4 their ability to do so is limited. However, oligodendrocyte progenitor cells (OPCs) are capable of directional differentiation into mature OLs; furthermore, OPCs demonstrate a high proliferative capacity and directional migration.Citation5 Therefore, the key to repairing myelin is to ensure massive proliferation of OPCs and their differentiation toward OLs under specific conditions.
Astrocytes (ASTs) are the most abundant and major macroglia in the brain and, importantly, are a component of the OPCs extracellular environment.Citation6 The proliferation and differentiation of OLs can be affected by multiple factors, including AST-secreted growth factors,Citation7,8 the cellular biologic clock, exogenous hydrophobic compounds, and OLs density-dependent negative feedback regulation.Citation9 Recently, ASTs have been shown to play a major role in regulating the proliferation and differentiation of OPCs through secretion or direct contact. However, the exact regulatory mechanism and many other issues remain unclear. Thus, the purpose of the present study was to investigate the effects and potential mechanisms of ASTs on the growth and differentiation of OPCs.
Results
Astrocytes change the cell cycle and promote the proliferation of oligodendrocyte progenitor cells through direct contact co-culture
Purified OPCs and ASTs were obtained by conditioned culture. The purity of both the OPCs and ASTs populations was higher than 95%, as determined by labeling with the specific antigens PDGFRα and GFAP, respectively ().
Figure 1. A: Phenotypic properties of cells in isolated culture. OPCs and ASTs were selected using PDGFRα (a) and GFAP (b) antibodies, respectively. B: Proliferation of OPCs under different culture conditions were observed by inverted microscope (200×). OPCs in the AST-OPC demonstrated a higher proliferative ability than those in the CO-OPC and OPC groups. C: The AST-OPC group showed a higher number of newly born OPCs, as detected by the EdU assay, than did the CO-OPC and OPC groups. D: The AST-OPC group was more likely to enter into S-phase compared with the CO-OPC and OPC groups.
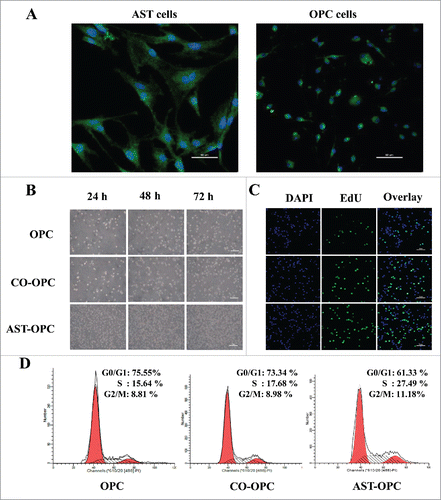
The proliferative ability of OPCs varied markedly under different culture conditions. The AST-OPC group demonstrated a substantially higher proliferative ability than the CO-OPC and OPC groups (). Additionally, a higher percentage of newly born OPCs was found for the AST-OPC group (41.17 ± 3.30) compared with the CO-OPC (29.59 ± 3.88) and OPC (16.07 ± 4.10) groups, as revealed by EdU assay in 10 fields selected at random (p < 0.001, ). Cells in the AST-OPC group were more likely to enter S-phase of the cell cycle; as revealed by flow cytometry analysis, a higher percentage of S-phase cells in the AST-OPC group (25 ± 3.01) was observed compared with the CO-OPC (18.94 ± 1.56) and OPC (15.74 ± 0.73) groups (p < 0.05, ).
Cx47 expression increases in OPCs co-cultured with ASTs and mediates proliferation of OPCs
The development of coupling among mammalian glial cells, especially oligodendrocytes, has not been carefully studied and is of interest because cell-cell coupling may influence the proliferation and differentiation of these cells.Citation12-14 Increasing evidence indicates that Cx-mediated communication among ASTs, among OLs or between ASTs and OLs may be important for the development of OLs, including proliferation, differentiation and myelination.Citation15 After transcriptome sequencing (RNA-Seq), significantly differentially expressed genes were defined as Log2Ratio > 1, false discovery rate (FDR) < 0.001, and p < 0.01. The results showed 9 unique connexin proteins expressed in OPCs. Significantly differentially expressed genes have 3. Cx47 was the most significant difference gene in direct contact with ASTs (). Cx47 expression was upregulated according to a hierarchically clustered heatmap ().
Figure 2. OPCs were harvested from each group after 48h, Cx47 may increase by co-culture with AST.A: Expression of 9 different connexin proteins under different culture conditions. Significantly differentially expressed genes have 3. Cx47 was the most significant. B: Differential expression of the Cx47 gene in a hierarchically clustered heatmap (red is upregulated; green is downregulated). C: RT-PCR verification of 10 differentially expressed genes selected at random; the results are consistent with RNA-Seq data.
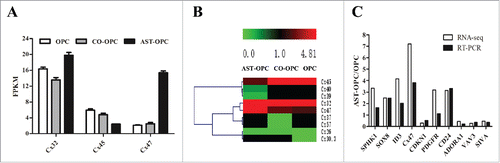
The accuracy of sequencing was verified by RT-PCR using 10 genes differentially expressed between the OPC and AST-OPC groups selected at random (). We compared changes between the results of RT-PCR and expression and sequencing analyses. The 2 sets of data were consistent, proving that the sequencing results were accurate and reliable.
Western blot analysis revealed that Cx47 was significantly elevated in the AST-OPC group (2.54 ± 0.06) compared with the OPC (1 ± 0.06) and CO-OPC (1.97 ± 0.07) groups (p < 0.001, ). Therefore, we performed a screen to identify siRNA effective at silencing Cx47 by RT-PCR and Western blot ().
Figure 3. OPCs were harvested from each group after 48 h. A : Cx47 expression under different culture conditions tested by western blot, **p < 0.001 vs OPC, ##p < 0.001 vs CO-OPC. B: Cx47 expression under different culture conditions detected by RT-PCR. *p < 0.01, **p < 0.001 vs AST-OPC. ##p < 0.001 vs NC siRNA. C: Western blot screening of Cx47 siRNA, **p < 0.001; D: Western blot assay of Cx47 expression after Cx47 siRNA treatment, **p < 0.001 vs AST-OPC, ##p < 0.001 vs NC siRNA.
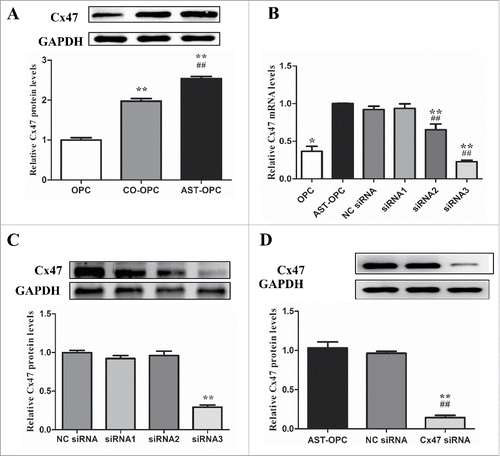
Increased Cx47 expression mediates Ca2+ elevation and promotes ERK1/2 phosphorylation in OPCs
In our transcriptome sequencing database (date not show), KEGG predictive analysis suggested that Cx47 regulate cell cycle and proliferation by Ca2+ activation of ERK1/2(http://www.kegg.jp/kegg-bin/show_pathway?ko04540). An intracellular Ca2+ assay using flow cytometry revealed the highest calcium signal in the AST-OPC group, and the intracellular calcium signal was significantly attenuated after Cx47 silencing (617 ± 54.24) compared with the AST-OPC (1044 ± 282.20) and NC siRNA (1008 ± 174.35) groups (p < 0.05, ). Laser confocal microscopy analysis also showed significantly reduced fluorescence intensity of the calcium signal after Cx47 siRNA treatment (p < 0.001 ).
Figure 4. Proliferation of OPCs by AST induced calcium signaling and phosphorylated ERK1/2. A: OPCs were treated with Cx47 siRNA for 48 h. Decreased calcium signaling was detected by flow cytometry. B: OPCs were treated with Cx47 siRNA for 48 h. Attenuated calcium signaling was revealed by confocal microscopy. C: p-ERK levels decreased after Cx47 siRNA treatment and U0126-mediated ERK1/2 inhibition (*p < 0.01, **p < 0.001 vs AST-OPC, ##p < 0.001 vs NC siRNA).
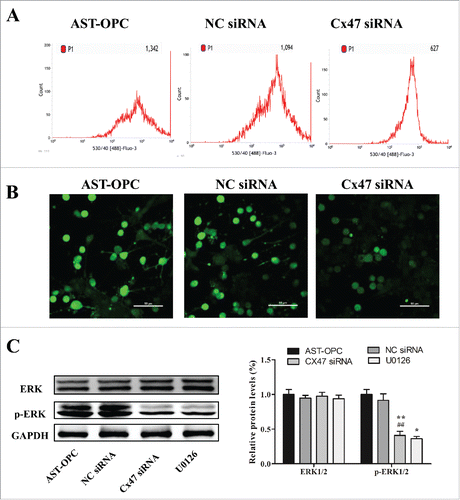
Western blot analysis showed that ERK1/2 expression did not significantly change after Cx47 silencing. However, the p-ERK1/2 level was significantly decreased in Cx47 siRNA group (0.41 ± 0.06), and this difference reached statistical significance compared with the AST-OPC (1 ± 0.07) and NC siRNA (0.91 ± 0.09) groups (p < 0.001, ).
Together, these results suggest that AST-OPC contact co-culture can increase intracellular Ca2+ concentrations and facilitate ERK1/2 phosphorylation in OPCs via Cx47. Silencing of Cx47 reduces Ca2+ influx, resulting in a lower intracellular Ca2+ concentration and decreased ERK1/2 phosphorylation.
Cx47 promotes OPCs proliferation by increasing ERK1/2 phosphorylation
ASTs might mediate OPCs proliferation through Cx47. Our EdU assay results showed that cell proliferation was markedly inhibited after Cx47 siRNA treatment. The number of newly born OPCs in the Cx47 siRNA group declined to (14.11 ± 2.67), and the difference was significant compared with the AST-OPC (53.37 ± 3.27) and NC siRNA (51.15 ± 5.07) groups (p < 0.001, ). These results suggest that ASTs can mediate the proliferative activity and markedly increase the number of OPCs through Cx47.
Figure 5. EdU assay of the percentage of newly born OPCs following Cx47 siRNA treatment and U0126-mediated inhibition of ERK1/2. The percentage was significantly decreased compared with the AST-OPC direct-contact and NC siRNA groups.
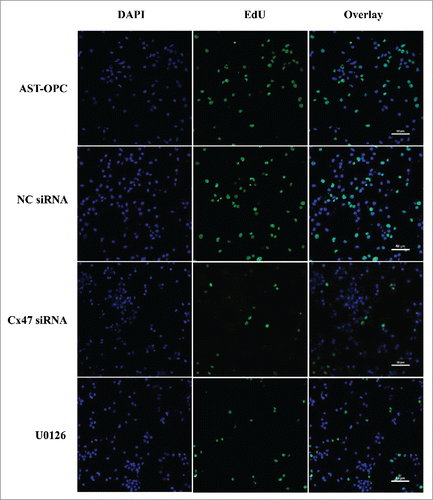
Blocking ERK1/2 phosphorylation with the inhibitor U0126 may prevent Cx47-mediated cell proliferation. Following a 48h treatment with U0126 and inhibition of ERK1/2 phosphorylation, EdU assay results showed a significant decrease in Cx47-mediated cell proliferation from (53.37 ± 3.27) to (13.74 ± 2.15) (p < 0.001, ). These results suggest that the ability of ASTs to promote the proliferation of OPCs is closely associated with Cx47-mediated ERK1/2 phosphorylation.
Cx47 upregulates expression of transcription factor Id4 and promotes proliferation of OPCs through ERK1/2 phosphorylation
Analysis of the cell cycle by flow cytometry revealed that Cx47 siRNA treatment altered the percentage of OPCs in different phases. Specifically, the percentage of S-phase cells decreased to (11.32 ± 0.70), and a significant difference was detected compared with the AST-OPC (28.02 ± 2.47) and NC siRNA (29.17 ± 3.16) groups (p < 0.001, ). In contrast, the percentage of G1-phase cells increased to (72.53 ± 1.74), with a significant difference compared with the AST-OPC (61.22 ± 1.23) and NC siRNA (61.95 ± 4.83) groups (p < 0.01, ).
Figure 6. A: After Cx47 siRNA treatment, the percentage of S-phase cells decreased, and a significant difference was detected compared with the AST-OPC and NC siRNA groups. After blocking ERK1/2 phosphorylation, the percentage of S-phase cells decreased compared with the AST-OPC. B: Cx47 siRNA treatment and U0126-mediated inhibition of ERK1/2 resulted in decreased expression of the downstream transcription factor Id4 (& p < 0.05, **p < 0.001 vs AST-OPC, ##p < 0.001 vs NC siRNA).
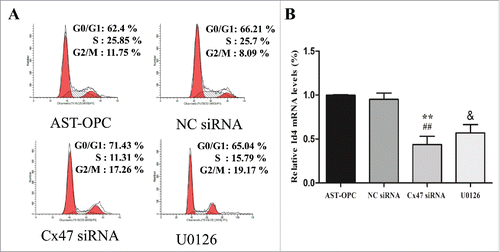
RT-PCR results showed a decrease in Id4 expression after Cx47 siRNA treatment. A significant difference was detected compared with the AST-OPC and NC siRNA groups (p < 0. 001, ).
Blocking ERK1/2 phosphorylation might suppress the ability of Cx47 to regulate cell cycle and promote cell proliferation. The results of Western blotting showed that U0126 caused a marked decrease in ERK1/2 phosphorylation (). Flow cytometry analysis of the cell cycle indicated that blocking ERK1/2 phosphorylation with U0126 suppressed the ability of Cx47 to increase the percentage of S-phase cells in OPCs, but an increased percentage of G1-phase cells. Both the decrease in S-phase cells and the increase in G1-phase cells reached statistical significance compared with the AST-OPC group (p < 0.05, ). Indeed, RT-PCR showed that U0126 treatment resulted in reduced levels of Id4 expression (p < 0.05, ).
The above results show that blocking ERK1/2 phosphorylation can reverse the ability of Cx47 to regulate cell cycle and promote cell proliferation. This leads to the hypothesis that ASTs can promote ERK1/2 phosphorylation and regulate the cell cycle through Cx47, thereby facilitating the proliferation of OPCs.
Discussion
Neurodegenerative disease is often progressive and has high morbidity, high mortality, and poor treatment response. Many degenerative diseases of the central nervous system are associated with pathological demyelination and inadequate development of OLs. Thus, myelin repair and regeneration are essential for treating demyelination. As the only myelin-forming cells in the central nervous system, OLs play an extremely important role in maintaining the structural integrity of myelin and in facilitating the transfer of information. OPCs, i.e., immature OLs, are pluripotent stem cells that function in in vivo proliferation, migration, and terminal differentiation into mature OLs as well as in myelin formation. Therefore, promoting OPCs proliferation has become a target of experimental research and disease treatment.
Previous studies have shown that polypeptide factors, including platelet-derived growth factor (PDGF-α), basic fibroblast growth factor (bFGF), and the nerve growth factor neurotrophin-3 (NT-3), are closely associated with OPC proliferation.Citation16-18 In the current study, we found that both CO-OPC and AST-OPC groups markedly promoted the proliferation of OPCs. With increasing culture time, a significant increase in the number of OPCs was observed compared with the OPC group, and this effect was most significant for the AST-OPC group. Cell cycle analysis of exponential-phase OPCs by flow cytometry showed the highest percentage of S-phase cells for the AST-OPC group, and significant differences were detected compared with the 2 other groups (OPC and CO-OPC groups). Moreover, the number of newly born OPCs detected by the EdU assay was higher for the AST-OPC group than for the OPC and CO-OPC groups. These results suggest that ASTs regulate the proliferation of OPCs through connexin.
Connexin is the basic functional protein constituting GJ channels. Six connexin proteins are connected by non-covalent bonds to form a hemichannel, and hemichannels on the membrane surface of 2 adjacent cells form a complete GJ intercellular channel.Citation19 GJs allow small molecules with a molecular weight of less than 1.2 kD and a diameter of less than 1.5 nm to pass through, including ions, metabolic molecules, and second messengers (e.g., Ca2+, IP3, cAMP, and ATP).Citation20 In the central nervous system, ASTs (the most abundant glial cells) and OLs form heterotypic GJs through connexin, thereby establishing gap-junctional intercellular communication. Connexin and intercellular communication play essential roles in regulating embryonic development, maintaining tissue/organ homeostasis, cell metabolism, and cell morphology and controlling growth.Citation21-23 Moreover, GJs have an important function in the generation and maintenance of myelin.Citation24 In this study, we analyzed changes in expression of all 9 connexin proteins found in OPCs under different culture conditions using next-generation transcriptome sequencing. Our results showed that OPCs mainly expressed Cx32, Cx47, and Cx45, unlike mature OLs, which mainly expressed Cx47, Cx32, and Cx29. Cx47 demonstrated the most significant change: its expression increased 7.2-fold under OPC and AST-OPC co-culture conditions. Following Cx47 siRNA interference, the ratio of OPCs was reduced in S-phase and arrested in G1-phase, and the proliferative ability of OPCs was markedly decreased. The results suggest that proliferation and metabolism-related material and information exchanges between ASTs and OPCs are primarily mediated by cell-surface Cx47.Citation25 Cx47 is mainly expressed by OLs.Citation24,26 Cx47 expression relies on Cx43 in ASTs, and heterotypic GJs formed by ASTs and OLs are the major form of (O/A) GJs.Citation27
GJs allow ASTs in different regions to form 3-dimensional channel structures with other glial cells and to propagate intracellular calcium signaling,Citation28 among other important functions. Herrero-González et al. noted that calcium signaling through GJs can cause an increase in free calcium in neighboring cells,Citation29 and a study by Parys et al. showed that calcium signaling can be transmitted from ASTs to OLs.Citation30 This is consistent with our confocal microscopy findings regarding Ca2+ content, whereby Cx47 siRNA treatment resulted in a marked reduction in Ca2+ influx. Stains et al. noted that a relationship exists between GJs and intracellular ERK1/2 activation.Citation31 Although we found no significant change in the relative expression of ERK1/2 after Cx47 siRNA treatment, p-ERK1/2 was substantially decreased. These results suggest that Cx47-mediated activation of p-ERK1/2 depends on increased Ca2+ in OPCs.
Id proteins comprise a family of helix-loop-helix (HLH) transcription factors, including Id1–Id4. Id proteins lack the basic amino acids essential for DNA binding and play a negative regulatory role in bHLH transcriptional activity, thereby inhibiting cell differentiation and promoting cell proliferation.Citation32 Expression of Id protein is upregulated in normal cells, which promotes DNA synthesis and cell cycle progression from G1 to S phase, thus contributing to normal cell proliferation. Moreover, Id can increase proliferation and suppress differentiation in OLs.Citation33,34 In the present study, Id4 gene expression was downregulated after Cx47 siRNA treatment and ERK1/2 inhibition compared with AST-OPC and NC siRNA groups. Furthermore, the percentage of G1-phase OPCs was significantly increased, and their proliferative ability was substantially reduced. Based on the above findings, we propose that Cx47 causes the proliferation of OPCs through ERK-induced Id4.
In summary, Cx47 is the leading factor that mediates AST-induced proliferation of OPCs after in vitro co-culture of ASTs and OPCs. ASTs can significantly increase intracellular Ca2+ concentrations, activate ERK/Id4, and regulate the cell cycle and proliferative ability of OPCs through Cx47, which is a key finding for future studies of remyelination.
Materials and methods
Animals
Neonatal Sprague-Dawley rats (P0–P3) were provided by the Experimental Animal Center of Chongqing Medical University. Animal use in this study was in the accordance with the internationally accepted principles for laboratory animal use and care. The neuroblastoma cell line B104 was kindly provided by the Third Military Medical University.
Collection of B104 supernatant
B104 cells were cultured using DMEM/F12 medium (Gibco) supplemented with 12% fetal bovine serum (FBS, 10099–141, Gibco) until they reached 80–90% confluence. After washing with phosphate-buffered saline (PBS), the cells were transferred to DMEM/F12 medium with 1% N-2 supplementation (A1370701, Gibco) and cultured for another 4 d. The supernatant was then collected, centrifuged, filtered and stored at −80°C.
Primary culture of OPCs
Neonatal Sprague-Dawley rats (P0–P3) were anesthetized by being buried in ice for 5 min. Then, the rats were sprayed with alcohol and decapitated. After washing with pre-cooled PBS, the telencephalon was separated, and the meninges, cerebrum, cerebellum, hippocampus and surrounding white matter, brainstem, and olfactory bulb were removed. The remaining brain gray matter and part of the white matter were used in the experiment. The tissues were chopped and pipetted to form a cell suspension. The cell suspension was plated into a poly-D-lysine (PDL)-pre-coated Petri dish, and the medium containing 12% FBS was changed every other day. When the cells reached 70–80% confluence after 3–5 days, the medium was changed to proliferation medium containing 15% B104 supernatant and 1% N-2 supplementation. The cells were cultured to 7–9 d and then digested using Ethylenediaminetetraacetic Acid (EDTA; Beyotime Biotechnology) for 10 min with appropriate pipetting. Sufficient cell number was gotten, culturing with proliferation medium for another 2–4 d. The same digestion and separation method were treated as described above. Finally, the cells were passaged and purified to obtain.
Primary culture of ASTs
Neonatal Sprague-Dawley rats (P0–P3) were treated as described in section 1.1.2. The brain gray matter and part of the white matter were chopped and then digested with trypsin. The digested solution was filtered through a 200-mesh cell strainer and centrifuged at 1,200 rpm for 5 min. The cells were resuspended in a glass Petri dish and cultured for adherent growth in an incubator for 20 min. The single cell suspension was plated onto a PDL-pre-coated Petri dish, and the medium containing 12% FBS was changed every other day.
Establishment of AST-OPC co-culture model experimental groups
Conventional OPC mono-culture group (OPC group): Purified OPCs (1–2 × 104/cm2) were evenly seeded into a 6-well plate and cultured in proliferation medium for 72 h.
Cell factor co-culture group (CO-OPC group): Purified ASTs were seeded into the upper compartment and cultured until 90% confluence was reached. Purified OPCs were then evenly seeded into a 6-well plate and cultured in proliferation medium for 72 h.
AST-OPC direct-contact co-culture group (AST-OPC group): After ASTs were seeded until 90% confluence, OPCs were then evenly seeded onto the surface of the ASTs and cultured in proliferation medium for 72 h.
Negative control Cx47 siRNA group (NC siRNA group), Cx47 siRNA group, and ERK1/2 blocker group (U0126 group): Purified ASTs were seeded into a 6-well plate and cultured until 90% confluence was reached. Purified OPCs were then evenly seeded onto the surface of the ASTs. When 50–60% confluence was reached, the transfection complex (Cx47 siRNA: CCGAGAAGACTGTCTTCTT; RiboBio, Guangzhou, China) and U0126 (10 μM, HY-12031, MedChem Express) were added for 48 h.
Immunofluorescence
Immunofluorescence was performed as described previously.Citation10 Antibodies and their sources are: GFAP (1:200,556330,BD Biosciences), PDGFαR (1:200,SC-338,Santa Cruz), Alexa Fluor-conjugated goat anti-mouse and anti-rabbit IgG (Beyotime Biotechnology).
Cell cycle analysis by flow cytometry
OPCs were harvested from each group, washed twice with PBS, and fixed in 70% cold ethanol at 4°C overnight. After centrifugation and PBS washes, propidium iodide and RNase working solutions were added to the cells for staining for 30 min. The cell cycle was analyzed by flow cytometry.
EdU assay of cell proliferation
OPCs were adjusted to a cell density of 1 × 106/ml and seeded into a 24-well plate pre-coated with PDL. Cell staining was performed using an EdU kit (RiboBio), and images were acquired using confocal microscopy.
RT-PCR validation of differentially expressed connexins
Total RNA was extracted from samples using Trizol and cDNA generated by cDNA Synthesis Kit (RR047A, Takara). The following primer sequences were used: Cx47, 5′-GAGGATGAGGACGAGGAACCA-3′ and 5′-CACCGTCTTTCCATCACCTCC −3′; and Id4, 5′-GCCCAACAAGAAAGTCAGCAA-3′ and 5′-CTGTCTCAGCAGAGCAGGGTG-3′. 2−ΔΔCt method was used to analyze real-time PCR data.
Intracellular Ca2+ assay
OPCs were treated for 48 h. Following treatment, cells were loaded with 5 µM Fluo 3-a.m. for 30 min at 37°C (Beyotime Biotechnology). After loading, the cells were washed with PBS and suspended in DMEM.
Western blotting for Cx47 and ERK1/2 protein expression
OPCs were processed in ice-cold lysis buffer followed by Western blot analysis as described previously.Citation11 Antibodies and their sources are: Cx47 (1:500,BS7447,Bioworld Technology), ERK (1 : 10000,ab76299,Abcam), p-ERK (1:1000,ab214036,Abcam), or GAPDH (1:10000, ab181602,Abcam).
Statistical analysis
All experiments were repeated at least 3 times. SPSS 17.0 statistical software was used for the statistical analysis. All values are expressed as the mean ± SD. Differences were tested using analysis of variance (ANOVA). P<0.05 was considered significant.
Disclosure of potential conflicts of interest
No potential conflicts of interest were disclosed.
1295183_Supplemental_Material.ppt
Download MS Power Point (70.5 KB)Funding
This work was financially supported by the Natural Science Foundation of China (NSFC 81671259); the Natural Science Foundation of China (NSFC 81470057); the Natural Science Foundation of China (NSFC 81501101); Research Foundation for 100 Academic and Discipline Talented Leaders of Chongqing, RP China.
References
- Pott F, Gingele S, Clarner T, Dang J, Baumgartner W, Beyer C, Kipp M. Cuprizone effect on myelination, astrogliosis and microglia attraction in the mouse basal ganglia. Brain Res 2009; 1305:137-49; PMID:19799876; http://dx.doi.org/10.1016/j.brainres.2009.09.084
- Lopez Juarez A, He D, Richard Lu Q. Oligodendrocyte progenitor programming and reprogramming: Toward myelin regeneration. Brain Res 2016; 1638(PtB):209-20; PMID:26546966; http://dx.doi.org/10.1016/j.brainres.2015.10.051
- Alvarez-Buylla A, Herrera DG, Wichterle H. The subventricular zone: source of neuronal precursors for brain repair. Prog Brain Res 2000; 127:1-11; PMID:11142024
- Dimou L, Simon C, Kirchhoff F, Takebayashi H, Götz M. Progeny of Olig2-expressing progenitors in the gray and white matter of the adult mouse cerebral cortex. J Neurosci 2008; 28:10434-42. PMID:18842903; http://dx.doi.org/10.1523/JNEUROSCI.2831-08.2008
- Baumann N, Pham-Dinh D. Biology of oligodendrocyte and myelin in the mammalian central nervous system. Physiol Rev 2001; 81:871-927; PMID:11274346
- Namihira M, Nakashima K. Mechanisms of astrocytogenesis in the mammalian brain. Curr Opin Neurobiol 2013; 23:921-7; PMID:23827784; http://dx.doi.org/10.1016/j.conb.2013.06.002
- Tsiperson V, Huang Y, Bagayogo I, Song Y, VonDran MW, DiCicco-Bloom E, Dreyfus CF. Brain-derived neurotrophic factor deficiency restricts proliferation of oligodendrocyte progenitors following cuprizone-induced demyelination. ASN Neuro 2015; 7(1); PMID:25586993; http://dx.doi.org/10.1177/1759091414566878
- Mason JL, Xuan S, Dragatsis I, Efstratiadis A, Goldman JE. Insulin-like growth factor (IGF) signaling through type 1 IGF receptor plays an important role in remyelination. J Neurosci 2003; 23:7710-8; PMID:12930811
- Brösamle C, Huber AB, Fiedler M, Skerra A, Schwab ME. Regeneration of lesioned corticospinal tract fibers in the adult rat induced by a recombinant, humanized IN-1 antibody fragment. J Neurosci 2000; 20:8061-8; PMID:11050127
- Yuan CX, Chu T, Liu L, Li HW, Wang YJ, Guo AC, Fan YP. Catalpol induces oligodendrocyte precursor cell-mediated remyelination in vitro. Am J Transl Res 2015; 7:2474-81; PMID:26807192
- Zhao M, Liu Q, Gong Y, Xu X, Zhang C, Liu X, Zhang C, Guo H, Zhang X, Gong Y, et al. GSH-dependent antioxidant defense contributes to the acclimation of colon cancer cells to acidic microenvironment. Cell Cycle 2016; 15:1125-33; PMID:26950675; http://dx.doi.org/10.1080/15384101.2016.1158374
- Von Blankenfeld G, Ransom BR, Kettenmann H. Development of cell-cell coupling among cells of the oligodendrocyte lineage. Glia 1993; 7(4):322-8; PMID:8320002; http://dx.doi.org/10.1002/glia.440070407
- Harris AL, Spray DC, Bennett MV. Control of intercellular communication by voltage dependence of gap junctional conductance. J Neurosci 1983; 3(1):79-100; PMID:6822860
- Lo Turco JJ, Kriegstein AR. Clusters of coupled neuroblasts in embryonic neocortex. Science 1991; 252(5005):563-6; PMID:1850552
- Li T, Giaume C, Xiao L. Connexins-mediated glia networking impacts myelination and remyelination in the central nervous system. Mol Neurobiol 2014; 49(3):1460-71; PMID:24395132. http://dx.doi.org/10.1007/s12035-013-8625-1
- Fu SL, Hu JG, Li Y, Wang YX, Jin JQ, Xui XM, Lu PH. A simplified method for generating oligodendrocyte progenitor cells from neural precursor cells isolated from the E16 rat spinal cord. Acta Neurobiol Exp (Wars) 2007; 67:367-77; PMID:18320715
- Kahn MA, Kumar S, Liebl D, Chang R, Parada LF, De Vellis J. Mice lacking NT-3, and its receptor TrkC, exhibit profound deficiencies in CNS glial cells. Glia 1999; 26:153-65, PMID:10384880
- Zeger M, Popken G, Zhang J, Xuan S, Lu QR, Schwab MH, Nave KA, Rowitch D, D'Ercole AJ, Ye P. Insulin-like growth factor type 1 receptor signaling in the cells of oligodendrocyte lineage is required for normal in vivo oligodendrocyte development and myelination. Glia 2007; 55:400-11; PMID:17186502; http://dx.doi.org/10.1002/glia.20469
- Hervé JC, Bourmeyster N, Sarrouilhe D, Duffy HS. Gap junctional complexes: from partners to functions. Prog Biophys Mol Biol 2007; 94:29-65; PMID:17507078; http://dx.doi.org/10.1016/j.pbiomolbio.2007.03.010
- Ponsioen B, van Zeijl L, Moolenaar WH, Jalink K. Direct measurement of cyclic AMP diffusion and signaling through connexin43 gap junctional channels. Exp Cell Res 2007; 313:415-23;PMID: 17150212; http://dx.doi.org/10.1016/j.yexcr.2006.10.029
- Yao J, Morioka T, Oite T. PDGF regulates gap junction communication and connexin43 phosphorylation by PI 3-kinase in mesangial cells. Kidney Int 2000; 57:1915-26; PMID:10792610; http://dx.doi.org/10.1046/j.1523-1755.2000.00041.x
- Chen M, Liu Y, Yi D, Wei L, Li Y, Zhang L. Tanshinone IIA promotes pulmonary artery smooth muscle cell apoptosis in vitro by inhibiting the JAK2/STAT3 signaling pathway. Cell Physiol Biochem 2014; 33:1130-8; PMID:24733040; http://dx.doi.org/10.1159/000358682
- Li H, Tang M, Liang H, Li Y, Wang J, Song Y, Zheng Y, Xi J, Zhang J, Hescheler J, et al. Coculture of embryonic ventricular myocytes and mouse embryonic stem cell enhance intercellular signaling by upregulation of connexin43. Cell Physiol Biochem 2013; 32:53-63. PMID:23867886; http://dx.doi.org/10.1159/000350124
- Menichella DM, Goodenough DA, Sirkowski E, Scherer SS, Paul DL. Connexins are critical for normal myelination in the CNS. J Neurosci 2003; 23:5963-73; PMID:12843301
- Zlomuzica A, Tress O, Binder S, Rovira C, Willecke K, Dere E. Changes in object recognition and anxiety-like behaviour in mice expressing a Cx47 mutation that causes Pelizaeus-Merzbacher-like disease. Dev Neurosci 2012; 34:277-87; PMID:22832166; http://dx.doi.org/10.1159/000339305
- Odermatt B, Wellershaus K, Wallraff A, Seifert G, Degen J, Euwens C, Fuss B, Büssow H, Schilling K, Steinhäuser C, et al. Connexin 47 (Cx47)-deficient mice with enhanced green fluorescent protein reporter gene reveal predominant oligodendrocytic expression of Cx47 and display vacuolized myelin in the CNS. J Neurosci 2003; 23:4549-59; PMID:12805295
- May D, Tress O, Seifert G, Willecke K. Connexin47 protein phosphorylation and stability in oligodendrocytes depend on expression of Connexin43 protein in astrocytes. J Neurosci 2013; 33:7985-96; PMID:23637189; http://dx.doi.org/10.1523/JNEUROSCI.5874-12.2013
- Blomstrand F, Venance L, Sirén AL, Ezan P, Hanse E, Glowinski J, Ehrenreich H, Giaume C. Endothelins regulate astrocyte gap junctions in rat hippocampal slices. Eur J Neurosci 2004; 19:1005-15. PMID:15009148; http://dx.doi.org/10.1111/j.0953-816X.2004.03197.x
- Herrero-González S, Valle-Casuso JC, Sánchez-Alvarez R, Giaume C, Medina JM, Tabernero A. Connexin43 is involved in the effect of endothelin-1 on astrocyte proliferation and glucose uptake. Glia 2009; 57:222-33; PMID:18756537; http://dx.doi.org/10.1002/glia.20748
- Parys B, Côté A, Gallo V, De Koninck P, Sík A. Intercellular calcium signaling between astrocytes and oligodendrocytes via gap junctions in culture. Neuroscience 2010; 167:1032-43; PMID:20211698; http://dx.doi.org/10.1016/j.neuroscience.2010.03.004
- Stains JP, Civitelli R. Gap junctions regulate extracellular signal-regulated kinase signaling to affect gene transcription. Mol Biol Cell 2005; 16:64-72; PMID:15525679; http://dx.doi.org/10.1091/mbc.E04-04-0339
- Norton JD. ID helix-loop-helix proteins in cell growth, differentiation and tumorigenesis. J Cell Sci 2000; 113:3897-905; PMID:11058077
- Kondo T, Raff M. The Id4 HLH protein and the timing of oligodendrocyte differentiation. EMBO J 2000; 19:1998-2007; PMID:10790366; http://dx.doi.org/10.1093/emboj/19.9.1998
- Wang S, Sdrulla A, Johnson JE, Yokota Y, Barres BA. A role for the helix-loop-helix protein Id2 in the control of oligodendrocyte development. Neuron 2001; 29:603-14; PMID:11301021; http://dx.doi.org/10.1016/S0896-6273(01)00237-9