ABSTRACT
Pancreatic cancer is a recalcitrant malignancy, partly due to desmoplastic stroma which stimulates tumor growth, invasion, and metastasis, and inhibits chemotherapeutic drug delivery. Transforming growth factor-β (TGF-β) has an important role in the formation of stromal desmoplasia. The present study describes the ability of color-coded intravital imaging to demonstrate the efficacy of a TGF-β inhibitor to target stroma in an orthotopic mouse model of pancreatic cancer. The BxPC-3 human pancreatic adenocarcinoma cell line expressing green fluorescent protein (GFP), which also has a high TGF-β expression level, was used in an orthotopic model in transgenic nude mice ubiquitously expressing red fluorescent protein (RFP). Fourteen mice were randomized into a control group (n = 7, vehicle, i.p., weekly, for 3 weeks) and a treated group (n = 7, SB431542 [TGF-β receptor type I inhibitor] 0.3 mg, i.p., weekly, for 3 weeks). Stromal cells expressing RFP and cancer cells expressing GFP were observed weekly for 3 weeks by real-time color-coded intravital imaging. The RFP fluorescence area from the stromal cells, relative to the GFP fluorescence area of the cancer cells, was significantly decreased in the TGF-β-inhibitor-treatment group compared to the control group. The present study demonstrated color-coded imaging in an orthotopic pancreatic-cancer cell-line mouse model can readily detect the selective anti-stromal-cell targeting of a TGF-β inhibitor.
Introduction
Pancreatic cancer is a recalcitrant disease with a 5-year overall survival of 6% despite multimodality treatments available.Citation1 Pancreatic cancer is characterized by desmoplastic stromaCitation2 that stores and releases growth factors such as transforming growth factor-β (TGF-β), fibroblast growth factor (FGF), and insulin-like growth factor-1 (IGF-1), which facilitate tumor growth, invasion, and metastasis.Citation3 In addition, increased interstitial fluid pressure induced by the desmoplastic stroma compresses intratumor blood vessels, resulting in inhibition of chemotherapeutic drug delivery and reduction of efficacy.Citation4 TGF-β plays a central role for establishment of the desmoplastic stroma.Citation5
Our laboratory has developed color-coded intravital and non-invasive imaging using spectrally-distinct fluorescent proteins, to distinguish cancer and stromal cells in mouse models of cancer.Citation6-9 Using color-coded imaging, we have demonstrated that stromal cells are drivers for metastasis.Citation10 We have also demonstrated using color-coded imaging that stromal cells, such as pancreatic-cancer stellate cells from the primary pancreatic tumor, accompany the cancer cells to distant metastatic sites.Citation11 We also showed by non-invasive imaging that acquired fluorescent protein-expressing stromal cells can continuously proliferate within the growing tumor.Citation12 In addition, we showed by non-invasive color-coded imaging the intimate relationship of cancer and stromal cells and how each are killed by standard chemotherapy.Citation13
In the present report, we demonstrate that color-coded imaging could detect selective stromal targeting by a TGF-β antagonist in an orthotopic mouse model of pancreatic cancer.
Results and discussion
Color-coded imaging demonstrates that a TGF-β inhibitor selectively targets stromal cells in orthotopic pancreatic tumors
An orthotopic mouse model of human pancreatic cancer with red fluorescent protein (RFP)-expressing stromal cells and green fluorescent protein (GFP)-expressing cancer cells was established (). The RFP-expressing stromal-cell area of tumors treated with the TGF-β inhibitor SB431542 gradually decreased as demonstrated by time-course color-coded intravital imaging (, ). The percentage of the RFP fluorescence area from the stromal cells relative to the total fluorescence area was significantly lower in the TGF-β inhibitor-treated group from days 7 to 21 (, ). Stromal area did not decrease from days 7 to 21 in the untreated control group (, ).
Figure 1. Orthotopic pancreatic tumor and real-time intravital color-coded imaging system. A representative dual-color orthotopic pancreatic cancer mouse model in bright field (A), GFP (B), and RFP flourescence (C). Images were obtained with the OV100 Small Animal Imaging System. The tumor (arrowheads) was exposed on the pancreas (arrows) through an incision (C). After cutting the tumor surface (D-F), mice were placed under the microscope for real- time color-coded intravital imaging with the FV-1000 confocal microscope (G, H). BF; bright field, GFP; green fluorescent protein, RFP; red fluorescent protein. Scale bars: 5 mm.
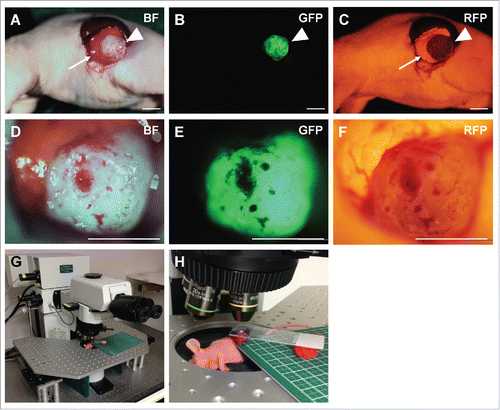
Figure 2. Color-coded real-time intravital cancer-cell/stromal-cell imaging in untreated tumors. Color-coded intravital imaging of a tumor in a control mouse (A-D). Intravital images were obtained weekly. Upper panels show GFP-expressing cancer cells, middle panels show RFP-expressing stromal cells, and lower panels show merged images with GFP and RFP. Scale bars: 1.0 mm.
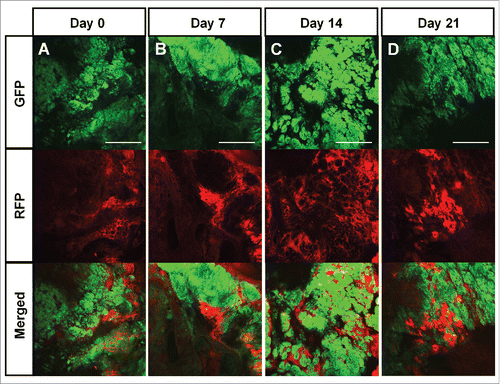
Figure 3. Color-coded real-time intravital cancer-cell/stroma-cell imaging after treatment with a TGF-β inhibitor. Color-coded tumor from a TGF-β inhibitor-treated mouse (A-D). Upper panels show GFP-expressing cancer cells; middle panels show RFP-expressing stromal cells, and lower panels show merged images with GFP and RFP. Scale bars: 1.0 mm.
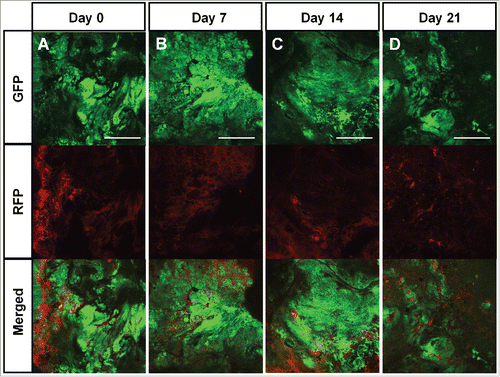
Figure 4. Quantitation of cancer cells and stromal cells in TGF-β inhibitor-treated and control pancreatic tumors. A) Line graphs show the percentage of RFP-expressing stromal area obtained by real-time intravital imaging in treated and untreated tumors. *P < 0.05; **P < 0.01; ***P < 0.001. Error bars: ± SD. B) Line graphs show real time increase of tumor volume in untreated and treated tumors.
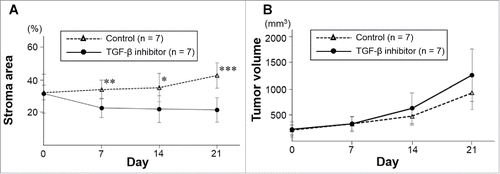
Excised whole tumors and frozen sections made from them, showed significantly lower stroma area in the TGF-β-treated tumors than control tumors (TGF-β inhibitor-treated: 34.7 ± 2.6% stromal fluorescent area, control: 54.0 ± 9.4% stromal fluorescent area, P < 0.001), confirming the results from real-time intravital imaging ().
Figure 5. Color-coded imaging of resected tumors and frozen sections from TGF-β inhibitors-treated and control tumors. Bright-field and fluorescence images of whole tumors were obtained with the OV100 Small Animal Imaging System (A). Representative images of frozen tumor sections in both groups imaged with the FV-1000 confocal microscope (B). Bar graph shows the percentage of RFP stromal area determined from frozen-section imaging (C). ***P < 0.001. Scale bars: 5 mm in (A); 500 μm in (B). Error bars: ± SD.
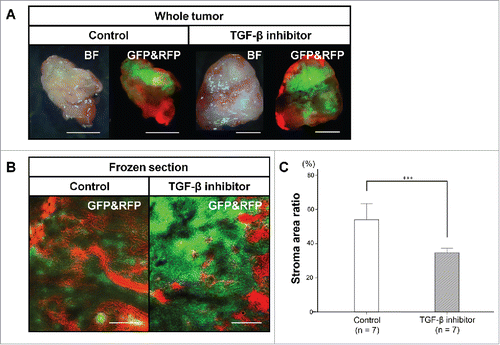
Figure 6. Comparison of color-coded fluorescent frozen sections and H&E-stained sections. Left panel shows a frozen section from a tumor imaged with the FV-1000 confocal microscope. Right panel is an image of same tumor stained with H&E. The GFP-expressing area in the frozen section overlapped with cancer cells in the H&E-stained slides. The RFP-expressing area in the frozen section overlapped with stromal cells in the H&E-stained slides. Scale bars: 200 μm.
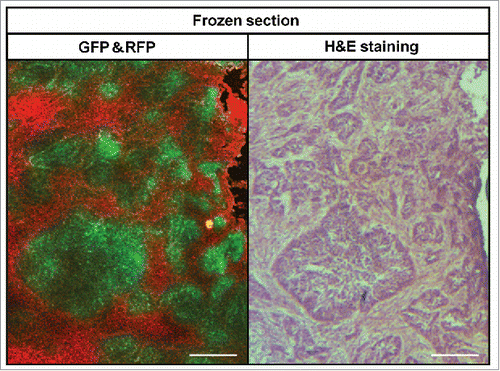
Frozen fluorescent sections and H&E stained slides of the same area from resected tumor specimens were compared (). The GFP-expressing area of the frozen sections corresponded to viable cancer cell nests in the H&E stained slides, and the RFP-expressing area of the frozen sections overlapped with area of stromal cells in the H&E stained slides.
The present study used the BxPC-3-GFP pancreatic cancer cell line orthotopically transplanted in nude mice. This cell line has been very well characterized by our laboratory and it behaves as a typical pancreatic cancer with regard to growth and metastasis.Citation14,15 With regard to changes in growth rates, surface markers, expression of other genes, cell function, and histology of epithelial or mesenchymal cancer cells, Cai et al.Citation16 have found that GFP expression does not change these fundamental properties of the cells. The study of Cai et al.Citation16 matches our own experience that GFP or other fluorescent proteins do not alter the fundamental properties of cancer cells.Citation9,17-19 In addition, transgenic mice, expressing fluorescent proteins in almost all tissues behave normally and have a normal life span, indicating that constitutive expression of fluorescent proteins in almost call the cells of a mouse does not alter their fundamental properties.Citation7,8,20
The present manuscript is a proof-of-principle that an agent could selectively target the stroma of a tumor in vivo as determined by color-coded imaging, in the present case, TGF-β1. Future experiments will investigate the effects of TGF-β1 in downstream targets.
In the present study, the cancer cells in the tumor are labeled with GFP and stromal cells with RFP. Future experiments will investigate the effects of a stromal inhibitor such as TGF-β on individual-cell types within the stroma.
Tumor volume was not affected by TGF-β1. Perhaps this was because cancer cell proliferation replaced inhibited stromal cells to an extent. Future experiments will compare GFP+ cancer-cell and RFP+ stromal-cell number over time during stromal-cell inhibition.
Previously-developed concepts and strategies of highly selective tumor targeting can take advantage of molecular targeting of tumors, including tissue-selective therapy which focuses on unique differences between normal and tumor tissues.Citation21-26
Conclusions
The present study has demonstrated that real-time intravital imaging of orthotopic pancreatic tumors could distinguish cancer and stromal cells expressing GFP and RFP, respectively. With this capability of intravital color-coded imaging, it was possible to demonstrate that the TGF-β antagonist SB431542 could selectively target stromal cells within the pancreatic tumor in the orthotopic mouse model. Future experiments will determine the individual cell types targeted by anti-stromal therapy in the color-coded model described in the present report.
Materials and methods
Cells
The human pancreatic cancer cell line BxPC-3 labeled with green fluorescent protein (GFP) was established as described previously.Citation14,15 BxPC-3 highly expressed TGF-β (Murakami, T., Hiroshima, Y., Hoffman, R.M., unpublished data).
Mice
Transgenic red fluorescent protein (RFP)-expressing athymic nu/nu nude miceCitation8 (AntiCancer Inc., San Diego, CA), 4–6 weeks old, were used in this study in accordance with the principles and procedures outlined in the Guide for the Care and Use of Laboratory Animals, 8th edition under Public Health Service Assurance Number A3873–1. Animals were housed with no more than 5 per cage in a barrier facility on a high efficiency particulate arrestance (HEPA)-filtered rack under standard conditions of 12-hour light/dark cycles. The animals were fed an autoclaved laboratory rodent diet. In order to minimize any suffering of the animals, anesthesia and analgesics were used for all surgical experiments. Animals were anesthetized by subcutaneous injection of a 0.02 ml solution of 25 mg/kg ketamine, 19 mg/kg xylazine, and 0.60 mg/kg acepromazine maleate. The response of animals during surgery was monitored to ensure adequate depth of anesthesia. The animals were euthanized by CO2 inhalation on day 21.
Subcutaneous injection of BxPC-3-GFP cell injection
BxPC-3-GFP cells were harvested by trypsynization after mono-layer culture and washed with medium. A total of 2 × 106 BxPC-3-GFP cells, suspended in 100 μl RPMI 1640 medium (Gibco Life Technologies, Grand Island, NY) with 50% Matrigel (Becton Dickinson, Franklin Lakes, NY) was injected subcutaneously to the flanks of RFP nude mice.
Establishment of dual-color fluorescent pancreatic-cancer model by surgical orthotopic implantation (SOI)
The subcutaneously grown tumors were harvested 3 weeks after cell injection. Tumors were cut into 4 mm3 fragments. A 5 mm left lateral incision was made in a transgenic RFP nude mouse. A tumor fragment was sutured orthotopically on the pancreatic tail using 8–0 nylon surgical sutures (Ethilon, Ethicon, Inc., NJ). The wound was closed with 6–0 nylon surgical suture (Ethilon).Citation27-30 Three weeks after orthotopic implantation, a color-coded fluorescent pancreatic-cancer mouse model was established.
Imaging
Whole-tumor macro imaging was performed using the OV100 Small Animal Imaging System (Olympus, Tokyo, Japan).Citation31 Bright-field and GFP- and RFP-fluorescence images were obtained. The OV100 contains an MT-20 light source (Olympus Biosystems, Planegg, Germany) and DP70 CCD camera (Olympus). The OV100 was used for subcellular imaging in live mice. The optics of the OV100 fluorescence imaging system have been specially developed for macroimaging as well as microimaging with high light-gathering capacity. The instrument incorporates a unique combination of high numerical aperture and long working distance. Individually optimized objective lenses, parcentered and parfocal, provide a 105-fold magnification range for seamless imaging of the entire body down to the subcellular level without disturbing the animal. The OV100 has the lenses mounted on an automated turret with a high magnification range of x1.6 to x16 and a field of view ranging from 6.9 to 0.69 mm. The optics and antireflective coatings ensure optimal imaging of multiplexed fluorescent reporters in small animals. High-resolution images were captured directly on a PC (Fujitsu Siemens, Munich, Germany). Images were processed for contrast and brightness and analyzed with the use of Paint Shop Pro 8 and CellR (Olympus Biosystems).Citation31
Intravital imaging
Confocal microscopy (Fluoview FV1000, Olympus Corp., Tokyo, Japan) was used for two- (x,y) and three-dimensional (3D, x,y,z) high-resolution intravital imaging with a cw semiconductor laser at 473 nm for GFP and RFP excitation (Newport-Spectra Physics, Irvine, CA). Deep tissue fluorescence imaging used the 20x/0.50 UPlan FLN and 40x/1.3 Oil Olympus UPLAN FLN objectives.Citation32
Intravital confocal imaging was performed four times: before treatment, day 7, day 14, and day 21. After mice were anesthetized by subcutaneous injection, a 5 mm left lateral incision was made. The pancreatic tail with a grown tumor was exposed through the incision. The tumor surface was cut by a scalpel and the capsule was removed in order to image the inside of the tumor. After hemostasis, the mouse was set under the FV1000 confocal microscope. The exposed tumor was placed between a sterilized board and a slide glass, exerting a slight pressure on the cut surface (). The entire cut surface area was scanned for color-coded fluorescence imaging of the GFP expressing cancer cells and RFP expressing stromal cells. The wound was closed with 6–0 nylon surgical sutures. Mice were returned to their cages when they began to walk. The percentage of the RFP fluorescence area from the stromal cells relative to the total fluorescence area was calculated with ImageJ v1.49f (National Institutes of Health, Bethesda, MD).
TGF-β inhibitor treatment
When the tumor diameter reached 7 mm, the mice (n = 14) were randomized into a control group (n = 7, 200 μl of vehicle, i.p., weekly, for 3 weeks) and a treated group (n = 7, SB431542 [ALK5] TGF-β1 inhibitor, Selleck chemicals, Houston, TX, 0.3 mg in 200 μl solution, i.p., weekly, for 3 weeks). Intraperitoneal administration of TGF-β1 in each group was performed on day 1, 8, and 15 using a 27-gauge needle.
Frozen sections
Resected tumors were washed with phosphate buffered saline was then dried. Tumors were sliced to 2–3 mm thickness, then embedded in Neg-50 Frozen Section Medium (Thermo Fisher Scientific, Waltham, MA) and immediately frozen with liquid nitrogen. Tissue sections (8 μm) were made with a cryostat (Leica, Buffalo Grove, IL).
Fluorescence imaging of frozen sections
To confirm results from intravital imaging, frozen sections, derived from resected tumor specimens, were imaged with the FV1000 confocal microscope. One slide from the center of each tumor was imaged, since as the tumor center most likely accurately reflects the characteristics of each tumor. The percentage of RFP fluorescent area from the stromal cells, relative to the total fluorescent area (GFP and RFP), was calculated with ImageJ.
Histological examination
Hematoxylin and eosin (H&E) staining was performed according to standard protocols: Fresh tumor specimens were fixed in 10% formalin and embedded in paraffin before sectioning and staining. Tissue sections were deparaffinized in xylene and rehydrated in an ethanol series. H&E staining was performed according to standard protocols. Histological examination was performed with a BHS system microscope. Images were acquired with INFINITY ANALYZE software (Lumenera Corporation, Ottawa, Canada).
Statistical analysis
SPSS statistics version 21.0 was used for all statistical analyses (IBM, New York City, NY, USA). Significant differences for continuous variables were determined using the Student's t-test. Both line graphs and bar graphs express the mean values and error bars show standard deviation (SD). A probability value of P ≤ 0.05 was considered statistically significant.
Dedication
This paper is dedicated to the memory of A. R. Moossa, M.D. and Sun Lee, M.D.
Disclosure of potential conflicts of interest
No potential conflicts of interest were disclosed.
References
- Siegel R, Ma J, Zou Z, Jemal A. Cancer statistics, 2014. CA Cancer J Clin 2014; 64:9-29; PMID:24399786; http://dx.doi.org/10.3322/caac.21208
- Hidalgo M. Pancreatic cancer. N Engl J Med 2010; 362:1605-17; PMID:20427809; http://dx.doi.org/10.1056/NEJMra0901557
- Korc M. Pancreatic cancer-associated stroma production. Am J Surg 2007; 194(4 Suppl):S84-86; PMID:17903452; http://dx.doi.org/10.1016/j.amjsurg.2007.05.004
- Saluja AK, Dudeja V, Banerjee S. Evolution of novel therapeutic options for pancreatic cancer. Curr Opin Gastroenterol 2016; 7:401-407.
- Katz LH, Likhter M, Jogunoori W, Belkin M, Ohshiro K, Mishra L. TGF-β signaling in liver and gastrointestinal cancers. Cancer Lett 2016; 379:166-172; PMID:27039259; http://dx.doi.org/10.1016/j.canlet.2016.03.033
- Yang M, Li L, Jiang P, Moossa A, R Penman S, Hoffman RM. Dual-color fluorescence imaging distinguishes tumor cells from induced host angiogenic vessels and stromal cells. Proc Natl Acad Sci USA 2003; 100:14259-262; PMID:14614130; http://dx.doi.org/10.1073/pnas.2436101100
- Yang M, Reynoso J, Jiang P, Li L, Moossa AR, Hoffman RM. Transgenic nude mouse with ubiquitous green fluorescent protein expression as a host for human tumors. Cancer Res 2004; 64:8651-56; PMID:15574773; http://dx.doi.org/10.1158/0008-5472.CAN-04-3118
- Yang M, Reynoso J, Bouvet M, Hoffman RM. A transgenic red fluorescent protein-expressing nude mouse for color-coded imaging of the tumor microenvironment. J Cell Biochem 2009; 106:279-84; PMID:19097136; http://dx.doi.org/10.1002/jcb.21999
- Hoffman RM, Yang M. Color-coded fluorescence imaging of tumor-host interactions. Nat Protocols 2006; 1:928-35; PMID:17406326; http://dx.doi.org/10.1038/nprot.2006.119
- Bouvet M, Tsuji K, Yang M, Jiang P, Moossa AR, Hoffman RM. In vivo color-coded imaging of the interaction of colon cancer cells and splenocytes in the formation of liver metastases. Cancer Res 2006; 66:11293-297; PMID:17145875; http://dx.doi.org/10.1158/0008-5472.CAN-06-2662
- Suetsugu A, Katz M, Fleming J, Truty M, Thomas R, Saji S, Moriwaki H, Bouvet M, Hoffman RM. Imageable fluorescent metastasis resulting in transgenic GFP mice orthotopically implanted with human-patient primary pancreatic cancer specimens. Anticancer Res 2012; 32:1175-80; PMID:22493347
- Suetsugu A, Katz M, Fleming J, Truty M, Thomas R, Saji S, Moriwaki H, Bouvet M, Hoffman RM. Non-invasive fluorescent-protein imaging of orthotopic pancreatic-cancer-patient tumorgraft progression in nude mice. Anticancer Res 2012; 32:3063-68; PMID:22843874
- Yang M, Jiang P, Hoffman RM. Whole-body subcellular multicolor imaging of tumor-host interaction and drug response in real time. Cancer Res 2007; 67:5195-200; PMID:17545599; http://dx.doi.org/10.1158/0008-5472.CAN-06-4590
- Bouvet M, Wang J, Nardin SR, Nassirpour R, Yang M, Baranov E, Jiang P, Moossa AR, Hoffman RM. Real-time optical imaging of primary tumor growth and multiple metastatic events in a pancreatic cancer orthotopic model. Cancer Res 2002; 62:1534-40; PMID:11888932
- Bouvet M, Yang M, Nardin S, Wang X, Jiang P, Baranov E, Moossa AR, Hoffman RM. Chronologically-specific metastatic targeting of human pancreatic tumors in orthotopic models. Clin Exp Metastasis 2000; 18:213-18; PMID:11315094; http://dx.doi.org/10.1023/A:1006767405609
- Cai WX, Zheng LW, Huang HZ, Zwahlen RA. Evidence of phenotypic stability after transduction of fluorescent proteins in two human tongue cancer cell lines. Archives Oral Biol 2017; 79:48-54; PMID:28288391; http://dx.doi.org/10.1016/j.archoralbio.2017.03.002
- Hoffman RM. The multiple uses of fluorescent proteins to visualize cancer in vivo. Nat Rev Cancer 2005; 5:796-806; PMID:16195751; http://dx.doi.org/10.1038/nrc1717
- Hoffman RM, Yang M. Subcellular imaging in the live mouse. Nat Protocols 2006; 1:775-82; PMID:17406307; http://dx.doi.org/10.1038/nprot.2006.109
- Hoffman RM, Yang M. Whole-body imaging with fluorescent proteins. Nat Protocols 2006; 1:1429-38; PMID:17406431; http://dx.doi.org/10.1038/nprot.2006.223
- Tran Cao HS, Reynoso J, Yang M, Kimura H, Kaushal S, Snyder CS, Hoffman RM, Bouvet M. Development of the transgenic cyan fluorescent protein (CFP)-expressing nude mouse for “Technicolor” cancer imaging. J Cell Biochem 2009; 107:328-34; PMID:19306297; http://dx.doi.org/10.1002/jcb.22128
- Blagosklonny MV. Matching targets for selective cancer therapy. Drug Discov Today 2003; 8:1104-07; PMID:14678733; http://dx.doi.org/10.1016/S1359-6446(03)02806-X
- Blagosklonny MV. Teratogens as anti-cancer drugs. Cell Cycle 2005; 4:1518-21; PMID:16258270; http://dx.doi.org/10.4161/cc.4.11.2208
- Blagosklonny MV. Treatment with inhibitors of caspases, that are substrates of drug ransporters, selectively permits chemotherapy-induced apoptosis in multidrug-resistant cells but protects normal cells. Leukemia 2001; 15:936-41; PMID:11417480; http://dx.doi.org/10.1038/sj.leu.2402127
- Blagosklonny MV. Target for cancer therapy: Proliferating cells or stem cells. Leukemia 2006; 20:385-91; PMID:16357832; http://dx.doi.org/10.1038/sj.leu.2404075
- Apontes P, Leontieva OV, Demidenko ZN, Li F, Blagosklonny MV. Exploring long-term protection of normal human fibroblasts and epithelial cells from chemotherapy in cell culture. Oncotarget 2011; 2:222-33; PMID:21447859; http://dx.doi.org/10.18632/oncotarget.248
- Blagosklonny MV. Tissue-selective therapy of cancer. Br J Cancer 2003; 89:1147-51; PMID:14520435; http://dx.doi.org/10.1038/sj.bjc.6601256
- Fu X, Guadagni F, Hoffman RM. A metastatic nude-mouse model of human pancreatic cancer constructed orthotopically from histologically intact patient specimens. Proc Natl Acad Sci USA 1992; 89:5645-49; PMID:1608975; http://dx.doi.org/10.1073/pnas.89.12.5645
- Furukawa T, Kubota T, Watanabe M, Kitajima M, Hoffman RM. A novel “patient-like” treatment model of human pancreatic cancer constructed using orthotopic transplantation of histologically intact human tumor tissue in nude mice. Cancer Res 1993; 53:3070-72; PMID:8319214
- Hoffman RM. Orthotopic metastatic mouse models for anticancer drug discovery and evaluation: A bridge to the clinic. Investigational New Drugs 1999; 17:343-59; PMID:10759402; http://dx.doi.org/10.1023/A:1006326203858
- Hoffman RM. Orthotopic is orthodox: Why are orthotopic-transplant metastatic models different from all other models? J Cell Biochem 1994; 56:1-3; http://dx.doi.org/10.1002/jcb.240560102
- Yamauchi K, Yang M, Jiang P, Xu M, Yamamoto N, Tsuchiya H, Tomita K, Moossa AR, Bouvet M, Hoffman RM. Development of real-time subcellular dynamic multicolor imaging of cancer-cell trafficking in live mice with a variable-magnification whole-mouse imaging system. Cancer Res 2006; 66:4208-14; PMID:16618743; http://dx.doi.org/10.1158/0008-5472.CAN-05-3927
- Uchugonova A, Duong J, Zhang N, König K, Hoffman RM. The bulge area is the origin of nestin-expressing pluripotent stem cells of the hair follicle. J Cell Biochem 2011; 112:2046-50; PMID:21465525; http://dx.doi.org/10.1002/jcb.23122