ABSTRACT
Mammalian oocyte chromosomes undergo 2 meiotic divisions to generate haploid gametes. The frequency of chromosome segregation errors during meiosis I increase with age. However, little attention has been paid to the question of how aging affects sister chromatid segregation during oocyte meiosis II. More importantly, how aneuploid metaphase II (MII) oocytes from aged mice evade the spindle assembly checkpoint (SAC) mechanism to complete later meiosis II to form aneuploid embryos remains unknown. Here, we report that MII oocytes from naturally aged mice exhibited substantial errors in chromosome arrangement and configuration compared with young MII oocytes. Interestingly, these errors in aged oocytes had no impact on anaphase II onset and completion as well as 2-cell formation after parthenogenetic activation. Further study found that merotelic kinetochore attachment occurred more frequently and could stabilize the kinetochore-microtubule interaction to ensure SAC inactivation and anaphase II onset in aged MII oocytes. This orientation could persist largely during anaphase II in aged oocytes, leading to severe chromosome lagging and trailing as well as delay of anaphase II completion. Therefore, merotelic kinetochore attachment in oocyte meiosis II exacerbates age-related genetic instability and is a key source of age-dependent embryo aneuploidy and dysplasia.
Introduction
Following the pre-meiotic S phases, the chromosomes of mammalian oocytes undergo 2 meiotic divisions to generate haploid gametes.Citation1,2 The division at meiosis I is unique, resulting in the dissolution of cohesin wrapped around chromosome arms and homologous chromosome segregation; the oocyte extrudes its first polar body, undergoing a brief period of interkinesis, and then arrests at the metaphase of the second meiotic division (MII).Citation2,3 The fertilizing sperm, or Sr2+ solution, triggers completion of meiosis II and sister chromatid (dyad) segregation, an event that is the same as the separation of sister chromatids in mitosis.Citation4 To successfully complete meiosis II, oocyte chromosomes must congress to the spindle equator and generate amphitelic kinetochore attachments in which each sister kinetochore is connected to microtubules from each of the 2 opposite poles. When a single kinetochore binds microtubules oriented toward both spindle poles, a prominent error called merotely will occur.Citation5,6 Merotelic (bi-directional) kinetochore orientation occurs very frequently during early mitosis in mammalian tissue cells.Citation6,7 This orientation is predominant in prometaphase of meiosis I and is then corrected to amphitelic attachments to establish stable chromosome bi-orientation in mouse oocytes.Citation8 The kinetochore attachment status of misaligned chromosomes in in vitro mature MII oocytes from aged MF1 mice has been shown.Citation9 However, the type of kinetochore-microtubule (k-MT) interaction in in vivo matured MII oocytes from naturally aged Swiss CD1 mice is not very clear.
Increasing evidence suggests that oocytes from older women become susceptible to chromosome missegregation and generate aneuploid embryos, leading to increased infertility and birth defects.Citation1,2,4 Down syndrome is the archetype of human aneuploidy. Recently, some researchers have suggested that age-related aneuploid embryos are predominantly a consequence of chromosome segregation errors during the first meiotic division.Citation10-12 Several hypotheses regarding the cause of maternal age-related aneuploidy have been proposed, including the loss of key proteins, deterioration of metabolic processes and increases in oxidative damage.Citation13-16 One attractive hypothesis is that aging oocytes lose both proteins responsible for maintaining cohesionCitation12,17,18 and protecting centromeric cohesion.Citation11,19 Such a loss could cause deterioration of cohesion.Citation4,11,12 Cohesion at the centromeres persists until anaphase II in oocytes.Citation12,14,20 In mitosis, centromeric cohesion produces tension by counteracting the pulling force of microtubules that capture sister kinetochores from opposite poles, and this tension has a crucial role in stabilizing attachment.Citation21 The principal kinetochore attachment to microtubules in meiosis II is thought to be virtually the same process as occurs in mitosis.Citation21 Therefore, when centromeric cohesion is lost in the aged MII oocytes, what changes in their k-MT orientation will occur? In addition, whatever factor causes age-related aberrant chromosome number before meiosis II, how these aneuploid MII oocytes evade the spindle assembly checkpoint (SAC) mechanism to complete later meiosis II and to form aneuploid embryos remains unknown. Most previous studies focused on the effect of aging on homologous chromosome segregation;Citation10-12 however, the oocyte must complete sister chromatid separation before fertilization to form the embryo. Aging whether affects sister chromatid separation is very poor.
To address the above problems, we first present a detailed examination of the k-MT interaction and the process of sister chromatid separation during meiosis II in oocytes from naturally aged mice using k-MT immunofluorescence and chromosome live-cell tracking. Our results suggest that merotelic kinetochore attachment in oocyte meiosis II exacerbates age-related genetic instability and is a key source of age-dependent embryo aneuploidy and dysplasia.
Results
Chromosome misalignment, premature dyad segregation and weakened cohesion in MII oocytes from aged mice
To examine chromosome alignment and cohesin protein expression in MII oocytes from 6–8-week-old (young group) and 11–12-month-old mice (aged group), oocytes were stained with 4′, 6-diamidino-2-phenylindole (DAPI) and anti-centromeric serum (Crest) to label chromosomes and centromeres, respectively. It was demonstrated that 23.8% of aged MII oocytes (20/84) and less than 3% of young oocytes (3/102) exhibited chromosome misalignment (). In addition, the rate of oocytes with premature dyad segregation in the young group was 10.8% (11/102), whereas the rate in the aged group reached 48.8% (41/84) (P < 0.01) ( and ).
Figure 1. Aging causes centromeric cohesion deterioration, chromosome misalignment and premature sister chromatid segregation. (A) Percentages of young and aged MII oocytes with chromosomes aligned or misaligned at the spindle equator. Chromosomes were labeled with DAPI. In total, 84 aged and 102 young MII oocytes from more than 3 experiments were analyzed. (B) Localization of chromosomes and chromatids in MII oocytes from young and old mice. One chromatid aligned at the spindle equator is shown by a white arrow, and a green arrow indicates one misaligned chromatid. Red, centromere (Crest); blue, chromosome (DAPI). (C) The rate of MII oocytes with single chromatids in aged and young mice. The data were analyzed using a chi-square test. (D) Representative images of Rec8 protein immunostaining of aged and young MII oocytes. Green, Rec8; blue, chromosome (DAPI); red, centromere (Crest). (E) The relative Rec8 intensity of kinetochores was quantified. The data are the means ± SEM, ≥ 260 sister pairs of each group were analyzed. In A, C and E, **P <0.01. Scale bars in B and D, 5 μm.
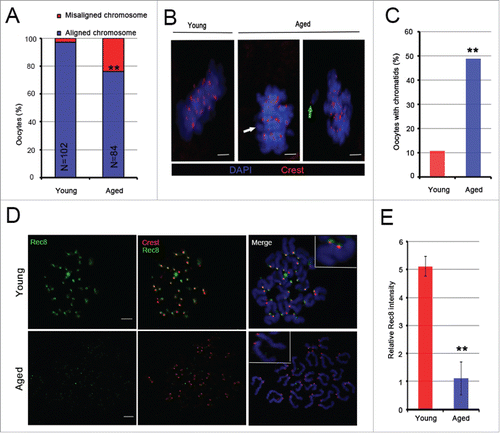
Sister chromatids are kept together by centromeric cohesion.Citation12,14,20 The proteins Rec8 and Smc3 are 2 cohesin subunits responsible for centromeric cohesion.Citation22,23 Therefore, to explore the reason for the increased misaligned chromosomes and single chromatids in the aged group, we examined the expression levels of Rec8 and Smc3 proteins surrounding the centromeres using immunofluorescence. We observed that the expression levels of Rec8 and Smc3 proteins were markedly decreased in the aged group ( and , Supplementary and ). Compared to the young group, the relative Rec8 and Smc3 fluorescence intensity had a significant decrease in the aged group (P < 0.01 and P < 0.05, respectively) (, Supplementary ). Thus, our results show that aging causes a substantial increase in chromosome misalignment and premature dyad segregation in MII oocytes, which may be explained by cohesion deterioration associated with maternal age.
Elevated bi-directional kinetochore attachment during oocyte meiosis II in aged mice
To explore the effect of misaligned or single chromatids on k-MT interactions, we assessed the type of k-MT attachment using kinetochore and microtubule immunostaining after destabilizing dynamic, non-kinetochore microtubules with cold treatment in fixed MII oocytes from young and aged mice. The slices analyzed by confocal microscopy revealed a total of 3 types of linkages formed by bundled kinetochore microtubules in young and aged MII oocytes, including polar (microtubules from one pole attach to one kinetochore on its end); merotelic (microtubules from 2 poles attach to one kinetochore), sister pair bi-directional (one bunch of microtubules crosses the central space of sister kinetochores) (). Polar attachment was strikingly reduced in the aged MII oocytes (64.7%, n = 486, N = 16) compared with the young MII oocytes (85.5%, n = 446, N = 14, P < 0.05) (). Further analysis found that at least one kinetochore had a merotelic interaction in 7/38 young and 26/46 aged MII oocytes (P < 0.01). The incidence of MII oocytes with at least one sister pair attached to the same microtubule bundle was also significantly increased in the aged group compared with the young group (41.3%, 19/46; 10.5%, 4/38, P < 0.01, respectively).
Figure 2. Merotelic kinetochore and sister pair bi-directional attachments are increased significantly in aged MII oocytes. (A) Representative images of spindles and chromosomes in young and aged MII oocytes are shown. The right side shows the amplified images of polar kinetochore (white arrow), merotelic kinetochore (yellow and red arrow) and sister pair bi-directional (blue arrow) attachments. i shows 2 ends-on attachment, and ii shows lateral attachment. i and ii both belong to the merotelic kinetochore attachment category. DNA: blue, kinetochore: red, α-tubulin: green. Scale bar, 5 μm. (B) The rate of polar kinetochore attachment was analyzed in 14 young and 16 aged MII oocytes with 446 and 486 chromatids, respectively. The data represent the means ± SEM and were collected from 5 independent experiments, *P < 0.05. (C) The percentages of MII oocytes with merotelic kinetochore and sister pair bi-directional attachments in young and aged groups are shown. The data were analyzed using a chi-square test. **P < 0.01.
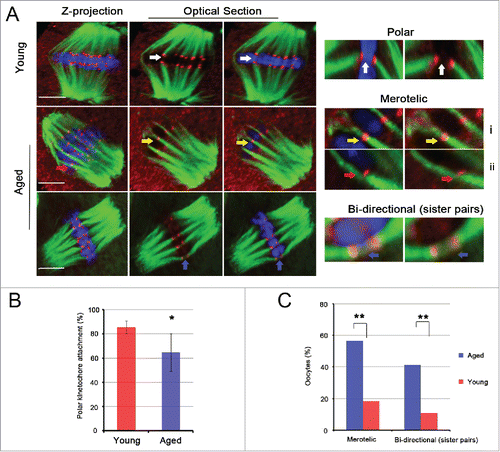
To investigate whether these bi-directional kinetochore attachments persisted during anaphase II, we checked the type of k-MT interaction in anaphase II of aged and young oocytes (). Here, anaphase II was divided into 2 stages, anaphase II A (moving chromatids poleward) and anaphase II B (elongating the spindle).Citation24-26 We found that bi-directional kinetochore attachments had a significant increase during anaphase II A in aged oocytes (204/578, N = 20) compared with young oocytes (101/635, N = 26) ().
Figure 3. Bi-directional kinetochore attachment persists during oocyte anaphase II. (A) Different states of k-MT attachments and chromosomes during oocyte anaphase II are exhibited. Anaphase II is divided into anaphase II A and anaphase II B. In anaphase II A, kinetochores are attached by polar attachments (red arrow), Normal; some kinetochores are attached by merotelic attachments (white arrow), or one dyad is attached by sister pair bi-directional attachment (yellow arrow), Abnormal. In anaphase II B, no lagging chromosome, Normal; at least one lagging chromosome (white asterisk), Abnormal. Scale bar, 5μm. (B) Kinetochore attached by bipolar spindle microtubules in anaphase II A was quantified in 20 aged and 26 young oocytes. P = 0.005. The data were collected from 6 independent experiments and are shown as means ± SEM.
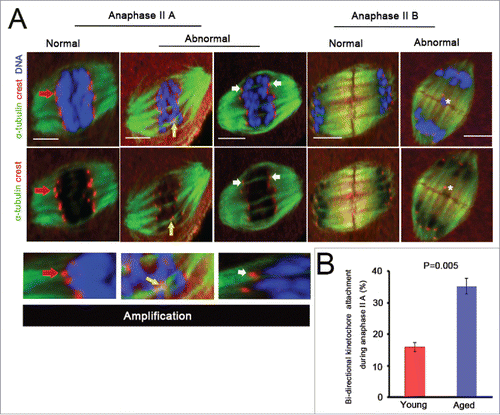
Misaligned or single chromatids were often attached by bi-directional kinetochore microtubule, as indicated in and Supplementary Figure 2. Taken together, aging causes a substantial increase in merotelic kinetochore interaction during oocyte meiosis II.
The SAC mechanism is inactive in the aged MII oocytes
SAC is a unique signaling mechanism that prevents anaphase until stable connections between the spindle microtubules and the kinetochores of all chromosomes are established.Citation27,28 To investigate the activation of the SAC mechanism in aged MII oocytes with substantial merotelic kinetochore attachment, the checkpoint protein Mad2 was detected at the kinetochores using immunofluorescence in aged and young MII oocytes (). We found that the relative Mad2 fluorescence intensities at the kinetochores were identical for the aged and young MII oocytes ( and ); that is, there was no Mad2 signal at their kinetochores and thus SAC silence occurred. To avoid the low Mad2 levels in the kinetochores in the aged MII oocytes caused by aging, we disrupted the spindle with the microtubule depolymerizing agent nocodazole in the aged MII oocytes (). We found that this treatment resulted in a marked increase in Mad2 levels in nearly all kinetochores in aged MII oocytes (). Further analysis found that Mad2 had similar intensities for the kinetochores in young and aged oocytes after treatment of 1 hour (h) in strontium activation medium containing nocodazole (). Furthermore, the aged MII oocytes entered into anaphase II as efficiently as the young MII oocytes, taking approximately 40 minutes (min) from activation to anaphase II onset ().
Figure 4. Aging has no impact on SAC mechanism. (A) Mad2 immunostaining was performed in young and aged MII oocytes and activated oocytes (60 min after Sr2+ addition) in the presence or absence of nocodazole. Images represent a single Z-plane. Scale bar, 5 μm. DNA: blue, kinetochore: red, Mad2: green. The right images are magnified pictures of the white framed areas. (B) Relative Mad2/Crest intensity was calculated in young and aged MII oocytes without (MII) or with nocodazole (MII + Noc) treatment and was calculated when nocodazole was added to the activation medium for 60 min (Activation + Noc). For each treatment, ≥ 310 kinetochores were analyzed. The data are the means ± SEM and were collected from 4 independent experiments.
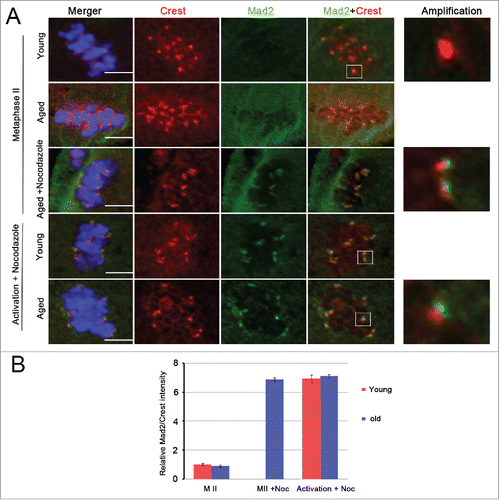
Figure 5. Chromosome lagging and trailing, and the delay of anaphase II completion in aged oocytes. The whole anaphase II of 33 young and 31 aged oocytes from 9 independent experiments was observed by time-lapse imaging every 3 or 5 min. The chromosomes were labeled using Hoechst 3342 dye (blue changes into red in the figure). In A and B, the data represent the means ± SEM, *P = 0.05. In C, the data were analyzed using a chi-square test. (D) Sister chromatids localize at the metaphase plate and segregate into 2 separate chromosome structures in the second meiosis division, Normal. Single chromatids or sister pairs remain at the metaphase plate, or do not reach a spindle pole during anaphase II, Lagging (white arrow). Misaligned chromatid before anaphase onset (green arrow) and chromosomes have a severe trailing phenomenon (blue arrow), Misalignment. Scale bar, 10 μm. (E) The percentage of oocytes with chromosome misalignment or lagging was calculated in the young and aged groups. The data represent the means ± SEM, ** P < 0.01. (F) The number of lagging chromosomes was determined in 4 young and 19 aged, fixed oocytes during anaphase II; * represents one chromosome. The experiments were replicated more than 4 times.
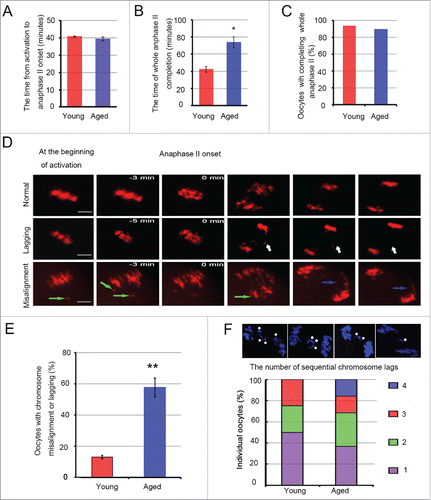
Collectively, these results show that the SAC mechanism is inactive in aged MII oocytes with substantial merotelic kinetochore attachment. Aging has no impact on SAC activation.
Delay of anaphase completion and sister chromatid segregation error in aged oocytes
To examine whether chromosome misalignment and merotelic kinetochore attachment obstruct the ability to complete the second meiotic division or influence sister chromatid segregation in mouse oocytes, we treated oocytes with SrCl2 activation solution to activate the MII-arrested oocytes and then monitored the whole anaphase II using live-cell imaging. We found that most oocytes could complete the whole anaphase II (∼90.0%) (), regardless of maternal age. However, aged oocytes took much more time to complete anaphase II than young oocytes (73.99 ± 6.34 vs 42.47 ± 3.33 min, P = 0.05, respectively) (). To investigate the reason for this phenomenon, the process of sister chromatid segregation in individual oocytes was studied (). The results showed that the number of misaligned or lagging chromosomes during anaphase II was strikingly increased in aged oocytes (19/33) compared with young oocytes (4/31) (P < 0.01) (). Misaligned chromatids in MII oocytes did not re-align at the spindle equator and were directly pulled to one spindle pole (). In addition, we also observed that 15.9% of aged oocytes containing lagging chromosomes had 4 lagging chromosomes ().
Briefly, aging causes chromosome lagging and trailing during oocyte anaphase II, which provides an explanation for the delay of anaphase II completion in aged oocytes.
Reduced rate and quality of blastocysts in aged mice
The above results showed that aging had no effect on anaphase II onset and completion in oocytes, but how about their post-development? Further parthenogenetic activation of young and aged MII oocytes was performed in vitro. The results showed that the percentage of oocytes that developed into 2-cell embryos was similar between the groups (P > 0.05) (). However, the percentage of oocytes that developed into blastocysts in the young group (78.5 ± 1.3%) was significantly higher than that of the aged group (56.9 ± 2.2%; P < 0.05) (). The cell nucleus and cleaved caspase 3 proteins of these blastocysts were stained (). A significant decrease in the total cell number (P = 0.045) and a striking increase in the expression of cleaved caspase 3 (P = 0.002) were observed in aged mice when compared with their young counterparts (, ). These results suggest that the embryo quality is poor in aged mice.
Figure 6. Blastocyst quality in aged mice shows an obvious decrease. The 2-cell and blastocyst embryos were collected 48 and 96 h after in vitro culturing. In total, 121 young and 72 aged oocytes were activated. (A) Left: representative images of 2-cell and blastocyst embryos are shown, scale bar = 350 μm. Right: the percentages of 2-cell and blastocyst embryos, * P < 0.05. (B) The blastocysts were stained with cleaved caspase 3 (white arrow, green). DNA was counterstained with DAPI (blue). Scale bar, 10 μm. (C) The average cell number of one blastocyst. (D) The number of cells containing cleaved caspase 3 signal in one blastocyst. The data are the means ± SEM, and experiments were replicated more than 4 times.
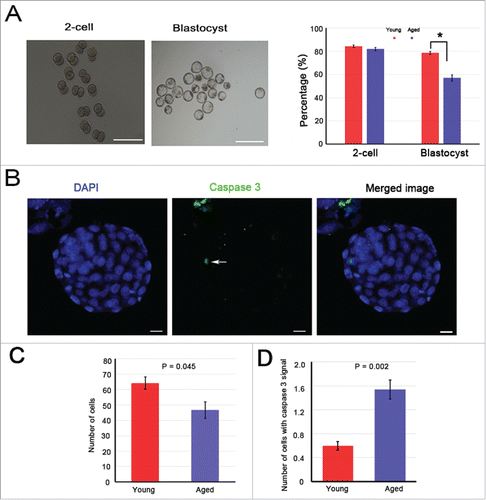
Discussion
Sister chromatids are always held together by meiotic cohesin until anaphase II centromeric cohesin dissolves in oocytes.Citation12,14,20 In mammals, meiotic cohesin is composed of several subunits, including Smc1β,Citation22 the kleisin Rec8,Citation23 Stag329 and Smc3.Citation14 In the present study, we found the Rec8 and Smc3 proteins at the kinetochores were strikingly decreased in the aged MII oocytes (, and Supplementary Fig. 1), and more than 45% of aged oocytes carried single chromatids (). In mice and Drosophila deficient in Smc1β, premature chromosome separation in oocytes was observed, and the loss of cohesin worsened with maternal age.Citation30,31 Loss of cohesin at the core of the centromere led to increased separation of sister kinetochores,Citation2,12,32 resulting in the formation of MII oocytes that carry unbalanced chromatids.Citation33,34 Therefore, centromeric cohesin deterioration is a major age-related lesion that provides an explanation for aberrant chromosome number in MII oocytes.
Cohesion between sister chromatids is important for preventing merotelic attachment.Citation21,35 Centromeric cohesion produces tension by counteracting the pulling force of microtubules that capture sister kinetochores from opposite poles, and this tension has a crucial role in stabilizing monopolar attachment.Citation21 When centromeric cohesin weakens, the tension generated by cohesion will be destroyed in the aged MII oocytes. Therefore, in aged MII oocytes, it is likely that monopolar attachment is replaced by bi-directional attachment to generate new tension and to contribute to microtubule stabilization. This hypothesis has been proposed in Sycp3−/− MII oocytes.Citation36 In addition, weakened centromeric cohesion can lead to the appearance of single chromatids,Citation33,34 as shown in our results (). Gregan et al. believe that it is possible that the merotelic orientation is the only way for single kinetochores to achieve stable attachment and satisfy the SAC mechanism.Citation35 Hence, weakened cohesion is one important factor in the increase of bi-directional kinetochore attachment in aged MII oocytes.
Merotelic k-MT attachment is an error that occurs when a single kinetochore is attached to microtubules emanating from both spindle poles.Citation35,37 The interaction between kinetochores and microtubules is stochastic, and sister kinetochores rarely attach to microtubules simultaneously.Citation21,38,39 Merotelically attached kinetochores are frequently observed but most are corrected in the early stages of mitosis and meiosis I.Citation7,8,25 However, our results showed that the merotelic kinetochore attachment significantly increased in aged MII oocytes () and persisted during anaphase II (). In mitosis, merotelically attached kinetochores that persist until anaphase can cause chromatids to lag on the mitotic spindle and hinder their timely segregation.Citation35 The data of Cimini et al. clearly indicate that merotelic orientation of individual kinetochores is the major mechanism responsible for lagging chromosomes at anaphase in mitosis.Citation6 In the present study, we found that one sister pair attached by the same microtubule bundle (we think that each kinetochore of sister pairs is attached by bi-orientation) still stayed at the spindle equator after oocytes had entered into anaphase II (). Thus, bi-directional kinetochore attachment in aged oocyte meiosis II is a key source of chromosome lagging, hindering sister chromatid segregation.
In the present study, tension between sister kinetochores is lacking in aged MII oocytes because of age-related cohesin deterioration. Cell cycle control mechanisms prevent anaphase onset in the absence of tension between sister kinetochores.Citation40,41 However, aged MII oocytes could rapidly enter into anaphase II, even without any delay (). Thus, it is very possible that tension established through bi-directional kinetochore attachment could help age-related aneuploid oocytes to inactivate the SAC mechanism (, ), promoting chromatid transmission.Citation36 Previously, some studies have suggested that merotelic kinetochore orientation represents a major mechanism of aneuploidy in mitotic cellsCitation6 and is the primary mechanism of chromosomal instability in cancer cells.Citation37,42 In mammalian oocytes, merotely is also suggested to be an important promoter of aneuploidy.Citation36 Furthermore, no matter which factor causes aberrant chromosome numbers before meiosis II in aged oocytes, if the interaction between microtubules and kinetochore attachment could not inactivate the SAC mechanism, these oocytes would not have any chance to generate aneuploid gametes and embryos. Therefore, merotelic kinetochore attachment during meiosis II in aged oocytes is the major mechanism responsible for age-dependent embryo aneuploidy and dysplasia.
Taken together, our results demonstrated that there is an increase in bi-directional kinetochore attachment, which may be associated with weakened centromeric cohesin in the aged MII oocytes. Merotelic kinetochore attachment may help age-related aneuploid MII oocytes to elude the SAC mechanism and enter into anaphase II, leading to chromosome lagging and trailing () as well as the delay of anaphase II completion. Finally, embryo quality in aged mice is poor. In conclusion, merotelic kinetochore attachment in oocyte meiosis II is a key source for age-related embryo aneuploidy and dysplasia.
Figure 7. Model for age-related sister chromatid segregation error in oocyte meiosis II. Aging causes the loss of centromere cohesion, which has a crucial role in stabilizing monopolar kinetochore attachment.Citation21 Sister chromatids wrapped by centromeric cohesion are attached by amphitelic spindle microtubules for arrangement at the spindle equator and silencing the SAC mechanism in young oocytes. When centromeric cohesion deteriorates in the aged MII oocytes, monopolar kinetochore attachments may be replaced by (i) merotelic kinetochore or (ii) sister pair bi-directional attachments. These replacements generate new tensions that elude the SAC tension-monitoring mechanism. Some chromosomes attached by these replacement orientations show lagging and trailing because a single kinetochore is pulled simultaneously by 2 polar microtubules during anaphase II. Hence, bi-directional kinetochore attachment is the major reason for sister chromatid segregation errors during oocyte meiosis II in aged mice.
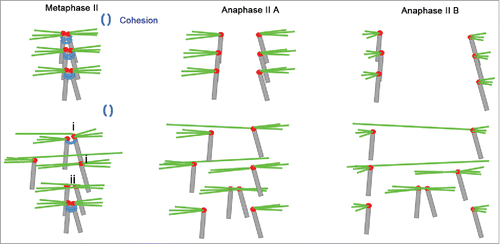
Materials and methods
All chemicals and media were purchased from Sigma Chemical Co. (St. Louis, MO, USA), unless otherwise indicated.
Antibodies
The following antibodies and dilutions were used for immunofluorescence studies: rabbit monoclonal anti-Smc3, 1:20 (Abcam, ab128919); rabbit polyclonal anti-Mad2, 1:20 (Biolegend, 924601); rabbit polyclonal anti-Rec8, 1:50 (Proteintech, 10793–1-AP); human anti-centromere autoimmune (Crest) serum, 1:200 (Immunovision, HTC-0100); mouse monoclonal anti-α-tubulin, 1:200 (Abcam, ab7291); and rabbit monoclonal anti-cleaved caspase 3, 1:50 (Cell Signaling, D175).
MII oocyte collection
Six- to 8-week-old and 11–12-month-old female mice (Swiss CD1, Vital River Laboratory Animal Technology Co. Ltd.) were superovulated as described previously.Citation43 Oocytes were retrieved 16 h after hCG administration with M2 medium and hyaluronidase (300 IU/mL). Animal handling was conducted in accordance with policies promulgated by the Ethics Committee of the Institute of Zoology, Chinese Academy of Sciences.
Parthenogenetic activation and embryo culture
Experiments were conducted as described previously.Citation43 Briefly, the activation medium that was used was Ca2+ free human tubal fluid (HTF) supplemented with 10 mM SrCl2. The oocytes were first incubated in activation medium for 2.5 h and then in regular HTF without SrCl2 for 3.5 h at 37°C in a humidified atmosphere with 5% CO2. The activation medium and HTF for the subsequent short culture were supplemented with 5 mg/ml cytochalasin D (ENZO, BML-T109–0001). Six hours after the onset of activation, the oocytes were removed from the medium and cultured in KSOM for 4 d. Embryos at the 2-cell and blastocyst stages were examined and recorded 24 and 96 h after the start of culturing, respectively.
Immunofluorescence and chromosome spreads
Experiments were conducted as previously reported.Citation2,44 Oocytes and blastocysts were fixed in a solution of 2% paraformaldehyde in PBS for 30 min at room temperature. Then, they were transferred to membrane permeabilization solution (0.2% Triton X-100) for 20 min. Blocking was performed in PBS with 1% BSA for 20 min. Finally, oocytes and blastocysts were incubated with primary antibodies diluted in blocking solution at 37°C for 1 h. After 3 washes with PBS, oocytes and blastocysts were labeled with secondary antibodies for 1 h at 37°C and then counterstained with 10 mg/ml 4, 6-diamidino-2-phenylindole (DAPI) for 10 min. Oocytes and blastocysts were mounted on glass slides and examined with a confocal laser scanning microscope (Zeiss LSM 780 META, Germany). For analysis of cold-stable microtubules, oocytes were exposed to ice-cold M2 medium for 10 min immediately before fixation. The entire spindle in fixed, intact oocytes was imaged at 63 × magnification every 0.5 μm using Zeiss LSM 780 and 710 microscopes. For Mad2 immunostaining, 400 nM nocodazole was added to M2 medium, or to activation medium, for 60 min. Images and protein (Rec8, Smc3, Mad2, and Crest) signals on kinetochores were processed and calculated using ImageJ and blue ZEN 2011 software. The average Mad2/Crest ratio at the centromeres without nocodazole treatment was normalized to 1 in the young MII oocytes and thereafter compared with the value with nocodazole.
For chromosome spreads, oocytes were mounted on glass slides and fixed in a solution of 1% paraformaldehyde in distilled H2O (pH 9.2) containing 0.15% Triton X-100 and 3 mM DTT after the zona pellucida was removed by a brief exposure to acidic Tyrode's solution. The slides were left to dry and then blocked with 1% BSA in PBS for 1 h at room temperature. The slides were incubated with primary antibodies diluted in blocking solution at 37°C for 1 h. After brief washes with PBS containing 0.03% BSA and 0.01% Triton X-100, the slides were then incubated with secondary antibody for 1 h at 37°C. Following DNA staining with DAPI, the slides were mounted on glass coverslips and examined with a laser scanning confocal microscope (Zeiss LSM 780 META, Germany).
Anaphase II imaging
For anaphase II imaging, superovulated MII oocytes were transferred to M2 medium supplemented with Hoechst 33342 (10 ng/ml) for 15 min and then were activated and cultured in individual 10 µl drops of activation medium prepared in a sterilized dish. Time-lapse imaging of oocytes undergoing the second meiotic division was performed through the PerkinElmer Ultra VIEW VOX Confocal Imaging System (PerkinElmer) equipped with a 37°C incubator and 5% CO2 supply. Images were captured every 3 or 5 min with a z-resolution of 2.0 μm at 20 × magnification. The tracking lasted for 8 h. The DNA in oocytes was labeled in blue using Hoechst 33342 and was changed into red in the figures and movies. Images were analyzed and treated using ImageJ software.
Statistical analysis
All experiments were repeated at least 3 times. Dichotomous data were analyzed using a chi-square test. Other data in the present study were analyzed using the Student's t-test in SPSS (Statistical Package for the Social Sciences) 19.0 software (SPSS, Inc., Chicago, IL, USA). P < 0.05 and P < 0.01 values were considered statistically significant.
Disclosure of potential conflicts of interest
The authors report no conflicts of interest.
Supplemental_Material.zip
Download Zip (1.7 MB)Acknowledgment
We thank Li-Juan Wang for technical support in live-cell imaging.
Funding
This work was supported by the Major Research Plan 973 Project under Grant 2012CB944702; the National Natural Science Foundation of China under Grants 31501953, 31471352, 31471400, 81270662, and 31171380; and Academician Workstation support (Shenyang, Changsha and Shan Dong).
References
- Jones KT, Lane SI. Molecular causes of aneuploidy in mammalian eggs. Development 2013; 140:3719-30; PMID:23981655; https://doi.org/10.1242/dev.090589
- Chiang T, Schultz RM, Lampson MA. Meiotic origins of maternal age-related aneuploidy. Biol Reprod 2012; 86:1-7; PMID:21957193; https://doi.org/10.1095/biolreprod.111.094367
- Holt JE, Lane SI, Jones KT. The control of meiotic maturation in mammalian oocytes. Curr Top Dev Biol 2013; 102:207-26; PMID:23287034
- Yun Y, Lane SI, Jones KT. Premature dyad separation in meiosis II is the major segregation error with maternal age in mouse oocytes. Development 2014; 141:199-208; PMID:24346700; https://doi.org/10.1242/dev.100206
- Salmon ED, Cimini D, Cameron LA, Deluca JG. Merotelic kinetochores in mammalian tissue cells. Philos Trans R Soc Lond B Biol Sci 2005; 360:553-68; PMID:15897180; https://doi.org/10.1098/rstb.2004.1610
- Cimini D, Howell B, Maddox P, Khodjakov A, Degrassi F, Salmon ED. Merotelic kinetochore orientation is a major mechanism of aneuploidy in mitotic mammalian tissue cells. J Cell Biol 2001; 153:517-27; PMID:11331303; https://doi.org/10.1083/jcb.153.3.517
- Cimini D, Moree B, Canman JC, Salmon ED. Merotelic kinetochore orientation occurs frequently during early mitosis in mammalian tissue cells and error correction is achieved by two different mechanisms. J Cell Sci 2003; 116:4213-25; PMID:12953065; https://doi.org/10.1242/jcs.00716
- Kitajima TS, Ohsugi M, Ellenberg J. Complete kinetochore tracking reveals error-prone homologous chromosome biorientation in mammalian oocytes. Cell 2011; 146:568-81; PMID:21854982; https://doi.org/10.1016/j.cell.2011.08.028 10.1016/j.cell.2011.07.031
- Shomper M, Lappa C, FitzHarris G. Kinetochore microtubule establishment is defective in oocytes from aged mice. Cell Cycle 2014; 13:1171-9; PMID:24553117; https://doi.org/10.4161/cc.28046
- Sakakibara Y, Hashimoto S, Nakaoka Y, Kouznetsova A, Hoog C, Kitajima TS. Bivalent separation into univalents precedes age-related meiosis I errors in oocytes. Nat Commun 2015; 6:7550; PMID:26130582; https://doi.org/10.1038/ncomms8550
- Lister LM, Kouznetsova A, Hyslop LA, Kalleas D, Pace SL, Barel JC, Nathan A, Floros V, Adelfalk C, Watanabe Y, et al. Age-related meiotic segregation errors in mammalian oocytes are preceded by depletion of cohesin and Sgo2. Curr Biol 2010; 20:1511-21; PMID:20817533; https://doi.org/10.1016/j.cub.2010.08.023
- Chiang T, Duncan FE, Schindler K, Schultz RM, Lampson MA. Evidence that weakened centromere cohesion is a leading cause of age-related aneuploidy in oocytes. Curr Biol 2010; 20:1522-8; PMID:20817534; https://doi.org/10.1016/j.cub.2010.06.069
- Jones KT, Lane SI. Chromosomal, metabolic, environmental, and hormonal origins of aneuploidy in mammalian oocytes. Exp Cell Res 2012; 318:1394-99; PMID:22394508; https://doi.org/10.1016/j.yexcr.2012.02.012
- Jessberger R. Age-related aneuploidy through cohesion exhaustion. EMBO Rep 2012; 13:539-46; PMID:22565322; https://doi.org/10.1038/embor.2012.54
- Nagaoka SI, Hodges CA, Albertini DF, Hunt PA. Oocyte-specific differences in cell-cycle control create an innate susceptibility to meiotic errors. Curr Biol 2011; 21:651-57; PMID:21497085; https://doi.org/10.1016/j.cub.2011.03.003
- Selesniemi K, Lee HJ, Muhlhauser A, Tilly JL. Prevention of maternal aging-associated oocyte aneuploidy and meiotic spindle defects in mice by dietary and genetic strategies. PNAS 2011; 108:12319-24; PMID:21730149; https://doi.org/10.1073/pnas.1018793108
- Duncan FE, Hornick JE, Lampson MA, Schultz RM, Shea LD, Woodruff TK. Chromosome cohesion decreases in human eggs with advanced maternal age. Aging Cell 2012; 11:1121-24; PMID:22823533; https://doi.org/10.1111/j.1474-9726.2012.00866.x
- Tachibana-Konwalski K, Godwin J, van der Weyden L, Champion L, Kudo NR, Adams DJ, Nasmyth K. Rec8-containing cohesin maintains bivalents without turnover during the growing phase of mouse oocytes. Genes Dev 2010; 24:2505-16; PMID:20971813; https://doi.org/10.1101/gad.605910
- Lee J, Kitajima TS, Tanno Y, Yoshida K, Morita T, Miyano T, Miyake M, Watanabe Y. Unified mode of centromeric protection by shugoshin in mammalian oocytes and somatic cells. Nat Cell Biol 2008; 10:42-52; PMID:18084284; https://doi.org/10.1038/ncb1667
- Liu L, Keefe DL. Defective cohesin is associated with age-dependent misaligned chromosomes in oocytes. Reprod BioMed Online 2008; 1:103-12; https://doi.org/10.1016/S1472-6483(10)60562-7
- Watanabe Y. Geometry and force behind kinetochore orientation: lessons from meiosis. Nat Rev Mol Cell Biol 2012; 13:370-82; PMID:22588367; https://doi.org/10.1038/nrm3349
- Revenkova E, Eijpe M, Heyting C, Gross B, Jessberger R. Novel meiosis-specific isoform of mammalian SMC1. Mol Cell Biol 2001; 21:6984-98; PMID:11564881; https://doi.org/10.1128/MCB.21.20.6984-6998.2001
- Watanabe Y, Nurse P. Cohesin Rec8 is required for reductional chromosome segregation at meiosis. Nature 1999; 400:461-4; PMID:10440376; https://doi.org/10.1038/22774
- Brust-Mascher I, Civelekoglu-Scholey G, Kwon M, Mogilner A, Scholey JM. Model for anaphase B: role of three mitotic motors in a switch from poleward flux to spindle elongation. PNAS 2004; 101:15938-43; PMID:15522967; https://doi.org/10.1073/pnas.0407044101
- Cimini D, CameronLA Salmon ED. Anaphase spindle mechanics prevent mis-segregation of merotelically oriented chromosomes. Curr Biol 2004; 14:2149-55; PMID:15589159; https://doi.org/10.1016/j.cub.2004.11.029
- Scholey JM, Brust-Mascher I, Mogilner A. Cell division. Nature 2003; 422:746-52; PMID:12700768; https://doi.org/10.1038/nature01599
- Etemad B, Kops GJ. Attachment issues: kinetochore transformations and spindle checkpoint silencing. Curr Opin Cell Biol 2016; 39:101-8; PMID:26947988; https://doi.org/10.1016/j.ceb.2016.02.016
- Kallio M, Eriksson JE, Gorbsky GJ. Differences in spindle association of the mitotic checkpoint protein Mad2 in mammalian spermatogenesis and oogenesis. Dev Biol 2000; 225:112-23; PMID:10964468; https://doi.org/10.1006/dbio.2000.9818
- Pezzi N, Prieto I, Kremer L, Perez JLA, Valero C, Mazo JD, Martinez-a C, Barbero JL. STAG3, a novel gene encoding a protein involved in meiotic chromosome pairing and location of STAG3-related genes flanking the WilliamsBeuren syndrome deletion. FASEB J 2000; 14:581-92; PMID:10698974
- Subramanian VV, Bickel SE. Aging predisposes oocytes to meiotic nondisjunction when the cohesin subunit SMC1 is reduced. PLoS Genet 2008; 4:e1000263; PMID:19008956; https://doi.org/10.1371/journal.pgen.1000263
- Hodges CA, Revenkova E, Jessberger R, Hassold TJ, Hunt PA. SMC1β-deficient female mice provide evidence that cohesins are a missing link in age-related nondisjunction. Nat Genet 2005; 37:1351-55; PMID:16258540; https://doi.org/10.1038/ng1672
- Sakuno T, Tada K, Watanabe Y. Kinetochore geometry defined by cohesion within the centromere. Nature 2009; 458:852-58; PMID:19370027; https://doi.org/10.1038/nature07876
- Handyside AH, Montag M, Magli MC, Repping S, Harper J, Schmutzler A, Vesela K, Gianaroli L, Geraedts J. Multiple meiotic errors caused by predivision of chromatids in women of advanced maternal age undergoing in vitro fertilisation. Eur J Hum Genet 2012; 20:742-7; PMID:22317970; https://doi.org/10.1038/ejhg.2011.272
- Angell R. First-meiotic-division nondisjunction in human oocytes. Am J Hum Genet 1997; 61:23-32; PMID:9245981; https://doi.org/10.1086/513890
- Gregan J, Polakova S, Zhang LJ, Tolic´-Nørrelykke IM, Cimini D. Merotelic kinetochore attachment: causes and effects. Trends Cell Biol 2011; 21:374-81; PMID:21306900; https://doi.org/10.1016/j.tcb.2011.01.003
- Kouznetsova A, Hernandez-Hernandez A, Hoog C. Merotelic attachments allow alignment and stabilization of chromatids in meiosis II oocytes. Nat Commun 2014; 5:4409; PMID:25007239; https://doi.org/10.1038/ncomms5409
- Thompson SL, Compton DA. Chromosome missegregation in human cells arises through specific types of kinetochore–microtubule attachment errors. PNAS 2011; 108:17974-78; PMID:21997207; https://doi.org/10.1073/pnas.1109720108
- Hayden JH, Bowser SS, Rieder CL. Kinetochores capture astral microtubules during chromosome attachment to the mitotic spindle: direct visualization in live newt lung cells. J Cell Biol 1990; 111:1039-45; PMID:2391359; https://doi.org/10.1083/jcb.111.3.1039
- Mitchison T, Kirschner M. Dynamic instability of microtubule growth. Nature 1984; 312:237-42; PMID:6504138; https://doi.org/10.1038/312237a0
- Rieder Cl, Salmon ED. The vertebrate cell kinetochore and its roles during mitosis. Trends Cell Biol 1988; 8:310-8
- Shah JV, Cleveland DW. Waiting for Anaphase: Mad2 and the Spindle Assembly Checkpoint. Cell 2000; 7:997-1000; https://doi.org/10.1016/S0092-8674(00)00202-6
- Thompson SL, Compton DA. Examining the link between chromosomal instability and aneuploidy in human cells. J Cell Biol 2008; 180:665-72; PMID:18283116; https://doi.org/10.1083/jcb.200712029
- Fu XW, Cheng JM, Hou YP, Zhu SE. The association between the oocyte pool and aneuploidy: a comparative study of the reproductive potential of young and aged mice. J Assist Reprod Genet 2014; 31:323-31; PMID:24362910; https://doi.org/10.1007/s10815-013-0160-5
- Cheng JM, Li J, Tang JX, Cheng SR, Deng SL, Jin C, Zhang Y, Wang XX, Zhou CX, Liu YX. Elevated intracellular pH appears in aged oocytes and causes oocyte aneuploidy associated with the loss of cohesion in mice. Cell Cycle 2016; 18:2454-63; https://doi.org/10.1080/15384101.2016.1201255