KEYWORDS:
Lipid-based membranes are self-assembling, multicomponent molecular layers that surround cells and organelles. They are made up of a variety of distinct lipids and transmembrane proteins, and also associate peripherally with proteins such as scaffolds that sense, induce or stabilize membrane-curvature, and/or molecular motors and cytoskeletal proteins that exert forces. Protein-induced dynamic changes in membrane curvature are essential for processes ranging from cell motility and cytokinesis, to neuroplasticity and intracellular trafficking.Citation1 Accordingly, defects in membrane remodeling are associated with many human pathologies including neuromuscular and autoimmune diseases and cancers.Citation2,3 Understanding how the cooperative contributions of multiple molecular components drive membrane remodeling is therefore a fundamental challenge in biology. Most studies aimed at addressing this challenge use reductionist model systems such as model membranes, cell culture, or cultured organs. While these approaches provide important insights, they do not faithfully recapitulate the complex environments and machineries required for membrane remodeling in live multicellular organisms (in vivo), and are thus limited in the extent of their physiological translatability.
To visualize and characterize membrane remodeling in vivo, we developed intravital subcellular microscopy (ISMic), a light microscopy-based technique tailored to image intracellular organelles in live rodents.Citation4,5 As our model system to study membrane dynamics we used the process of regulated exocytosis in mouse salivary glands. During this process (), large (∼1.5 µm) membrane-bound salivary granules (SGs) containing proteinous cargoes fuse with the canalicular-apical plasma membrane (APM) of salivary acinar cells upon GPCR stimulation. The consequent opening of the fusion pore then allows release of the granular contents into the canaliculi, and the SG membrane slowly integrates into the APM over ∼60 seconds. Since the diameter of acinar canaliculi is in the range of 0.3 µm, almost an order of magnitude smaller than SG diameter, the complete integration of the SG membrane into the APM is energetically unfavorable, raising a number of questions: What external mechanical forces drive the integration of SG membranes into the APM? What proteins supply and regulate these forces? And what is the spatiotemporal order in which these force-generating proteins are recruited?
Figure 1. Model proposing how NMII is recruited to fused secretory granules (SGs), and the distinct role of each isoform in driving integration of the SG membrane (diameter 1.5 μm) into the canalicular membrane (diameter 0.3 μm): 1) Before fusion, SGs containing cargo proteins reside in the vicinity of acinar canaliculi, which are coated in F-actin and NMII; 2) After stimulation, F-actin and NMII clear from the canaliculus, allowing the SG to fuse. The fused SG is then rapidly decorated with F-actin and NMII; 3) An NMII receptor localizes to the fused SG and recruits NMIIA and NMIIB; 4) NMIIB is required for initial integration of the fused SG, likely cross-linking F-actin and generating force, via slow contractions, to push the SG membrane towards the canalicus; 5) NMIIA is required to complete the integration process, continuing to push the SG membrane towards the canaliculs via fast contractions, and also maintaining the fusion pore open; 6) SG integration is completed within 60s, and the canaliculus is once again decorated with F-actin and NMII.
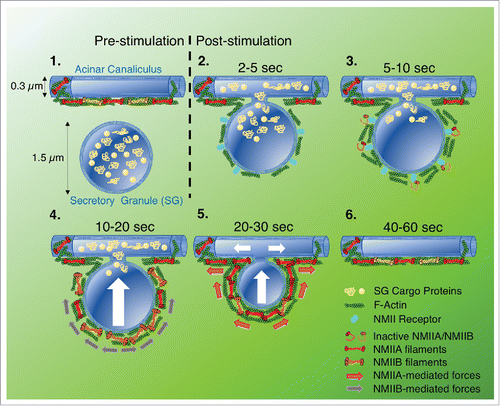
Investigating the role of the cytoskeleton in SG integration in vivo, we previously showed that pharmacological inhibition of either F-actin assembly or non-muscle myosin II (NMII) motor activity impeded integrationCitation6, indicating that the acto-myosin cytoskeleton provides the driving force required to complete this process. More recently,Citation7 using new mouse models, we sought to further advance the molecular characterization of SG integration by investigating: 1) the specific role(s) played by distinct NMII isoforms, and 2) the mechanisms of their recruitment and activation. Using mice expressing fluorescently-tagged NMII isoforms, we first determined that two isoforms of NMII, namely NMIIA and NMIIB, localize to fused SGs. Next, using ISMic in live mice expressing a reporter for F-actin (LifeAct) in addition to either fluorescently-tagged NMII isoform, we discovered that both isoforms appear on fused SGs seconds after F-actin. While this initially suggested that F-actin may be recruiting NMII to the SG surface, we were surprised to find that pharmacological disruption of F-actin assembly did not affect the localization of either NMIIA or NMIIB to fused SGs. This finding introduces the fascinating prospect of a yet-to-be-identified, actin-independent mode of NMII recruitment ().
To examine the specific roles of NMIIA and NMIIB in the integration process in acinar cells, we used transgenic mice in which genes encoding either NMIIA or NMIIB or both NMIIA/NMIIB were floxed, and knockdown was achieved by in vivo transfection of Cre-recombinase. Analyzing the kinetics of granule integration in each knockdown we found that: 1) In the absence of NMIIA, the kinetics of initial integration remained unaffected until SGs reached 50–60% of their initial diameter, at which point integration was stalled; 2) In NMIIB knockdown cells, SGs underwent an initial increase in diameter, followed by a delayed (but complete) integration; and 3) ablation of both NMIIA and NMIIB blocked integration in all but a small percentage of SGs, raising the possibility of an additional, myosin-independent mechanism. Based on these data, we propose the following model where NMIIA and NMIIB play separate roles during the integration process, consistent with their different enzymatic properties: First NMIIB stabilizes the membranes of fused SGs and, through slow contractions and cross-linking, drives the initial process of integration. NMIIA is then needed to complete the integration process, possibly through faster contractions and by regulating the progressive maintenance and expansion of the fusion pore ().
Exciting new questions have arisen from these results that will now need to be addressed to validate our model: How are NMIIA, NMIIB and F-actin recruited to the membrane of fused granules? What is their specific organization on the curved membrane surface that enables them to generate distinct forces during granule integration? And what other molecular players are involved in the assembly, dynamics and disassembly of this sophisticated cytoskeletal machinery? These studies also highlight the importance of ISMic as a powerful new approach to dissect essential physiological molecular machineries in vivo, and a paradigm for future studies that will continue to be bolstered by technological improvements and the development of assays for in vivo biophysical measurements.
Disclosure of potential conflicts of interest
No potential conflicts of interest were disclosed.
References
- McMahon HT, Gallop JL. Membrane curvature and mechanisms of dynamic cell membrane remodelling. Nature. 2005;438:590-6. doi:10.1038/nature04396. PMID:16319878
- Cowling BS, Toussaint A, Muller J, Laporte J. Defective membrane remodeling in neuromuscular diseases: insights from animal models. PLoS Genet. 2012;8:e1002595. doi:10.1371/journal.pgen.1002595. PMID:22496665
- Rohrig F, Schulze A. The multifaceted roles of fatty acid synthesis in cancer. Nat Rev Cancer. 2016;16:732-49. doi:10.1038/nrc.2016.89. PMID:27658529
- Amornphimoltham P, Masedunskas A, Weigert R. Intravital microscopy as a tool to study drug delivery in preclinical studies. Adv Drug Deliv Rev. 2011;63:119-28. doi:10.1016/j.addr.2010.09.009. PMID:20933026
- Weigert R, Porat-Shliom N, Amornphimoltham P. Imaging cell biology in live animals: ready for prime time. J Cell Biol. 2013;201:969-79. doi:10.1083/jcb.201212130. PMID:23798727
- Masedunskas A, Sramkova M, Parente L, Sales KU, Amornphimoltham P, Bugge TH, Weigert R. Role for the actomyosin complex in regulated exocytosis revealed by intravital microscopy. Proc Natl Acad Sci U S A. 2011;108: 13552-7. doi:10.1073/pnas.1016778108. PMID:21808006
- Milberg O, Shitara A, Ebrahim S, Masedunskas A, Tora M, Tran DT, Chen Y, Conti MA, Adelstein RS, Ten Hagen KG, Weigert R. Concerted actions of distinct nonmuscle myosin II isoforms drive intracellular membrane remodeling in live animals. J Cell Biol. 2017;216:1925-36. doi:10.1083/jcb.201612126. PMID:28600434