KEYWORDS:
In 1954, Dr. David Bloom reported three cases of a developmental disorder involving short height and a characteristic rash, which he suggested were caused by a common genetic disorder. As further cases of ‘Bloom Syndrome’ were identified, it became clear that the patient cells had high levels of genomic instability, leading to a high frequency of cancer in Bloom Syndrome patients. These symptoms were subsequently shown to be caused by homozygous mutations affecting the BLM gene [Citation1]. BLM encodes a helicase that can be detected in basically all human tissues, but how does it protect against genomic instability and cancer? Our recent study (Patel et. al.) published in the Journal of Cell Biology [Citation2] offers a new perspective on this question.
Cells from Bloom Syndrome patients show very high rates of exchange of genetic material between sister chromatids. These exchanges take place during repair of DNA damage by the homologous recombination (HR) pathway. During HR, damaged DNA is repaired using a homologous sequence (typically a sister chromatid) as a template. At the final stages of HR, a complex of BLM, Topoisomerase III-alpha and RMI1/2 helps separate the template DNA strand from the repaired DNA [Citation3]. When BLM is not present, the HR products are instead processed by ‘resolvase’ enzymes, which produce a higher rate of inter-chromatid exchanges. This activity of BLM in dissolving the products of HR has long been considered to be its key contribution toward maintaining genomic integrity ().
Figure 1. BLM promotes (a) initial ‘resection’ of DNA double-strand breaks during homologous recombination (HR), but, (b) as reinforced by recent data, also destabilizes RAD51 at resected DNA breaks. This inhibitory effect of BLM may help ensure recombination only takes place between highly-homologous sequences. BLM also acts to (c) disassemble ‘D-loops’ formed after pairing of homologous sequences, and (d) in ‘dissolution’ of HR intermediates.
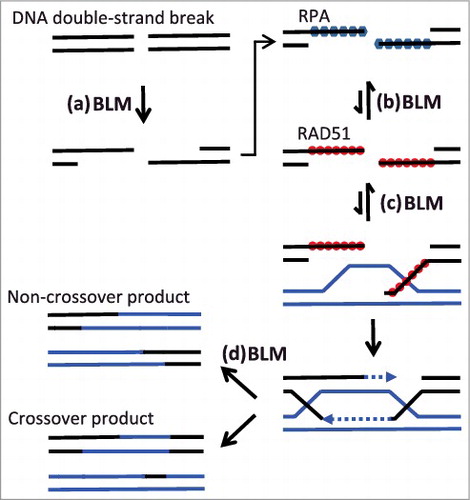
BLM is involved at several other steps of HR and DNA replication, however. BLM acts with the endonuclease Dna2 during the ‘resection’ phase at the initiation of HR, when single-stranded DNA is formed. Biochemical evidence also indicates that BLM displaces RAD51 from single-stranded DNA overhangs formed after resection. BLM furthermore acts to displace RAD51-loaded DNA from ‘D-loops’ formed by pairing of homologous DNA sequences. Any of these activities might contribute to the genomic instability seen in Bloom Syndrome cells when BLM is mutated.
Our study used knockout mice to test the importance of BLM in resection and recruitment of RAD51, a key HR factor, to DNA break sites. We observed that cells lacking the tumor suppressor, BRCA1, show a defect in RAD51 recruitment, which causes an HR defect in BRCA1-deficient cells. When BLM is also deleted from BRCA1-deficient cells, RAD51 foci form at normal levels. BLM therefore prevents the stable assembly of RAD51 at DNA breaks in BRCA1-deficient cells. We further found that deletion of BLM rescues RAD51 recruitment in other HR mutants, and that overexpression of BLM can disrupt RAD51 loading in WT cells. These results confirm that the ability of BLM to displace RAD51, which was previously observed using biochemical substrates in vitro [Citation4], is also relevant in cells.
BLM therefore has more than one ‘anti-recombinase’ activity. It prevents genetic exchanges arising at the final stages of HR, but also prevents recombination by destabilizing RAD51 on resected DNA double-strand breaks. Mammalian cells seem to express a number of factors that are responsible for reducing or altering the efficiency of HR. The chromatin factor, 53BP1, limits DNA break resection [Citation5], and a growing list of mammalian ‘anti-recombinases’ include RTEL, FBH1, RECQ5, PARI and WRN, are also known to limit HR [Citation6]. Some of these factors act by removing RAD51 from DNA, whereas others act by disassembling D-loops.
At this time we don't yet have a clear insight into how exactly BLM reduces RAD51 association with resected DNA. Destabilization of RAD51 requires the helicase activity of BLM, but does not appear to require interaction with its partners, Topoisomerase III-alpha or RMI1/2. In general, it isn't clear how mammalian anti-recombinases work, or why so many proteins with anti-recombinase activity are expressed. Do these factors work redundantly, or do some of them have specific functions in different cell types? Is it possible to selectively kill cancer cells by targeting potential differences in ‘anti-recombinase’ activity? And why do cells aim to control HR so tightly? One potential answer to this last question is that HR may sometimes cause mutations and chromosome translocations if it takes place between DNA sequences that are not fully homologous. By limiting the amount of RAD51 loaded onto resected DNA breaks, BLM may ensure that stable pairing only occurs between sequences with extensive homology. The yeast ortholog of BLM, Sgs1, has been shown to maintain the fidelity of RAD51-mediated pairing by suppressing the association of moderately-diverged DNA sequences [Citation7].
Clearly, getting complete answers to these questions will require further comparison and characterization of mammalian anti-recombinases. Future work should also aim to further dissect the importance of BLM activity at different stages of HR. By separating the function of BLM in different steps of HR, it may be possible to better understand the causes of genomic instability in Bloom Syndrome patients, holding out hope that it may be possible to reduce their symptoms by rebalancing cellular repair activities.
Disclosure of potential conflicts of interest
No potential conflicts of interest were disclosed.
Additional information
Funding
References
- Larsen NB, Hickson ID. RecQ helicases: conserved guardians of genomic integrity. Adv Exp Med Biol. 2013;767:161–184. doi:10.1007/978-1-4614-5037-5_8. PMID:23161011
- Patel DS, Misenko SM, Her J, Bunting SF. BLM helicase regulates DNA repair by counteracting RAD51 loading at DNA double-strand break sites. J Cell Biol. 2017;216:3521–3534. doi:10.1083/jcb.201703144. PMID:28912125
- Wu L, Hickson ID. The Bloom's syndrome helicase suppresses crossing over during homologous recombination. Nature. 2003;426:870–874. doi:10.1038/nature02253. PMID:14685245
- Bugreev DV, Yu X, Egelman EH, Mazin AV. Novel pro- and anti-recombination activities of the Bloom's syndrome helicase. Genes Development. 2007;21:3085–3094. doi:10.1101/gad.1609007. PMID:18003860
- Bunting SF, Callen E, Wong N, Chen HT, Polato F, Gunn A, et al. 53BP1 inhibits homologous recombination in Brca1-deficient cells by blocking resection of DNA breaks. Cell. 2010;141:243–254. doi:10.1016/j.cell.2010.03.012. PMID:20362325
- Krejci L, Van Komen S, Li Y, Villemain J, Reddy MS, Klein H, et al. DNA helicase Srs2 disrupts the Rad51 presynaptic filament. Nature. 2003;423:305–309. doi:10.1038/nature01577. PMID:12748644
- Sugawara N, Goldfarb T, Studamire B, Alani E, Haber JE. Heteroduplex rejection during single-strand annealing requires Sgs1 helicase and mismatch repair proteins Msh2 and Msh6 but not Pms1. Proc Nat Acad Sci United States Am. 2004;101:9315–9320. doi:10.1073/pnas.0305749101. PMID:15199178