ABSTRACT
Oocyte is arrested at metaphase of the second meiosis until fertilization switching on [Ca2+]i oscillations. Oocyte activation inefficiency is the most challenging problem for failed fertilization and embryonic development. Mitochondrial function and intracellular [Ca2+]i oscillations are two critical factors for the oocyte’s developmental potential. We aimed to understand the possible correlation between mitochondrial function and [Ca2+]i oscillations in oocytes. To this end, mitochondrial uncoupler CCCP which damages mitochondrial function and two small molecule mitochondrial agonists, L-carnitine (LC) and BGP-15, were used to examine the regulation of [Ca2+]i by mitochondrial functions. With increasing CCCP concentrations, [Ca2+]i oscillations were gradually diminished and high concentrations of CCCP led to oocyte death. LC enhanced mitochondrial membrane potential and [Ca2+]i oscillations and even improved the damage induced by CCCP, however, BGP-15 had no beneficial effect on oocyte activation. We have found that mitochondrial function plays a vital role in the generation of [Ca2+]i oscillations in oocytes, and thus mitochondria may interact with the ER to generate [Ca2+]i oscillations during oocyte activation. Improvement of mitochondrial functions with small molecules can be expected to improve oocyte activation and embryonic development in infertile patients without invasive micromanipulation.
Introduction
At least 15% of couples in developed countries cannot have children within five years of marriage (data according to World Health Organization 2016), half of which are due to female infertility [Citation1]. Oocyte activation inefficiency is a major problem causing female infertility [Citation2]. Directly following sperm penetration, oocyte activation begins with a series crucial steps by periodical repetitive increases and decreases of intracellular Ca2+ ([Ca2+]i) concentrations also known as [Ca2+]i oscillations [Citation3,Citation4]. Although [Ca2+]i oscillations are required for oocyte activation [Citation5] and a hallmark of mammalian fertilization events, which include resumption of meiosis [Citation6], male chromatin decondensation and pronuclear formation [Citation7], as well as subsequent development [Citation5], we presently have only limited knowledge on how [Ca2+]i oscillations are regulated.
Mitochondria are vitally important organelles in oocytes; and they give rise to the entire mitochondria population in the offspring organs, and their transmission, replication and inheritance are tightly regulated [Citation2]. Mitochondrial damage in oocytes caused by obesity, diabetes and aging reduces a woman’s reproductive capacity [Citation8]. In addition to the important roles of endoplasmic reticulum as Ca2+ stores, mitochondria also take up cytosolic [Ca2+]i in the endoplasmic reticulum-mitochondria Ca2+ transfer process [Citation9], and thereby may contribute to influence the patterns of [Ca2+]i responses, while simultaneously promoting ATP regulation [Citation10]. Mitochondria play an important role in replenishing Ca2+ in the cytoplasm and the endoplasmic reticulum. Here, we set out to find out whether [Ca2+]i oscillations are affected by mitochondrial functions in mouse oocytes. We tested the effects of several mitochondrial modulators on [Ca2+]i oscillations in mouse oocytes. CCCP, a mitochondrial potential uncoupling agent [Citation11], was added to the cell culture medium to reduce the mitochondrial potential and terminal oxidative phosphorylation (OXPHOS) for ATP production [Citation12]. Mitochondria and lipids are crucial for energy production and supplementation of energy resources by OXPHOS [Citation13]. L-carnitine (LC) participates in the oxidation of fatty acids by transporting acyl-COA from the cytoplasm to the mitochondrial matrix with the help of carnitine acyltransferases, where β-oxidation and OXPHOS occur [Citation14]. LC can effectively enhance the mitochondrial metabolic efficiency of fatty acids and thus increase intracellular mitochondrial function. Another agonist BGP-15 (salubrinal) is a hydroximic acid derivative that is a potent inducer of the chaperone HSP72 in muscle [Citation15], which increases sarcoplasmic and/or endoplasmic reticulum Ca2+-ATPase (SERCA) activity [Citation16] and protects against obesity-induced insulin resistance of type II diabetes [Citation17]. BGP-15 reverses mitochondrial depolarization, restoring mitochondrial polarization in a liver disease model [Citation18]. In female reproduction, BGP-15 is able to improve mitochondrial dysfunctions in obesity-affected mouse oocytes and improves embryo and fetal development by intraperitoneal injection [Citation19], but its direct effects on oocytes is not yet known.
The transmission of Ca2+ between ER and mitochondria is strictly regulated, but the understanding of the effect of mitochondrial metabolism on intracellular calcium oscillations is not well understood in oocyte. By using mitochondrial modulators and their combinations as well as time-lapse recording of [Ca2+]i changes, we found that mitochondrial function is important for generating [Ca2+]i oscillations during mouse oocyte activation.
Results
Mitochondrial membrane potential in CCCP-treated oocytes after parthenogenetic activation
Ovulated MII oocytes were obtained and parthenogenetically activated in Ca2+-free SrCl2-CZB medium or kept in M2 medium for 4 h to observe the death rate. CCCP change the permeability of mitochondrial inner membrane and remove mitochondrial membrane potential. Oocyte death rate was judged by oocyte membrane rupture. If oocytes activation at the same time of mitochondrial membrane potential disappeared, oocyte death is accelerated. The results showed that oocytes died with increasing CCCP concentrations in both activated and non-activated oocytes. No significant difference was observed between the 0.1μM group and 0μM control group, but the death rate was significantly increased when treated with more than 1μM CCCP (). The mitochondrial membrane potential probe JC-1 was used to detect mitochondrial activity at different concentrations of CCCP: 0μM, 0.1μM and 1μM CCCP (). The high mitochondrial membrane potential (red) of parthenogenetic eggs began to decrease with 0.1μM CCCP treatment and was significantly reduced when the CCCP concentration was 1μM ().
Figure 1. Effect of CCCP gradient concentrations in M2 medium and Ca2+-free SrCl2-CZB medium on oocyte survival. With the increase of CCCP concentrations, the death rate increased gradually in both MII oocytes and parthenogenetic oocytes. Parthenogenetic oocytes had higher death rates (marked with *) than MII oocytes keep in M2 medium. * indicated significant difference (P < 0.05) .
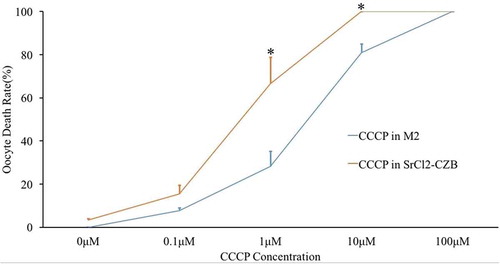
Figure 2. Mitochondrial potential of CCCP-treated oocytes after parthenogenetic activation. (a) Parthenogenetic oocytes stained with JC-1, a potential-sensitive fluorescent mitochondrial dye, which emits red fluorescence in higher membrane potential mitochondria and emits green fluorescence in lower potential mitochondria. Bar indicated 50μm in panel A. (b) Relative fluorescence intensity was compared with that of the 0μM CCCP group. With the increase of CCCP concentrations, high potential mitochondria (red) decreased significantly. * indicated significant difference (P < 0.05) compared with the CTRL group.
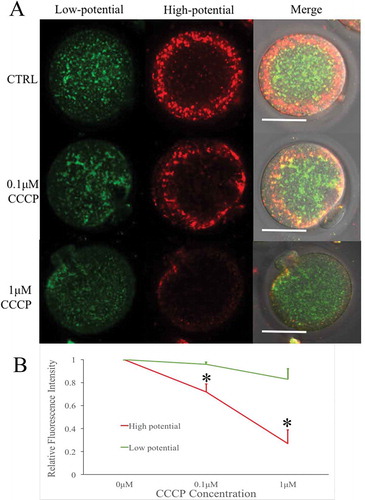
[Ca2+]i oscillation patterns of CCCP-treated oocytes after parthenogenetic activation
Once sperm penetrates into the oocyte, [Ca2+]i oscillations are initiated. In our study, we adopted the 0μM group as a control group, and concentrations of 0.1μM, 1μM, 10μM and 100μM CCCP were respectively added to the parthenogenetic activation medium to observe the effect of CCCP on [Ca2+]i oscillations. The results showed that 0.1μM CCCP could activate oocytes in controls, however, 1μM CCCP severely affected parameters of [Ca2+]i oscillations, including elongated cycles (). Treatment with more than 10μM CCCP resulted in disappearance of [Ca2+]i oscillations (A: 0μM CTRL and B: 10μM CCCP groups as examples shown in Supplemental Video). The leakage of [Ca2+]i from ER induced an increase of [Ca2+]i and cell swelling to death (Supplemental Video).
Effect of L-carnitine and BGP-15 on oocyte mitochondrial membrane potential after parthenogenetic activation
Previous studies have found that both L-carnitine (LC) and BGP-15 enhance mitochondrial function in some disease models. But their function in female gametes have not been known until now. Oocytes were treated with LC (0.75 mg/ml) [Citation20] and BGP-15 (30 μM) [Citation21] after parthenogenetic activation by Ca2+-free SrCl2-CZB medium () to observe their effects on mitochondrial membrane potential. LC enhanced mitochondrial membrane potential, while BGP-15 had no significant improving effect.
Enhancement of mitochondrial effect on [Ca2+]i oscillations
Two mitochondrial agonists, LC and BGP-15 were added to SrCl2-CZB to observe the effects of mitochondrial function on the first 10 Mitochondrial membrane potential oscillations (). When LC was added, the first 10 [Ca2+]i oscillations showed significantly faster frequency, shorter cycle, and the maintaining of the [Ca2+]i rise period became longer (). However, after BGP-15 treatment, [Ca2+]i oscillations ceased earlier and the peak level decreased. The mitochondrial enhancement effect induced by BGP-15 could only make [Ca2+]i rise faster in the short term, but failed to maintain long-term high-intensity Ca2+ oscillations.
Figure 3. [Ca2+]i oscillation patterns after parthenogenetic activation of oocytes treated with CCCP. (a) [Ca2+]i oscillation patterns were influenced by gradually increasing CCCP concentrations. 0μM group as a control group, and 0.1μM, 1μM, 10μM and 100μM CCCP were respectively added into the parthenogenetic activation medium to observe the effect of CCCP on [Ca2+]i oscillations. (b) [Ca2+]i oscillation parameters in oocytes treated with 0.1μM and 1μM CCCP. With CCCP concentrations increasing from 0μM to 1μM, [Ca2+]i oscillations became gradually diminished. Once the CCCP concentration reached 10μM, there was only a slow increase in [Ca2+]i and no [Ca2+]i oscillations were observed. * indicated significant difference (P < 0.05) .
![Figure 3. [Ca2+]i oscillation patterns after parthenogenetic activation of oocytes treated with CCCP. (a) [Ca2+]i oscillation patterns were influenced by gradually increasing CCCP concentrations. 0μM group as a control group, and 0.1μM, 1μM, 10μM and 100μM CCCP were respectively added into the parthenogenetic activation medium to observe the effect of CCCP on [Ca2+]i oscillations. (b) [Ca2+]i oscillation parameters in oocytes treated with 0.1μM and 1μM CCCP. With CCCP concentrations increasing from 0μM to 1μM, [Ca2+]i oscillations became gradually diminished. Once the CCCP concentration reached 10μM, there was only a slow increase in [Ca2+]i and no [Ca2+]i oscillations were observed. * indicated significant difference (P < 0.05) .](/cms/asset/c3c8ea61-2c0f-465f-bbf2-0b7c97fe5a85/kccy_a_1489179_f0003_oc.jpg)
Figure 4. Effect of L-carnitine and BGP-15 on mitochondrial membrane potential after parthenogenetic activation. (a) Mitochondrial membrane potential after parthenogenetic activation. Bar indicated 50μm in panel A. (b) Relative fluorescence intensity analysis of mitochondrial membrane potential. LC could increase mitochondrial membrane potential (red) intensity. BGP-15 had no significant improvement effect. Red fluorescence indicated mitochondria with higher membrane potential and green fluorescence indicated lower potential mitochondria.* indicated significant difference (P < 0.05) compared to the CTRL group.
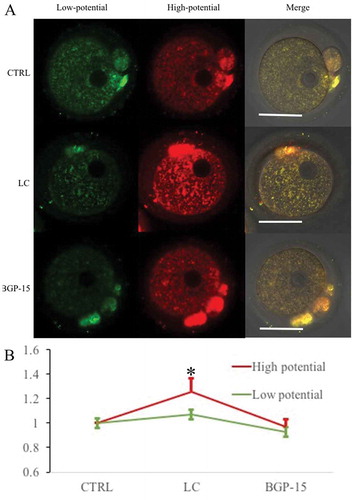
Figure 5. L-carnitine (LC) and BGP-15 affected [Ca2+]i oscillation in parthenogenetic oocytes. (a) Influence of 0.75 mg/ml LC and 30μM BGP-15 on [Ca2+]i oscillation patterns. (b) Relative [Ca2+]i oscillation parameters. [Ca2+]i oscillations of LC-treated oocytes showed significantly faster frequency, shorter cycle, a longer maintaining period and a shorter fluorescence rest. However, after earlier similar patterns, [Ca2+]i oscillations induced by BGP-15 treatment ceased and the peak decreased. * indicated significant difference (P < 0.05) .
![Figure 5. L-carnitine (LC) and BGP-15 affected [Ca2+]i oscillation in parthenogenetic oocytes. (a) Influence of 0.75 mg/ml LC and 30μM BGP-15 on [Ca2+]i oscillation patterns. (b) Relative [Ca2+]i oscillation parameters. [Ca2+]i oscillations of LC-treated oocytes showed significantly faster frequency, shorter cycle, a longer maintaining period and a shorter fluorescence rest. However, after earlier similar patterns, [Ca2+]i oscillations induced by BGP-15 treatment ceased and the peak decreased. * indicated significant difference (P < 0.05) .](/cms/asset/53466b7a-3f09-427f-88c8-7f71dc811ef9/kccy_a_1489179_f0005_oc.jpg)
Mitochondrial membrane potential decrease induced by CCCP is improved by LC and BGP-15
Mitochondrial membrane potential was reduced after parthenogenetic activation with 0.1μM and 1μM CCCP, and it was thus clear that mitochondrial inner-membrane openning could reduce mitochondrial membrane potential () and disrupt [Ca2+]i oscillations (), leading to insufficient activation of oocytes. We next tested whether mitochondrial potential damage by CCCP could be improved by LC and BGP-15. When treated with 0.1μM CCCP treatment, mitochondria membrane potential decrease was improved by LC and BGP-15 (), but there was no significant difference in the 1μM treatment groups (Supplemental Fig. 2). It could be concluded that enhancement of mitochondrial function by LC and BGP-15 can improve the mitochondrial potential injury caused by 0.1μM CCCP treatment, but mitochondrial potential damage could not be improved when CCCP concentration reached 1μM.
Enhancement of mitochondrial membrane potential during in vitro maturation by LC
GV oocytes were cultured in the medium containing both milrinone to inhibit germinal vesicle breakdown and LC or BGP-15 for 6h. The LC treatment of GV oocytes significantly enhanced mitochondrial membrane potential when compared to the CTRL and BGP-15 groups (). Red fluorescence indicated higher membrane potential mitochondria and green fluorescence indicated lower potential mitochondria. Unexpectedly, green fluorescent staining was increased as well. After maturation of GV oocytes from different treatment groups, LC treated mature oocytes also showed stronger staining of both red and green fluorescence compared to the GV oocytes (). Thus, LC could improve GV mitochondrial function from GV to MII stages, but BGP-15 had no such effect during in vitro maturation (IVM) of oocytes.
Figure 6. Mitochondrial membrane potential damage induced by 0.1μM CCCP was improved by LC and BGP-15. (a) Fluorescent staining of mitochondrial membrane potential after different treatments. 0.1μM CTRL group: treated with 0.1μM CCCP and DMSO; 0.1μM LC group: treated with 0.1μM CCCP and 0.75 mg/ml LC; 0.1μM BGP-15 group: treated with 0.1μM CCCP and 30μM BGP-15. (b) Relative fluorescence intensity analysis. Red fluorescence indicated mitochondria with higher membrane potential and green fluorescence indicated lower potential mitochondria. * indicated significant difference (P < 0.05) compared to the 0.1μM CTRL group. Bar indicated 50μm in panel A.
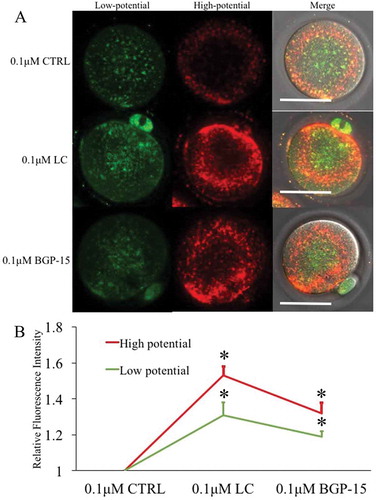
Figure 7. Effects of LC and BGP-15 on mitochondrial membrane potential during in vitro maturation of oocytes. (a) Mitochondrial membrane potential staining of GV oocytes after GVBD inhibition with milrinone. LC improved both high and low mitochondrial membrane potential staining. (b) Mitochondrial membrane potential staining of MII oocytes after IVM. (c) Relative fluorescence intensity analysis. Red fluorescence indicated mitochondria with higher membrane potential and green fluorescence indicated lower potential mitochondria.* indicated significant difference (P < 0.05) compared to CTRL group. Bar indicated 50μm in panel A.
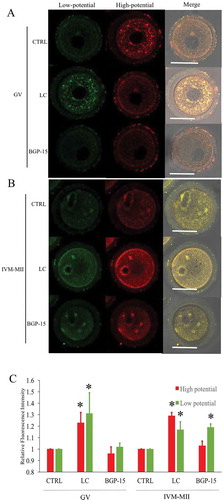
Effect of LC and BGP-15 on [Ca2+]i oscillations of IVM oocytes
We evaluated the effect of mitochondrial function on the maturation quality of oocytes by the parameters of [Ca2+]i oscillations (). LC or BGP-15 was added during in vitro maturation of oocytes. LC treatment could increase frequency and decrease cycle length of [Ca2+]i oscillations in parthenogenetic IVM-MII oocytes. However, BGP-15 significantly disrupted [Ca2+]i oscillations of IVM-MII oocytes and decreased the quality of oocyte maturation, because the cleavage rate was significantly reduced due to the increased rate of fragmentation after activation (Supplemental Table 1).
Figure 8. Influence of L-carnitine (LC) and BGP-15 on IVM oocyte oscillations. (a) [Ca2+]i oscillations in IVM-MII oocytes treated by L-carnitine and BGP-15. (b) Relative [Ca2+]i oscillation parameters after treatment with mitochondrial agonists. [Ca2+]i oscillations of LC treatment showed stronger patterns, while BGP-15 significantly reduced the quality of oocyte maturation. * indicated significant difference (P < 0.05) in comparison between the same parameter.
![Figure 8. Influence of L-carnitine (LC) and BGP-15 on IVM oocyte oscillations. (a) [Ca2+]i oscillations in IVM-MII oocytes treated by L-carnitine and BGP-15. (b) Relative [Ca2+]i oscillation parameters after treatment with mitochondrial agonists. [Ca2+]i oscillations of LC treatment showed stronger patterns, while BGP-15 significantly reduced the quality of oocyte maturation. * indicated significant difference (P < 0.05) in comparison between the same parameter.](/cms/asset/a3390214-b6c3-47a1-826b-cce1c82e9262/kccy_a_1489179_f0008_oc.jpg)
Discussion
Obese, diabetic and aging women have a low efficiency of oocyte activation, altering the development of preimplantation embryos and even pregnancy rates [Citation22–Citation26]. Such disorders are strongly related to mitochondrial damage [Citation27,Citation28]. Mitochondrial damage-caused inefficient oocyte activation is a challenge of assisted reproductive technology (ART) for infertile patients [Citation29]. In mammals, liberation from MII arrest, sperm head disconnection, pronuclear formation and initiation of the embryonic development, commonly termed oocyte activation after fertilization, is a result of a continuous series of oocyte intracellular Ca2+ concentration ([Ca2+]i) increase and decrease known as [Ca2+]i oscillations [Citation30,Citation31]. Ca2+ signaling into specific organelles, such as the nucleus for gene-regulatory events [Citation32] and the mitochondrial matrix for oxidative metabolism [Citation33] regulates organelle specific cell functions in Xenopus [Citation34]. However, excessive or prolonged [Ca2+]i increase disturbs the oscillatory patterns, and can cause fragmentation and apoptosis [Citation35,Citation36]. To prevent this, elevated [Ca2+]i is rapidly removed from the cytoplasm and replenished into the ER with high Ca2+ concentrations, which is supported by mitochondrial produced ATP [Citation10,Citation37,Citation38].
In our study, we set out to confirm the mitochondrial regulatory function in [Ca2+]i oscillation homeostasis in oocytes. Toward this end, an advanced [Ca2+]i probe Fluo-4 AM allowing to measure [Ca2+]i dynamics in live cells and time-lapse laser scanning confocal microscopy [Citation39] were employed. We measured several relative parameters of [Ca2+]i oscillations, such as the frequency, cycle, maintenance, peak and moving speed after egg activation (Supplemental Figure 1). We used uncoupler CCCP-treated oocytes as a mitochondria-damage model [Citation40,Citation41]. Consistent with a previous study [Citation42] and our data, it can be seen that higher CCCP concentrations make a higher death ratio () and a lower mitochondrial membrane potential (). We found that, with CCCP concentrations increasing from 0μM to 1μM, mitochondrial membrane potential was reduced. Correspondingly, [Ca2+]i oscillation patterns were altered and became gradually diminished. Most oocytes could survive to parthenogenetic activation. The [Ca2+]i oscillation cycle became lengthened, and Ca2+ replenishing was delayed in the 1 μM CCCP group. When increasing the concentration of CCCP from 1μM to 10μ M, Ca2+ showed a slow spill (), similar as another uncoupler FCCP function described in a previous study [Citation39], and high [Ca2+]i concentrations led to cell rupture. Once treated with more than 10μM CCCP, there was a slow increase in [Ca2+]i and no Ca2+ ER replenishing and [Ca2+]i oscillations were observed in oocytes (), and oocytes went to death within one hour; [Ca2+]i oscillations failed to occur and oocytes disintegrated (). It has been reported that after Ca2+ rise mitochondrial membrane potential was decreased, and synthesis of large quantities of ATP provided energy for oocyte activation [Citation43,Citation44]. Inactivation of mitochondrial function will influence PM Ca2+-ATPase (PMCA) [Citation45] and sarco-endoplasmic reticulum Ca2+-ATPases (SERCA), abruptly increased [Ca2+]i, induced a sustained elevation in [Ca2+]i and altered the pattern of oscillations [Citation4]. Since mitochondrial membrane potential damage caused ATP function failure, especially regarding ER replenishing, an inverse concentration [Ca2+]i movement process is needed for adequate ATP supply [Citation4]. ER Ca2+ channel regulators especially Ca2+ active transportor agonists could become a potential therapy for infertile women with in vitro fertilization failure.
Mitochondria play an important role in ATP synthesis for oocytes maturation and activation [Citation46,Citation47]. We further selected two small molecules as agonists to study mitochondria function in [Ca2+]i oscillations. LC was first isolated from bovine muscle in 1905 [Citation48]. It is well known that LC is a small, hydrophilic molecule which can promote fatty acid and energy utilization by transporting fatty acids across the inner mitochondrial membrane for β oxidation, and synthesis of ATP [Citation49]. In addition, LC protects mitochondrial potential, reduces ROS [Citation50], and avoids mitochondrial-dependent apoptosis [Citation51]. LC has wide clinical applications for various diseases, including cardiovascular diseases [Citation52], urinary diseases [Citation53], nervous system diseases [Citation54], and low sperm quality [Citation55]. It may be beneficial for improving pregnancy outcomes in assisted reproductive technologies (ART) [Citation56], especially for patients with polycystic ovary syndrome and endometriosis-affected infertile woman [Citation57]. Furthermore, an exciting report in porcine showed that LC could protect cumulus cells from apoptosis and improve mitochondrial activity during oocyte in vitro maturation [Citation58]. The other agonist in our study, hydroximic acid derivative BGP-15 (O-[3-piperidino-2-hydroxy-1-propyl]-nicotinic amidoxime) is a small (350 Da) multi-target molecule, which integrates into membranes and stabilizes their lipid rafts [Citation15], reduces the levels of reactive oxygen species by enhancing mitochondrial efficiency, and improve insulin resistance as a chaperone of Hsp72 [Citation59–Citation64]. It has been reported that more than 90% of BGP-15 accumulated in the mitochondria, which raises the possibility that BGP-15 may protect cells via mitochondrial mechanisms [Citation21,Citation61]. Treatment of obese women with BGP-15 before ovulation increased the amount of mitochondrial replication factor TFAM and mtDNA content in oocytes [Citation19].
We focused on the effects of LC and BGP-15 on mitochondrial membrane potential and [Ca2+]i oscillations in parthenogenetic oocytes, expecting that they may improve mitochondria induced energy metabolism and oocyte activation efficiency. We analyzed the effects of LC and BGP-15 on mitochondrial potential as stained by JC-1 (). After ovulated MII oocytes underwent parthenogenetic activation, LC significantly improved mitochondrial potential, while BGP-15 had no such effect. Such result divergence may be due to the fact that LC can produce more ATP, while BGP-15 increases the activity of the SERCA pumps dependent on existing ATP[Citation16]. [Ca2+]i oscillations became faster in the early activation stages in oocytes treated with LC (). Since 0.1μM and 1μM CCCP reduced mitochondrial potential significantly, we tried to improve uncoupler damaged oocytes with LC and BGP-15. Under 0.1μM CCCP conditions, both LC and BGP-15 improved mitochondrial membrane potential. Whereas, 1μM CCCP caused an unrepairable damage of mitochondrial potential ().
In the agonist LC-treated GV oocytes, both red and green fluorescence intensities were increased. Compared to ovulated MII oocytes, overall mitochondria analysis indicated that LC treatment of GV oocytes improved mitochondria membrane potential, supporting the previous findings that LC treatment during IVM oocytes improved oocyte quality and developmental potential [Citation65,Citation66]. LC-treated oocytes showed [Ca2+]i oscillations with a higher frequency, shorter cycle and faster move (); according to the dynamic changes of mitochondrial membrane potential and [Ca2+]i oscillations. From these results, we concluded that the treatment with LC significantly increased the activation efficiency of IVM oocytes.
Many patients’ infertility is due to insufficient activation of oocytes. Ca2+ modulators such as Ca2+ inophore A23187 have been used to activate oocytes in human ART clinics. Interaction between mitochondrial and ER is important for cellular Ca2+ regulation. Application of spindle transfer [Citation67] or mitochondrial injection [Citation68] is tested to improve oocyte quality, but the follow-up damage caused by oocyte manipulation raises concerns. Continuing to find a better method for improving oocyte mitochondrial function without disrupting the integrity of the oocyte will be an important approach. We found that [Ca2+]i oscillations were severely disturbed by impaired mitochondrial function, suggesting that mitochondrial function is crucial for oocyte activation. LC could improve mitochondrial function in both ovulated and IVM oocytes and improved the mitochondrial function damage in CCCP-treated oocytes, which may have a potential value in human ART.
Materials and methods
Ethics statement
Female ICR mice were purchased from the Beijing Vital River Laboratory Animal Technology Co., Ltd. All mice were handled in accordance with the institutional animal care policies of the Institute of Zoology, Chinese Academy of Sciences. Mice were maintained under a 12-h light and 12-h darkness cycle in a specific pathogen-free stage at the Central Animal Laboratory of the Institute of Zoology. The Laboratory Animal Care and Use Committee of the Institute of Zoology approved this study.
Animals
Female mice were administrated intraperitoneal injections of 10 IU pregnant mare serum gonadotropin (PMSG, also called equine chorionic gonadotropin, eCG). After 44 to 48 hours, germinal vesicle (GV) oocyte were collected by chopping with a blade. Female mice were injected with 10 IU pregnant mare serum gonadotropin (PMSG) followed 44 to 48 hours later by injection of 10 IU human chorionic gonadotropin (HCG) (both from Ningbo Hormone Product Co. Ltd., Cixi, China). After 14 h of HCG, ovulated cumulus-oocyte complexes (COCs) were collected and denuded in 1mg/ml hyaluronidase.
Analysis of oocyte mitochondrial potential
Oocytes were denuded as described above [Citation69], and incubated in M2 culture medium (M7167, Sigma-Aldrich, USA) with JC-1 (C2005, Beyotime, China), a cell permeable voltage-sensitive fluorescent mitochondrial dye, for 30 minutes [Citation70]. Briefly, after washing once with M2 medium, the oocytes were imaged immediately in green and red fluorescence channels using a Zeiss 780 spectral scanning confocal microscope at identical magnification and gain settings throughout the experiments. JC-1 emits red fluorescence in highly energized mitochondrial potential (aggregated dye), while depolarized mitochondrial potential emits green fluorescence (monomer dye). A software Zeiss Zen Pro was used to analyze fluorescence intensity.
Real-time recording of [Ca2+]i changes
Oocyte [Ca2+]i oscillations induced by 10 mM strontium chloride (SrCl2, 10025–70-4, Sangon Biotech, Shanghai in Ca2+-free CZB) was detected using lμM Fluo-4 AM calcium probe [Citation39,Citation71] in M2 medium (IVM group) or CZB (ovulated MII oocytes) after treatment with different concentrations of CCCP (0μM, 0.1μM, 1μM, 10μM, 100μM), a mitochondrial uncoupler, LC (0.75 mg/ml)- [Citation20] or BGP-15 (30 μM) [Citation21]. Oocyte mitochondrial membrane potential and oocyte survival were examined 4 h after parthenogenetic activation. Real-time images were obtained using a time-lapse confocal laser microscope (UltraVIEW-VoX; PerkinElmer) and recorded at 2 frames per minute. [Ca2+]i intensity was detected using an argon laser at 488 nm (Supplemental Video). Several parameters of the [Ca2+]i oscillation curve are shown in Supplemental Figure 1.
Statistical analysis
All experiments were conducted at least three times. No less than 20 oocytes were collected and examined in each groups. We presented information of samples with Means and Standard Deviations (SD). Results were analyzed by SPSS 19.0. The significance of differences between groups was analyzed by the Chi-square test and p values less than 0.05 were considered statistically significant.
Author contributions
Feng Wang, Qing-Yuan Sun and Xiang-Hong Ou conceived and designed the experiments. Feng Wang and others conducted experiments. Feng Wang and Qing-Yuan Sun analyzed the data. Feng Wang, Heide Schatten, Qing-Yuan Sun and Xiang-Hong Ou wrote the manuscript.
Supplemental Material
Download Zip (1.2 MB)Disclosure statement
No potential conflict of interest was reported by the authors.
Supplementary material
Supplemental data for this article can be accessed here.
Additional information
Funding
References
- Chow KM, Cheung MC, Cheung IK. Psychosocial interventions for infertile couples: a critical review. J Clin Nurs. 2016 Aug;25(15–16):2101–2113. PubMed PMID: 27278496.
- Schatten H, Sun QY, Prather R. The impact of mitochondrial function/dysfunction on IVF and new treatment possibilities for infertility. Reprod Biol Endocrinol. 2014 Nov 24;12:111. PubMed PMID: 25421171; PubMed Central PMCID: PMCPMC4297407.
- Stricker SA. Comparative biology of calcium signaling during fertilization and egg activation in animals. Dev Biol. 1999 Jul 15;211(2):157–176. PubMed PMID: 10395780.
- Wakai T, Zhang N, Vangheluwe P, et al. Regulation of endoplasmic reticulum Ca(2+) oscillations in mammalian eggs. J Cell Sci. 2013 Dec 15;126(Pt 24):5714–5724. PubMed PMID: 24101727; PubMed Central PMCID: PMCPMC3860313.
- Yazawa H, Yanagida K, Sato A. Human round spermatids from azoospermic men exhibit oocyte-activation and Ca2+ oscillation-inducing activities. Zygote. 2007 Nov;15(4):337–346. PubMed PMID: 17967213.
- Morrill GA, Kostellow AB, Mahajan S, et al. Role of calcium in regulating intracellular pH following the stepwise release of the metabolic blocks at first-meiotic prophase and second-meiotic metaphase in amphibian oocytes. Biochim Biophys Acta. 1984 May 22;804(1):107–117. PubMed PMID: 6609721.
- Deng MQ, Fan BQ. Intracellular Ca2+ during fertilization and artificial activation in mouse oocytes. Zhongguo Yao Li Xue Bao. 1996 Jul;17(4):357–360. PubMed PMID: 9812724.
- Jungheim ES, Travieso JL, Hopeman MM. Weighing the impact of obesity on female reproductive function and fertility. Nutr Rev. 2013 Oct;71 Suppl 1:S3–8. PubMed PMID: 24147921; PubMed Central PMCID: PMCPMC3813308.
- Bustos G, Cruz P, Lovy A, et al. Endoplasmic reticulum-mitochondria calcium communication and the regulation of mitochondrial metabolism in cancer: a novel potential target. Front Oncol. 2017;7:199. PubMed PMID: 28944215; PubMed Central PMCID: PMCPMC5596064.
- Hajnoczky G, Robb-Gaspers LD, Seitz MB, et al. Decoding of cytosolic calcium oscillations in the mitochondria. Cell. 1995 Aug 11;82(3):415–424. PubMed PMID: 7634331.
- Boulais M, Soudant P, Le Goic N, et al. Involvement of mitochondrial activity and OXPHOS in ATP synthesis during the motility phase of spermatozoa in the pacific oyster, crassostrea gigas. Biol Reprod. 2015 Nov;93(5):118. PubMed PMID: 26423125.
- Pedriali G, Rimessi A, Sbano L, et al. Regulation of endoplasmic reticulum-mitochondria Ca(2+) transfer and its importance for anti-cancer therapies. Front Oncol. 2017;7:180. PubMed PMID: 28913175; PubMed Central PMCID: PMCPMC5583168.
- Van Blerkom J. Mitochondrial function in the human oocyte and embryo and their role in developmental competence. Mitochondrion. 2011 Sep;11(5):797–813. PubMed PMID: 20933103.
- Adeva-Andany MM, Calvo-Castro I, Fernandez-Fernandez C, et al. Significance of l-carnitine for human health. IUBMB Life. 2017 Aug;69(8):578–594. PubMed PMID: 28653367.
- Chung J, Nguyen AK, Henstridge DC, et al. HSP72 protects against obesity-induced insulin resistance. Proc Natl Acad Sci U S A. 2008 Feb 5;105(5):1739–1744. PubMed PMID: 18223156; PubMed Central PMCID: PMCPMC2234214.
- Gehrig SM, van der Poel C, Sayer TA, et al. Hsp72 preserves muscle function and slows progression of severe muscular dystrophy. Nature. 2012 Apr 4;484(7394):394–398. PubMed PMID: 22495301.
- Literati-Nagy B, Kulcsar E, Literati-Nagy Z, et al. Improvement of insulin sensitivity by a novel drug, BGP-15, in insulin-resistant patients: a proof of concept randomized double-blind clinical trial. Horm Metab Res. 2009 May;41(5):374–380. PubMed PMID: 19214941.
- Nagy G, Szarka A, Lotz G, et al. BGP-15 inhibits caspase-independent programmed cell death in acetaminophen-induced liver injury. Toxicol Appl Pharmacol. 2010 Feb 15;243(1):96–103. PubMed PMID: 19931551.
- Wu LL, Russell DL, Wong SL, et al. Mitochondrial dysfunction in oocytes of obese mothers: transmission to offspring and reversal by pharmacological endoplasmic reticulum stress inhibitors. Development. 2015 Feb 15;142(4):681–691. PubMed PMID: 25670793.
- Fathi M, El-Shahat KH. L-carnitine enhances oocyte maturation and improves in vitro development of embryos in dromedary camels (Camelus dromedaries). Theriogenology. 2017 Aug 04;104:18–22. PubMed PMID: 28802113.
- Ohlen SB, Russell ML, Brownstein MJ, et al. BGP-15 prevents the death of neurons in a mouse model of familial dysautonomia. Proc Natl Acad Sci U S A. 2017 May 9;114(19):5035–5040. PubMed PMID: 28439028; PubMed Central PMCID: PMCPMC5441694.
- Minge CE, Bennett BD, Norman RJ, et al. Peroxisome proliferator-activated receptor-gamma agonist rosiglitazone reverses the adverse effects of diet-induced obesity on oocyte quality. Endocrinology. 2008 May;149(5):2646–2656. PubMed PMID: 18276752.
- Igosheva N, Abramov AY, Poston L, et al. Maternal diet-induced obesity alters mitochondrial activity and redox status in mouse oocytes and zygotes. PLoS One. 2010 Apr 9;5(4):e10074. PubMed PMID: 20404917; PubMed Central PMCID: PMCPMC2852405.
- Jungheim ES, Schoeller EL, Marquard KL, et al. Diet-induced obesity model: abnormal oocytes and persistent growth abnormalities in the offspring. Endocrinology. 2010 Aug;151(8):4039–4046. PubMed PMID: 20573727; PubMed Central PMCID: PMCPMC2940512.
- Shankar K, Zhong Y, Kang P, et al. Maternal obesity promotes a proinflammatory signature in rat uterus and blastocyst. Endocrinology. 2011 Nov;152(11):4158–4170. PubMed PMID: 21862610; PubMed Central PMCID: PMCPMC3199010.
- Luzzo KM, Wang Q, Purcell SH, et al. High fat diet induced developmental defects in the mouse: oocyte meiotic aneuploidy and fetal growth retardation/brain defects. PLoS One. 2012;7(11):e49217. PubMed PMID: 23152876; PubMed Central PMCID: PMCPMC3495769.
- Gesink Law DC, Maclehose RF, Longnecker MP. Obesity and time to pregnancy. Hum Reprod. 2007 Feb;22(2):414–420. PubMed PMID: 17095518; PubMed Central PMCID: PMCPMC1924918.
- Rittenberg V, Sobaleva S, Ahmad A, et al. Influence of BMI on risk of miscarriage after single blastocyst transfer. Hum Reprod. 2011 Oct;26(10):2642–2650. PubMed PMID: 21813669.
- Ou XH, Sun QY. Mitochondrial replacement techniques or therapies (MRTs) to improve embryo development and to prevent mitochondrial disease transmission. J Genet Genomics. 2017 Aug 20;44(8):371–374. PubMed PMID: 28847473.
- Ozil JP, Banrezes B, Toth S, et al. Ca2+ oscillatory pattern in fertilized mouse eggs affects gene expression and development to term. Dev Biol. 2006 Dec 15;300(2):534–544. PubMed PMID: 16996050.
- Ducibella T, Huneau D, Angelichio E, et al. Egg-to-embryo transition is driven by differential responses to Ca(2+) oscillation number. Dev Biol. 2002 Oct 15;250(2):280–291. PubMed PMID: 12376103.
- Bengtson CP, Bading H. Nuclear calcium signaling. Adv Exp Med Biol. 2012;970:377–405. PubMed PMID: 22351065.
- Griffiths EJ, Rutter GA. Mitochondrial calcium as a key regulator of mitochondrial ATP production in mammalian cells. Biochim Biophys Acta. 2009 Nov;1787(11):1324–1333. PubMed PMID: 19366607.
- Marchant JS, Ramos V, Parker I. Structural and functional relationships between Ca2+ puffs and mitochondria in Xenopus oocytes. Am J Physiol Cell Physiol. 2002 Jun;282(6):C1374–86. PubMed PMID: 11997252.
- Gordo AC, Rodrigues P, Kurokawa M, et al. Intracellular calcium oscillations signal apoptosis rather than activation in in vitro aged mouse eggs. Biol Reprod. 2002 Jun;66(6):1828–1837. PubMed PMID: 12021069.
- Ozil JP, Markoulaki S, Toth S, et al. Egg activation events are regulated by the duration of a sustained [Ca2+]cyt signal in the mouse. Dev Biol. 2005 Jun 1;282(1):39–54. PubMed PMID: 15936328.
- Berridge MJ, Lipp P, Bootman MD. The versatility and universality of calcium signalling. Nat Rev Mol Cell Biol. 2000 Oct;1(1):11–21. PubMed PMID: 11413485.
- Bootman MD, Lipp P, Berridge MJ. The organisation and functions of local Ca(2+) signals. J Cell Sci. 2001 Jun;114(Pt 12):2213–2222. PubMed PMID: 11493661.
- Hagen BM, Boyman L, Kao JP, et al. A comparative assessment of fluo Ca2+ indicators in rat ventricular myocytes. Cell Calcium. 2012 Aug;52(2):170–181. PubMed PMID: 22721780; PubMed Central PMCID: PMCPMC3393790.
- Jones DP. Mitochondrial dysfunction during anoxia and acute cell injury. Biochim Biophys Acta. 1995 May 24;1271(1):29–33. PubMed PMID: 7599222.
- Mehlmann LM, Kline D. Regulation of intracellular calcium in the mouse egg: calcium release in response to sperm or inositol trisphosphate is enhanced after meiotic maturation. Biol Reprod. 1994 Dec;51(6):1088–1098. PubMed PMID: 7888488.
- Wakai T, Fissore RA. Ca(2+) homeostasis and regulation of ER Ca(2+) in mammalian oocytes/eggs. Cell Calcium. 2013 Jan;53(1):63–67. PubMed PMID: 23260016; PubMed Central PMCID: PMCPMC4120948.
- Malhotra JD, Kaufman RJ. The endoplasmic reticulum and the unfolded protein response. Semin Cell Dev Biol. 2007 Dec;18(6):716–731. PubMed PMID: 18023214; PubMed Central PMCID: PMCPMC2706143.
- Vannuvel K, Renard P, Raes M, et al. Functional and morphological impact of ER stress on mitochondria. J Cell Physiol. 2013 Sep;228(9):1802–1818. PubMed PMID: 23629871.
- Dumollard R, Marangos P, Fitzharris G, et al. Sperm-triggered [Ca2+] oscillations and Ca2+ homeostasis in the mouse egg have an absolute requirement for mitochondrial ATP production. Development. 2004 Jul;131(13):3057–3067. PubMed PMID: 15163630.
- Van Blerkom J, Davis PW, Lee J. ATP content of human oocytes and developmental potential and outcome after in-vitro fertilization and embryo transfer. Hum Reprod. 1995 Feb;10(2):415–424. PubMed PMID: 7769073.
- Dumollard R, Duchen M, Carroll J. The role of mitochondrial function in the oocyte and embryo. Curr Top Dev Biol. 2007;77:21–49. PubMed PMID: 17222699.
- Zhou X, Liu F, Zhai S. Effect of L-carnitine and/or L-acetyl-carnitine in nutrition treatment for male infertility: a systematic review. Asia Pac J Clin Nutr. 2007;16 Suppl 1:383–390. PubMed PMID: 17392136.
- Vanella A, Russo A, Acquaviva R, et al. L -propionyl-carnitine as superoxide scavenger, antioxidant, and DNA cleavage protector. Cell Biol Toxicol. 2000;16(2):99–104. PubMed PMID: 10917565.
- Gulcin I. Antioxidant and antiradical activities of L-carnitine. Life Sci. 2006 Jan 18;78(8):803–811. PubMed PMID: 16253281.
- Pillich RT, Scarsella G, Risuleo G. Reduction of apoptosis through the mitochondrial pathway by the administration of acetyl-L-carnitine to mouse fibroblasts in culture. Exp Cell Res. 2005 May 15;306(1):1–8. PubMed PMID: 15878327.
- Sharma S, Black SM. Carnitine homeostasis, mitochondrial function, and cardiovascular disease. Drug Discov Today Dis Mech. 2009;6(1–4):e31–e39. PubMed PMID: 20648231; PubMed Central PMCID: PMCPMC2905823.
- Nelson HK, Lauber RP, Sheard NF. Effect of various levels of supplementation with sodium pivalate on tissue carnitine concentrations and urinary excretion of carnitine in the rat. J Nutr Biochem. 2001 Apr;12(4):242–250. PubMed PMID: 11287220.
- Jones LL, McDonald DA, Borum PR. Acylcarnitines: role in brain. Prog Lipid Res. 2010 Jan;49(1):61–75. PubMed PMID: 19720082.
- Isidori AM, Pozza C, Gianfrilli D, et al. Medical treatment to improve sperm quality. Reprod Biomed Online. 2006 Jun;12(6):704–714. PubMed PMID: 16792845.
- Mansour G, Abdelrazik H, Sharma RK, et al. L-carnitine supplementation reduces oocyte cytoskeleton damage and embryo apoptosis induced by incubation in peritoneal fluid from patients with endometriosis. Fertil Steril. 2009 May;91(5 Suppl):2079–2086. PubMed PMID: 18394615.
- Jamilian H, Jamilian M, Samimi M, et al. Oral carnitine supplementation influences mental health parameters and biomarkers of oxidative stress in women with polycystic ovary syndrome: a randomized, double-blind, placebo-controlled trial. Gynecol Endocrinol. 2017 Jun;33(6):442–447. PubMed PMID: 28277138.
- Hashimoto S. Application of in vitro maturation to assisted reproductive technology. J Reprod Dev. 2009 Feb;55(1):1–10. PubMed PMID: 19276618.
- Gombos I, Crul T, Piotto S, et al. Membrane-lipid therapy in operation: the HSP co-inducer BGP-15 activates stress signal transduction pathways by remodeling plasma membrane rafts. PLoS One. 2011;6(12):e28818. PubMed PMID: 22174906; PubMed Central PMCID: PMCPMC3236211.
- Henstridge DC, Bruce CR, Drew BG, et al. Activating HSP72 in rodent skeletal muscle increases mitochondrial number and oxidative capacity and decreases insulin resistance. Diabetes. 2014 Jun;63(6):1881–1894. PubMed PMID: 24430435; PubMed Central PMCID: PMCPMC4030108.
- Sumegi K, Fekete K, Antus C, et al. BGP-15 protects against oxidative stress- or lipopolysaccharide-induced mitochondrial destabilization and reduces mitochondrial production of reactive oxygen species. PLoS One. 2017;12(1):e0169372. PubMed PMID: 28046125; PubMed Central PMCID: PMCPMC5207682.
- Crul T, Toth N, Piotto S, et al. Hydroximic acid derivatives: pleiotropic HSP co-inducers restoring homeostasis and robustness. Curr Pharm Des. 2013;19(3):309–346. PubMed PMID: 22920902.
- Literati-Nagy B, Tory K, Peitl B, et al. Improvement of insulin sensitivity by a novel drug candidate, BGP-15, in different animal studies. Metab Syndr Relat Disord. 2014 Mar;12(2):125–131. PubMed PMID: 24386957.
- Salah H, Li M, Cacciani N, et al. The chaperone co-inducer BGP-15 alleviates ventilation-induced diaphragm dysfunction. Sci Transl Med. 2016 Aug 3;8(350):350ra103. PubMed PMID: 27488897.
- Moawad AR, Xu B, Tan SL, et al. l-carnitine supplementation during vitrification of mouse germinal vesicle stage-oocytes and their subsequent in vitro maturation improves meiotic spindle configuration and mitochondrial distribution in metaphase II oocytes. Hum Reprod. 2014 Oct 10;29(10):2256–2268. PubMed PMID: 25113843.
- Moawad AR, Tan SL, Xu B, et al. L-carnitine supplementation during vitrification of mouse oocytes at the germinal vesicle stage improves preimplantation development following maturation and fertilization in vitro. Biol Reprod. 2013 Apr;88(4):104. PubMed PMID: 23446455.
- Greenfield A, Braude P, Flinter F, et al. Assisted reproductive technologies to prevent human mitochondrial disease transmission. Nat Biotechnol. 2017 Nov 9;35(11):1059–1068. PubMed PMID: 29121011.
- Igarashi H, Takahashi T, Abe H, et al. Poor embryo development in post-ovulatory in vivo-aged mouse oocytes is associated with mitochondrial dysfunction, but mitochondrial transfer from somatic cells is not sufficient for rejuvenation. Hum Reprod. 2016 Oct;31(10):2331–2338. PubMed PMID: 27591230.
- Meng TG, Hu MW, Ma XS, et al. Oocyte-specific deletion of furin leads to female infertility by causing early secondary follicle arrest in mice. Cell Death Dis. 2017 Jun 1;8(6):e2846. PubMed PMID: 28569793; PubMed Central PMCID: PMCPMC5520891.
- Liu Y, Han M, Li X, et al. Age-related changes in the mitochondria of human mural granulosa cells. Hum Reprod. 2017 Dec 1;32(12):2465–2473. PubMed PMID: 29045673.
- Yoon SY, Eum JH, Lee JE, et al. Recombinant human phospholipase C zeta 1 induces intracellular calcium oscillations and oocyte activation in mouse and human oocytes. Hum Reprod. 2012 Jun;27(6):1768–1780. PubMed PMID: 22456923.