ABSTRACT
Although the impact of MAPT (Tau) expression has been well documented for neuronal cells in the context of tauopathies and neurodegenerative diseases, the impact and role of Tau expression in cancer, and specifically cancers of neuronal origin, is in its infancy. To determine the correlation between MAPT expression and survival in pediatric neuroblastoma, MAPT gene expression for samples from the TARGET pediatric neuroblastoma dataset was assessed. Initial analyses indicated that increased MAPT expression correlated with increased overall survival in neuroblastoma but not in ovarian cancer. Expression of apoptosis- and proliferation-effector genes in the neuroblastoma samples was consistent with the MAPT related survival result. Furthermore, we determined that higher neuroblastoma expression of APP also associated with neurodegeneration, correlated with better neuroblastoma survival rates. In sum, Gene expression associated with neuronal degenerative diseases was associated with a better neuroblastoma outcome.
Abbreviations: ALS: Amyotrophic Lateral Sclerosis; APP: Amyloid Precursor Protein gene; CASP3: Caspase 3 gene; CASP9: Caspase 9 gene; H2AFX: H2A histone family, member X gene; HIST1H2AL: Histone H2A type 1 gene; HIST1H2BK: Histone H2B type 1-K gene; HIST1H3J: Histone H3J gene; HIST1H4B: Histone H4B gene; HIST2H2BE: Histone H2B type 2-E gene; HUGO: human genome organization; KM: Kaplan-Meier survival curve; MAPT: Tau gene; OV: Ovarian cancer; SNCA: alpha-syneculin gene; TARDBP: Transactive response DNA binding protein 43 kDa; TCGA: the cancer genome atlas
1. Introduction
Expression of the Tau protein, encoded by the MAPT gene (Microtubule Associated Protein – Tau), is associated with several neurodegenerative disorders that result in neuronal cell death [Citation1,Citation2]. The majority of research into the function of the Tau protein is driven by the context of tauopathies. There has been relatively little research investigating the role of Tau in cancer, particularly in cancers of neuronal origin.
Neuroblastoma is an aggressive pediatric cancer of neuronal origin that causes approximately 13% of all pediatric cancer deaths and is the primary cause of cancer-related death for children between the ages of one and five years [Citation3]. It is the third most common pediatric cancer, after leukemia and brain cancers [Citation4]. Furthermore, neuroblastoma tumors are able to mature spontaneously, and there are certain phenotypes, dubbed “ultra high risk” that tend to have increased resistance to therapy [Citation5–Citation7]. The International Neuroblastoma Risk Group classification system [Citation8] was developed to aid in the clinical management of pediatric neuroblastoma. Based on molecular and clinical features, patients are grouped into either low, intermediate, or high risk groups, with high risk groups having poor survival outcomes. Thus, there is a need to identify biological characteristics that may help better inform clinicians regarding the risk-status of the neuroblastoma patients [Citation5]. In this report, we examined the connection between Tau expression in neuroblastoma and survival rates.
2. Methods
2.1. MAPT, SNCA, APP, TARDBP, apoptosis-effector gene and pro-proliferative gene microarray and rnaseq analysis
Microarray and RNASeq values for MAPT were downloaded from cBioPortal.org. Also, microarray values for SNCA, APP, TARDBP, and a previously studied panel of apoptosis- and proliferation-effector genes [Citation9] (Tables S1, S2), were downloaded from cBioPortal.org. Pearson correlation coefficients were obtained using Graphpad Prism software version 7.0. Only Pediatric neuroblastoma barcodes with either microarray or RNAseq data available, as indicated in Results, were used in this report.
2.2. Kaplan-meier analyses and microarray data
Clinical data, including overall survival data, for TARGET Pediatric Neuroblastoma and TCGA Provisional Ovarian Serous Cystadenocarcinoma (OV) were downloaded from cBioPortal.org. The barcodes (patients identifiers) representing 249 TARGET pediatric neuroblastoma samples with microarray data, 143 TARGET pediatric neuroblastoma samples with RNAseq data, and 558 TCGA provisional OV (ovarian cancer) samples with available microarray data were sorted from high to low based on the respective gene expression values (microarray or RNAseq). Next, overall survival relationships were obtained by matching gene expression data with survival data as indicated in Results. The overall survival data were then processed using Graphpad Prism software version 7.0, to generate Kaplan-Meier (KM) survival curves and to assess median survivals for each cohort tested.
2.3. MYCN amplification analysis
Amplification data for the MYCN gene for TARGET Pediatric Neuroblastoma barcodes with available microarray data (n = 249) were downloaded from cBioPortal.org. Amplification data were then matched onto barcode microarray data. A Student’s t-test was conducted, using the Microsoft Excel function, to distinguish MYCN amplification data representing the top and bottom 20% of MAPT microarray values
2.4. Risk group analysis
Risk group classifications for TARGET pediatric neuroblastoma barcodes with available microarray data (n = 249) were obtained from cBioPortal.org. Barcodes were first stratified by risk designation and then matched to barcode microarray data. A Student’s t-test was conducted to distinguish MAPT expression levels among the high risk cohort representing the top 20% of the MAPT microarray values and all remaining, high risk barcodes (bottom 80%). Barcodes were then matched to overall survival data and KM analyses were conducted.
2.5. Analysis of MAPT, APP, and TARDBP using the R2 genomics analysis and visualization platform
Overall survival data and gene expression data for additional, independent neuroblastoma datasets were assessed using the ‘R2: Genomics Analysis and Visualization Platform (http://r2.amc.nl)’. Survival distinctions based on MAPT expression were assessed using the Kaplan scan analysis tool as indicated in Results. The cutoff used for overall survival comparison was Median MAPT mRNA expression across the indicated dataset. Kaplan-Meier overall survival curves were generated using the R2 algorithm to compare survival outcomes between the top half and bottom half of MAPT expressers in each indicated dataset.
3. Results
3.1. MAPT (tau) expression and survival rates
To determine whether Tau expression may confer a survival advantage in pediatric neuroblastoma, we compared the rate of overall survival for patients representing the top and bottom quintiles (20%) of MAPT expressers, based on MAPT microarray values obtained from cBioPortal.org. The microarray values, including averages, for the top and bottom 20% levels of expression, respectively, for MAPT for pediatric neuroblastoma are provided in the SOM (Table S3). Results indicated that the higher MAPT microarray values were associated with a significantly increased overall survival rate in pediatric neuroblastoma (), Table S4). The median survival periods and p-values for the log-rank tests are given in the legend for . (See also Table S5.)
Figure 1. Kaplan-Meier curves representing distinct MAPT gene expression levels. (A) Kaplan-Meier (KM) overall survival (OS) curve representing pediatric neuroblastoma barcode microarray values in the top quintile of MAPT expressers (n = 48, gray, arrow), compared to the OS for the bottom quintile of MAPT expressers (n = 49, black). Mean OS for the top quintile of MAPT expressers, undefined months; mean OS for bottom quintile of MAPT expressers, 37 months. Log rank comparison p-value = 0.0023. (B) KM OS curve for ovarian serous cystadenocarcinoma (OV) barcode microarray values in the top quintile of MAPT expressers (n = 103, gray, arrow), compared to the OS for the bottom quintile of MAPT expressers (n = 102, black). Mean OS for the top quintile of MAPT expressers, 38.40 months; mean OS for bottom quintile of MAPT expressers, 47.57 months. Log rank comparison p-value = 0.3471 (not significant). (C) KM OS curve for pediatric neuroblastoma barcode microarray values in the top quintile of SNCA expressers (n = 48, gray, arrow), compared to the OS for the bottom quintile of SNCA expressers (n = 49, black). Mean OS for the top quintile of SNCA expressers, undefined months; mean OS for bottom quintile of SNCA expressers, 52 months. Log rank comparison p-value = 0.1370 (not significant). (D) KM OS curve for pediatric neuroblastoma barcode RNAseq values in the top half of MAPT expressers (n = 68, gray, arrow), compared to the OS for the bottom half of MAPT expressers (n = 66, black). Mean OS for the top half of MAPT expressers, 125 months; mean OS for bottom half of MAPT expressers, 44 months. Log rank comparison p-value = 0.0074. (E) KM OS curve for pediatric neuroblastoma barcode RNAseq values in the top quintile of MAPT expressers (n = 27, gray, arrow), compared to the OS for the bottom quintile of MAPT expressers (n = 28, black). Mean OS for the top quintile of MAPT expressers, 80 months; mean OS for bottom quintile of MAPT expressers, 34 months. Log rank comparison p-value = 0.2205.
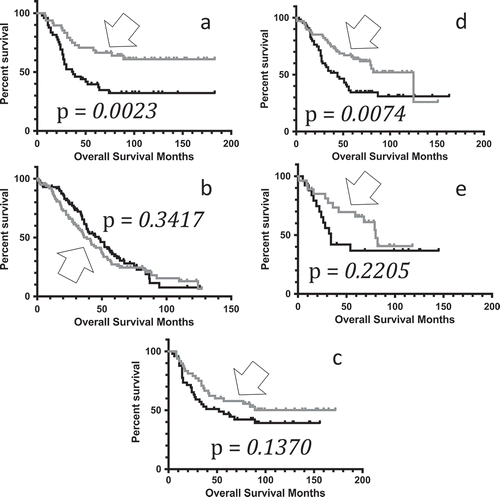
To determine whether this association of increased MAPT microarray levels with increased overall survival rates was specific to pediatric neuroblastoma, we downloaded overall survival data for the OV dataset from cBioPortal.org, and the microarray and KM analyses were conducted (Tables S4, S6). Results indicated that for the OV dataset, MAPT expression levels were not associated with distinct overall survival advantages ()).
To determine whether the increased survival advantage in high MAPT-expressing pediatric neuroblastoma patients was specific for Tau, we assessed whether there was a survival advantage conferred by alpha-syneculin (SNCA), using the microarray expression values in pediatric neuroblastoma patients from the same cohort as was used for the MAPT microarray analysis (Table S7). Results indicated that there were no significant overall survival differences represented by the pediatric neuroblastoma barcodes for the top and bottom SNCA expression quintiles (), Table S8).
To further verify the initial results of MAPT microarray values associating with a survival advantage in pediatric neuroblastoma, we repeated the analysis using RNAseq values obtained from cBioPortal.org and compared the top 50% and 20% of MAPT expressers with their corresponding lower level MAPT expressers (bottom 50% and bottom 20%, respectively) for overall survival. In both cases, the initial result was supported, and the same trend towards increased survival in high MAPT was observed (). Because there was a higher sample size of barcodes with microarray data available (n = 249) than RNAseq data available (n = 143), the microarray values were used for the remaining analyses in this report.
3.2. Pediatric neuroblastoma apoptosis-effector gene microarray values correlated with MAPT microarray values
Because Tau is associated with neurodegenerative disease and neuronal cell death, we determined whether apoptosis-effector genes (Tables S1) were expressed at higher levels in the neuroblastoma samples represented by the barcodes with higher levels of MAPT microarray values. We first evaluated a set of 28 apoptosis effector genes [Citation9] for microarray expression in the same cohort of pediatric neuroblastoma barcodes which were used for the previous KM analyses. Of those 28 genes, we identified a subset of seven apoptosis-effector genes that had significantly increased microarray values associated with barcodes that represented the top 20% of MAPT expressers when compared to the bottom 20% of MAPT expressers: AIFM2, CASP3, CASP9, COX6A1, CRADD, and GZMB ().
Table 1. Average microarray values for pediatric neuroblastoma barcodes representing high and low expression of MAPT.
To verify the above positive correlations of apoptosis-effector gene microarray values with MAPT microarray values, we obtained the Pearson correlation coefficients for the MAPT expression levels and the expression levels of AIFM2, CASP3, CASP9, COX6A1, CRADD, and GZMB in pediatric neuroblastoma. In each case, there was a statistically significant positive correlation with MAPT expression ().
Figure 2. Correlation of MAPT and apoptosis-effector gene expression levels. MAPT microarray expression vs. apoptosis-effector gene microarray expression. The p-values for the Pearson Correlation Coefficients are presented after each relationship, respectively. (A) MAPT vs. AIFM2; p = 0.0008; (B) MAPT vs. BAD; p = 0.0051; (C) MAPT vs. CASP3; p = 0.0050; (D) MAPT vs. CASP9; p < 0.0001; (E) MAPT vs. COX6A1; p = 0.0005; (F) MAPT vs. CRADD; p < 0.0001; (G) MAPT vs. GZMB; p = 0.0205.
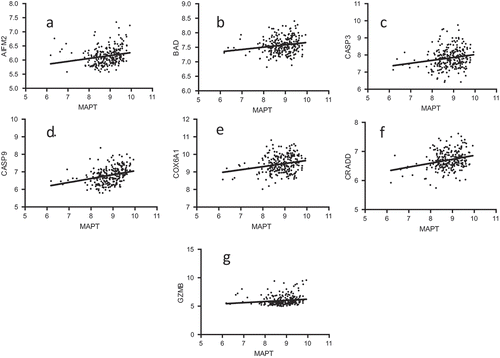
To determine whether any of the seven above identified apoptosis-effector genes could represent independent markers of survival rates, the overall survival data for pediatric neuroblastoma barcodes representing the top quintile (20%) and bottom quintile (20%) of AIFM2, CASP3, CASP9, COX6A1, CRADD, and GZMB expression levels were obtained for KM analysis, using the same method as indicated previously. Results indicated that both CASP3 and CASP9, two critical components of apoptosis functionality, represented independent markers of survival, with barcodes representing the highest 20% of both CASP3 and CASP9 having significantly increased survival rates (; Table S9, S10).
Figure 3. Kaplan-Meier curves representing distinct apoptosis-effector gene expression levels. (A) KM OS curve for pediatric neuroblastoma barcode microarray values in the top quintile of CASP3 expressers (n = 49, gray, arrow), compared to the OS for the bottom quintile of CASP3 expressers (n = 49, black). Mean OS for the top quintile of CASP3 expressers, undefined months; mean OS for bottom quintile of CASP3 expressers, 37 months. Log rank comparison p-value, p = 0.0052. (B) KM OS curve for pediatric neuroblastoma barcode microarray values in the top quintile of CASP9 expressers (n = 49, gray, arrow), compared to the OS for the bottom quintile of CASP9 expressers (n = 49, black). Mean OS for the top quintile of CASP9 expressers, undefined months; mean OS for bottom quintile of CASP9 expressers, 38.5 months. Log rank comparison p-value, p = 0.0353.
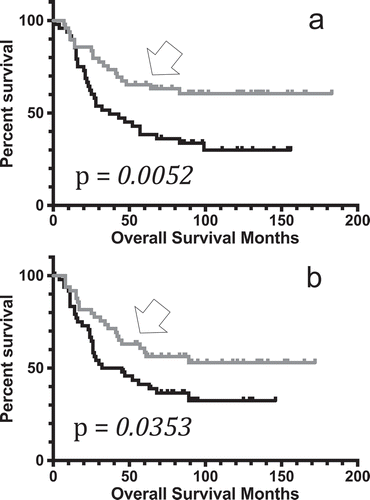
3.3. Pediatric neuroblastoma proliferation-effector gene microarray values inversely correlated with MAPT microarray values
We next determined whether low levels of MAPT expression were associated with increased proliferation-effector gene expression (Table S2). We first evaluated a set of 11 histone genes [Citation9] for microarray expression in the same cohort of pediatric neuroblastoma barcodes with microarray data available and used for the previous KM and apoptosis analyses. Of the 11 histone genes, we identified 10 genes that had increased microarray values statistically, significantly associated with barcodes that represented the bottom 20% of MAPT expressers (when compared to the top 20% of MAPT expressers): H1FX, H2AFX, HIST1H2AL, HIST1H2BK, HIST1H3J, HIST1H4B, HIST1H4J, HIST2H2BE, HIST2H3D, and HIST3H2A (). Only H3F3B expression represented the opposite trend, with the top quintile of MAPT expressers also expressing increased H3F3B ().
Table 2. Average microarray values for pediatric neuroblastoma barcodes representing high and low expression of MAPT.
To verify the above inverse correlations of proliferation-effector gene microarray values with MAPT microarray values, we obtained the Pearson correlation coefficients for the MAPT expression levels and the expression levels of H1FX, H2AFX, H3F3B, HIST1H2AL, HIST1H2BK, HIST1H3J, HIST1H4B, HIST1H4J, HIST2H2BE, HIST2H3D, and HIST3H2A in pediatric neuroblastoma. In all cases, except for H3F3B, there was a statistically significant inverse correlation with MAPT expression (Fig. S1).
To determine whether the expression of any of the above histone genes could represent an independent marker for survival rates, the overall survival data for pediatric neuroblastoma barcodes representing the top quintile (20%) and bottom quintile (20%) for H1FX, H2AFX, HIST1H2AL, HIST1H2BK, HIST1H3J, HIST1H4B, HIST1H4J, HIST2H2BE, HIST2H3D, and HIST3H2A expression levels were obtained for KM analysis. Results indicated that both H1FX and HIST2H3D represented independent markers of survival, with barcodes representing the highest 20% of both H1FX and HIST2H3D having significantly decreased survival rates (Fig. S2; Tables S11, S12).
3.4. MYCN amplification correlated with MAPT microarray values
We next determined whether MAPT expression could be correlated with other risk factors for pediatric neuroblastoma. Amplification of MYCN is classically associated with neuroblastoma, and neuroblastoma patients with MYCN amplifications have worse outcomes [Citation10–Citation12]. As such, we next determined whether MAPT expression correlated with MYCN amplification. We compared MYCN amplification between the top quintile of MAPT expressers and bottom quintile of MAPT expressers. We determined that the bottom quintile of MAPT-expressing barcodes, that also had decreased overall survival, also had a significantly greater incidence of MYCN amplification (; Table S13). Interestingly, there were no barcodes in the top quintile of MAPT expressers that had an MYCN amplification.
Table 3. Identifying MYCN copy number variation associations with MAPT expression (For details, see Table S13).
3.5. Risk group correlated with MAPT microarray values
Given that pediatric neuroblastoma is grouped into three risk categories (high, intermediate, and low) based on clinical and molecular characteristics of patients, we determined whether MAPT expression could be a biomarker for the high risk patients. We first stratified the barcodes used in the previous analyses by risk group based on risk group distinction available in the original TARGET dataset (Table S14). We next established statistically significant difference in MAPT expression between the top 20% and all remaining (bottom 80%) barcodes, solely among the high risk cohort of the barcodes (Fig. S3, Table S15). Finally, we performed a KM analysis on pediatric neuroblastoma barcodes representing the top quintile of MAPT expressers and the bottom 80% of MAPT expressers. Results indicated that the high risk barcodes representing the top quintile of MAPT expressers (Fig. S3) had significantly increased survival rates when compared to all remaining high risk barcodes (Fig. S3, Table S16, S17). (The median survival periods and p-values for the log-rank tests are given in the legend for Fig. S3.)
3.6. APP (amyloid precursor protein) and TARDBP (TAR dna-binding protein 43) expression and survival rates for neuroblastoma
To determine whether the increased survival advantage in high MAPT-expressing pediatric neuroblastoma patients could be observed for other neurotoxic proteins, we assessed whether there was a survival advantage associated with high APP microarray expression values in pediatric neuroblastoma patients (Table S18). Results indicated that higher APP microarray values were associated with a significantly increased overall survival rate in pediatric neuroblastoma (Fig. S4A, Table S19, S20).
We next determined whether increased microarray expression values of TARDBP, the expression of which has been associated with dementia [Citation13,Citation14] and Amyotrophic Lateral Sclerosis (ALS) [Citation15], correlates with a survival advantage for the pediatric neuroblastoma patients. The microarray values, including averages, for the top and bottom 20% levels of expression, respectively, for TARDBP for pediatric neuroblastoma are provided in the SOM (Table S21). (These microarray value averages represented statistically significant differences.) Results indicated that the higher TARDBP microarray values were associated with a significantly increased overall survival rate in pediatric neuroblastoma (Fig. S4B, Table S22). The median survival periods and p-values for the log-rank tests for both APP and TARDBP expression are given in the figure legend for Fig. S4.
3.7. Analysis of survival associations with MAPT, APP, and TARDBP expression in additional neuroblastoma datasets from the R2: genomics analysis and visualization platform
To determine whether MAPT mRNA expression is associated with improved survival outcomes in additional, independent neuroblastoma datasets, the R2: Genomics analysis and visualization platform was used. Two additional neuroblastoma datasets were analyzed, with results indicating a strong, statistically significant association of MAPT expression with longer survial (Fig. S5). Also, the two replicative datasets were consistent with the above results for APP expression, which indicated the a higher level of APP was associated with a higher rate of survival. However, these two R2-based datasets were not consistent with above results for TARDBP and did not replicate the association of high TARDBP expression levels with longer survival.
4. Discussion
Although the overall survival rates for pediatric neuroblastoma have been steadily increasing from 53% between 1975–1977 to 74% between 2005–2011, there is continued expectation that investigating the molecular basis for neuroblastoma will improve patient outcomes [Citation16]. The above results indicated that high MAPT expression correlated with better survival for pediatric neuroblastoma, including the subset of the high-risk cohort of patients. While the MAPT expression differences studied here can seem as modest distinctions, despite tests for statistical significance that indicate otherwise, the differences likely represent the expression gradient realities [Citation17,Citation18] of a natural cellular and tissue context, rather than an experimental “on/off” construction. In any event, the distinction of expression levels between quintiles, for MAPT, was also supported by correlation plots representing the entire MAPT expression dataset, as well as with two additional, replicative datasets.
The result that higher MAPT expression correlates with better outcomes was consistent with higher expression of certain apoptosis-effector genes among the samples represented by the high MAPT expression. Likewise, the result was consistent with the lower expression of the pro-proliferative histone genes among the samples that had a high MAPT expression. Importantly, the expression of MAPT, the apoptosis effector genes, and the histone genes could all be used as independent biomarkers of survival distinctions. Finally, consistent with the neuronal degradation impact of increased expression of MAPT, well known in neurodegenerative conditions, the overexpression of the APP gene also correlated with better survival rates for pediatric neuroblastoma, for all three datasets, i.e., the cBioPortal.org dataset and the R2 datasets.
There were TARGET microarray data for 249 patients and RNAseq data available for 143 patients. Thus, our analyses only incorporated the subset of the total TARGET pediatric neuroblastoma dataset (n = 1076) that had gene expression data available. Regardless, when the bottom quintile of MAPT expressers (n = 49) used in our analyses was compared to all remaining barcodes in the population of barcodes in the TARGET pediatric neuroblastoma dataset the result is consistent with the results presented in this report. The bottom quintile of MAPT expressers used in our analyses has a statistically significant worse survival outcome than all remaining barcodes in the pediatric neuroblastoma population in the TARGET pediatric neuroblastoma dataset (n = 1027, p < 0.0001) (Table S23).
There have been previous studies conducted assessing Tau in neuroblastoma cell lines [Citation19–Citation22]. For example, Monroy-Ramirez et al. found that overexpression of the Tau protein causes significant deformity in nuclear architecture in a neuroblastoma cell line (SH-SY5Y) [Citation23]. However, the majority of these previous reports investigated Tau in the context of Alzheimer’s and tauopathies, often using neuroblastoma cell lines as a surrogate for neural cells. That is, the role of Tau in the development of neurological cancers has not been well studied. Cancer research, and treatment relating to Tau has focused on cancers of non-neurological origin where Tau has been a target because of it’s role as a microtubule stabilizer. For example, Tau downregulation has been demonstrated to increase sensitivity to taxane in breast cancer cells [Citation24].
Interestingly, Caspase-3 is the primary caspase associated with neural cell death in Alzheimer’s disease cleavage of the amyloid-beta 4A precursor protein [Citation25]. Caspase-3 cleaves the APP family members (including APLP1 and APLP2), generating a C-terminal peptide that contributes to neurotoxicity [Citation26]. Consistent with our results in APP, the top quintile of APLP2 expressers had increased overall survival when compared to the bottom quintile of APLP2 expressers in the TARGET pediatric neuroblastoma dataset (unpublished observations).
Microtubule-stabilizing agents are widely used in cancer treatment, including paclitaxel, whose mechanism of microtubule stabilization was first elucidated in the late 1970s [Citation27]; with clinical trials following thereafter [Citation28]. Given the Tau protein’s analogous functionality to paclitaxel function [Citation29–Citation31], it is not surprising that over-expression of Tau would inhibit cell proliferation. Bouge and colleagues have demonstrated in a Drosophila model that an excess expression of human Tau protein induces mitotic arrest, which leads to aneuploidy and cell death [Citation32]. Moreover, a MAPT-EGF fusion protein has demonstrated a proliferation-dependent cytotoxicity and the ability to inhibit mitosis in EGFR-overexpressing HEK293 kidney cancer cells through the stabilization of microtubules [Citation33]. Tau function being analogous to paclitaxel activity, and causing an anti-mitotic effect, may lie at the heart of an explanation for the negative correlation between MAPT expression and proliferation-effector gene expression.
Importantly, the above results may help to inform ongoing clinical trials. Fenretinide is currently being studied in clinical trials for neuroblastoma (clinicaltrials.gov). Fenretinide causes the buildup of ceramides in tumor cells [Citation34]. It also has been established that ceramides are key mediators of neuronal apoptosis in Alzheimer’s disease [Citation35]. Moreover, in vitro cell culture work suggests that sphingolipids, including ceramides, may cause or accelerate APP and MAPT-mediated pathology [Citation36]. These data, coupled with the above results, raise the question of whether Fenretinide could facilitate MAPT- and APP-mediated cell death in neuroblastoma, particularly in patients where MAPT expression is low prior to treatment?
Research involving human participants and/or animals
Not applicable.
Informed consent
Not applicable.
Supplemental Material
Download PDF (1.4 MB)Acknowledgments
Authors acknowledge the financial support of the taxpayers of the State of Florida. Saif Zaman and Boris Chobrutskiy are Morsani College of Medicine, RISE fellowship recipients.
Disclosure statement
Authors have nothing to declare.
Supplemental material
Supplemental data for this article can be accessed here.
References
- Spillantini MG, Goedert M. Tau pathology and neurodegeneration. Lancet Neurol. 2013;12:609–622.
- Buee L, Troquier L, Burnouf S, et al. From tau phosphorylation to tau aggregation: what about neuronal death? Biochem Soc Trans. 2010;38:967–972.
- Louis CU, Shohet JM. Neuroblastoma: molecular pathogenesis and therapy. Annu Rev Med. 2015;66:49–63.
- Maris JM, Hogarty MD, Bagatell R, et al. Neuroblastoma. Lancet. 2007;369:2106–2120.
- Davidoff AM. Neuroblastoma. Semin Pediatr Surg. 2012;21:2–14.
- Russo R, Cimmino F, Pezone L, et al. Kinome expression profiling of human neuroblastoma tumors identifies potential drug targets for ultra high-risk patients. Carcinogenesis. 2017;38:1011–1020.
- Saarinen-Pihkala UM, Jahnukainen K, Wikstrom S, et al. Ultrahigh-risk group within the high-risk neuroblastoma category. J Pediatr Hematol Oncol. 2013;35:e254–9.
- Cohn SL, Pearson AD, London WB, et al. The international neuroblastoma risk group (INRG) classification system: an INRG task force report. J Clin Oncol. 2009;27:289–297.
- Mauro JA, Blanck G. Functionally distinct gene classes as bigger or smaller transcription factor traps: a possible stochastic component to sequential gene expression programs in cancer. Gene. 2014;536:398–406.
- Cheng JM, Hiemstra JL, Schneider SS, et al. Preferential amplification of the paternal allele of the N-myc gene in human neuroblastomas. Nat Genet. 1993;4:191–194.
- Emanuel BS, Balaban G, Boyd JP, et al. N-myc amplification in multiple homogeneously staining regions in two human neuroblastomas. Proc Natl Acad Sci U S A. 1985;82:3736–3740.
- Brodeur GM, Seeger RC, Schwab M, et al. Amplification of N-myc in untreated human neuroblastomas correlates with advanced disease stage. Science. 1984;224:1121–1124.
- Borroni B, Bonvicini C, Alberici A, et al. Mutation within TARDBP leads to frontotemporal dementia without motor neuron disease. Hum Mutat. 2009;30:E974–83.
- Floris G, Borghero G, Cannas A, et al. Clinical phenotypes and radiological findings in frontotemporal dementia related to TARDBP mutations. J Neurol. 2015;262:375–384.
- Harms MM, Miller TM, Baloh RH. TARDBP-related amyotrophic lateral sclerosis. In: Adam MP, Ardinger HH, Pagon RA, et al., editors. Seattle (WA): GeneReviews((R)); 2009;1993–2018.
- Siegel RL, Miller KD, Jemal A. Cancer statistics, 2016. CA Cancer J Clin. 2016;66:7–30.
- Ford SA, Blanck G. Signal persistence and amplification in cancer development and possible, related opportunities for novel therapies. Biochim Biophys Acta. 2014;1855:18–23.
- Kellogg RA, Tay S. Noise facilitates transcriptional control under dynamic inputs. Cell. 2015;160:381–392.
- Lee YJ, Kim JE, Kwak MH, et al. Selenium treatment significantly inhibits tumor necrosis factor-alpha-induced cell death and tau hyperphosphorylation in neuroblastoma cells. Mol Med Rep. 2014;10:1869–1874.
- Lee M, McGeer E, McGeer PL. Activated human microglia stimulate neuroblastoma cells to upregulate production of beta amyloid protein and tau: implications for Alzheimer’s disease pathogenesis. Neurobiol Aging. 2015;36:42–52.
- Petroni D, Tsai J, Agrawal K, et al. Low-dose methylmercury-induced oxidative stress, cytotoxicity, and tau-hyperphosphorylation in human neuroblastoma (SH-SY5Y) cells. Environ Toxicol. 2012;27:549–555.
- Choi S, Oh JH, Kim H, et al. Protective effect of tat PTD-Hsp27 fusion protein on tau hyperphosphorylation induced by okadaic acid in the human neuroblastoma cell line SH-SY5Y. Cell Mol Neurobiol. 2015;35:1049–1059.
- Monroy-Ramirez HC, Basurto-Islas G, Mena R, et al. Alterations in the nuclear architecture produced by the overexpression of tau protein in neuroblastoma cells. J Alzheimers Dis. 2013;36:503–520.
- Ikeda H, Taira N, Hara F, et al. The estrogen receptor influences microtubule-associated protein tau (MAPT) expression and the selective estrogen receptor inhibitor fulvestrant downregulates MAPT and increases the sensitivity to taxane in breast cancer cells. Breast Cancer Res. 2010;12:R43.
- Gervais FG, Xu D, Robertson GS, et al. Involvement of caspases in proteolytic cleavage of Alzheimer’s amyloid-beta precursor protein and amyloidogenic A beta peptide formation. Cell. 1999;97:395–406.
- Galvan V, Chen S, Lu D, et al. Caspase cleavage of members of the amyloid precursor family of proteins. J Neurochem. 2002;82:283–294.
- Schiff PB, Fant J, Horwitz SB. Promotion of microtubule assembly in vitro by taxol. Nature. 1979;277:665–667.
- Wiernik PH, Schwartz EL, Strauman JJ, et al. Phase I clinical and pharmacokinetic study of taxol. Cancer Res. 1987;47:2486–2493.
- Samadi N, Bekele RT, Goping IS, et al. Lysophosphatidate induces chemo-resistance by releasing breast cancer cells from taxol-induced mitotic arrest. PloS one. 2011;6:e20608.
- Snyder JP, Nettles JH, Cornett B, et al. The binding conformation of Taxol in beta-tubulin: a model based on electron crystallographic density. Proc Natl Acad Sci U S A. 2001;98:5312–5316.
- Arnal I, Wade RH. How does taxol stabilize microtubules? Current Biology: CB. 1995;5:900–908.
- Bouge AL, Parmentier ML. Tau excess impairs mitosis and kinesin-5 function, leading to aneuploidy and cell death. Dis Model Mech. 2016;9:307–319.
- Akinrinmade OA, Jordaan S, Hristodorov D, et al. Human MAP tau based targeted cytolytic fusion proteins. Biomedicines. 2017;5:36.
- Wu JM, DiPietrantonio AM, Hsieh TC. Mechanism of fenretinide (4-HPR)-induced cell death. Apoptosis. 2001;6:377–388.
- Jazvinscak Jembrek M, Hof PR, Simic G. Ceramides in Alzheimer’s disease: key mediators of neuronal apoptosis induced by oxidative stress and abeta accumulation. Oxid Med Cell Longev. 2015;2015:346783.
- Mielke MM, Haughey NJ, Bandaru VVR, et al. Cerebrospinal fluid sphingolipids, beta-amyloid, and tau in adults at risk for Alzheimer’s disease. Neurobiol Aging. 2014;35:2486–2494.