ABSTRACT
Oxidative stress is considered as a major pathogenesis in myocardial damage; however, effective therapies are limited so far. The present study aimed to investigate the in vitro antioxidative mechanism of Catalpol in cardiomyocytes. The results indicated that Catalpol attenuated high glucose (HG)-induced apoptosis in mouse cardiomyocytes via significantly downregulating long noncoding RNA (lncRNA) nuclear paraspeckle assembly transcript 1 (Neat1) expression. Furthermore, Catalpol downregulated Neat1 expression and attenuated apoptosis by inhibiting production of intracellular reactive oxygen species (ROS) in HG-treated cardiomyocytes. Moreover, Catalpol also suppressed HG-induced degradation of IκBα and the nuclear localization of nulear factor-κB (NF-κB) by decreasing the intracellular ROS levels. Additionally, chromatin immunoprecipitation (ChIP) and dual-luciferase activity assays validated that NF-κB bound to Neat1 promoter to activate Neat1 expression. In summary, these results implied that Catalpol protected mouse cardiomyocytes against oxidative injury at least partly through ROS-NF-κB-Neat1 axis.
Introduction
Diabetic cardiomyopathy (DCM) is one of the most common causes of disability and death associated with myocardial metabolic abnormalities provoked by diabetes mellitus [Citation1]. Oxidative stress caused by hyperglycemia plays a crucial role in the pathogenesis of DCM. To the best of our knowledge, oxidative stress induced by the excessive generation of reactive oxygen species (ROS) results in aberrant signaling cascades that triggers myocardial apoptosis, leading to cardiac fibrosis and remodeling [Citation2,Citation3]. Therefore, the inhibition of oxidative stress is of great importance to the treatment of DCM.
Catalpol is an iridiod glucoside that is predominantly derived from the roots of Rehmannia glutinose. As previously reported, Catalpol exerted cardioprotection against myocardial ischemia/reperfusion (I/R) injury by reducing peroxynitrite formation [Citation4]. Catalpol was also shown to produce potential beneficial effects, including antihyperglycemic, antidiabetic and antioxidant activities in streptozotocin (STZ)-induced diabetic rats [Citation5,Citation6]. Recently, Choi et al revealed that Catalpol suppressed inflammatory response induced by advanced glycation end-products (AGEs) by decreasing ROS-NF-κB pathway in human monocytic THP-1 cells [Citation7]. However, the role of Catalpol in the development of DCM remains unclear.
Long noncoding RNA (LncRNA), which are defined as a novel class of RNA transcripts more than 200 nucleotides with narrow protein coding functions, are involved in regulating various cell biological processes, containing cell growth, differentiation and apoptosis [Citation8]. Accumulating evidence has strongly implied that several lncRNAs play vital roles in the occurrence and development of DCM. For example, Zhou et al reported that lncRNA myocardial infarction-associated transcript (MIAT) functioned as a competing endogenous RNA (ceRNA) to upregulate DAPK2 expression by sponging miR-22-3p, and subsequently promoted cardiomyocyte apoptosis in the pathogenesis of DCM [Citation9]. Yang et al elucidated that that knockdown of lncRNA KCNQ1OT1 alleviated high glucose (HG)-induced cardiac pyroptosis by targeting miR-214-3p and caspase-1 in DCM [Citation10]. LncRNA nuclear paraspeckle assembly transcript 1 (Neat1) is located at 11q13.1 locus, which has been reported to be associated with many disorders, particularly those related to cardiovascular disease (CVD). Ahmed et al found that Neat1 promoted the proliferation and migration of vascular smooth muscle cells (VSMCs) via sequestering the key chromatin modifier WD Repeat Domain 5 (WDR5) from SM-specific gene loci [Citation11]. Ma et al indicated that silencing of Neat1 alleviated myocardial I/R injury by inhibiting myocardial apoptosis and autophagy in STZ-induced diabetic rats [Citation12]. However, the expression and mechanisms of Neat1 in DCM are still unknown. A recent study found that NF-κB might bind to Neat1 promoter to activate Neat1 expression after lipopolysaccharide (LPS)-stimulated p65 nucleus translocation in lung adenocarcinoma cells [Citation13].
Thus, it was logical to speculate that the cardioprotective effect of Catalpol treatment was mediated by suppressing the ROS-NF-κB pathway-mediated Neat1 downregulation in the progression of DCM. The results might provide a novel insight into potential cardioprotective therapies for DCM using Catalpol.
Materials and methods
Isolation and culture of mouse cardiomyocytes
Newborn C57BL/6J mice purchased from Center for Animal Experiment of First Affiliated Hospital, Heilongjiang University of Chinese Medicine were housed individually in an air-conditioned room maintained at a temperature of 22°C ± 2°C and 12-/12-h light/dark cycle and were fed a normal rodent diet. Myocardial tissues were obtained, minced and re-suspended overnight at 4°C in HEPES-buffered saline solution. The suspension was digested in 0.15% collagenase/dispase mixture (Roche Applied Science, Mannheim, Germany) for 20 min. Dulbecco’s modified Eagle medium (DMEM; Life Technologies, Carlsbad, CA, USA) containing 10% fetal bovine serum (FBS; Gibco, Carlsbad, CA, USA) was subsequently added to terminate the digestion. The harvested primary cardiomyocytes were cultured in DMEM supplemented with 10% FBS, 100 g/ml penicillin/streptomycin, and 2 mM glutamine (all from Life Technologies). All animal procedures and protocols were approved by the Ethics Committee of the First Affiliated Hospital, Heilongjiang University of Chinese Medicine.
Cell treatment
Mouse cardiomyocytes were exposed to DMEM containing 5.5 mM D-glucose (normal glucose; NG), 5.5 mM D-glucose plus 27.5 mM D-mannitol (high mannitol, HM) or 33 mM D-glucose (high glucose; HG) or together with low (1 mg/ml), middle (2 mg/ml), high dose (4 mg/ml) of Catalpol which was obtained from the National Institute for the Control of Pharmaceutical and Biological Products (Beijing, China) for 24 h following 72 h treatment with ROS scavenger N-acetyl-L-cysteine (NAC, 10 mM) or ROS donor xanthine oxidase/hypoxanthine (XO/HX, 50 mM) (all from Sigma-Aldrich, St. Louis, MO, USA).
Cell transfection
Plasmid for NF-κB overexpression was constructed by inserting the amplified cDNA into pcDNA 3.1 vector (Invitrogen, Shanghai, China), resulting in pcDNA-NF-κB. Small interfering RNAs (siRNAs) targeting Neat1 or NF-κB as well as negative control siRNAs (si-Ctrl) were designed and synthesized by Shanghai GenePharma Co., Ltd. (Shanghai, China) for knockdown experiments. For cell transfection, mouse cardiomyocytes were seeded in 96-well plates and pre-incubated overnight in antibiotics-free DMEM, until 70% confluence and transfected with plasmids or siRNAs (50 nM) using Lipofectamine 2000 (Invitrogen) following the manufacturer’s instructions. Transfected cells were cultured for another 48 h prior to treatment with 33 mM D-glucose alone or together with 4 mg/ml Catalpol.
Chromatin immunoprecipitation (chIP)
Primary cardiomyocytes transfected with si-Neat1 or si-Ctrl were fixed with 1% paraformaldehyde and then sheared to approximately 400 bp DNA. Chromatin DNA was immunoprecipitated using antibodies against p65 or normal IgG (Abcam) as a negative control. The immunoprecipitated DNA was applied for PCR amplification by using primers for NF-κB p65.
Luciferase reporter assay
A wild-type and mutated Neat1 promoter (WT-Neat1 and Mut-Neat1 containing any or both of the two predicted sites of the p65-responsive elements) luciferase reporter gene vectors were constructed and co-transfected into HEK-293 cells (American Type Culture Collection, ATCC, Manassas, VA, USA) using Lipofectamine 2000. Luciferase assay was performed 48 h after transfection using the Dual-Luciferase Reporter Assay System (Promega, Madison, WI, USA) according to the manufacturer’s instructions.
Quantitative RT-PCR analysis
Total RNA was extracted using Trizol reagent (Invitrogen) and reversely transcribed to cDNA. Neat1 expression was measured by real-time PCR using SYBR Green I real-time PCR kit (CoWin Bioscience Co., Beijing, China) on an ABI 7500 Real-Time PCR system (Applied Biosystems, Carlsbad, CA, USA).
TUNEL staining
Cells were fixed with 4% paraformaldehyde for 15 min, permeabilized in 0.25% Triton-X 100, and prepared for terminal deoxynucleotidyl transferase dUTP nick end labeling (TUNEL) reaction according to the manufacturer’s instructions (Roche Applied Science) for 45 min. The coverslips were mounted onto glass slides and examined by a scanning microscope.
Western blot
Mouse cardiomyocytes were lysed in Radio-Immunoprecipitation Assay buffer (Santa Cruz Biotechnology, Santa Cruz, CA, USA), loaded on 10% sodium dodecyl sulfate-polyacrylamide gel electrophoresis (SDS-PAGE) and transferred onto polyvinylidene fluoride (PVDF) membranes (Millipore, Bedford, MA, USA). After blocking with 5% skim milk, the blots were probed with primary antibodies against caspase-3 (#9665), Bax (#5023), Bcl-2 (#4223), IκBα (#9242), β-actin (#4970), p65 (#8242) and Lamin B (#13,435) (1:1000 dilution; all from Cell Signaling Technology, Boston, MA, USA) overnight at 4°C and subsequently incubated with horseradish peroxidase (HRP)-conjugated secondary antibody (1:2000 dilution; Abcam, Cambridge, UK). Enhanced chemiluminescence reagent (Beckman Colter, Brea, CA, USA) was used to visualize signals. β-actin and Lamin B were used for endogenous control.
Immunofluorescence
The translocation of NF-κB in cardiomyocytes was determined by immunofluorescent staining. Briefly, mouse cardiomyocytes were fixed in 4% paraformaldehyde for 10 min, washed with PBS and lysed in 0.1% Triton X-100 for 5min. After overnight incubation with anti-p65 (#8242; 1:400 dilution; Cell Signaling Technology), cells were stained with FITC-conjugated anti-rabbit secondary antibody (1:200 dilution) for 1 h at room temperature. Nuclei were stained with DAPI. The slides were examined by confocal spectral microscope (Leica Microsystems, Wetzlar, Germany).
Measurement of intracellular ROS
Mouse cardiomyocytes treated with NG or HG or together with Catalpol were harvested and incubated with dichlorofluorescin diacetate (DCFH-DA; Sigma-Aldrich) solution (10 µM) diluted in serum-free DMEM for 30 min at 37°C and washed with serum-free DMEM. Intracellular ROS accumulation was measured using spectrofluorophotometer (Shimadzu Corporation, Tokyo, Japan) at the wavelength 488/525 nm.
Statistical analysis
All data were represented as the mean ± standard deviation (SD). Comparisons between groups were examined by two-sided Student’s t test using the SPSS 22.0 (IBM, Armonk, NY, USA), besides, P < 0.05 was considered as statistically significant.
Results
Catalpol inhibited apoptosis of HG-stimulated cardiomyocytes by downregulating Neat1
As shown in ), Neat1 was highly expressed in HG-stimulated mouse cardiomyocytes, while Catalpol significantly decreased the expression of Neat1 in a dose-dependent manner. Catalpol effectively inhibited apoptotic death of cardiomyocytes, as evidenced by the decrease in the number of TUNEL-positive cells ()), and caspase-3 and Bax expression as well as the upregulation of Bcl-2 expression ()). Further experiments demonstrated that the overexpression of Neat1 promoted the apoptosis of cardiomyocytes in the existence of Catalpol. We further confirmed the underlying effects of Neat1 on HG-induced apoptosis of cardiomyocytes, and found that the downregulation of Neat1 led to the decrease of HG-induced apoptosis, which was associated with the reduction in TUNEL-positive cells ()), and caspase-3 and Bax expressions, and an increase in Bcl-2 expression ()). These results indicated that Catalpol suppressed HG-induced apoptosis in cardiomyocytes through decreasing Neat1 expression.
Figure 1. Effect of Catalpol on Neat1 expression and myocardial apoptosis. Neat1 expression (a) using qRT-PCR in mouse cardiomyocytes exposed to 5.5 mM D-glucose (NG), 5.5 mM D-glucose plus 27.5 mM D-mannitol (HM), 33 mM D-glucose (HG) or HG plus 1 mg/ml (HG+L), 2 mg/ml (HG+M) or 4 mg/ml (HG+H) Catalpol. Apoptotic index (b) and the protein levels of caspase-3, Bax and Bcl-2 (c) in mouse cardiomyocytes in the groups of NG, HG, HG+Catalpol (4 mg/ml), HG+Catalpol+Vector and HG+Catalpol+Neat1 were determined by TUNEL staining and western blotting.
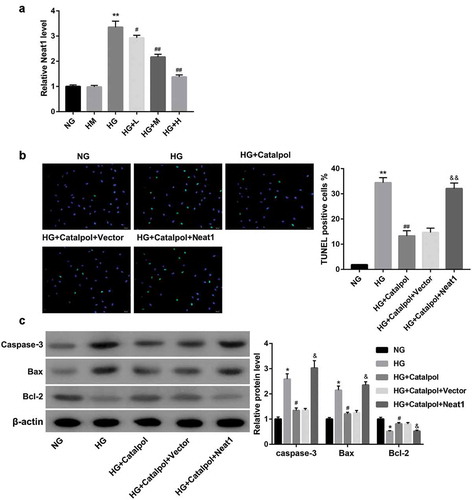
Catalpol downregulated Neat1 expression and attenuated apoptosis by inhibiting intracellular ROS production in HG-treated cardiomyocytes
Several lines of evidence suggest that Catalpol modulates ROS generation in diverse cells [Citation14,Citation15]. As such, we evaluated the potential of Catalpol to disrupt the oxidative stress threshold in HG-induced cardiomyocytes. The intracellular ROS level was determined using DCFH-DA. As shown in ), HG induced the excessive release of ROS from mouse cardiomyocytes, whereas treatment with Catalpol (4 mg/ml) for 24 h had inhibitory effects on HG-induced ROS production. To further determine whether the cardioprotective effect of Catalpol was due to a reduction in ROS production, ROS scavenger NAC and ROS donor XO/HX were added to culture medium followed by Catalpol treatment. It turned out that NAC aggravated Catalpol-induced Neat1 downregulation ()), the decrease in myocardial apoptosis index ()) and the protein expressions of caspase-3 and Bax and the increase in Bcl-2 expression ()), which were all attenuated by XO/HX incubation. These data suggested that Catalpol downregulated HG-induced Neat1 expression and attenuated HG-induced apoptosis in cardiomyocytes by inhibiting ROS levels.
Figure 3. Catalpol downregulated HG-induced Neat1 expression and attenuated HG-induced apoptosis by inhibiting ROS levels. (a) Intracellular ROS levels in mouse cardiomyocytes in NG, HG and HG+Catalpol (4 mg/ml) groups using DCFH-DA staining. **P < 0.01 vs. NG group; ##P < 0.01 vs. HG group. n = 3. Neat1 expression (b), Apoptotic index (c) and the protein levels of caspase-3, Bax and Bcl-2 (d) in mouse cardiomyocytes treated with HG and/or Catalpol for 24 h or together with NAC (10 mM) or XO/HX (50 mM) for 72 h. *P < 0.05, **P < 0.01 vs. HG group; #P < 0.05, ##P < 0.01 vs. HG+Catalpol group. n = 3.
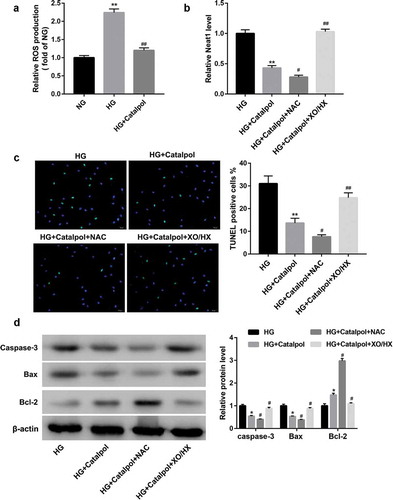
Catalpol inhibited the transcriptional activity of NF-κB by decreasing the intracellular ROS levels
A recent work uncovered that Catalpol could suppress the degradation of IκBα and the nuclear localization of NF-κB induced by AGEs in human monocytic THP-1 cells[Citation7], we thus considered the possibility that Catalpol-induced cardioprotective effects was attributable to the suppression of NF-κB activation. As illustrated in ), the total protein levels of IκBα were downregulated, while nucleus p65 protein levels were upregulated in HG-treated mouse cardiomyocytes, which were reversed by Catalpol. Immunofluorescence confocal microscopy further revealed that Catalpol treatment abolished the nuclear translocation of the NF-κB p65 subunit induced by HG ()). Besides, Catalpol-induced IκBα accumulation, p65 inactivation ()) and the decreased nuclear translocation of NF-κB p65 ()) were promoted by NAC, but were abrogated by XO/HX. Collectively, Catalpol inhibited the transcriptional activity of NF-κB by repressing HG-induced ROS production in mouse cardiomyocytes.
Figure 4. Catalpol inhibited the transcriptional activity of NF-κB by decreasing the intracellular ROS levels. The protein levels of IκBα and nucleus p65 (a), the nuclear translocation of p65 (b) detected by immunofluorescence assays in mouse cardiomyocytes in NG, HG and HG+Catalpol (4 mg/ml) groups. **P < 0.01 vs. NG group; ##P < 0.01 vs. HG group. n = 3. The protein levels of IκBα and nucleus p65 (c), the nuclear translocation of p65 (d) in mouse cardiomyocytes treated with HG and/or Catalpol for 24 h or together with NAC (10 mM) or XO/HX (50 mM) for 72 h. **P < 0.01 vs. HG group; ##P < 0.01 vs. HG+Catalpol group. n = 3.
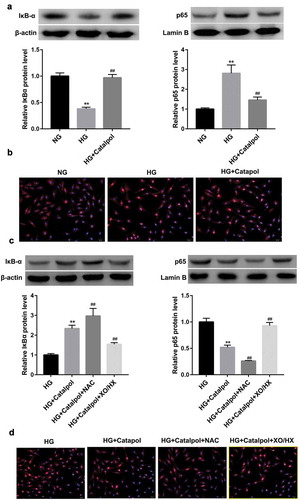
NF-κB bound to the neat1 promoter to activate Neat1 expression
We next investigated whether Catalpol downregulated Neat1 expressing by blocking p65 nucleus translocation. Mouse cardiomyocytes were transfected with NF-κB or si-NF-κB vector in the presence of HG and Catalpol stimulation, and the expression of Neat1 was determined by qRT-PCR. The results manifested that NF-κB overexpression partially attenuated, while si-p65 transfection amplified the inhibitory effect of Catapol on Neat1 expression ()). We then validated the predicted binding between NF-κB and Neat1 promoter region using ChIP assay. The levels of NF-κB p65 antibody binding to the predicted sites in Neat1 promoter region were higher than those binding to IgG. Moreover, Neat1 knockdown markedly downregulated NF-κB p65 expression compared to si-Ctrl group ()). Furthermore, as shown by luciferase reporter gene assay, Neat1 overexpression observably amplified, but Neat1 silencing significantly abolished the luciferase activity of WT‐Neat1 or mutated Neat1 containing any of the two putative binding elements ()), suggesting that NF-κB signaling might bind to the predicted binding site 1 and site 2 within Neat1 promoter to activate Neat1 expression in mouse cardiomyocytes.
Figure 5. The interaction between NF-κB and Neat1 in mouse cardiomyocytes. (a) Neat1 expression in mouse cardiomyocytes transfected with Vector, NF-κB, si-Ctrl and si-NF-κB following treatment with HG and Catalpol. **P < 0.01 vs. vector group; ##P < 0.01 vs. si-Ctrl group. n = 3. (b) The real-time ChIP assay showed that the level of p65 antibody binding to Neat1 promoter was much greater than that of IgG. **P < 0.01 vs. IgG+si-Ctrl group; ##P < 0.01 vs. IgG+si-Neat1 group. n = 3. (c) A schematic diagram of potential p65 binding element (two possible binding sites) in the promoter region of Neat1. A WT-Neat1 promoter luciferase reporter vector and a mut-Neat1 promoter luciferase reporter vector constructed by mutating any or both of the predicted binding sites were transfected into HEK293 cells and then transfected with vector, Neat1, si-Ctrl and si-Neat1; the luciferase activity was determined. **P < 0.01 vs. Vector or si-Ctrl group. n = 3.
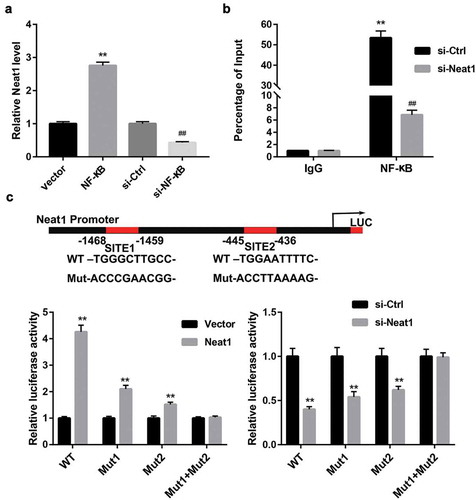
Discussion
DCM is characterized by ventricular dysfunction and structural abnormalities, which is commonly caused by disordered glucose metabolism[Citation1]. As a hallmark of DCM, abnormal cardiomyocyte apoptosis is closely associated with the increased blood glucose levels in both the development and subsequent progression of DCM[Citation16]. Excess cardiomyocyte apoptosis leads to the loss of contractile units, which consequently reduces cardiac function and triggers cardiac remodeling and hypertrophy[Citation17]. In the present study, when primary cultured neonatal mouse cardiomyocytes were exposed to 33 mmol/L HG over 24 h, TUNEL-positive staining apoptotic cells were significantly increased. Since apoptosis is tightly controlled by a series of regulatory proteins, we further examined the protein levels of pro-apoptotic proteins caspase-3 and Bax and anti-apoptotic proteins Bcl-2. As expected, the highly expressed caspase-3 and Bax and decreased Bcl-2 levels were observed in the mouse cardiomyocytes exposed to HG.
Catalpol, a bioactive component of Rehmannia glutinosa, is a novel drug candidate against myocardial injury for the treatment of cardiovascular diseases. Hu et al indicated for the first time that Catalpol pretreatment could protect against H2O2-induced H9C2 cell apoptosis through modulating mitochondrial-dependent caspase pathway[Citation18]. Lin et al discovered that Catalpol inhibited apoptosis and oxidative stress in glucose-deprived H9C2 cells[Citation19]. Bi et al manifested Catalpol afforded cardioprotective effects on isoproterenol (ISO)-induced cardiomyocyte apoptosis, at least in part, through apelin/APJ signaling pathway[Citation20]. In the present study, Catalpol treatment inhibited HG-induced cardiomyocyte apoptosis as indicated by a decrease in the TUNEL-positive cell ratio, a decline in caspase-3 activity and alterations in the Bax and Bcl-2 expression levels. More importantly, Catalpol treatment concentration-dependently decreased the expression of Neat1 in HG-treated mouse cardiomyocytes. Transfection experiments confirmed that Neat1 had a pro-apoptotic effect in HG-induced cardiomyocytes. These results concluded that Catalpol might be a promising candidate against cardiomyocyte apoptosis induced by HG by significantly downregulating Neat1 expression.
Oxidative stress is attributed to the imbalance between the antioxidant defense and intracellular oxidative actions, which further results in DNA damage, apoptosis processes and tissue injury[Citation21]. In recent years, several researches have proposed that Catalpol plays a strong antioxidative role for the treatment of cancers [Citation22,Citation23], atherosclerosis[Citation24], and neurodegenerative diseases [Citation15,Citation25]. Here, in our study, high levels of ROS production were determined in HG-treated mouse cardiomyocytes. On the contrary, Catalpol negatively regulated Neat1 expression and cell apoptosis. ROS has been widely explored in regulating apoptotic response. Hence, we supposed that Catalpol-induced apoptosis inhibition was associated with decreased ROS generation. In order to verify this hypothesis, the ROS scavenger NAC and activator XO/HX were used. Obviously, NAC aggravated and XO/HX application reversed Catalpol-induced Neat1 downregulation and apoptosis inhibition. The findings above indicated that Catalpol downregulated Neat1 expression and inhibited apoptosis by producing antioxidative effects in mouse cardiomyocytes.
The transcription factor NF-κB is a central regulator of various cellular genes involved in oxidative stress and apoptosis. Increased ROS levels further activates NF-κB to regulate the expression of genes controlling cardiac apoptosis [Citation26,Citation27]. Catalpol has been reported to suppress the AGE-induced ROS production through mainly interrupting the activation of the NF-κB signaling pathway in human monocytic THP-1 cells[Citation7]. Consistent with these findings, our results showed that upon the stimulation of HG, p65 nucleus translocation was significantly increased, which could be attenuated by Catalpol treatment, indicating that Catalpol diminished HG-mediated degradation of IκBα and nuclear localization of NF-κB p65. Our findings further included that NF-κB could bind to Neat1 promoter to upregulate its transcriptional activity as evidenced by the results from ChIP and luciferase reporter gene assays.
In conclusion, these results suggested that the potential cardioprotective capacity of Catalpol was at least partly, mediated through negative regulation of the ROS-NF-κB-Neat1 signaling pathway.
Disclosure statement
No potential conflict of interest was reported by the authors.
Additional information
Funding
References
- Jia G, Whaley-Connell A, Sowers JR. Diabetic cardiomyopathy: a hyperglycaemia- and insulin-resistance-induced heart disease. Diabetologia. 2018;61:21–28.
- Lu Z, Chuan-Bo S, Chao L, et al. Upregulation of arginase activity contributes to intracellular ROS production induced by high glucose in H9c2 cells. Int J Clin Exp Pathol. 2015;8:2728–2736.
- Jiang P, Zhang D, Qiu H, et al. Tiron ameliorates high glucose-induced cardiac myocyte apoptosis by PKCdelta-dependent inhibition of osteopontin. Clin Exp Pharmacol Physiol. 2017;44:760–770.
- Huang C, Cui Y, Ji L, et al. Catalpol decreases peroxynitrite formation and consequently exerts cardioprotective effects against ischemia/reperfusion insult. Pharm Biol. 2013;51:463–473.
- Ja-Ping S, Kai-Chun C, Hsien-Hui C, et al. Plasma glucose lowering mechanisms of catalpol, an active principle from roots of Rehmannia glutinosa, in streptozotocin-induced diabetic rats. J Agric Food Chem. 2011;59:3747–3753.
- Zhu H, Wang Y, Liu Z, et al. Antidiabetic and antioxidant effects of catalpol extracted from Rehmannia glutinosa (Di Huang) on rat diabetes induced by streptozotocin and high-fat, high-sugar feed. Chin Med. 2016;11:25.
- Choi HJ, Jang HJ, Chung TW, et al. Catalpol suppresses advanced glycation end-products-induced inflammatory responses through inhibition of reactive oxygen species in human monocytic THP-1 cells. Fitoterapia. 2013;86:19–28.
- Chen LL, Zhao JC. Functional analysis of long noncoding RNAs in development and disease. Adv Exp Med Biol. 2014;825:129–158.
- Zhou X, Zhang W, Jin M, et al. lncRNA MIAT functions as a competing endogenous RNA to upregulate DAPK2 by sponging miR-22-3p in diabetic cardiomyopathy. Cell Death Dis. 2017;8:e2929.
- Yang F, Qin Y, Wang Y, et al. LncRNA KCNQ1OT1 mediates pyroptosis in diabetic cardiomyopathy. Cell Physiol Biochem. 2018;50:1230–1244.
- Ahmed ASI, Dong K, Liu J, et al. Long noncoding RNA NEAT1 (nuclear paraspeckle assembly transcript 1) is critical for phenotypic switching of vascular smooth muscle cells. Proc Natl Acad Sci U S A. 2018;115:E8660–E7.
- Min M, Jie H, Qi-Yin Z, et al. Long non-coding rna nuclear-enriched abundant transcript 1 inhibition blunts myocardial ischemia reperfusion injury via autophagic flux arrest and apoptosis in streptozotocin-induced diabetic rats. Atherosclerosis. 2018;277:113–122.
- Zhou W, Chen X, Hu Q, et al. Galectin-3 activates TLR4/NF-kappaB signaling to promote lung adenocarcinoma cell proliferation through activating lncRNA-NEAT1 expression. BMC Cancer. 2018;18:580.
- Wenna C, Ximing L, Lian-Qun J, et al. Neuroprotective activities of catalpol against CaMKII-dependent apoptosis induced by LPS in PC12 cells. Br J Pharmacol. 2013;169:1140–1152.
- Jing B, Bo J, Andrew Z, et al. Catalpol inhibits LPS plus IFN-γ-induced inflammatory response in astrocytes primary cultures. Toxicol in Vitro. 2013;27:543–550.
- Kuethe F, Sigusch HH, Bornstein SR, et al. Apoptosis in patients with dilated cardiomyopathy and diabetes: a feature of diabetic cardiomyopathy? Hormone Metab Res. 2007;39:672–676.
- Dhalla NS, Nobuakira T, Delfin RL, et al. Mechanisms of subcellular remodeling in heart failure due to diabetes. Heart Fail Rev. 2014;19:87–99.
- Hu LA, Sun YK, Zhang HS, et al. Catalpol inhibits apoptosis in hydrogen peroxide-induced cardiac myocytes through a mitochondrial-dependent caspase pathway. Biosci Rep. 2016;36:e00348.
- Lin C, Lu Y, Yan X, et al. Catalpol protects glucose-deprived rat embryonic cardiac cells by inducing mitophagy and modulating estrogen receptor. Biomed Pharmacothe. 2017;89:973–982.
- Bi F, Xu Y, Sun Q. Catalpol pretreatment attenuates cardiac dysfunction following myocardial infarction in rats. Anatol J Cardiol. 2018;19:296–302.
- Bai J, Yu N, Mu H, et al. Histidine protects human lens epithelial cells against H2 O2 -induced oxidative stress injury through the NF-small ka, CyrillicB pathway. J Cell Biochem. 2018;119:1637–1645.
- Wang ZH, Hu ZS. Catalpol inhibits migration and induces apoptosis in gastric cancer cells and in athymic nude mice. Biomed Pharmacother. 2018;103:1708–1719.
- Wang L, Xue GB. Catalpol suppresses osteosarcoma cell proliferation through blocking epithelial-mesenchymal transition (EMT) and inducing apoptosis. Biochem Biophys Res Commun. 2018;495:27–34.
- Zhang Y, Wang C, Jin Y, et al. Activating the PGC-1alpha/TERT pathway by catalpol ameliorates atherosclerosis via modulating ROS production, DNA damage, and telomere function: implications on mitochondria and telomere link. Oxid Med Cell Longev. 2018;2018:2876350.
- Huang JZ, Wu J, Xiang S, et al. Catalpol preserves neural function and attenuates the pathology of Alzheimer’s disease in mice. Mol Med Rep. 2016;13:491–496.
- Zhong P, Wu L, Qian Y, et al. Blockage of ROS and NF-kappaB-mediated inflammation by a new chalcone L6H9 protects cardiomyocytes from hyperglycemia-induced injuries. Biochim Biophys Acta. 2015;1852:1230–1241.
- Wei C, Li L, Kim IK, et al. NF-kappaB mediated miR-21 regulation in cardiomyocytes apoptosis under oxidative stress. Free Radic Res. 2014;48:282–291.