ABSTRACT
Osteosarcoma (OS) is the most common primary malignancy of bone that mostly affects children, adolescents, and young people. Despite advances have been made in multimodal therapy of OS, the long-term survival rate has reached a plateau, and the main obstacles are bad response to chemotherapy and gained chemoresistance. In this study, we tested the therapeutic effect of a newly reported drug, DMAMCL, on OS. Five human OS cell lines (143B, MNNG, MG63, Saos-2, U-2OS), and the mouse fibroblast cell line (NIH3T3) and human retinal epithelial cell (ARPE19) were used. The anti-tumor effect of DMAMCL was studied by MTS assay or IncuCyte-Zoom (in vitro), and Xenograft-mice-model (in vivo). Changes of cell cycle, apoptotic cells, caspase3/7 activities, and stemness after DMAMCL treatment were investigated. BAX siRNAs were used to knockdown the expression of BAX. Expressions of CyclinB1, CDC2, BCL-2 family, PARP, CD133, and Nanog were measured by Western Blotting. DMAMCL-induced dose-dependent OS cell death in vitro, and suppressed tumor growth and extended the survival of xenograft-bearing mice. DMAMCL-induced G2/M phase arrest in vitro, and apoptosis both in vitro and in vivo. Down-regulation of BAX expression attenuated the DMAMCL-induced OS cell death in vitro. We also found that DMAMCL inhibited the stemness in OS cells. These results indicated that DMAMCL possess therapeutic value in OS and may be a promising candidate for the new drug discovery for OS therapy.
Introduction
Osteosarcoma (OS) is the most common primary malignancy of bone that mostly affects children, adolescents, and young people [Citation1]. The predilection site of osteosarcoma is metaphysis of long bone, such as the distal femur and proximal tibia [Citation2]. Osteosarcoma has a great tendency to spread to lung which accounts for over 85% of the metastatic disease, nearly 15%-20% of patients will have clinically detectable metastases at presentation [Citation1,Citation3]. Although advances in multimodal therapy, including multi-agent chemotherapy and surgery, have been made since late 1970 s [Citation3,Citation4], the long-term survival rate has reached a plateau. Osteosarcoma is still the second leading cause of cancer-related death in children and young adults [Citation2]. The 5-y survival rate of patients with localized disease remains at 60-70% [Citation5–7], even worse, the rate of patients with metastatic and recurrent disease is less than 20% [Citation8–10]. The primary causes considered for the poor prognosis include bad response to the chemotherapy and gained resistance to chemotherapy [Citation3,Citation11]. Therefore, it is urgent to identify novel drugs for osteosarcoma treatment.
Micheliolide (MCL) is a guaianolide sesquiterpene lactone isolated from Micheliacompressa and Micheliachampaca, which can also be synthesized from Parthenolide and more stable than the natural product [Citation12]. Recently, MCL has been reported to selectively inhibit the growth of Acute Myelocytic Leukemia (AML) stem cells and progenitor cells, cisplatin resistance breast cancer cells, glioma cells, and attenuate carcinogenesis in AOM/DSS-induced colitis-associated cancer [Citation12–15]. Dimethylaminomicheliolide (DMAMCL), the dimethylamino Michael adduct of MCL, which releases MCL as a metabolite slowly but continuously in plasma, exhibits better pharmacokinetic properties. Importantly, DMAMCL shows remarkable therapeutic efficacy in the AML glioma and rhabdomyosarcoma mice models with very low acute toxicity [Citation13,Citation16,Citation17]. These findings indicate that DMAMCL is a promising drug for cancer therapy. To date, the effects of DMAMCL on osteosarcoma have not been reported.
Considering the predilection site and the epidemiological characteristics of osteosarcoma, some researchers speculate there is a correlation between the rapid bone growth and osteosarcoma development [Citation6]. During the past years, emerging evidence has placed mesenchymal stem cells (MSCs) and/or their direct lineage progenitors as the most likely cell-of-origin for many types of sarcomas including OS [Citation2,Citation7,Citation8,Citation18,Citation19]. Some researchers recognize osteosarcoma as a de-differentiation disease caused by genetic and epigenetic changes that interrupt osteoblast differentiation from mesenchymal stem cells [Citation7,Citation20]. Meanwhile, increasing evidence has indicated that osteosarcoma contains a hierarchy of cells defined as cancer stem cells (CSCs) or side population (SP) cells which possess the stem cell-like properties and responsible for metastasis, recurrence, and resistance to therapy [Citation2,Citation11,Citation21]. Thus, targeting CSCs in osteosarcoma may be essential in the treatment of osteosarcoma patients.
In this study, we investigated the anti-tumor growth effects of DMAMCL in vitro and in vivo, and studied its potential target gene in vitro, and also detected its inhibition on stemness in OS cells. Results show that DMAMCL induces tumor growth inhibition and cell apoptosis of OS cells in vitro and in vivo, as well as has cell stemness inhibition effect on OS cell lines, and down-regulation of BAX expression attenuated the DMAMCL-induced OS cell death. Our study gives evidence that DMAMCL possess potential therapeutic effects on osteosarcoma.
Materials and Methods
Regents
DMAMCL was kindly gifted by Dr. Yue Chen (College of Pharmacy, The State Key Laboratory of Elemento-Organic Chemistry, and Tianjin Key Laboratory of Molecular Drug Research, Nankai University, Tianjin, China). 3-(4,5-dimethylthiazol-2-yl)-5-(3-carboxymethoxyphenyl)-2-(4-sulfophenyl)-2 H-tetrazolium, inner salt assay (MTS; inner salt assay) and Caspase-Glo 3/7 Assay Kit were purchased from Promega (America), RNase A and Propidium iodide (PI) were from Sigma (America), Annexin V-FITC Apoptosis Detection Kit was from Dojindo laboratories (Japan), the primary antibodies against PARP, BAK, BAX, BCL2, and MCL1 were from CST (America), antibodies against CyclinB1, CDC2, CD133, and Nanog were from Wanleibio (China), antibodies againstα-Tubulin was from Biotechnology (China), goat anti-rabbit antibody and goat anti-mouse antibody were from Beijing Zhongshan Golden Bridge Biotechnology Co. Ltd (China), Lysis buffer and Hoechst33342 Staining Solution for Living Cells were from Beyotime (China), TUNEL Apoptosis Assay Kit was from Roche (Switzerland), Giemsa Stain solution (10× Stock solution) was from Solarbio (China), Matrigel was from BD (America), DMEM medium, Fetal bovine serum, Glutamine, Antibiotics (penicillin 100 units/ml, streptomycin 100 μg/ml), and PBS were from Bioind (Israel), KnockOut™ Serum Replacement was from Gibco (America).
Cell lines and culture condition
Five human OS cell lines (143B, MNNG, MG63, Saos-2, U-2OS) were used in this study. MNNG, MG63, Saos-2, and U-2OS were purchased from Cell bank of the Chinese Academy of Sciences, 143B cell line was a kindly gift from Dr. Shuhong Luo (Department of Pathobiology, University of Illinois at Urbana-Champaign). The mouse fibroblast cell line (NIH3T3) and human retinal epithelial cell (ARPE19) were received from Dr. Carol J. Thiele (Cellular and Molecular Biology Section, Pediatric Oncology Branch, National Cancer Institute, National Institutes of Health, Bethesda, MD, USA). The cell lines were cultured in DMEM medium with 10% fetal bovine serum, 2 mM glutamine, and antibiotics at 37°C in 5% CO2 incubator. The culture medium for 143B was supplied with 0.015 mg/ml 5-bromo-2ʹ-deoxyuridine as the instructions of ATCC.
Cell survival analysis
To investigate the effect of DMAMCL on cell survival, osteosarcoma cells (143B, MNNG, MG63, Saos-2, U-2OS) and normal cells (NIH3T3 and ARPE19) were seeded into 96-well microtiter plates in triplicate and cultured overnight, then the cells were treated with DMAMCL at concentrations gradient from 0 to 30 μM for 72 h. The morphological changes were observed by the inverted microscope and Incucyte Zoom Live-Cell Imaging System from Essen Bioscience (Ann Arbor, MI, USA) which was set to scan every 4 h from 0 h to 72 h after treatment. By the end of the experiments, MTS reagent was added to each well and incubated according to the manufacturer’s protocol. The absorbance was detected by Microplate Reader (BIO-RAD, America) at 490 nm wavelength. The MTS data calculation was performed as described previously [Citation17,Citation22]. Cell confluence (%) was calculated and analyzed by using Incucyte Zoom software according to the phase-contrast images.
Cell cycle analysis
143B, MNNG, MG63, Saos-2, and U-2OS cells were seeded in 6-well plates or culture dishes and incubated overnight. Then, the cells were treated with different concentrations of DMAMCL (0, 10, 20, 30 µM) for 36 h. All the cells were harvested, washed twice with PBS, and resuspended and fixed with cold 70% ethyl alcohol at 4°C overnight. Then, the cells were washed again with PBS and then incubated with RNase A (100 μg/ml) and PI (50 μg/ml) at room temperature in the dark for 30 min. Then, the stained cells were analyzed by Flow cytometry (Becton, Dickinson and Company, America). The percentage of cells in each phases of cell cycle was analyzed by using the Software of the system (Becton, Dickinson and Company, America).
Caspase-3/7 activity assay
OS cells were seeded into 96-well plates in triplicate and cultured overnight, and then treated with DMAMCL (0, 20, 25 µM) for 24 h. Then, the caspase-3/7 activity was detected using Caspase-3/7 Assay Kit according to the manufacturer’s instructions. In brief, after the drug treatment, the Caspase-Glo 3/7reagent was added into the wells and cultured at room temperature in the dark for 1 h, then luminescence was detected by luminometer (Victor 3, BioTec, America).
Apoptosis analysis by flow cytometry
Three OS cell lines (143B, MG63, Saos-2) were treated with DMAMCL (0, 10, 20, 30 µM) for 24 h, and stained with Annexin V and PI, and then apoptotic cells were measured by Flow cytometry. Briefly, following the manufacturer’s instructions, all cells were harvested, washed twice with PBS, resuspended the cells in 1× Annexin V Binding Solution and diluted to 1 × 106 cells/ml, and transferred to new eppendorf tubes. Then, 5 µl Annexin V or PI staining solution or both of them were added to the eppendorf tubes and incubated at room temperature in the dark for 15 min, and additional 400 μl 1× Annexin V Binding Solution were added before analyzed by Flow cytometry (Becton, Dickinson and Company, America).
Hoechst 33342staining of nuclei for detection of apoptosis
OS cells (143B, MG63) were seeded into 24-well plates in triplicate and cultured overnight and then treated with DMAMCL (0, 20 µM) for 24 h. The Hoechst33342 staining solution was added into the medium with a final concentration of 10 µg/ml and incubated for 20 min at room temperature in the dark as the manufacturer’s recommendation. Cells were washed twice with PBS very gently before images were captured by Fluorescence microscope (Olympus, Tokyo, Japan) in randomly chosen fields around the center of each well. The counting principle was as follows, normal nuclei: uniform blue chromatin with organized structure, and apoptotic cells: bright fluorescent chromatin which is highly condensed or fragmented.
Colony formation assay
Single-cell suspension of 143B and MG63 OS cells was carefully prepared, and the cells were seeded into 6 cm culture dishes at the density of 2000 cells per dish. After 5-d culture with or without 5 µM-DMAMCL treatment until the single-cell colonies were visible to the naked eyes, the cells were fixed with methyl alcohol, and then dyed with 1× Giemsa working solution (diluted from 10× Stock solution with PBS) for 30 min at room temperature. The dishes with dyed-colonies were washed gently with water and dried before captured by microscope. The colonies were counted by Image J software.
Sphere formation assay
143B and MNNG cells in Logarithmic growth phase were carefully prepared to single-cell suspension, and then seeded into ultra-low adherent 6-well plates (Corning 3471, USA) at 1 × 104cells per well, and cultured in 3 mL DMEM medium supplemented with 10% Gibco KnockOut™ Serum Replacement instead of fetal bovine serum, 2 mM glutamine, and antibiotics at 37°C in 5% CO2 incubator. After 7-d culture with or without 10 µM-DMAMCL treatment, the pictures at the center of each dishes were captured and spheres were counted.
TUNEL assay
Apoptosis detection was performed using a TUNEL Apoptosis Assay Kit according to the manufacturer’s instructions. Briefly, paraffin-embedded slides of tumor samples from in vivo experiment were prepared, and then deparaffinized with xylene and ethanol, and rehydrated by proteinase K. Sections were rinsed twice and then incubated with TUNEL reaction mixture for 1 h at 37°C in humidified atmosphere in the dark. Apoptotic cells on the slides were observed, and images were captured under fluorescence microscope (Olympus, Tokyo, Japan) in randomly chosen fields.
Cell transfection
Small interfering RNAs (siRNAs) (Tongyong, Shanghai, China) were used to knockdown the expression of BAX. The sequences of siRNAs were: BAX siRNA #1: 5ʹ-CCAAGGUGCCGGAACUGAUTT-3ʹ, 5ʹ-AUCAGUUCCGGCACCUUGGTT-3ʹ; BAX siRNA #2: 5ʹ-GUGCCGGAACUGAUCAGAATT-3ʹ, 5ʹ-UUCUGAUCAGUUCCGGCACTT-3ʹ. 143B and MG63 cells were seeded into 6-well plate (1 × 105/well), and cultured overnight. The siRNAs were transfected into cells using jetPRIME (Polyplus Transfection, Illkirsch, France). After 16 h, cells were collected and seeded into 96-well plate for MTS assay or 6-well plate for western blotting, and treated with DMAMCL (15 μM) 72 h for MTS assay or (15 μM) 24 h for Western Blotting.
In vivo studies
The in vivo experiment was approved and conducted by the Animal Care and Use Committee of Chinese Medical University. Female mice (Beijing Huafukang Bioscience Co. Inc., China) at ages of 4–5 wk were housed in a standard animal laboratory with free access to water and food. 143B and MG63 cells were digested and washed twice with cold PBS, and resuspended uniform to the final concentration of 4 × 107 cells/ml in cold PBS and Matrigel (1:1, v/v). When tumors reached over the average volume of 100 mm3, the mice were randomly divided into 3 groups: Control group and two DMAMCL treated groups (75 mg/kg/day and 100 mg/kg/day). DMAMCL were given to mice once a day by oral gavage for 3 wk. To investigate the effect of DMAMCL on tumor growth, the tumor volume (V) was measured three times a week for 1 month by digital caliper, and calculated as L× W2/2 (L = length, millimeter; W = width, millimeter). To study the mice survival, we counted the days from the beginning of the treatment to the end of the experiment. The endpoint included natural mortality and euthanasia according to the ethics principles. For the PARP protein expression analysis and TUNEL Staining in the tumor tissues, we treated the mice bearing 143B and MG63 xenograft tumors with DMAMCL (100 mg/kg/day) for 2 wk and tumor tissues were harvested. All experiments involving animals were approved by the experimental Animal Ethical Committee of Shengjing Hospital of China Medical University (2014PS48 K).
Western Blotting
OS cells were cultured in 6-well plates, treated with DMAMCL (0, 10, 20 µM) for 24 h, washed with cold PBS, and harvested. Tumor tissue samples were harvested from the mice with OS xenograft tumors that were treated with DMAMCL for 1 wk. Total protein was extracted with Lysis Buffer (Beyotime, China) following the manufacturer’s protocol. Protein concentrations were quantified using the Bradford reagent (Beyotime, China) according to the manufacturer’s instruction. Equal amounts (30 μg) of total protein in each condition were loaded and separated by 10-12% gel (Bio-Rad, America) and then transferred to PVDF membrane (Millipore, USA). After blocking with 5% skim milk in TBST buffer, the membranes were incubated with primary antibody at 4°C overnight. The membranes were washed with TBST and incubated with the peroxidase-conjugated goat anti-rabbit (1:5000) or anti-mouse (1:5000) antibodies for 2 h at room temperature. The binds of target proteins were detected by the enhanced chemiluminescence (ECL) reagents (Thermo Scientific, USA).
Statistical Analyses
Means ± SD of independent experiments were analyzed by student’s T-test. P values less than 0.05 was considered as statistically significant. Data was analyzed by using SPSS software.
Results
DMAMCL inhibits OS cell proliferation in vitro
To determine the effect of DMAMCL on cell survival, five OS cell lines (143B, MG63, MNNG, Saos-2, U-2OS) and the mouse fibroblast cell NIH3T3 cells, which represent nontumorous cells, were treated with different concentrations of DMAMCL for 72 h, at the end point of the experiment, MTS assay was used to detect the cell survival (). Cell confluency (% of the surface area of cells) was dynamically detected and calculated using Incucyte Zoom live-cell imaging platform and its software by phase-contrast images (), and the end point’s cell confluency was calculated and analyzed, and was shown in histogram (supplemental ). shows that DMAMCL treatment induced a concentration-dependent cell death in these OS cells, while NIH3T3 cells were less sensitive to DMAMCL. The IC50 of DMAMCL in the OS cells ranged from 11.73 μM (143B) to 13.16 μM (Saos-2). The IC50 of DMAMCL were 25.24 μM in NIH3T3 cells and 42.01 μM in ARPE19 cells, which were approximately 2-fold and 4-fold of the OS cells. The Incucyte Zoom results () showed that the proliferation of OS cells was inhibited in a concentration-dependent way, which was similar to the results of MTS assay. is the typical morphological changes of OS cells after 72 h treatment with 15 μM DMAMCL. All these data indicated that DMAMCL induced dose-dependent cell death in OS cells.
Figure 1. DMAMCL suppresses OS cell proliferation in vitro. (a) Five OS cell lines (143B, MG63, MNNG, Saos-2, and U-2OS) and the mouse fibroblast cell line NIH3T3 cell and human retinal epithelial cell line ARPE19 cell were treated with different concentrations of DMAMCL for 72 h. Cell survival was detected by MTS assay. The percentage of surviving cells was calculated by normalizing the absorbance value of the treated cells by the absorbance value of the control cells within every cell line. Means and SD were shown. (b) The real-time evaluation of cell confluence after DMAMCL treatment in each cell line was performed by IncuCyte ZOOM. The points showed means and SD of three replicates. (c) The images of OS cell lines after treated with DMAMCL (0, 15 µM) for 72 h were shown, respectively
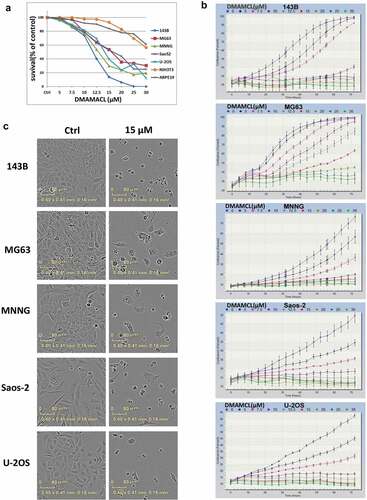
DMAMCL suppresses tumor growth in vivo
To investigate whether DMAMCL affects OS tumor growth in vivo, we treated the mice bearing 143B or MG63 xenograft tumors with DMAMCL at 75 mg/kg/day and 100 mg/kg/day doses by oral gavage for 3 wk. As shown in , the tumors grow lower in DMAMCL-treated groups than that in the control group. Compared to the tumor size of the control group, 100 mg/kg/day DMAMCL treatment significantly inhibited tumor growth in both 143B xenografts (P < 0.05) and MG63 xenografts (P < 0.05). Although the 75 mg/kg/day group did not show statistical significance, there was a trend of tumor growth inhibition at this dose. Kaplan-Meier survival curves showed a significant survival advantage in DMAMCL-treated xenografts compared to control group (). The median survival time for control group versus DMAMCL 75 mg/kg/day group and 100 mg/kg/day group was 18 d versus 24 d (P = 0.0417) and 29 d (P = 0.0118) in mice bearing 143B tumors; 29 d versus 37 d (P = 0.0026) and 37 d (P = 0.0039) in mice bearing MG tumors. These data indicated that DMAMCL had anti-tumor growth effect and extended the survival of mice bearing OS xenograft tumors.
Figure 2. DMAMCL suppresses OS tumor growth and increases tumor-bearing mice survival in vivo. Xenograft mice models were established by injecting 4 × 106 of OS cells (143B, MG63) into the right flank of 4–5-wk-old female nude mice. Mice bearing xenograft tumors were treated with DMAMCL (75 or 100 mg/kg/day) for 3 wk. (a) The tumor volume was measured three times a week for 4 wk, and calculated as L*W2/2 (L = length, millimeter; W = width, millimeter). At d 29, comparison of tumor volumes between control group and treatment group was shown. Means, SE, *, P < 0.05, DMAMCL-treated group vs control group. 143B (n = 9), and MG63 (n = 9). (b) Survival curve of the mice bearing xenograft OS tumors was plotted by Kaplan–Meier analysis
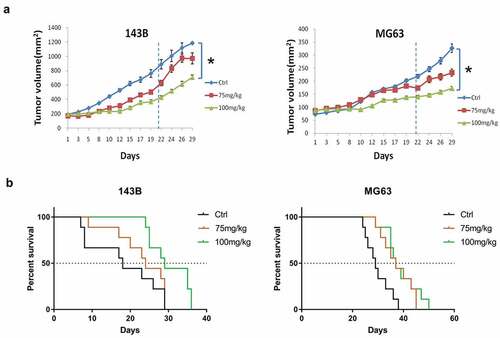
DMAMCL treatment of OS cells leads to G2/M arrest
To investigate how DMAMCL regulates cell proliferation, the cell cycle analysis was performed by flow cytometry after DMAMCL treatment (10 μM,20 μM,30 μM) for 36 h in all five OS cell lines. shows that with the increase of DMAMCL concentration there was an increase of cells in G2/M phase and then in SubG1 phase. The analysis of the cells in G2/M phase as shown in , the proportion of cells in G2/M phase increased at a maximum of 13.73% in 143B cells, 19.76% in MG63 cells, 10.50% in MNNG cells, 10.05% in Saos-2 cells, 22.49% in U-2OS cells. Despite an increase of cycline B1 was observed after DMAMCL treatment, we found that DMAMCL decreases CDC2, a component of cycline B1/CDC2 complex that required for G2/M progression, indicating DMAMCL treatment leads to a G2/M arrest through reducing the activity of cycline B1/CDC2 complex (). These data indicated that DMAMCL increases the G2/M proportion to suppress cell proliferation.
Figure 3. DMAMCL treatment of OS leads to a G2/M phase arrest. Five OS cell lines (143B, MG63, MNNG, Saos-2, and U-2OS) were treated with DMAMCL (10, 20, and 30 μM) for 36 h. The cells were stained with PI and analyzed by flow cytometry. The results are shown in histograms (a). The percentages of cells in G2/M phase (b) and sub-G1 phase (c) were indicated. Data represent means and SD of three independent experiments. * P < 0.05, ** P < 0.01 DMAMCL (+) vs. control. (d) Four OS cell lines (143B, MG63, MNNG, and U-2OS) were treated with DMAMCL (10 and 20 μM) for 24 h. The expressions of Cyclin B1 and CDC2 were detected by Western Blotting
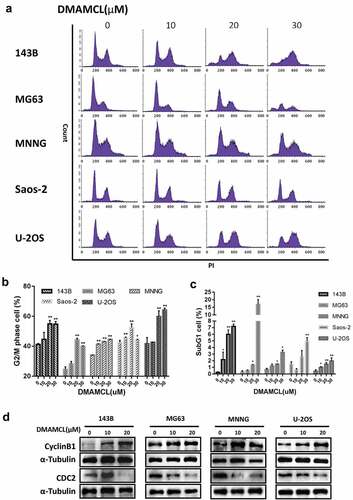
DMAMCL induces apoptosis of osteosarcoma in vitro and in vivo
We detected an increase of cells in the SubG1 phase after DMAMCL treatment ().To verify whether DMAMCL induces cell death via apoptosis, the OS cell lines (143B, MG63, Saos-2) were treated with DMAMCL (10 μM,20 μM,30 μM) for 24 h and then stained with Annexin V/PI to detect the apoptotic cells by flow cytometry. shows that the number of both the early and late apoptotic cells was increased in a dose-dependent manner after DMAMCL treatment. The proportion of early apoptotic cells increased from 1.45% (0 μM) to 21.25% (30 μM) in 143B cells, from 3.03% (0 μM) to 50.75% (30 μM) in MG63 cells, from 8.37% (0 μM) to 16.85% (30 μM) in Saos-2 cells. Consistently, the cell apoptosis induced by DMAMCL was further demonstrated by the significant increase of caspase 3/7 activity after DMAMCL treatment in the five OS cell lines (). Considering the cleavage of PARP by caspase-3and −7 has been thought to be a hallmark of apoptosis [Citation23–25], the expression of cleaved-PARP was studied after DAMACL treatment by Western Blotting. shows that there was an increase of cleaved-PARP expression in OS cells (143B, MG63, MNNG).
Figure 4. DMAMCL induces OS cell apoptosis in vitro. (a) Three OS cell lines (143B, MG63, and Saos-2) were treated with DMAMCL (10, 20, and 30 μM) for 24 h. Apoptotic cells were detected by flow cytometry with Annexin V/PI double staining. (b) Three OS cell lines (143B, MG63, and MNNG) were treated with DMAMCL (10 and 20 μM) for 24 h. The expressions of Cleaved-PARP (89KD) were detected by Western Blotting. (c) Five OS cell lines (143B, MG63, MNNG, Saos-2, and U-2OS) were treated with DMAMCL (20 and 25 μM) for 24 h. The caspase3/7 activity was detected. * P < 0.05, ** P < 0.01 DMAMCL (+) vs. control. Hoechst33342 staining was performed after cells treated with DMAMCL (20uM) for 24 h. (d) Typical pictures of 143B and MG63 cells were shown, and karyopyknosis (red arrow) and karyorrhexis (yellow arrow) was observed. (e) Histogram showed the percentage of apoptotic cells (143B, MG63, MNNG, Saos-2, and U-2OS). ** P < 0.01 DMAMCL (+) vs. control. (f) The expressions of BAK, BAX, MCL1, and BCL2 in three OS cell lines (143B, MG63, and MNNG) were detected by Western Blotting after 24-h treatment with DMAMCL (10 and 20 μM)
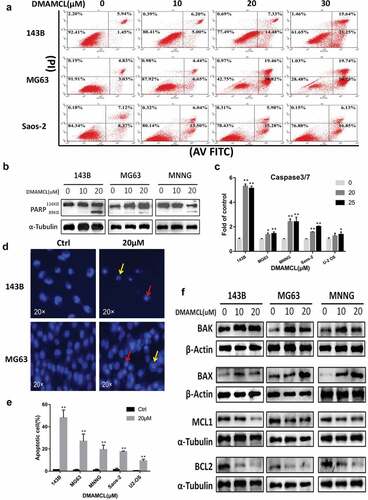
The Hoechst33342 staining was also performed in OS cell lines. The typical pictures and the statistical data are shown in and e. The increase of karyopyknosis (, red arrow) and karyorrhexis (, yellow arrow) was observed after OS cells treated with 20 μM-DMAMCL for 24 h. The number of the apoptotic cells increased significantly as shown in .
To further investigate how DMAMCL induced cell apoptosis, we evaluated the expressions of BCL-2 family members and found that the protein levels of pro-apoptotic molecules BAX and BAK increased, and the protein levels of anti-apoptotic molecules BCL2 and MCL1 decreased in OS cells after DMAMCL treatment (10 μM, 20 μM) for 24 h ().
BAX is a key effector of BCL-2 family members during apoptosis, and the expression of BAX significantly increased after DMAMCL treatment in OS cells. To further identify whether BAX is a target gene of DMAMCL, the expression of BAX was down-regulated by transfecting BAX siRNAs (#1 and #2) into OS cells (143B and MG63), and these cells were treated with DMAMCL (15 µM) for 72 h, then cell survival was evaluated by MTS assay. The expressions of BAX in the BAX siRNA transfected cells in the presence or absence of DMAMCL were detected by Western Blotting. The data showed that DMAMCL-induced increased expression of BAX could be blocked by transfecting BAX siRNAs (#1, #2) in both 143B cells and MG63 cells (). The MTS assay showed that compared to the DMAMCL-treated group, cell survival of DMAMCL+BAX-siRNA-treated groups significantly increased in 143B cells (DMAMCL-treated group: 19.85%, DMAMCL+BAX-siRNA#1-treated group: 46.45%, DMAMCL+BAX-siRNA#2-treated group: 28.76%) and MG63 cells (DMAMCL-treated group: 37.12%, DMAMCL+BAX-siRNA#1-treated group: 50.44%, DMAMCL+BAX-siRNA#2-treated group: 53.04%) ().These data indicated that down-regulation of BAX attenuated the DMAMCL-induced cell death in OS cells.
Figure 5. BAX mediates DMAMCL-induced OS cell death. (a) The expression of BAX in BAX-siRNAs-transfected OS cell lines (143B and MG63) was detected by Western Blotting after 24-h treatment with DMAMCL (15 μM). (b) The MTS assay was performed for BAX-siRNAs-transfected 143B and MG63 cells treated with DMAMCL (15 μM) for 72 h. ** P < 0.01 DMAMCL (+) vs. DMAMCL+BAX-siRNA (+)
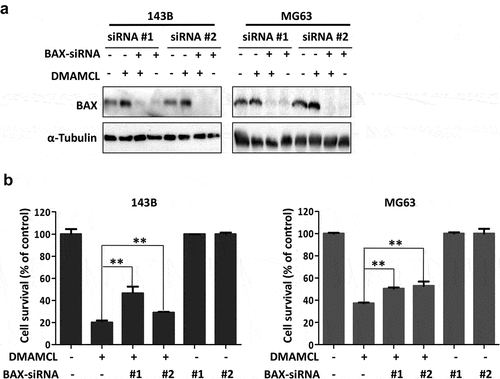
To investigate whether DMAMCL treatment induces apoptosis in vivo, we harvested the tumor tissues from OS xenografts after 2-wk treatment of DMAMCL for TUNEL staining and cleaved-PARP assay. We found that DMAMCL treatment leads to an increased number of cells with positive TUNEL staining and elevated protein levels of cleaved-PARP compared to control groups ().
Figure 6. DMAMCL induces OS cell apoptosis in vivo. Tumor samples were collected from 143B tumor-bearing mice and MG63 tumor-bearing mice after treated with DMAMCL (100 mg/kg/d) for 2 wk. (a) Representative images showed TUNEL staining (green) in tumor sections. Nuclei were stained with DAPI (blue). Scale bar: 10 μm. (b) The expressions of Cleaved-PARP in tumor tissue were detected by Western Blotting
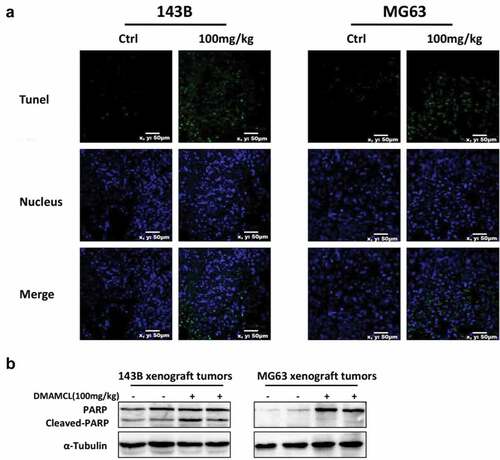
These data indicated that DMAMCL treatment induced apoptosis in OS cells in vitro and in OS xenograft tumor tissues in vivo, and BAX may be a target gene of DMAMCL in OS cells.
The effect of DMAMCL on the stemness of OS cells
In OS, CSCs and SP cells have been proved to responsible for metastasis, recurrence, and resistance to therapy [Citation2,Citation11,Citation21], and DMAMCL treatment induced cell death of CSCs in AML and breast cancers. In this study, we also investigated the effect of DMAMCL treatment on the stemness in OS cells. We first detected the expressions of CD133 and NANOG, markers for cancer stem cells, and found that 24-h DMAMCL treatment led to a decrease of the protein levels of CD133 and NANOG in OS cell lines (). The colony formation assay results showed that DMAMCL treatment leads to a ~ 70% decrease of the numbers of the colonies compared to control group (). Similarly, the sphere formation assay results showed that the DMAMCL treatment leads to an 80–90% decrease of the number of the spheres compared to the control group in different OS cell lines ().
Figure 7. DMAMCL decreases stemness of OS stem cell. (a) The expressions of CD133 and Nanog in three OS cell lines (143B, MG63, and MNNG) were detected by Western Blotting after 24-h treatment with DMAMCL (10 and 20 μM). (b) The Colony formation assay was performed for 143B and MG63 cells treated with DMAMCL (5 μM). Respective images were shown (left panel), and statistical analysis was done using Image J software (right panel). * P < 0.05, ** P < 0.01 DMAMCL (+) vs. control. (c) The Sphere formation assay was performed for 143B and MNNG cells treated with DMAMCL (10 μM). Respective images were shown (left panel), and statistical analysis was done using Image J software (right panel). ** P < 0.01 DMAMCL (+) vs. control
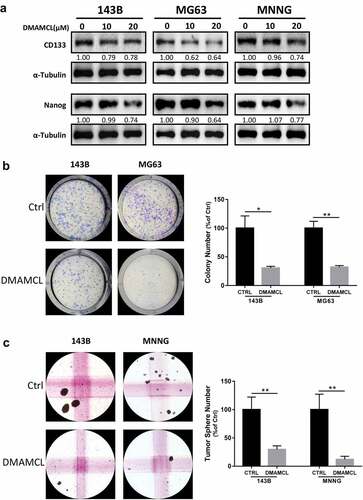
These data indicated that DMAMCL could decrease the stemness of OS stem cells to some extent at the cellular level.
Discussion
In this study, we find that the novel agent DMAMCL as a single drug induces cell apoptosis and cell cycle arrest at G2/M phase in OS cells in vitro. Moreover, we find that DMAMCL suppresses tumor growth and increases the overall survival of mice bearing OS xenograft tumors in vivo. Furthermore, DMAMCL treatment leads to a decrease of the number of stem-like OS cells based on colony formation assay and sphere formation assay.
Conventional chemotherapy and radiotherapy for cancers usually induce cell apoptosis by DNA damage and/or mitotic division inhibition. In normal cells, DNA damage response (DDR) and DNA repair mechanisms protect the cell from DNA damage-mediated death. Unfortunately, these mechanisms are inherited by tumor cells and even enhanced in CSCs [Citation14,Citation26,Citation27]. A large-scale screening program for antitumor agents was launched in1960 by the National Cancer Institute (USA), 35,000plant samples were evaluated. The extracts from plants rich in sesquiterpene lactones (SL) which possess the anti-cancer and anti-inflammatory properties had gained considerable interest, and some of them have been used in cancer clinical trials [Citation28]. Despite many large-scale screening of antitumor agents have been performed, until the year 2010, parthenolide (PTL) was thought to be the only small molecule that has been reported to selectively target several types of cancer stem cells, including AML stem cells and progenitor cells, breast cancer stem cells and prostate cancer stem cells [Citation29–32]. Parthenolide and its water-soluble Michael adduct, DMAPT (dimethylamino-parthenolide), had entered clinical trial for cancer therapy, although there were still some drawbacks. The DMAPT converts to PTL in vivo shortly after oral administration and PTL is unstable in both acidic and basic conditions [Citation12,Citation14,Citation28,Citation33]. Thus, the structurally related compound MCL/DMAMCL was developed recently, which is also a subtype of sesquiterpene lactones and can be synthesized from PTL. Further studies demonstrated that MCL/DMAMCL, like PTL, can also selectively inhibit AML stem or progenitor cell growth. DMAMCL, which can release MCL in the plasma continuously and stably, exhibited remarkable therapeutic efficacy in AML and rhabdomyosarcoma murine models [Citation13,Citation17]. It is reported that the drug/prodrug form of MCL/DMAMCL has at least three advantages over PTL/DMATP: higher stability of active drug, less toxic to normal cells and normal stem cells, and more sustainable release of active drug from prodrug [Citation14]. Therefore, it is reasonable to consider DMAMCL as a better candidate for the new drug development to overcome obstacle of cancer therapy. Up to date MCL or DMAMCL was reported to be effective against AML, colitis-associated cancer, glioma, and breast cancer in vitro and in vivo [Citation12–16,Citation34]. In this study, we find that DMAMCL induces cell apoptosis in OS, which is similar to the published data in other types of cancers.
Most studies reported that PTL, MCL, or DMAMCL induced cell death via apoptosis in different types of cancers, such as AML, breast cancer, glioma. Our study on DMAMCL in OS cells shows a similar cell death mechanism. BCL-2 family proteins play a pivotal role in mediating the apoptosis process [Citation25,Citation35,Citation36]. Previous studies discovered that DMAMCL and its analogues or prodrugs induced apoptosis in glioma cells, leukemia cells, and leukemia stem cells by regulating the expression of BCL-2 family members [Citation16,Citation29,Citation37]. In this study, we found increased expressions of BAX and BAK, and decreased expressions of BCL-2 and MCL-1 after DMAMCL treatment. Furthermore, we found that down-regulation of BAX expression by siRNAs transfection attenuated the DMAMCL-induced OS cell death. These data indicate that different BCL-2 family members may be involved in the DMAMCL-induced cell death based on different types of cancers, and BAX may be a target gene of DMAMCL in OS.
The mitotic cell cycle plays an important role in organism [Citation38]. There is evidence showing the relationship between apoptosis and cell cycle through some regulatory proteins in the cells, and manipulation of these regulatory proteins may prevent or induce apoptosis [Citation39,Citation40]. The G2 checkpoint prevents cells from entering mitosis when DNA is damaged and ensures the propagation of error-free copies of the genome to each daughter cell, many anti-cancer drugs cause G2/M arrest or target on G2/M checkpoint [Citation40–44]. Despite the complexity of G2/M checkpoint regulation, the Cyclin B1/CDC2 complex, and Cyclin-dependent kinase 1 (CDK1), play critical roles in the cell survival or cell death [Citation42,Citation43]. In this study, DMAMCL treatment led to a decrease of the activity of cyclin B1/CDC2 complex since the protein level of CDC2 is down-regulated despite an increase of cycline B1 was observed ( G2/M arrest and decreased expression of CDC2 in OS cells). Although there is an increase of cycline B1 after DMAMCL treatment (), but it will not be functional since the loss of CDC2, a key component of cycline B1/CDC2 complex. These results are in accord with previous studies on its analogues or precursors of DMAMCL [Citation37]. Similar changes of cyclin B1 and CDC2 expressions, and G2/M arrest had been reported in the studies of other reagents induced cell apoptosis in osteosarcoma [Citation41–43].
Previous studies showed that DMAMCL selectively inhibited the AML stem and progenitor cells [Citation14]. Cell surface markers, such as CD133, are commonly used to identify CSCs. Several studies found that CD133-positive cells exhibit stem-like properties in osteosarcoma cell lines [Citation2,Citation45]. Transcription factors Oct3/4, Sox2, and Nanog play central roles in the development of CSCs in a variety of tumors including osteosarcoma, and maintaining pluripotency and self-renewal in undifferentiated embryonic stem cells [Citation2]. In this study, we find that DMAMCL treatment decreases the expressions of CD133 and Nanog, and reduces the colony formation ability and sphere formation ability of OS cells. These data indicate that DMAMCL decreases the stemness of osteosarcoma stem cells, which is consistent with function of DMAMCL in other types of cancer stem cells [Citation14].
Although we found that DMAMCL had an anti-tumor growth effect in vitro and in vivo on OS, there are still some limitations for the present study, which warrants further investigation. DMAMCL was only tested its anti-tumor effect as a single drug on osteosarcoma, the effect of a combination of DMAMCL with other anti-tumor agents is worth further study. Moreover, the mechanisms of how DMAMCL decreases stemness of osteosarcoma stem cell population need to be further investigated.
In conclusion, DMAMCL treatment induces apoptosis and cell cycle arrest in OS cells in vitro, inhibits OS xenograft tumor growth and extends the mice survival in vivo, and suppresses the stemness ability of OS cells. DMAMCL may be a promising candidate for the development of new drugs in the osteosarcoma treatment and is worth further comprehensive study.
Authors’ contributions
Gen Ba contributed to the study design, collection and interpretation of data, writing of the manuscript; Zhongyan Hua contributed to the collection of data and writing of the manuscript; Ning Xu and Simeng Zhang contributed to collection of data; Zhihui Liu, and Carol J. Thiele contributed to the interpretation of the data, and the writing of the manuscript; Zhijie Li contributed to the study design, interpretation of the data, the writing of the manuscript, and the submission of the manuscript for publication.
Supplemental Material
Download Zip (342.9 KB)Acknowledgments
We thank the staff from Medical Research Center of Shengjing Hospital who gave us support throughout the experiments. We thank the support from the National Natural Science Foundation of China (No. 81972515); Key Research and Development foundation of Liaoning Province (2019JH8/10300024), 2013 Liaoning Climbing Scholar Foundation, and 345 Talent Project of Shengjing Hospital of China Medical University.
Supplemental Material
Supplemental data for this article can be accessed here
Disclosure statement
The authors declare no conflict of interest.
Correction Statement
This article has been republished with minor changes. These changes do not impact the academic content of the article.
Additional information
Funding
References
- Isakoff MS, Bielack SS, Meltzer P, et al. Osteosarcoma: current Treatment and a Collaborative Pathway to Success. J Clin Oncol. 2015;33:3029–3035.
- Yan GN, Lv YF, Guo QN. Advances in osteosarcoma stem cell research and opportunities for novel therapeutic targets. Cancer Lett. 2016;370:268–274.
- Gvozdenovic A, Arlt MJ, Campanile C, et al. CD44 enhances tumor formation and lung metastasis in experimental osteosarcoma and is an additional predictor for poor patient outcome. J Bone Miner Res. 2013;28:838–847.
- Angulo P, Kaushik G, Subramaniam D, et al. Natural compounds targeting major cell signaling pathways: a novel paradigm for osteosarcoma therapy. J Hematol Oncol. 2017;10, 10. DOI:10.1186/s13045-016-0373-z.
- Biermann JS, Chow W, Reed DR, et al. NCCN Guidelines Insights: bone Cancer, Version 2.2017. J Natl Compr Canc Netw. 2017;15:155–167.
- Broadhead ML, Clark JC, Myers DE, et al. The molecular pathogenesis of osteosarcoma: a review. Sarcoma. 2011;2011:959248.
- Lu J, Song G, Tang Q, et al. MiR-26a inhibits stem cell-like phenotype and tumor growth of osteosarcoma by targeting Jagged1. Oncogene. 2017;36:231–241.
- Abarrategi A, Tornin J, Martinez-Cruzado L, et al. Osteosarcoma: cells-of-Origin, Cancer Stem Cells, and Targeted Therapies. Stem Cells Int. 2016;2016:3631764.
- Gupte A, Baker EK, Wan SS, et al. Systematic Screening Identifies Dual PI3K and mTOR Inhibition as a Conserved Therapeutic Vulnerability in Osteosarcoma. Clin Cancer Res. 2015;21:3216–3229.
- Lin YH, Jewell BE, Gingold J, et al. Osteosarcoma: molecular Pathogenesis and iPSC Modeling. Trends Mol Med. 2017;23:737–755.
- Yang J, Guo W, Wang L, et al. Cisplatin-resistant osteosarcoma cells possess cancer stem cell properties in a mouse model. Oncol Lett. 2016;12:2599–2605.
- Viennois E, Xiao B, Ayyadurai S, et al. Micheliolide, a new sesquiterpene lactone that inhibits intestinal inflammation and colitis-associated cancer. Lab Invest. 2014;94:950–965.
- Ma WW, Shi QQ, Ding YH, et al. Synthesis of micheliolide derivatives and their activities against AML progenitor cells. Molecules. 2013;18:5980–5992.
- Zhang Q, Lu Y, Ding Y, et al. Guaianolide sesquiterpene lactones, a source to discover agents that selectively inhibit acute myelogenous leukemia stem and progenitor cells. J Med Chem. 2012;55:8757–8769.
- Jia Y, Zhang C, Zhou L, et al. Micheliolide overcomes KLF4-mediated cisplatin resistance in breast cancer cells by downregulating glutathione. Onco Targets Ther. 2015;8:2319–2327.
- An Y, Guo W, Li L, et al. Micheliolide derivative DMAMCL inhibits glioma cell growth in vitro and in vivo. PLoS One. 2015;10:e0116202.
- Xu N, Hua Z, Ba G, et al. The anti-tumor growth effect of a novel agent DMAMCL in rhabdomyosarcoma in vitro and in vivo. J Exp Clin Cancer Res. 2019;38:118.
- Xiao W, Mohseny AB, Hogendoorn PC, et al. Mesenchymal stem cell transformation and sarcoma genesis. Clin Sarcoma Res. 2013;3:10.
- Mutsaers AJ, Walkley CR. Cells of origin in osteosarcoma: mesenchymal stem cells or osteoblast committed cells? Bone. 2014;62:56–63.
- Quist T, Jin H, Zhu JF, et al. The impact of osteoblastic differentiation on osteosarcomagenesis in the mouse. Oncogene. 2015;34:4278–4284.
- Martins-Neves SR, Paiva-Oliveira DI, Wijers-Koster PM, et al. Chemotherapy induces stemness in osteosarcoma cells through activation of Wnt/beta-catenin signaling. Cancer Lett. 2016;370:286–295.
- Hua Z, Zhan Y, Zhang S, et al. P53/PUMA are potential targets that mediate the protection of brain-derived neurotrophic factor (BDNF)/TrkB from etoposide-induced cell death in neuroblastoma (NB). Apoptosis. 2018;23:408–419.
- Los M, Mozoluk M, Ferrari D, et al. Activation and caspase-mediated inhibition of PARP: a molecular switch between fibroblast necrosis and apoptosis in death receptor signaling. Mol Biol Cell. 2002;13:978–988.
- Smulson ME, Simbulan-Rosenthal CM, Boulares AH, et al. Roles of poly(ADP-ribosyl)ation and PARP in apoptosis, DNA repair, genomic stability and functions of p53 and E2F-1. Adv Enzyme Regul. 2000;40:183–215.
- Yokoyama T, Kohn EC, Brill E, et al. Apoptosis is augmented in high-grade serous ovarian cancer by the combined inhibition of Bcl-2/Bcl-xL and PARP. Int J Oncol. 2017. DOI:10.3892/ijo.2017.3914
- Wion D, Berger F. Cancer stem cells. N Engl J Med. 2006;355:2703. author reply 2703.
- Zhao J. Cancer stem cells and chemoresistance: the smartest survives the raid. Pharmacol Ther. 2016;160:145–158.
- Ghantous A, Gali-Muhtasib H, Vuorela H, et al. What made sesquiterpene lactones reach cancer clinical trials? Drug Discov Today. 2010;15:668–678.
- Guzman ML, Rossi RM, Karnischky L, et al. The sesquiterpene lactone parthenolide induces apoptosis of human acute myelogenous leukemia stem and progenitor cells. Blood. 2005;105:4163–4169.
- Guzman ML, Rossi RM, Neelakantan S, et al. An orally bioavailable parthenolide analog selectively eradicates acute myelogenous leukemia stem and progenitor cells. Blood. 2007;110:4427–4435.
- Zhou J, Zhang Y. Cancer stem cells: models, mechanisms and implications for improved treatment. Cell Cycle. 2008;7:1360–1370.
- Kawasaki BT, Hurt EM, Kalathur M, et al. Effects of the sesquiterpene lactone parthenolide on prostate tumor-initiating cells: an integrated molecular profiling approach. Prostate. 2009;69:827–837.
- Neelakantan S, Nasim S, Guzman ML, et al. Aminoparthenolides as novel anti-leukemic agents: discovery of the NF-kappaB inhibitor, DMAPT (LC-1). Bioorg Med Chem Lett. 2009;19:4346–4349.
- Jia Y, Zhou L, Tian C, et al. Dynamin-related protein 1 is involved in micheliolide-induced breast cancer cell death. Onco Targets Ther. 2015;8:3371–3381.
- Thomadaki H, Scorilas A. BCL2 family of apoptosis-related genes: functions and clinical implications in cancer. Crit Rev Clin Lab Sci. 2006;43:1–67.
- Siddiqui WA, Ahad A, Ahsan H. The mystery of BCL2 family: bcl-2 proteins and apoptosis: an update. Arch Toxicol. 2015;89:289–317.
- Kim YR, Eom JI, Kim SJ, et al. Myeloperoxidase expression as a potential determinant of parthenolide-induced apoptosis in leukemia bulk and leukemia stem cells. J Pharmacol Exp Ther. 2010;335:389–400.
- Visconti R, Della Monica R, Grieco D. Cell cycle checkpoint in cancer: a therapeutically targetable double-edged sword. J Exp Clin Cancer Res. 2016;35:153.
- Evan GI, Vousden KH. Proliferation, cell cycle and apoptosis in cancer. Nature. 2001;411:342–348.
- Cheah FK, Leong KH, Thomas NF, et al. Resveratrol analogue, (E)-N-(2-(4-methoxystyryl) phenyl) furan-2-carboxamide induces G2/M cell cycle arrest through the activation of p53-p21(CIP1/WAF1) in human colorectal HCT116 cells. Apoptosis. 2018;23:329–342.
- Li HY, Zhang J, Sun LL, et al. Celastrol induces apoptosis and autophagy via the ROS/JNK signaling pathway in human osteosarcoma cells: an in vitro and in vivo study. Cell Death Dis. 2015;6:e1604.
- Wang H, Zhang T, Sun W, et al. Erianin induces G2/M-phase arrest, apoptosis, and autophagy via the ROS/JNK signaling pathway in human osteosarcoma cells in vitro and in vivo. Cell Death Dis. 2016;7:e2247.
- Wang G, Zhang T, Sun W, et al. Arsenic sulfide induces apoptosis and autophagy through the activation of ROS/JNK and suppression of Akt/mTOR signaling pathways in osteosarcoma. Free Radic Biol Med. 2017;106:24–37.
- Parida PK, Mahata B, Santra A, et al. Inhibition of cancer progression by a novel trans-stilbene derivative through disruption of microtubule dynamics, driving G2/M arrest, and p53-dependent apoptosis. Cell Death Dis. 2018;9:448.
- Brown HK, Tellez-Gabriel M, Heymann D. Cancer stem cells in osteosarcoma. Cancer Lett. 2017;386:189–195.