ABSTRACT
After fertilization, highly differentiated sperm and oocyte are reprogrammed to totipotent embryo, which subsequently cleavages and develops into an individual through spatial-temporal differentiation. Histone modifications play critical roles to coordinate with other reprogramming events in early stages of embryogenesis. However, most of studies focus on modifications at N-terminus of histones, those at nucleosome core were not well understood. Here, we characterize the three key acetylation events in the histone H3 core, H3K56/K64/K122ac, in early human embryos. The three residues localize at DNA entry-exit position of the nucleosome. Globally, H3K56ac, H3K64ac and H3K122ac were detectable throughout preimplantation stages, with H3K64ac levels being relatively stronger and H3K122ac levels being much weaker. Besides, H3K56ac level had a peak at two-cell stage. Moreover, we found that LINEs also peak at two-cell stage, and H3K56ac was enriched at young LINE-1 in human ESCs, supporting that H3K56ac is an important driving force for young LINE-1 activation in human preimplantation embryos. Our results suggest that acetylation in the nucleosome core of histone H3 is dynamic and various during preimplantation development, and may drive diverse chromatin remodeling events in this developmental window.
Introduction
Early mammalian embryogenesis is a complex process with changing nutritional requirements and metabolic patterns at different developmental stages, accompanied by dynamic epigenetic modifications and gene expression [Citation1]. More and more epigenetic modifications have been identified especially in reproductive system [Citation2,Citation3], while one of the most important ones in mammalian embryogenesis is histone hyperacetylation. Histone acetylation and deacetylation are vital portions of epigenetic modifications [Citation4]. It plays a key role in transcriptional regulation of mammalian oocyte maturation and early embryo development [Citation5–Citation7]. The most studied modifications are at the N-terminal tails and some C-terminal tails of histone protruding from the nucleosome. However, histones are also modified in positions close to DNA in the core of the nucleosome [Citation8]. In particular, H3 can be modified by acetylation of K56, K64, and K122, which are key residues at globular domains forming the nucleosomal core. Acetylation at the H3K122 and H3K64 residues on genome is associated with an active chromatin region, both of which have been shown to have direct and evoked effects in stimulating transcription [Citation9–Citation11]. H3K56ac has been well studied in yeast to show its role in chromatin opening [Citation12,Citation13], but its function in mammalian cells remains controversial. H3K56ac is enriched in transcriptional active regions and has been connected to the core transcriptional network in mouse and human embryonic stem cells [Citation14,Citation15] and chromatin structure in mouse zygotes [Citation16]. In vitro experiments showed that the acetylation modification neutralizes the positive charge on the basic amino acid residues on the histones, and weakens the binding between histones and negatively charged DNA, thereby promoting transcription of the gene [Citation17]. Hence, modifications of H3 K56, K64, and K122 within the nucleosome core may directly affect the binding and encapsulation of DNA around the histone octamer, thereby mediating large-scale epigenetic reprogramming. Moreover, histone acetylation may also be involved in nucleosome assembly, mediating recognition of nucleosomes by specific epigenetic factors, and higher-order chromatin architecture. Although core histone acetylation was previously studied in mouse oocytes and early embryos [Citation18], these modifications in human oocytes and early embryos remain elusive. Considering that many molecular events happen at different developmental stages in mouse and human embryos, such as zygotic genome activation [Citation19], histone core acetylation marking opening chromatin may also be different between mouse and human early embryos. In this study, we identified dynamic changes of three key acetylation residues in the histone core, H3K56ac, H3K64ac, and H3K122ac, observed their diverse intensities and localizations in different developmental stages, and showed that H3K56ac may play crucial roles in activation of retrotransposons in human early embryos.
Materials and methods
Oocyte and embryo collection with ethical approval
Human GV oocytes and preimplantation embryos were obtained from Reproductive Medicine Center, Tongji Medical College, Huazhong University of Science and Technology. GV oocytes were cultured in G-1 medium (Vitrolife, 10,127, Sweden) for 16–24 hours to develop into MII stage. A total of 27 oocytes from oocyte donation and 43 Frozen-thawed embryos abandoned by patients were included in this study. Ethical approval for the study was obtained by the CEIC (Ethics Committee for Clinical Research) of Reproductive Medicine Center, Tongji Medical College, Huazhong University of Science and Technology. All people included in the study gave informed consent.
Immunostaining
Embryos were fixed with 4% paraformaldehyde for 30 min. After permeabilization, embryos were washed 3 times in PBS-T (0.05% Tween in PBS), blocking for 30 min and incubated with the primary antibodies for 12 h at 4°C, followed by 3 washes in PBS-T, incubation for 2 h at RT with the corresponding secondary antibodies: Alexa Fluor 488 secondary antibody (Thermo Fisher Scientific) and 20 μg/ml Hoechst (Thermo Fisher Scientific). The primary antibodies used were: H3K56ac (Abcam ab76307, 1:100 dilution), H3K64ac (Abcam ab71956, 1:100 dilution), H3K122ac (abcam ab33309, 1:100 dilution).
Confocal imaging
Confocal microscopy was performed using laser-scanning microscope (LSM780, Zeiss) in Tongji Medical College, Huazhong University of Science and Technology. Fluorescence intensity profiles were analyzed using Zeiss LSM software (ZEN blue). Images were generated by GraphPad Prism or Excel, followed by organization by Adobe Illustrator.
High-throughput data analysis
Raw reads were processed with cutadapt to perform quality trimming with default parameters except for: quality-cutoff = 20, pair-filter = both. To include as many non-uniquely mapped reads as possible, trimmed reads were firstly aligned to human genome hg19 by STAR with default settings with parameters “–winAnchorMultimapmax 2000 – outFilterMultimapNmax 1000.” RSEM was used to calculate FPKM value of genes. Heatmap was generated by R. TE transcript program with default parameters was used to get counts for transposable elements. RNA-seq data of early human embryos and ESC are from GSE36552. H3K56ac ChIPseq data of human ESC is from GSE16256.
Results
To examine dynamic change of histone core modifications in human oocytes and embryos, we collected abandoned human oocytes and early embryos for following experiments (). Histone acetyltransferases (HATs) and histone deacetyltransferases (HDACs) collectively regulate histone acetylation levels to orchestrate gene expression and control cell fate. We analyzed expression of various HATs and HDACs in human oocytes and early embryos using public dataset [Citation20], and found that most HATs are abundant in oocytes and early embryos, while more than half of HDACs are weakly expressed in these stages (Figure S1). This result supports that the chromatin environment in human oocytes and early embryos is quite opened.
Figure 1. Representative images of human preimplantation embryos for collection. Images show human GV oocyte, MII oocyte, two-cell embryo, four-cell embryo, eight-cell embryo, and blastocyst for further immunostaining analysis. Scale bar, 20 μm.

Immunostaining with H3K56ac antibody identified consistent fluorescence signal at chromatin in GV oocytes, MII oocytes, and throughout the subsequent developmental stages up to the blastocyst (, S2). We found that H3K56ac signal recapitulated Hoechst localization in all detected stages. This showed that H3K56ac is evenly distributed at chromatin of mature oocytes and preimplantation embryos. Interestingly, we noticed that detected H3K56ac signal was relatively strong in two-cell stage, probably priming zygotic genome activation starting from four-cell stage.
Figure 2. Dynamics of H3K56ac during human preimplantation development. (a) Immunofluorescence of H3K56ac in preimplantation embryos. Oocytes and frozen-thawed embryos were fixed and stained with H3K56ac antibody (Red). DNA was stained by Hoechst as shown in blue. Blastocysts were shown as full projections of Z-sections taken every 8 μm on a confocal microscope. (b) Fluorescence intensity profiles of H3K56ac and DNA of all stages of embryos. Lines were drawn on the images of nuclei to quantify intensities for H3K56ac (red) and DNA (blue) along the lines. Scale bar, 20 μm.
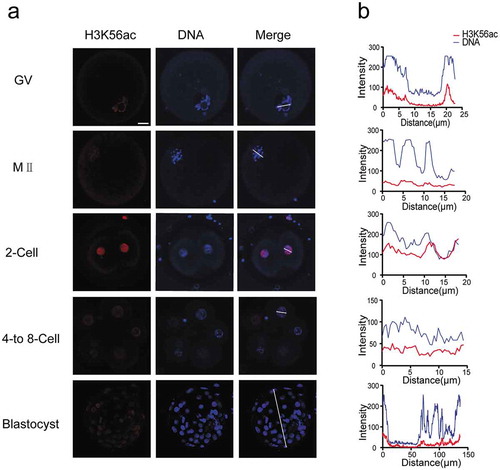
H3K64ac is associated with chromatin opening and transcriptional activation. We found that this modification showed very strong fluorescence signal during oocyte maturation and early embryonic development (). In both GV and MII oocytes, we detected strong H3K64ac level and complete colocalization with Hoechst. After fertilization, H3K64ac level was kept high until blastocyst stage. Notably, we found H3K64ac signal was mainly enriched near the nuclear envelope in the cleavage embryos. Additionally, H3K64ac signal was absent in DNA-dense heterochromatic regions in cleavage stages, showing that H3K64ac was distributed at euchromatin area.
Figure 3. Dynamics of H3K64ac during human preimplantation development. (a) Immunofluorescence of H3K64ac in preimplantation embryos. Oocytes and frozen-thawed embryos were fixed and stained with H3K64ac antibody (red). DNA is shown in blue. (b) Fluorescence intensity profiles of H3K64ac and DNA of all stages of embryos. Lines were drawn on the images of nuclei to quantify intensities for H3K64ac and DNA along the lines. Scale bar, 20 μm.
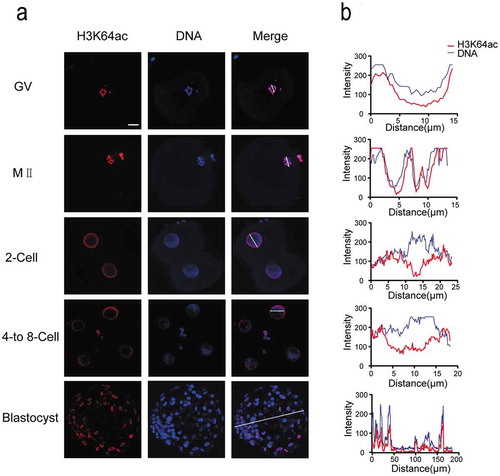
Furthermore, we assessed the distribution of H3K122ac in oocytes and early embryos, which exhibited low levels in all detected developmental stages (). H3K122ac was visible in GV oocytes and colocalized with Hoechst-stained DNA signal. At MII stage, H3K122ac signal was just above detection. The abundance of H3K122ac decreased in two-cell stage embryos and remained very low throughout cleavage stages. H3K122ac was also observable in blastocyst stage with quite weak signal.
Figure 4. H3K122ac is present at low levels in all stages of early human embryos. (a) H3K122ac was analyzed in oocytes and preimplantation embryos. Oocytes and frozen-thawed embryos were fixed and stained with H3K122ac antibody (red). DNA is shown in blue. (b) Fluorescence intensity profiles of H3K122ac and DNA of all stages of embryos. Lines were drawn on the images of nuclei to quantify intensities for H3K122ac and DNA along the lines. Scale bar, 20 μm.
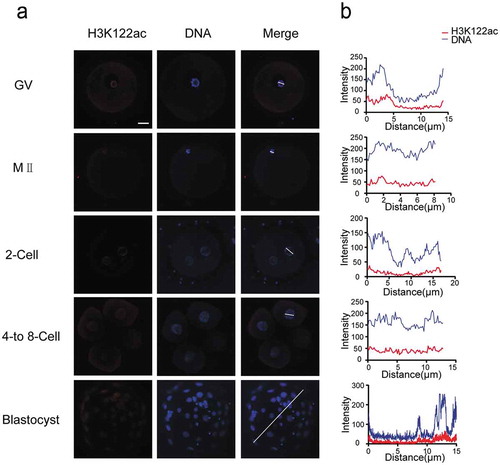
Additionally, we compared intensities and distributions of these three modifications in inner cell mass and trophectoderm at blastocyst stage (). The result showed that levels of the three modifications were comparable between inner cell mass and trophectoderm, indicating that they are not involved in the first cell fate determination. H3K56ac and H3K122ac showed quite even distribution in nuclei of both inner cell mass and trophectoderm. In contrast, H3K64ac showed negative correlation with DNA-dense regions in both inner cell mass and trophectoderm, indicating its function in chromatin opening and gene activation.
Figure 5. Three histone acetylation intensities of inner cell mass and trophectoderm in human blastocysts, including H3K56ac (a), H3K64ac (b), H3K122ac (c). Frozen-thawed embryos were fixed and stained with H3K56ac, H3K64ac, and H3K122ac antibody (red). DNA is shown in blue. Fluorescence intensity profiles of histone acetylation and DNA in the nucleus of inner cell mass and trophectoderm are shown at right. Scale bar, 5 μm.
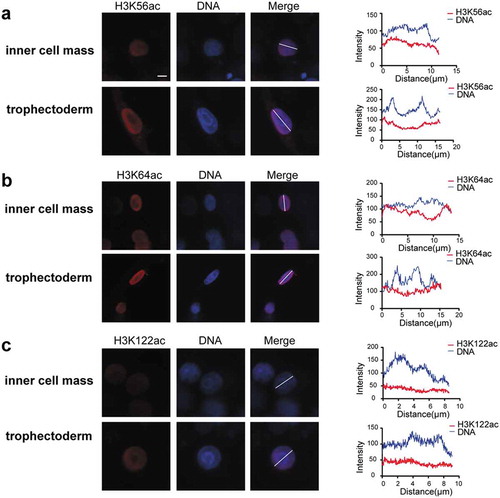
We summarize relative intensities of the core histone modifications and found that all three modifications are present in human oocytes and early embryos (, S3). Interestingly, H3K56ac peaks at two-cell stage, indicating that specific gene sets are activated at two-cell stage, before canonical zygotic genome activation at four- to eight-cell stage during human preimplantation development [Citation21]. Transposable elements (TE) are mobile DNA elements enriched in mammalian early embryos, with retrotransposons as important members which replicate themselves in host genome using RNA intermediates [Citation22]. We analyzed dynamic levels of different TE families, and observed that levels of LINE and LTR retrotransposon are highest at two-cell stage ()). We therefore propose that H3K56ac intensity largely reflects activation of LINE and LTR. LINE is a well-known autonomous retrotransposon, we therefore focus on LINE in following studies. We next analyzed H3K56ac ChIP-seq data in human ESCs to find enrichment of H3K56ac at LINE, SINE and LTR retrotransposon (), left panel). Additional analysis indicated H3K56ac at LINE-1 to be mostly represented in LINE subfamilies (), right panel). Evolutionary age analysis on LINE-1 members showed that expression levels and H3K56ac levels of LINE-1 members were higher when LINE-1 members are younger [Citation23] ()), demonstrating that H3K56ac is involved in orchestrating activities of LINE-1 members depending on their evolutionary ages.
Figure 6. Analysis of the relative intensities of acetylation-to-DNA in oocytes and preimplantation embryos. The intensities of H3K56ac (blue), H3K64ac (red), and H3K122ac (green) are compared to DNA (Hoechst) in different stages of human preimplantation embryos.
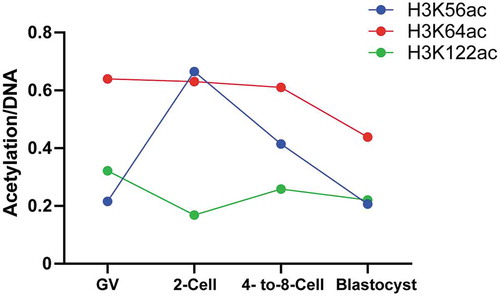
Figure 7. H3K56ac is enriched at transposable element (TE). (a) Expression patterns of different TE including LINE, SINE, LTR, Satellite, DNA are distinct and dynamic during human preimplantation development, while expression of non-TE genes is quite consistent. (b) Distribution of H3K56ac reads at different TE (left panel) and LINE subfamilies (right panel) in human ESCs. (c) Evolutionary younger LINE-1 members had higher expression level (left panel) and higher H3K56ac enrichment (right panel) in human ESCs. Myr, million years.
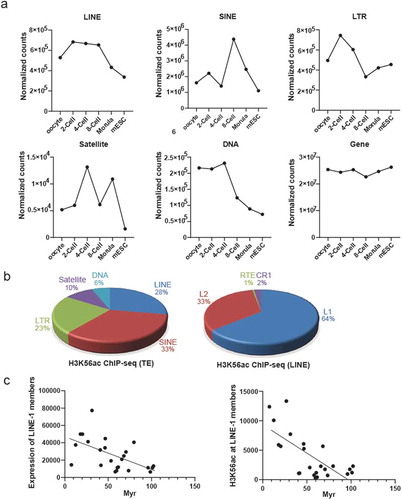
Taken together, we analyzed levels of three histone modifications, H3K56ac, H3K64ac and H3K122ac in human oocytes and preimplantation embryos, and identified that they are dynamic in intensity and localization at different developmental stages, indicating their various functions in human early embryos. Meanwhile, they did not show differential intensities in inner cell mass and trophectoderm, indicating that they are not involved in regulating cell fate decision in early human embryo. Specifically, we noticed both intensity of H3K56ac and expression of LINE to be highest at 2-cell stage, in agreement with our further discovery that there are abundant H3K56ac reads at LINE loci and there is negative correlation of H3K56ac enrichment and evolutionary ages of LINE members using human ESC as the studying model.
Discussion
During mammalian fertilization, chromosome structure is changed dramatically [Citation24–Citation26]. In this process, the zygotic genome is reprogrammed to activate the embryonic genome and obtain the totipotency of development. Core histone acetylation may play crucial roles in this process to ensure successful embryonic development. Here, we studied dynamic patterns of the three key acetylation sites of histone H3, H3K56ac, H3K64ac, and H3K122ac. All three modifications are present in human oocytes and preimplantation embryos. However, they exhibited different patterns of intensities and distributions. H3K56ac and H3K64ac were abundant throughout preimplantation stages, while H3K122ac showed low levels. H3K56ac is associated with active chromatin but seems evenly distributed in human oocytes and embryos. H3K64ac is the most abundant in all stages, while H3K122ac signal is very weak. Both H3K64ac and H3K122ac colocalized with euchromatin in human oocytes and embryos, indicating their function in transcriptional activation. Currently, dynamics and functions of histone modifications at nucleosome core are largely unknown and how they regulate embryonic program remain elusive. Here, we find that LINE and LTR levels are enriched at two-cell stage, and hence propose that they open global chromatin for zygotic genome activation in later stages through associating with their genomic loci [Citation27]. Meanwhile, SINE is mainly enriched at eight-cell stage, supporting that SINE has direct functions in regulating zygotic genome activation through initiating or enhancing embryonic genes. Further studies on distributions and catalyzing enzymes of different histone acetylation in oocytes and early embryos will pave the way for deepening the understanding of how zygotic genome is activated for totipotency establishment and improving assisted reproduction technology. Additionally, mammalian genetic system may need to be developed in the future to identify functions of specific residues of histones [Citation28,Citation29].
Author contributions
Li-quan Zhou and Ping Su conceived and designed the project. Xiao-fei Wang, Shi-ming Xie and Shi-meng Guo performed the experiment. Xiao-fei Wang and Shi-meng Guo wrote the initial manuscript. Li-quan Zhou and Ping Su revised the manuscript. All authors have read and approved the final manuscript.
Supplemental Material
Download MS Word (574.7 KB)Supplemental Material
Download Zip (435.4 KB)Disclosure statement
The authors declare no conflict of interest.
Supplementary material
Supplemental data for this article can be accessed here.
Additional information
Funding
References
- Zhou LQ, Dean J. Reprogramming the genome to totipotency in mouse embryos. Trends Cell Biol. 2015;25:82–91.
- Tan M, Luo H, Lee S, et al. Identification of 67 histone marks and histone lysine crotonylation as a new type of histone modification. Cell. 2011;146:1016–1028.
- Zhang L, Eugeni EE, Parthun MR, et al. Identification of novel histone post-translational modifications by peptide mass fingerprinting. Chromosoma. 2003;112:77–86.
- Kouzarides T. Chromatin modifications and their function. Cell. 2007;128:693–705.
- Lu Y, Dai X, Zhang M, et al. Cohesin acetyltransferase Esco2 regulates SAC and kinetochore functions via maintaining H4K16 acetylation during mouse oocyte meiosis. Nucleic Acids Res. 2017;45:9388–9397.
- Wu X, Hu F, Zeng J, et al. generation is essential for quality control of aged oocytes. Aging Cell. 2019;18:e12955.
- Chan SH, Tang Y, Miao L, et al. Brd4 and P300 confer transcriptional competency during zygotic genome activation. Dev Cell. 2019;49:867–81 e8.
- Tropberger P, Schneider R. Scratching the (lateral) surface of chromatin regulation by histone modifications. Nat Struct Mol Biol. 2013;20:657–661.
- Di Cerbo V, Mohn F, Ryan DP, et al. Acetylation of histone H3 at lysine 64 regulates nucleosome dynamics and facilitates transcription. eLife. 2014;3:e01632.
- Simon M, North JA, Shimko JC, et al. Histone fold modifications control nucleosome unwrapping and disassembly. Proc Natl Acad Sci U S A. 2011;108:12711–12716.
- Tropberger P, Pott S, Keller C, et al. Regulation of transcription through acetylation of H3K122 on the lateral surface of the histone octamer. Cell. 2013;152:859–872.
- Xu F, Zhang K, Grunstein M. Acetylation in histone H3 globular domain regulates gene expression in yeast. Cell. 2005;121:375–385.
- Ozdemir A, Spicuglia S, Lasonder E, et al. Characterization of lysine 56 of histone H3 as an acetylation site in Saccharomyces cerevisiae. J Biol Chem. 2005;280:25949–25952.
- Tan Y, Xue Y, Song C, et al. Acetylated histone H3K56 interacts with Oct4 to promote mouse embryonic stem cell pluripotency. Proc Natl Acad Sci U S A. 2013;110:11493–11498.
- Xie W, Song C, Young NL, et al. Histone h3 lysine 56 acetylation is linked to the core transcriptional network in human embryonic stem cells. Mol Cell. 2009;33:417–427.
- Zhou L, Baibakov B, Canagarajah B, et al. Genetic mosaics and time-lapse imaging identify functions of histone H3.3 residues in mouse oocytes and embryos. Development. 2017;144:519–528.
- Neumann H, Hancock SM, Buning R, et al. A method for genetically installing site-specific acetylation in recombinant histones defines the effects of H3 K56 acetylation. Mol Cell. 2009;36:153–163.
- Ziegler-Birling C, Daujat S, Schneider R, et al. Dynamics of histone H3 acetylation in the nucleosome core during mouse pre-implantation development. Epigenetics. 2016;11:553–562.
- Xue Z, Huang K, Cai C, et al. Genetic programs in human and mouse early embryos revealed by single-cell RNA sequencing. Nature. 2013;500:593–597.
- Yan L, Yang M, Guo H, et al. Single-cell RNA-Seq profiling of human preimplantation embryos and embryonic stem cells. Nat Struct Mol Biol. 2013;20:1131–1139.
- Lu X, Gao Z, Qin D, et al. A maternal functional module in the mammalian oocyte-to-embryo transition. Trends Mol Med. 2017;23:1014–1023.
- Guerra UP, Pizzocaro C, Terzi A, et al. New tracers for the imaging of the medullary thyroid carcinoma. Nucl Med Commun. 1989;10:285–295.
- Khan H, Smit A, Boissinot S. Molecular evolution and tempo of amplification of human LINE-1 retrotransposons since the origin of primates. Genome Res. 2006;16:78–87.
- Wu J, Xu J, Liu B, et al. Chromatin analysis in human early development reveals epigenetic transition during ZGA. Nature. 2018;557:256–260.
- Gao L, Wu K, Liu Z, et al. Chromatin accessibility landscape in human early embryos and its association with evolution. Cell. 2018;173:248–59 e15.
- Liu L, Leng L, Liu C, et al. An integrated chromatin accessibility and transcriptome landscape of human pre-implantation embryos. Nat Commun. 2019;10:364.
- Lu JY, Shao W, Chang L, et al. Genomic repeats categorize genes with distinct functions for orchestrated regulation. Cell Rep. 2020;30:3296–311 e5.
- Gunesdogan U, Jackle H, Herzig A. A genetic system to assess in vivo the functions of histones and histone modifications in higher eukaryotes. EMBO Rep. 2010;11:772–776.
- Zhang W, Zhang X, Xue Z, et al. Probing the function of metazoan histones with a systematic library of H3 and H4 mutants. Dev Cell. 2019;48:406–19 e5.