ABSTRACT
RepoMan is a chromosome-associated scaffold protein that integrates signaling of multiple kinases and phosphatases to coordinate spindle-kinetochore interactions, chromosome (de)condensation and nuclear envelope (dis)assembly during mitosis. Another key mitotic event is the assembly of a microtubule-based spindle, which involves redundant pathways emanating from the centrosomes, microtubules and chromosomes. Here we describe a novel mitotic function of RepoMan in regulating chromosome-dependent microtubule assembly. At limiting concentrations of microtubule-destabilizing agents, RepoMan-depleted cells showed enhanced chromosome clustering. This clustering was completely dependent on the partial inhibition of microtubule growth originating from the chromosome-dependent pathway. We also demonstrated that RepoMan interacts with prime regulators of the chromosome-dependent spindle assembly such as NuSAP1, NuMA, and TPX2. In addition, RepoMan was required to enable or maintain phosphorylation of NuSAP1 at CDK sites, thereby enabling activation of NuSAP1 through dissociation of inhibitory importin β/7. Our data identify RepoMan as an enhancer of microtubule assembly at chromosomes.
Introduction
The assembly of a microtubule (MT)-based spindle is essential for chromosome segregation during cell division [Citation1]. The mitotic spindle forms bipolar attachments with kinetochores of sister chromatids, enabling their separation to opposite poles during anaphase. MTs are tubular structures consisting of α- and β-tubulin heterodimers [Citation2]. MT polymerization is highly dependent on the concentration of α/β-tubulin, but is also endorsed by MT-associated proteins [Citation3,Citation4]. Based upon the subcellular location of the initial steps of MT assembly, three distinct spindle-assembly pathways have been described [Citation1,Citation5,Citation6]. The centrosome-dependent pathway is the major source of MT nucleation and originates from the duplicated centrioles. The MT-dependent pathway is regulated by the Augmin complex and allows new MTs to branch out from preexisting MTs [Citation7]. Finally, the chromosome-dependent pathway promotes MT outgrowth from the chromosomes. The three pathways of MT assembly act redundantly but also fulfil distinct functions. The centrosome-dependent pathway defines the position of the spindle poles and, hence, the axis of cell division [Citation6]. The MT-dependent pathway increases the number of MTs, thereby reinforcing the mitotic spindle [Citation6]. Finally, MTs formed at chromosomes are stabilized by kinetochores, giving rise to the formation of bundles of 20–40 MTs, known as kinetochore fibers or K-fibers [Citation6].
A concentration gradient of RanGTP governs the chromosome-dependent pathway of MT assembly. On the one hand, the chromatin-associated Regulator of Chromosome Condensation 1 (RCC1), a guanine nucleotide exchange factor (GEF), generates RanGTP at the chromosomes [Citation1,Citation7]. On the other hand, RanGTP diffuses away from the chromosomes and is rapidly converted to RanGDP by a soluble complex, consisting of the Ran-Binding Protein 1/2 (RanBP1/2) and a Ran GTPase Activating protein (RanGAP), which stimulates the intrinsic GTPase activity of Ran. These two counteracting mechanisms create a steep RanGTP gradient, with the highest RanGTP levels close to the chromosomes. RanGTP contributes to MT polymerization at the chromosomes by activating spindle assembly factors (SAFs) [Citation7,Citation8]. Activation of SAFs involves the RanGTP-induced dissociation of inhibitory importins. Many SAFs have been described and include proteins as diverse as Targeting Protein for Xkpl2 (TPX2), Nuclear Mitotic Apparatus 1 (NuMA) and Nucleolar Spindle-Associated Protein 1 (NuSAP1), which all stabilize and/or crosslink MTs in a tightly regulated manner [Citation9]. For example, NuSAP1 is bound to importin α, importin β and importin 7 [Citation10,Citation11]. Importin α and importin 7 inhibit the MT-stabilizing activity of NuSAP1, while importin β blocks its MT cross-linking ability and counteracts its degradation [Citation12]. Aside from its regulation by importins, NuSAP1 is also regulated by phosphorylation at Thr300 and Thr338 by cyclin-dependent kinase 1 (CDK1), which reduces its binding-affinity for MTs [Citation13].
RepoMan, for recruits PP1 onto mitotic chromatin at anaphase, is a mitotic chromosome-associated scaffold for protein kinase CDK1 as well as protein phosphatases PP1 and PP2A-B56 in vertebrates [Citation14–17]. Spatiotemporally regulated signaling through these RepoMan-associated enzymes contributes to the centromeric targeting of protein kinase Aurora B during (pro)metaphase, where it destabilizes erroneous MT-kinetochore interactions [Citation15,Citation16,Citation18]. However, RepoMan has also functions in chromosome (de)condensation [Citation19,Citation20], nuclear envelope (dis)assembly [Citation21,Citation22], and the organization and repair of interphase chromatin [Citation19,Citation21,Citation23]. In addition, we previously observed that RepoMan-depleted cells, blocked in prometaphase with the MT-depolymerizing drug nocodazole, show a reduced spatial distribution of the chromosomes [Citation15]. Here, we report that the latter phenotype stems from an inhibition of the chromosome-dependent pathway of MT assembly, thereby hampering MT-guided chromosome spreading. We also show that RepoMan binds multiple SAFs and promotes chromosome-dependent MT assembly through the activation of NuSAP1 by disrupting its interaction with inhibitory importins.
Results and discussion
A depletion of RepoMan causes chromosome clustering in prometaphase-arrested cells
The knockdown of RepoMan with either of two siRNAs resulted in clustered chromosomes in U2OS cells arrested in prometaphase with 0.33 μM nocodazole, as visualized by DAPI staining ()) as well as by staining for the kinetochore marker ACA (Fig. S1). Since RepoMan shows a diffuse staining during prometaphase, we used an indirect readout to identify RepoMan-depleted cells, namely phosphorylation of the PP1:RepoMan substrate histone H3 at Thr3 (H3T3ph) [Citation15,Citation16]. However, the knockdown of RepoMan and its correlation with increased H3T3ph levels was also verified by immunoblotting (Fig. S2a). The area occupied by the chromosomes (DAPI staining) was reduced by ~50% in RepoMan-depleted cells (); Fig. S1). Chromosome clustering was also observed in nearly diploid RPE-1 cells ()), indicating that the phenotype was not an artifact of cancer cells or a consequence of polyploidy. We also performed live imaging on cells stably overexpressing mCherry-Histone H2B, which revealed that chromosome clustering in RepoMan-depleted cells became visible immediately after nuclear envelope breakdown (NEBD) ()). However, we did not observe microscopic changes in chromatin distribution in G1, S and G2. Together, these data indicated that mitotic chromosome clustering in RepoMan-depleted cells was not the consequence of a prolonged mitotic arrest or chromosome defects generated during interphase. Since RepoMan has been implicated in the regulation of chromosome architecture [Citation21–23], we have also examined whether the depletion of RepoMan affected chromosome condensation in prometaphase-arrested cells. However, we did not detect a visual difference in chromosome spreads and in chromosome-width measurements (Fig. S3).
Figure 1. Chromosome clustering in RepoMan-depleted cells during prometaphase
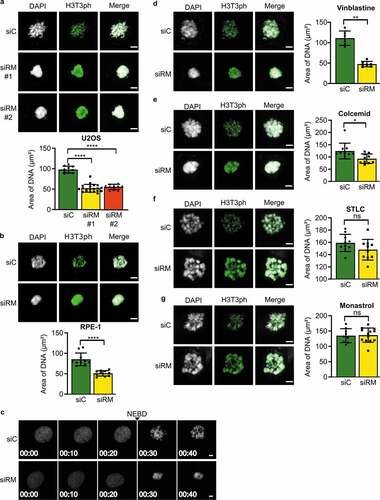
Next we examined the extent to which the chromosome-clustering phenotype was dependent on the nature of the compound that was used to arrest the cells in prometaphase. Nocodazole, vinblastine and colcemid bind to tubulin and inhibit the polymerization of MTs [Citation24]. S-Trityl-L-Cysteine (STLC) and monastrol are inhibitors of the Eg5 kinesin and inhibit the antiparallel sliding of polar MTs [Citation25]. Hence, STLC and monastrol block cells in prometaphase without disrupting MT nucleation. Strikingly, only compounds that interfered with MT nucleation (nocodazole, vinblastine and colcemid) elicited the chromosome-clustering phenotype (,d-g)), hinting at a role of RepoMan in MT stabilization. Finally, we verified that transfection with siRNA per se had no effect on chromosome clustering (Fig. S2b-g).
A depletion of RepoMan reduces MT stability
We noted that chromosome clustering was critically dependent on the concentration of nocodazole ()). At 0.33 µM nocodazole, the knockdown of RepoMan was associated with clustered chromosomes. However, no such effect on the chromosomes was seen at lower (0.1 µM) concentrations of nocodazole. At higher nocodazole concentrations (1 µM) control cells also showed a similar chromosome-clustering phenotype. These data indicated that the clustering of chromosomes is linked with the destabilization of MTs and that the knockdown of RepoMan caused chromosome clustering by increasing the sensitivity to nocodazole. Consistent with this notion, we found that chromosome clustering after the depletion of RepoMan was rescued by addition of the MT-stabilizing agent taxol ()). Moreover, when cells were released from a nocodazole arrest, allowing the regrowth of MTs, the chromosome-clustering phenotype disappeared ()). The reversal of the chromosome-clustering effect following the spontaneous or induced regrowth of MTs demonstrates the link between chromosome clustering and MT destabilization. Moreover, the rescue of the phenotype by regrowth of MTs also showed that the observed clustering cannot be interpreted as an early event of apoptosis.
Figure 2. Chromosome clustering in RepoMan-depleted cells is reversible
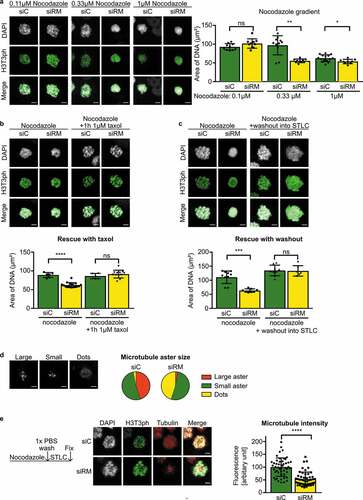
We proceeded to examine in a more direct manner the effect of RepoMan depletion on MT stability. At limiting concentrations of nocodazole (0.33 μM), RepoMan-depleted cells often contained smaller MT asters as compared to those in control cells ()). Also, following a short release from a nocodazole arrest in the presence of STLC, MT asters rapidly increased in size but this response was blunted after the knockdown of RepoMan ()). Collectively, these data show that chromosome clustering in RepoMan-depleted cells was explained by a decreased MT stability.
RepoMan interacts with regulators of MT nucleation and stability
To explore at the molecular level how RepoMan interferes with MT assembly, we screened for protein interactors with established functions in MT nucleation and stability. Using published lists of RepoMan interactors that were identified by mass-spectrometric methods [Citation14,Citation26,Citation27], we obtained numerous candidate-interactors of RepoMan with functions in mitotic spindle formation, including karyopherins, spindle assembly factors and components of the RanGTP pathway ()). We could confirm 7 out of 12 (58%) tested interactions by co-immunoprecipitation with ectopically expressed EGFP-tagged RepoMan. The confirmed interactors comprised the spindle assembly factors NuSAP1, NuMA and TPX2 as well as karyopherins (importin α, importin β and importin 7) that are inhibitory to these SAFs ()). These data suggested that RepoMan stabilizes MTs through the regulation of SAF-importin complexes at the chromosomes [Citation28].
Figure 3. RepoMan interacts with essential microtubule-associated proteins
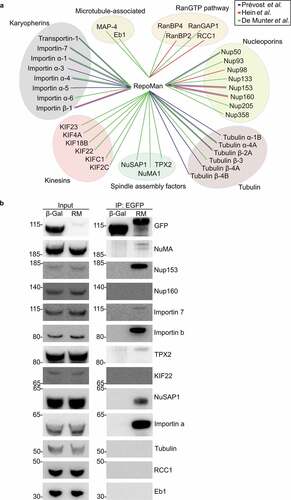
RepoMan is an activator of NuSAP1
Since RepoMan integrates signaling through multiple kinases and phosphatases, we subsequently examined whether RepoMan contributes to the activation of SAFs. The depletion of RepoMan increased the interaction of EGFP-tagged NuSAP1 with importin β and importin 7, but not with importin α ()), indicating that RepoMan contributes to the activation of NuSAP1. This effect was specific for NuSAP1 and not detected for TPX2 and NuMA. NuSAP1 is an established substrate of CDK1 [Citation13], and we found that the knockdown of RepoMan decreased the phosphorylation of NuSAP1 at consensus CDK sites (pSP/pTP) by about 70% ()), indicating that RepoMan promoted the phosphorylation of NuSAP1 by CDK1. Importantly, the inhibition of CDK1 with roscovitine increased the NuSAP1-importin β/7 interaction to the same extent as seen following the knockdown of RepoMan. In contrast, inhibition of other key mitotic kinases, i.e. Aurora B with hesperadine or Polo-like kinase 1 with BI2536, did not affect the NuSAP1-importin β/7 interaction. Finally, incubation of the cells with calyculin A, an inhibitor of PPP-type protein Ser/Thr phosphatases, including PP1 and PP2A, abolished the NuSAP1-importin β/7 interaction induced by the knockdown of RepoMan. Together, these data show that both CDK1 and RepoMan promote the phosphorylation and activation of NuSAP1.
Figure 4. The depletion of RepoMan increases the NuSAP1-importin interaction in a phosphorylation-dependent manner
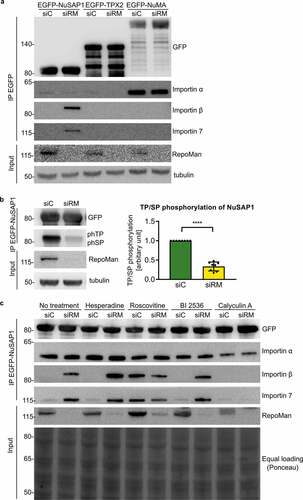
Conclusions
The knockdown of RepoMan rendered cells more sensitive to MT-polymerization inhibitors, resulting in an increased clustering of prometaphase chromosomes ( and ). This phenotype correlated with a decreased stability of K-fibers ( and ), which are formed and stabilized close to the chromosomes. We envisage that the smaller K-fibers in prometaphase-arrested and RepoMan-depleted cells are less efficient in pushing chromosomes apart by growth at their +-end, resulting in chromosome clustering. Consistent with a role for RepoMan in K-fiber stabilization we identified K-fiber stabilizing SAFs such as NuSAP1, TPX2 and NuMA as interactors of RepoMan (). Since RepoMan is dynamically associated with mitotic chromosomes [Citation16,Citation17,Citation29], it is tempting to speculate that RepoMan stabilizes chromosomal MTs by targeting SAFs to the chromosomes, where they can be activated through RanGTP-mediated dissociation of importins. We also found that RepoMan is involved in the activation of NuSAP1 through regulation of its inhibitory interaction with importin β/7 (). These data establish RepoMan as a regulator of the chromosome-dependent pathway of spindle-assembly (). Further studies are needed to distinguish between a role for RepoMan in MT nucleation and assembly, and to delineate the contribution of RepoMan to spindle assembly in normal and acentrosomal cells. Importantly, the three spindle-assembly pathways are functionally redundant but show a different sensitivity to MT poisons. The chromosome-dependent pathway is most resistant to MT destabilization [Citation6,Citation30–36], explaining why a chromosome-clustering phenotype is only detected at high concentrations of nocodazole (1 µM) or at intermediate concentrations of nocodazole (0.33 µM) following the depletion of RepoMan. We furthermore demonstrated that RepoMan was also needed to enable or maintain phosphorylation of NuSAP1 at CDK site(s). Since the knockdown of RepoMan as well as the CDK1 inhibitor Roscovitine increased the NuSAP1-importin β/7 interaction, we speculate that RepoMan promotes MT assembly, at least in part, by promoting the CDK1 phosphorylation of NuSAP1, thereby inhibiting its interaction with importin β/7 and promoting its MT stabilizing and crosslinking function. NuSAP1 has many consensus sites for CDK phosphorylation, but the site(s) that are regulated by RepoMan and control its interaction with importin β/7 remain to be identified. Previous work has implicated RepoMan in the timely dephosphorylation of mitotic proteins by associated PP1 and PP2A-B56 [Citation14,Citation16,Citation17]. However, RepoMan is also associated with cyclin B/CDK1, which phosphorylates RepoMan to modulate its binding to PP1 and PP2A-B56 [Citation14,Citation16,Citation17]. We hypothesize that the RepoMan-associated pool of cyclin B/CDK1 also phosphorylates proteins, including NuSAP1, which are recruited via RepoMan. Collectively, our data disclose a mitotic function for RepoMan in the stabilization of MT generated by the chromosome-dependent spindle-assembly pathway.
Figure 5. Model on the role of RepoMan in chromosome-dependent spindle assembly
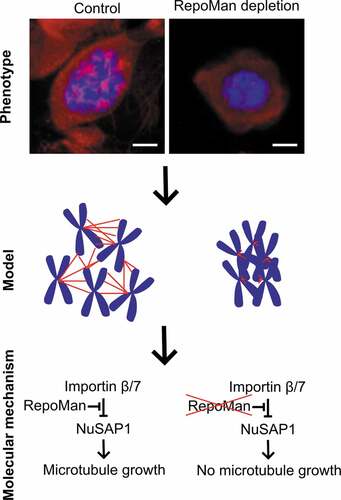
Methods
Cell culture, transient transfection and knock-down
HEK293T cells (ATCC CRL-11,268 – 293 T/17) were cultured in high-glucose Dulbecco’s modified Eagle’s medium (4,500 mg/liter glucose, Sigma-Aldrich), supplemented with 10% fetal calf serum (Sigma-Aldrich). U2OS (ATCC-HTB-96) and HeLa (ECACC 93,021,013) cells were cultured in low-glucose Dulbecco’s modified Eagle’s medium, supplemented with 10% FCS. All media contained 100 units/ml penicillin and 100 µg/ml streptomycin. The cells were transfected with Genius (Westburg, Leusden, the Netherlands), jetPRIME (PolyPlus Transfection, Strasbourg, France) or Fugene (Promega, Madison, WI, USA), according to the manufacturer’s protocol. Transient knockdown was obtained with 50 nM of siRNA using the standard jetPRIME (PolyPlus Transfection, Strasbourg, France) protocol. siControl: UAA GGCUAUGAAGAGAUACdTdT (Dharmacon). siRM1: UGACAGACUUGACCAGAAAdTdT (Dharmacon). siRM2: CAAAUACUCCAUUGCGUAA/GCACUGUAUCGAAAUGUUA/UAAUxUGGUCUCCAGAUAUU/GAAGACGUUCCAUGUGUUA/UAAUUGGUCUCCAGAUAUU (Dharmacon; siGenome Smartpool).
Immunofluorescence and microscopy
U2OS or RPE-1 cells were grown on polylysine-coated coverslips in 24-well well plates. The cells were fixed with 4% paraformaldehyde/PBS, permeabilized with 0.5% Triton X-100/PBS and blocked in 3% BSA/PBS. The coverslips were incubated overnight at 4°C with primary antibodies in 1% BSA/PBS, and with secondary antibodies for 3 h at room temperature. The coverslips were incubated with DAPI for DNA staining. Images were acquired using a Leica TCS SPE laser-scanning confocal system mounted on a Leica DMI 4000B microscope, and equipped with an ACS APO 40 × 1.15NA oil objective (Leica, Wetzlar, Germany) and a live-imaging chamber (37°C, 5% CO2). For data analysis, ImageJ was used. All transformation of brightness or intensities were linear and consistently used on all data of the same experiment. The area of DNA was measured using the DAPI staining and the centromeric area was measured using an ACA staining. In both cases the borders were defined by manually tracking the boundary.
Immunoprecipitations
Cells were lysed using 50 mM Tris/HCl at pH 7.5, 300 mM NaCl, 0.5% Triton-X 100, 0.5 mM phenylmethanesulfonyl fluoride, 0.5 mM benzamidine, 5 μM leupeptin, 0.5 μM microcystin, 20 mM sodium fluoride and 1 mM sodium orthovanadate. After 15 min of incubation on ice and 10 min of centrifugation at 3,440 × g, the supernatant was used for SDS-PAGE or immunoprecipitation. For GFP-based immunoprecipitation, 25 µl of GFP nanobody beads (1:1 suspension in 0.1% NP-40/TBS) were added to the supernatant and incubated on a spinning wheel during 2–3 h at 4°C. The beads were washed once with 0.1 M LiCl/TBS and three times with 0.1% NP-40/TBS. Subsequently, SDS sample buffer was added and the samples were processed for western blot analysis (WB).
Chromosome spreads
U2OS cells were transfected with control siRNA (siC) or RepoMan siRNA (siRM) for 48 hrs. Cells were synchronized by treatment with 2 mM thymidine for 24hrs, followed by a release into fresh medium and a second block, 4 hrs after the release, with 0.33 µM nocodazole overnight. The next morning mitotic cells were harvested by mitotic shake off, after which the cells were treated with pre-warmed 0.075 M KCl containing 0.33 µM nocodazole for 10’ at 37°C. Cells were pelleted by centrifugation and fixed by three repeated cycles of dropwise addition of MeOH/acetic acid (3/1) followed by centrifugation. The obtained cell-pellet was dissolved in MeOH/acetic acid (3/1), drops were pipetted on a glass plate and dried in a humid chamber at 50°C. DNA was stained with DAPI and images were acquired using a Leica TCS SPE laser-scanning confocal system mounted on a Leica DMI 4000B microscope, equipped with an ACS APO 40 × 1.15NA oil objective (Leica, Wetzlar, Germany). For data analysis, ImageJ was used.
Antibodies
The following antibodies were used: anti-GFP (Santa Cruz Biotechnology, sc-9996, Dallas, TX, WB: 1:3.000); anti-histone H3 (Sigma-Aldrich, h0164, St. Louis, MO, WB: 1:5.000); anti-TPX2 (Novus Biologicals, NB500-179, Centennial, CO, WB 1:3.000, IF: 1:1.000); anti-α tubulin (Sigma-Aldrich, T6074, St. Louis, MO, WB: 1:50.000, IF: 1:4.000); anti-NuSAP1 (Proteintech, 12,024-1-AP, Rosemont, IL, WB: 1:3.000); anti-importin β (Genetex, GTX22811, Centennial, CO, WB: 1:10.000); anti-importin 7 (Novus Biologicals, NB100-1081, Centennial, CO, WB: 1:2.000); anti-importin α (Genetex, GTX106323, Centennial, CO, WB: 1:2.000); anti-histone H3 Thr3ph (Upstate Biotechnology Inc., 07–424, Lake Placid, NY, WB: 5.000, IF: 1:30.000); anti-RepoMan (Sigma-Aldrich, HPA030049, St. Louis, MO, WB: 1:2.000); anti-NuMA (Novus Biologicals, NB500-174, Centennial, WB: 1:2500); anti-HA (Covance, MMS-101P, Princeton, NJ, WB: 1:5.000); anti-Nup153 (Abcam, ab84872, Cambridge, WB: 1:5000); anti-Nup160 (Abcam, ab74147, Cambridge, WB: 1:2.500); anti-Kif22 (Sigma-Aldrich, HPA041076, St. Louis, MO, WB: 1:2.500); anti-RCC1 (Santa Cruz Biotechnology, sc-55,559, Dallas, TX, WB: 1:1.000); anti-Eb1 (BD Transduction Laboratories, 610,535, San Jose, CA, WB: 1:1000); anti-phospho-Ser/Thr-Pro, MPM-2 (Mitotic protein monoclonal #2) (Millipore, 05–368, Burlington, MA, WB: 1:1.000); ACA (autoantibody against centromere)(ImmunoVision, HCT-0100, IF: 1:3000); polyclonal swine anti-rabbit IgG/horseradish peroxidase (Dako, P0217, Santa Clara, CA, WB: 1:5.000); polyclonal rabbit anti-mouse IgG/horseradish peroxidase (Dako, P0260, Santa Clara, CA, WB: 1:5.000); polyclonal rabbit anti-goat IgG/ horseradish peroxidase (Dako, P0160, Santa Clara, CA, WB: 1:5.000); polyclonal goat anti-human IgG/ AlexaFluor 633 (ThermoFisher, A-21,091, Waltham, MA IF: 1:1000), polyclonal donkey anti-rabbit IgG/ AlexaFluor 555 (ThermoFisher, A-31,572, Waltham, MA IF: 1:1000).
Fractionation
HEK293T cells were lysed using 150 mM NaCl, 50 mM Tris/HCl at pH 7.5, 0.5% Triton-X, 0.5 mM benzamidine, 5 µM leupeptin and centrifuged at 1700 x g for 5 minutes. The supernatans was boiled with SDS sample buffer (= cytoplasmatic fraction) and the pellet was further lysed using 50 mM Tris/HCl at pH 7.5, 1.5 mM CaCl2, 150 units micronuclease, 4 units DNase I, 25 mM MgCl2, 0.5 mM benzamidine and 5 µM leupeptin, and incubated in a shaker for 30 minutes at 37°C. This fraction was boiled with SDS sample buffer (= chromatin-enriched fraction). Both fractions were analyzed by immunoblotting.
Abbreviations
CDK1, cyclin-dependent kinase 1
NuMA, nuclear mitotic apparatus protein 1
NuSAP1, nucleolar and spindle associated protein 1
MT, microtubule; PP1, protein phosphatase-1
SAF, spindle assembly factor
TPX2, targeting protein for Xklp2
Author contributions
SD and GV designed and performed the experiments, analysed the data and generated the figures. SD and MB wrote the manuscript. All authors approved the final version of the manuscript.
Supplemental Material
Download Zip (4.2 MB)Acknowledgments
This work was supported by the fund for Scientific Research-Flanders (grant G0B9917N) and a Flemish Concerted Research Action (GOA 15/016]. We thank Dr. Monique Beullens for advice during the initial stages of this research project.
Disclosure statement
The authors declare no competing interests
Data Availability Statement
No datasets were generated during the current study.
Supplementary material
Supplemental data for this article can be accessed here.
Additional information
Funding
References
- Prosser SL, Pelletier L. Mitotic spindle assembly in animal cells: a fine balancing act. Nat Rev Mol Cell Biol. [Internet]. 2017;18:187–201.
- Kollman JM, Merdes A, Mourey L, et al. Microtubule nucleation by γ-tubulin complexes. Nat Rev Mol Cell Biol. [Internet] 2011; 12:709–721. Available from: http://www.ncbi.nlm.nih.gov/pubmed/21993292
- Desai A, Mitchison TJ. Microtubule polymerization dynamics. Annu Rev Cell Dev Biol. [Internet] 1997; 13:83–117. Available from: http://www.annualreviews.org/doi/abs/10.1146/annurev.cellbio.13.1.83
- Job D, Valiron O, Oakley B. Microtubule nucleation. Curr Opin Cell Biol. 2003;15:111–117.
- Lüders J, Meunier S, Vernos I, et al. The Microtubule Cytoskeleton. Organisation, function and role in disease. Springer, Vienna. 2016. http://doi.org/10.1007/978-3-7091-1903-7
- Meunier S, Vernos I. Microtubule assembly during mitosis - from distinct origins to distinct functions? J Cell Sci. [Internet] 2012 [cited 2015 Jun 3]; 125:2805–2814. Available from http://www.ncbi.nlm.nih.gov/pubmed/22736044
- Meunier S, Vernos I. Acentrosomal microtubule assembly in mitosis: the Where, When, and How. Trends Cell Biol. [Internet]. 2016;26:80–87.
- Chen JWC, Barker AR, Wakefield JG. The ran pathway in drosophila melanogaster mitosis. Front Cell Dev Biol. [Internet]. 2015;3:1–20.
- Raemaekers T, Ribbeck K, Beaudouin J, et al. NuSAP, a novel microtubule-associated protein involved in mitotic spindle organization. J Cell Biol. [Internet] 2003 [cited 2015 Feb 26]; 162:1017–1029. Available from: http://www.pubmedcentral.nih.gov/articlerender.fcgi?artid=2172854&tool=pmcentrez&rendertype=abstract
- Ribbeck K, Groen AC, Santarella R, et al. NuSAP, a mitotic RanGTP target that stabilizes and cross-links microtubules. Mol Biol Cell. 2006;17:2646–2660.
- Ribbeck K, Raemaekers T, Carmeliet G, et al. Role for NuSAP in linking microtubules to mitotic chromosomes. Curr Biol. [Internet] 2007 [cited 2015 Mar 26]; 17:230–236. Available from: http://linkinghub.elsevier.com/retrieve/pii/S0960982206025681
- Song L, Rape M. Regulated degradation of spindle assembly factors by the anaphase-promoting complex. Mol Cell. [Internet]. 2010;38:369–382.
- Chou H-Y, Wang T-H, Lee S-C, et al. Phosphorylation of NuSAP by Cdk1 regulates its interaction with microtubules in mitosis. Cell Cycle. [Internet] 2011 [cited 2015 Mar 29]; 10:4083–4089. Available from: http://www.ncbi.nlm.nih.gov/pubmed/22101338
- Prévost M, Chamousset D, Nasa I, et al. Quantitative fragmentome mapping reveals novel, domain-specific partners for the modular protein RepoMan (recruits PP1 onto mitotic chromatin at anaphase). Mol Cell Proteomics. [Internet] 2013; 12:1468–1486. Available from]. ;:. : http://www.pubmedcentral.nih.gov/articlerender.fcgi?artid=3650352&tool=pmcentrez&rendertype=abstract
- Qian J, Lesage B, Beullens M, et al. PP1/repo-man dephosphorylates mitotic histone H3 at T3 and regulates chromosomal aurora B targeting. Curr Biol. 2011;21:766–773.
- Qian J, Beullens M, Lesage B, et al. Aurora B defines its own chromosomal targeting by opposing the recruitment of the phosphatase scaffold Repo-Man. Curr Biol. [Internet]. 2013;23:1136–1143.
- Qian J, Beullens M, Huang J, et al. Cdk1 orders mitotic events through coordination of a chromosome-associated phosphatase switch. Nat Commun. [Internet] 2015; 6:1–13.
- Kelly AE, Funabiki H. Correcting aberrant kinetochore microtubule attachments: an Aurora B-centric view. Curr. Opin. Cell Biol. 200921:51–58.
- de Castro IJ, Budzak J, Di Giacinto ML, et al. Repo-Man/PP1 regulates heterochromatin formation in interphase. Nat Commun. [Internet] 2017; 8:1–16. Available from: http://www.nature.com/doifinder/10.1038/ncomms14048
- Vagnarelli P, Hudson DF, Ribeiro SA, et al. Condensin and Repo-Man-PP1 co-operate in the regulation of chromosome architecture during mitosis. Nat Cell Biol. [Internet] 2006 [cited 2015 Feb 3]; 8:1133–1142. Available from : http://www.pubmedcentral.nih.gov/articlerender.fcgi?artid=2741681&tool=pmcentrez&rendertype=abstract
- Vagnarelli P, Ribeiro S, Sennels L, et al. Repo-Man coordinates chromosomal reorganization with nuclear envelope reassembly during mitotic exit. Dev Cell. [Internet] 2011 [cited 2015 Feb 5]; 21:328–342. Available from: http://www.pubmedcentral.nih.gov/articlerender.fcgi?artid=3480639&tool=pmcentrez&rendertype=abstract
- Vagnarelli P, Earnshaw WC, Vagnarelli P, et al. Repo-Man-PP1 A link between chromatin remodelling and nuclear envelope reassembly. Nucleus. 2012;3:138–142.
- Vagnarelli P. Repo-man at the intersection of chromatin remodelling, DNA repair, nuclear envelope organization, and cancer progression. Cancer Biol Nucl Envel. [Internet] 2014; 773:401–414. Available from: http://link.springer.com/10.1007/978-1-4899-8032-8
- Wang Y, Zhang H, Gigant B, et al. Structures of a diverse set of colchicine binding site inhibitors in complex with tubulin provide a rationale for drug discovery. Febs J. 2016;283:102–111.
- Brier S, Lemaire D, DeBonis S, et al. Molecular dissection of the inhibitor binding pocket of mitotic kinesin Eg5 reveals mutants that confer resistance to antimitotic agents. J Mol Biol. 2006;360:360–376.
- De Munter S, Görnemann J, Derua R, et al. Split-BioID: a proximity-biotinylation assay for dimerization-dependent protein interactions. FEBS Lett. [Internet]. 2016;591:415–424.
- Hein MY, Hubner NC, Poser I, et al. A human interactome in three quantitative dimensions organized by stoichiometries and abundances. Cell. 2015;163:712–723.
- Clarke PR, Zhang C. Spatial and temporal coordination of mitosis by Ran GTPase. Nat Rev Mol Cell Biol. [Internet] 2008 [cited 2015 Jun 14]; 9:464–477. Available from: http://www.ncbi.nlm.nih.gov/pubmed/18478030
- Trinkle-Mulcahy L, Andersen J, Lam YW, et al. Repo-Man recruits PP1 gamma to chromatin and is essential for cell viability. J Cell Biol. [Internet] 2006 [cited 2015 Feb 3]; 172:679–692. Available from: http://www.pubmedcentral.nih.gov/articlerender.fcgi?artid=2063701&tool=pmcentrez&rendertype=abstract
- Bakhoum SF, Thompson SL, Manning AL, et al. Genome stability is ensured by temporal control of kinetochore – microtubule dynamics. Nat Cell Biol. 2009;11:27–35.
- Salic A, Waters JC, Mitchison TJ. Vertebrate Shugoshin Links sister centromere cohesion and kinetochore microtubule stability in mitosis. Cell. 2004;118:567–578.
- Salmon ED, Mckeel M, Hays T. Rapid rate of tubulin dissociation from microtubules in the mitotic spindle in vivo measured by blocking polymerization with colchicine. J Cell Biol. 1984;99:1066–1075.
- Zhai Y, Kronebusch PJ, Borisy GG. Kinetochore microtubule dynamics and the metaphase-anaphase transition. J Cell Biol. 1995;131:721–734.
- Mitchison TJ, Salmon ED. Poleward kinetochore fiber movement occurs during both metaphase and anaphase-A in newt lung cell mitosis. J Cell Biol. 1992;119:569–582.
- Mitchison TJ. Polewards microtubule flux in the mitotic spindle: evidence from Photoactivation of Fluorescence. J Cell Biol. 1989;109:637–652.
- Deluca JG, Gall WE, Ciferri C, et al. Kinetochore microtubule dynamics and attachment stability are regulated by Hec1. Cell. 2006;127:969–982.