ABSTRACT
The oncosuppressor protein p53 plays a major role in transcriptionally controlling the expression of a number of genes, which in turn regulates many functions in response to DNA damage, oncogene triggering, oxidative, and additional cell stresses. A developing area of interest in p53 is the studies related to its cytoplasmic function(s). Many investigations revealed the significant role of p53 in the cytoplasm, acting in a transcriptional-independent manner in important processes related to cell homeostasis such as; apoptosis, autophagy, metabolism control, drug, and oxidative stress response. The studies on cytoplasmic p53 have shown intricate mechanisms by which posttranslational modifications allow p53 to perform its cytoplasmic functions. A number of ubiquitins, deubiquitins, and small ubiquitin-like proteins, have a pivotal role in controlling cytoplasmic stability and localization. Recently, HOPS/TMUB1 a novel small ubiquitin-like protein has been described as a vital molecule stabilizing p53 half-life, directing it to the mitochondria and favoring p53-mediated apoptosis. Furthermore, HOPS/TMUB1 competing with importin-α lessens p53 nuclear localization, thereby increasing cytoplasmic concentration. HOPS/TMUB1 as p53 modifiers could be attractive candidates to elucidate apoptosis or other important transcriptional-independent functions which are key in cancer research in order to develop new therapeutic approaches.
Introduction
p53 is certainly one of the most studied proteins in biology and pathology. p53 governs the pathways that suppress cancer-cell growth by mediating cell-cycle arrest, apoptosis, and senescence [Citation1,Citation2]. p53 mutations are present in more of 50% of all cancers and inactivation of the p53 pathway is found in a high percentage of tumors derived from all tissues [Citation3]. The majority of p53 functions are played by its transcription factor activity, which in turn transcribes a number of genes that determine the p53 oncosuppressor activity. Indeed, other important roles have been attributed to p53 in the control of cell homeostasis such as autophagy, metabolism, and antioxidant activity [Citation4]. Other than transcriptional activity, a growing number of studies is describing important p53 functions independent of its transcriptional ability, differentiating between nuclear and cytoplasmic effects [Citation5,Citation6] ().
Figure 1. Schematic representation of the different activities of p53
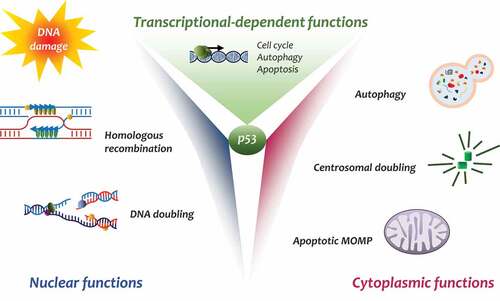
Cytoplasmic p53
The nuclear transcriptional activity of p53 is well studied, less is known about its cytoplasmic role. Many studies indicate p53 accumulation in the cytoplasm is essential to perform its functions. p53 ubiquitination and degradation occur in the cytoplasm. Here, p53 mainly induces apoptosis via MOMP, allows centrosome duplication, and has an important role in controlling cell autophagy [Citation7,Citation8]. Many studies have shown that, following stress stimuli, p53 moves from nucleus to cytoplasm via nuclear-export receptor CRM1 upon activation of FOXO3a [Citation9]. To perform its cytoplasmic functions, p53 – as an oligomeric protein – is subjected to a number of posttranslational modifications allowing its distribution in different regions of the cytoplasm [Citation10]. The p53 destiny in the cytoplasm is complex and, presently, not well defined. p53 posttranslation modifications such as phosphorylation, conformational modifications, acetylation, or ubiquitylation are fundamental to sustain p53’s cytoplasmic activities [Citation11]. Many studies have highlighted p53 shuttling from the nucleus to cytoplasm and its subsequent increase in the cytoplasm and the mitochondria after DNA damage. DNA damage signaling triggers homeodomain-interacting protein kinase-2 (HIPK2), which in turn phosphorylates p53 on Serine46 [Citation12]. This event has been well studied, the phosphorylation requires the recruitment of a phospho-specific prolyl-isomerase, Pin1, which binds phospho-p53 causing conformational changes that reduce p53-MDM2 affinity in the nucleus and the cytoplasm [Citation13]. Modified p53 acquires the ability to bind MDM4 through BCL-2 activated MOMP [Citation14]. Indeed, HIPK1 and Pin1 cooperate to promote translocation of p53 to the mitochondria to prompt direct apoptosis [Citation15]. Notably, other important post-translational modifications, such as acetylation, appear to be significant in controlling p53 cytoplasmic functions [Citation16]. Acetylated p53 at Lysine120 has been found in the mitochondria allowing mitochondrial apoptosis, demonstrating that p53 modifications are relevant in its transcription-independent functions [Citation17,Citation18].
P53 ubiquitin and modifier
Ubiquitylation represents one of the most important posttranslational modifications that occur to p53. Undoubtedly, p53 degradation happens in the cytoplasm by proteasome machinery, but posttranslational modifications, such as mono and multi mono-ubiquitylation, allow p53 to localize in different cytoplasmic compartments. MDM2 is the most important E3 in regulating p53 half-life [Citation19,Citation20] and has a preeminent role in regulating posttranslational modifications. The MDM2 level in the cell is crucial for p53 stability, low MDM2 levels enhance p53 half-life, while high MDM2 levels drive proteasome-mediated degradation [Citation21]. Moreover, the role played by HAUSP in controlling mitochondrial p53 levels by deubiquitylation [Citation22–24] must be considered. Indeed, after the discovery of HAUSP, a number of deubiquitins regulating p53 has been described [Citation25–30]. Indeed, the fine regulation of cytoplasmic levels of p53 is more complex than just MDM2-dependent concentration. Many functions exerted by p53 are directly related to its half-life in the cell and p53 protein stabilization is a determinant factor for its roles in the nucleus and cytoplasm. However, while the principal role of MDM2 as p53-E3 ubiquitin-ligase is clear, many studies have identified a number of molecules playing a role in p53 ubiquitylation. Among them, there are at least 15 proteins that can act as a MDM2-independent p53 E3-ligase. These p53-E3 ubiquitin ligases show different conformational structures and domains and some of them, such as E4F1 and UBC13, lack typical E3 domains [Citation31]. Aside from the poly-ubiquitylation resulting in p53 degradation as a control mechanism of p53 stability, other molecules such as Ubiquitin-Like modifiers (UBL) are important not only in p53 stability, but also in its cytoplasmic localization [Citation32]. Many investigations have revealed the role of Small Ubiquitin-like MOdifier (SUMO) in regulating p53 activity. SUMO proteins conjugated via an isopeptide bond to lysine 386 of p53 at its C-terminal regulatory domain. Some authors suggest that SUMOylation has a possible role in favoring p53 nuclear export to the cytoplasm, allowing p53 accumulation in physiological or pathological functions [Citation33]. As of now, this is a point currently under debate. p53 SUMOylation in the nucleus has been described as a mechanism involved in lessening p53 transcriptional activity and allowing p53 enrichment in nuclear bodies. Moreover, p53 SUMOylation has been observed to have an important role in the pathogenesis of atherosclerosis [Citation34]. Other than SUMO, another known p53 modification by UBL, Neural precursor cell Expressed Developmentally Downregulated protein 8 (NEDD8) has been defined [Citation35]. Interestingly, NEDDylation on p53 utilizes different E3s depending on the lysine residues; when NEDDylation occurs at C-terminus lysines, K370, K372, K373 [Citation36], it involves Mdm2, while FBXO11 promotes NEDDylation in lysines K320 and K321 [Citation37]. p53 NEDDylation by Mdm2 or FBXO11 leads to a reduction in transcriptional activity.
HOPS/TMUB1 in brief
Recently, a novel UBL modifier, Hepatocyte Odd Shuttling Protein/Trans Membrane Ubiquitin 1 (HOPS/TMUB1) has been identified in controlling p53 stability and cytoplasmic accumulation [Citation38]. As observed, HOPS seems to play a pivotal role in directing p53-mediated mitochondrial apoptosis in response to DNA damage, suggesting an involvement in the fine-tuning of p53 activity control (). HOPS/TMUB1 (from hereinafter HOPS) is a ubiquitous shuttling protein characterized by a ubiquitin-like (UBL) domain, three transmembrane segments, with proline-rich and C-terminus leucine-rich regions [Citation39]. Hops transcribes a single mRNA, which codifies for three proteins with different molecular weights. HOPS localization in cells is primarily in the nucleus, but during proliferation or after stress stimuli, such as genotoxic or oxidative agents, HOPS migrates to the cytoplasm. Hops has been isolated in liver regeneration together with other novel genes [Citation40,Citation41]. In normal livers, HOPS is mostly present in the nucleus in quiescent hepatocytes, but after partial hepatectomy, it rapidly migrates in the cytoplasm where it remains until the end of the first M-phase. It has been demonstrated that cAMP or EGF are the major factors involved in HOPS shuttling. Similar results have been obtained in hepatoma cells induced to proliferate or arrested with serum deprivation. HOPS contains a Nuclear Export Signal (NES) domain that allows the export outside the nucleus through the exportin Chromosome Region Maintenance 1 (CRM-1) [Citation42].
Figure 2. HOPS crosstalk with other p53 modulators
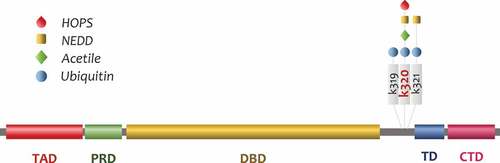
Interestingly, it has been demonstrated that in the cytoplasm, HOPS plays a role in mitotic spindle assembly and centrosome duplication and its reduction in the cell leads to altered mitosis figures with multipolar spindle and cytokinesis failure [Citation43]. HOPS has been found to participate in the ERAD pathway, playing an important role in the control of cholesterol biosynthetic enzyme HMG-CoA reductase [Citation44]. HOPS is abundantly expressed in the brain, where it plays a role in the regulation of basal synaptic transmission [Citation45,Citation46]. Notably, HOPS has been shown to interact and control the stabilization of the tumor suppressor p19Arf. It has been demonstrated that HOPS not only acts as a stabilizer of p19Arf, but regulates its nucleolar localization and mediates the interaction with the oncosuppressor protein nucleophosmin (NPM) [Citation47]. Moreover, it has been demonstrated that p19Arf stabilization by HOPS results in p53 overexpression. However, the presence of a UBL in the HOPS structure supports the idea of direct involvement of UBL as a modifier in p19Arf survival (Manuscript in preparation).
Recently, the HOPS-Knockout mouse model has been developed. Hops−/- mouse is viable at birth, even if they present a slightly reduced Mendelian frequency with respect to Hops+/+ mouse [Citation38].
HOPS and p53
Importantly, when the Hops−/- mice are treated with DNA damage drugs such as etoposide or campothecin, they are resistant to apoptosis due to reduced activation of p53. A link between lack of HOPS and altered p53 response has been established through analyzing the reduced p53 response after etoposide treatment. In particular, a direct binding between p53 and the UBL domain of HOPS has been shown, which defines a prolonged half-life in p53. Mutational analysis in the terminal glycine of HOPS-UBL (G176) demonstrates the reduction in the ability of HOPS to sustain p53 stability. The decreased contribution of p53 in apoptosis in Hops−/- mouse has been analyzed in vivo and in vitro to understand the involvement of HOPS in apoptosis. As above described, HOPS is a shuttling protein that migrates to the cytoplasm, upon stress or in proliferation. After etoposide treatment, it has been found that HOPS and p53 migrate and their binding increases in the cytoplasm. Both proteins utilize the exportin CRM-1 to shuttle to the cytoplasm [Citation38,Citation42,Citation48]. In particular, both proteins show accumulation in the mitochondria. Notably, comparing the p53 mitochondrial levels in Hops−/- mice after etoposide treatment, a reduced amount of p53 in the thymus was found in the spleen and testis; this indicates an important contribution of HOPS in maintaining the mitochondrial p53 levels which in turn directs apoptosis after DNA damage. When overexpressed HOPS not only increases p53 levels, but it competes with importin-α in binding to lysine-320 of the p53 NLS, restraining p53 import and promoting its accumulation in the cytoplasm [Citation38] (). Interestingly, as HOPS and p53 also bind in the nucleus, many investigations are directed toward understanding the contribution of HOPS in regulating the transcriptional activity of p53; this would establish the role of HOPS in p53-related transcriptional dependent and independent apoptosis.
Figure 3. Overview of HOPS impact on p53 activity in the cellHOPS is concerned in lengthening of p53 half-life inhibiting MDM2 E3 ligase activity. Upon DNA damage stresses, HOPS enhances p53 stability sustaining p53 cytoplasmic pool. Cytoplasmic p53 is than available for mitochondrial translocation and MOMP induction for apoptotic outcome. Moreover, HOPS can modulate importin α binding to p53, responsible for p53 nuclear import, thus reducing p53 transcriptional activation (upper panel). In the absence of HOPS apoptotic response to DNA damage inputs is reduced. In HOPS−/- models p53 half-life is shortened leading to cytoplasmic pool drop and in turn reduced mitochondrial apoptosis. Importin α binding to p53 is not hampered by HOPS competition and nuclear import is increased (bottom panel)
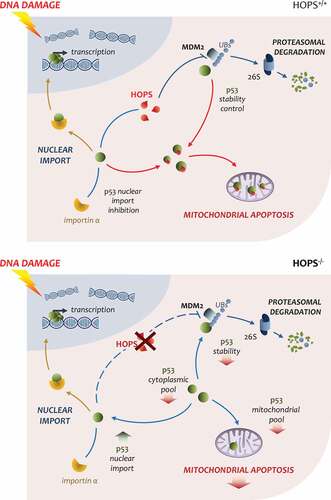
Conclusions
The discovery of the cytoplasmic role of p53 in apoptosis and other important systems such as autophagy and metabolism is critical for cancer therapeutics. Indeed, the transcriptional-independent role of p53 in apoptosis has been well studied, but controversial results have been observed in how p53 drives mitochondrial apoptosis. Many difficulties derive from the limited information regarding how p53 orchestrates its functions toward a specific pathway rather than another [Citation49,Citation50]. To this end, HOPS has been found not only to increase the p53 stability, but localizes p53 to the mitochondria to facilitate mitochondrial apoptosis.
HOPS similarly to other modifiers, such as SUMO and NEDD, seems to play an important role in controlling the cytoplasmic function of p53. The posttranslation modifications of p53 are of great and growing interest in cancer research to address deeper understanding of this function with the aim of utilizing them in therapeutic approaches. Many data indicate HOPS is an important regulator of p53 in cytoplasmic functions and adds another solid contribution to better understand the intricate and fascinating role of p53 in the cell.
Besides SUMOylation or NEDDylation, the HOPSylation may have a novel and significant function not only related to p53, but other HOPS targets are to be discovered to better understand the HOPS role in biology and pathology.
Acknowledgments
We wish to thank the members of our laboratory, Damiano Scopetti, Simona Ferracchiato, Nicola Di Iacovo, and Silvano Pagnotta for the fruitful discussions and support. The original studies in the authors’ own laboratory were supported in part by Associazione Umbra Contro il Cancro-AUCC, Fondazione Cassa di Risparmio di Perugia-FCRP and Comitato Maria Grazia Frasconi. G.S. is recipient of a PRIN 2015 Project 20152CB22L_004.
Disclosure statement
No potential conflict of interest was reported by the author.
Additional information
Funding
References
- Li T, Kon N, Jiang L, et al. Tumor suppression in the absence of p53-mediated cell-cycle arrest, apoptosis, and senescence. Cell. 2012;149:1269–1283.
- Oren M. Decision making by p53: life, death and cancer. Cell Death Differ. 2003;10:431–442.
- Muller PA, Vousden KH. p53 mutations in cancer. Nat Cell Biol. 2013;15:2–8.
- Vousden KH, Prives C. Blinded by the light: the growing complexity of p53. Cell. 2009;137:413–431.
- Comel A, Sorrentino G, Capaci V, et al. The cytoplasmic side of p53’s oncosuppressive activities. FEBS Lett. 2014;588:2600–2609.
- Green DR, Kroemer G. Cytoplasmic functions of the tumour suppressor p53. Nature. 2009;458:1127–1130.
- Contadini C, Monteonofrio L, Virdia I, et al. p53 mitotic centrosome localization preserves centrosome integrity and works as sensor for the mitotic surveillance pathway. Cell Death Dis. 2019;10:850.
- Tasdemir E, Maiuri MC, Galluzzi L, et al. Regulation of autophagy by cytoplasmic p53. Nat Cell Biol. 2008;10:676–687.
- You H, Yamamoto K, Mak TW. Regulation of transactivation-independent proapoptotic activity of p53 by FOXO3a. Proc Natl Acad Sci U S A. 2006;103:9051–9056.
- Hock AK, Vousden KH. The role of ubiquitin modification in the regulation of p53. Biochim Biophys Acta. 2014;1843:137–149.
- Liu Y, Tavana O, Gu W. p53 modifications: exquisite decorations of the powerful guardian. J Mol Cell Biol. 2019;11:564–577.
- D’Orazi G, Cecchinelli B, Bruno T, et al. Homeodomain-interacting protein kinase-2 phosphorylates p53 at Ser 46 and mediates apoptosis. Nat Cell Biol. 2002;4:11–19.
- Zacchi P, Gostissa M, Uchida T, et al. The prolyl isomerase Pin1 reveals a mechanism to control p53 functions after genotoxic insults. Nature. 2002;419:853–857.
- Mancini F, Di Conza G, Pellegrino M, et al. MDM4 (MDMX) localizes at the mitochondria and facilitates the p53-mediated intrinsic-apoptotic pathway. Embo J. 2009;28:1926–1939.
- Sorrentino G, Mioni M, Giorgi C, et al. The prolyl-isomerase Pin1 activates the mitochondrial death program of p53. Cell Death Differ. 2013;20:198–208.
- Wu SY, Chiang CM. Crosstalk between sumoylation and acetylation regulates p53-dependent chromatin transcription and DNA binding. Embo J. 2009;28:1246–1259.
- Nemajerova A, Erster S, Moll UM. The post-translational phosphorylation and acetylation modification profile is not the determining factor in targeting endogenous stress-induced p53 to mitochondria. Cell Death Differ. 2005;12:197–200.
- Sykes SM, Stanek TJ, Frank A, et al. Acetylation of the DNA binding domain regulates transcription-independent apoptosis by p53. J Biol Chem. 2009;284:20197–20205.
- Honda R, Tanaka H, Yasuda H. Oncoprotein MDM2 is a ubiquitin ligase E3 for tumor suppressor p53. FEBS Lett. 1997;420:25–27.
- Momand J, Zambetti GP, Olson DC, et al. The mdm-2 oncogene product forms a complex with the p53 protein and inhibits p53-mediated transactivation. Cell. 1992;69:1237–1245.
- Kulikov R, Letienne J, Kaur M, et al. Mdm2 facilitates the association of p53 with the proteasome. Proc Natl Acad Sci U S A. 2010;107:10038–10043.
- Kon N, Kobayashi Y, Li M, et al. Inactivation of HAUSP in vivo modulates p53 function. Oncogene. 2010;29:1270–1279.
- Li M, Brooks CL, Kon N, et al. A dynamic role of HAUSP in the p53-Mdm2 pathway. Mol Cell. 2004;13:879–886.
- Li M, Chen D, Shiloh A, et al. Deubiquitination of p53 by HAUSP is an important pathway for p53 stabilization. Nature. 2002;416(6881):648–653.
- Fu S, Shao S, Wang L, et al. USP3 stabilizes p53 protein through its deubiquitinase activity. Biochem Biophys Res Commun. 2017;492(2):178–183.
- Ke JY, Dai CJ, Wu WL, et al. USP11 regulates p53 stability by deubiquitinating p53. J Zhejiang Univ Sci B. 2014;15:1032–1038.
- Liu H, Li X, Ning G, et al. The machado-joseph disease deubiquitinase ataxin-3 regulates the stability and apoptotic function of p53. PLoS Biol. 2016;14:e2000733.
- Luo J, Lu Z, Lu X, et al. OTUD5 regulates p53 stability by deubiquitinating p53. PLoS One. 2013;8:e77682.
- Piao S, Pei HZ, Huang B, et al. Ovarian tumor domain-containing protein 1 deubiquitinates and stabilizes p53. Cell Signal. 2017;33:22–29.
- Tu R, Kang W, Yang X, et al. USP49 participates in the DNA damage response by forming a positive feedback loop with p53. Cell Death Dis. 2018;9:553.
- Le Cam L, Linares LK, Paul C, et al. E4F1 is an atypical ubiquitin ligase that modulates p53 effector functions independently of degradation. Cell. 2006;127:775–788.
- Marchenko ND, Wolff S, Erster S, et al. Monoubiquitylation promotes mitochondrial p53 translocation. Embo J. 2007;26:923–934.
- Liu G, Xirodimas DP. NUB1 promotes cytoplasmic localization of p53 through cooperation of the NEDD8 and ubiquitin pathways. Oncogene. 2010;29:2252–2261.
- Dehnavi S, Sadeghi M, Penson PE, et al. The role of protein SUMOylation in the pathogenesis of atherosclerosis. J Clin Med. 2019;8. DOI:10.3390/jcm8111856
- Rabut G, Peter M. Function and regulation of protein neddylation. ‘Protein modifications: beyond the usual suspects’ review series. EMBO Rep. 2008;9:969–976.
- Xirodimas DP, Saville MK, Bourdon JC, et al. Mdm2-mediated NEDD8 conjugation of p53 inhibits its transcriptional activity. Cell. 2004;118:83–97.
- Abida WM, Nikolaev A, Zhao W, et al. FBXO11 promotes the Neddylation of p53 and inhibits its transcriptional activity. J Biol Chem. 2007;282:1797–1804.
- Castelli M, Piobbico D, Chiacchiaretta M, et al. HOPS/TMUB1 retains p53 in the cytoplasm and sustains p53-dependent mitochondrial apoptosis. EMBO Rep. 2020;21:e48073.
- Castelli M, Piobbico D, Bartoli D, et al. Different functions of HOPS isoforms in the cell: HOPS shuttling isoform is determined by RIP cleavage system. Cell Cycle. 2014;13:293–302.
- Bellet MM, Piobbico D, Bartoli D, et al. NEDD4 controls the expression of GUCD1, a protein upregulated in proliferating liver cells. Cell Cycle. 2014;13:1902–1911.
- Della Fazia MA, Piobbico D, Bartoli D, et al. lal-1: a differentially expressed novel gene during proliferation in liver regeneration and in hepatoma cells. Genes Cells. 2002;7:1183–1190.
- Della Fazia MA, Castelli M, Bartoli D, et al. HOPS: a novel cAMP-dependent shuttling protein involved in protein synthesis regulation. J Cell Sci. 2005;118:3185–3194.
- Pieroni S, Della Fazia MA, Castelli M, et al. HOPS is an essential constituent of centrosome assembly. Cell Cycle. 2008;7:1462–1466.
- Jo Y, Sguigna PV, DeBose-Boyd RA. Membrane-associated ubiquitin ligase complex containing gp78 mediates sterol-accelerated degradation of 3-hydroxy-3-methylglutaryl-coenzyme A reductase. J Biol Chem. 2011;286:15022–15031.
- Yang H, Takagi H, Konishi Y, et al. Transmembrane and ubiquitin-like domain-containing protein 1 (Tmub1/HOPS) facilitates surface expression of GluR2-containing AMPA receptors. PLoS One. 2008;3:e2809.
- Zhang W, Savelieva KV, Suwanichkul A, et al. Transmembrane and ubiquitin-like domain containing 1 (Tmub1) regulates locomotor activity and wakefulness in mice and interacts with CAMLG. PLoS One. 2010;5:e11261.
- Castelli M, Pieroni S, Brunacci C, et al. Hepatocyte odd protein shuttling (HOPS) is a bridging protein in the nucleophosmin-p19 Arf network. Oncogene. 2013;32:3350–3358.
- Freedman DA, Levine AJ. Nuclear export is required for degradation of endogenous p53 by MDM2 and human papillomavirus E6. Mol Cell Biol. 1998;18:7288–7293.
- Hafner A, Bulyk ML, Jambhekar A, et al. The multiple mechanisms that regulate p53 activity and cell fate. Nat Rev Mol Cell Biol. 2019;20:199–210.
- Murray-Zmijewski F, Slee EA, Lu X. A complex barcode underlies the heterogeneous response of p53 to stress. Nat Rev Mol Cell Biol. 2008;9:702–712.