ABSTRACT
The endothelialization of endothelial progenitor cells (EPCs) was proven to facilitate the vascular repair of aneurysm. MiR-17-5p regulated angiogenesis in various cancers. This research focused on exploring the effect of miR-17-5p on EPCs and the vascular repair of aneurysm. In vivo study: the aneurysm rat model was established and treated with AgomiR-17-5p; the histopathology of aneurysm tissues was examined by hematoxylin-eosin staining; and the level of EPCs in the aneurysm tissues and peripheral blood of rats were evaluated by immunofluorescence and flow cytometry, respectively. In vitro study: EPCs were cultured and identified using flow cytometry; the target of miR-17-5p was proven by dual-luciferase reporter assay; after transfection, the viability, migration, and tube formation of the EPCs were detected by MTT, wound healing, and tube formation assays, respectively; the expressions of VEGFA and factors related to PTEN-mediated PI3K/AKT pathway were detected by ELISA, qPCR, or Western blot as needed. In vivo study: miR-17-5p overexpression promoted the vascular repair in aneurysm rats and increased the level of EPCs in the aneurysm tissues and peripheral blood of the rats. In vitro study: miR-17-5p overexpression promoted the viability, migration, and tube formation of EPCs, up-regulated the expressions of VEGFA, p-PI3K, and p-AKT, and down-regulated the PTEN expression in EPCs; miR-17-5p silencing did the opposite; PTEN was targeted by miR-17-3p and further abrogated the effects of miR-17-5p overexpression on EPCs. MiR-17-5p promoted the endothelialization of EPCs to facilitate the vascular repair of aneurysm by regulating PTEN-mediated PI3K/AKT/VEGFA pathway.
Introduction
Aortic aneurysm is defined as a pathological condition characterized by permanent dilatation of the aortic wall, which generally occurs in the infra-renal and proximal thoracic regions [Citation1]. Although usually asymptomatic, progressive aneurysm dilatation is associated with the devastating consequences of aortic rupture [Citation1]. If the progression of aortic aneurysm is not controlled, rupture and bleeding can occur in the late stage, and the mortality rate of emergency patients is as high as 90% [Citation2]. At present, the prevention of aortic rupture in aortic aneurysm is limited to surgical repair, and there is no effective pharmaceutical or novel treatment to prevent progressive growth or rupture [Citation1,Citation3]. Therefore, safer and more effective methods for the prevention and treatment of aortic aneurysm are urgently needed.
Endothelial progenitor cells (EPCs) are a class of multipotent stem/progenitor cells that exist in the bone marrow and peripheral blood, and are the precursor cells of endothelial cells [Citation4–6]. Previous studies have proved that EPCs play an irreplaceable role in vascular endothelial repair and angiogenesis, owing that EPCs not only have the surface markers and proliferation ability of stem cells that can differentiate into mature endothelial cells, but also display pathological chemotaxis under the action of specific cytokines, which enables them to quickly concentrate on the vascular injury or intimal defect, and then participate in the repair and regeneration of blood vessels [Citation5,Citation7,Citation8]. Therefore, boosting the endothelialization of EPCs in aneurysm tissues might become a novel approach for aneurysm treatment.
Since miRNAs were proved to pose a crucial regulatory effect on a series of physiological and pathological processes, many researchers have started to focus on their regulatory effect in the process of aneurysm [Citation9–12]. For example, miR-195 inhibited the progression of abdominal aortic aneurysm by regulating TNF-α/NF-κB pathway [Citation11]; miR-574-5p acts as a circulating marker for thoracic aortic aneurysm [Citation9]; miR-31a-5p can induce the endothelialization of EPCs in an aneurysm neck after coil embolization [Citation13]. As an oncogene, miR-17-5p was previously discovered to be abnormally expressed in vascular diseases such as thoracic aortic dissection and aneurysm, and it is also involved in the functions of vascular endothelial cells [Citation14–16]. However, the specific role of miR-17-5p in aneurysm and its effect on the endothelialization of EPCs are still unclear.
Therefore, in this study, we established aortic aneurysm rat model and cultured primary EPCs, and then treated the rats and cells with miR-17-5p in an attempt to investigate the effect of miR-17-5p on the endothelialization of EPCs and the vascular repair of aneurysm.
Methods
Animal and ethics statement
Four male SD rats (age: 3 weeks, weight: 80–100 g) and 26 male SD rats (age: 10 weeks, weight: 280–300 g) were bought from ALF Biotechnology (Jiangsu, China). All the rats were raised with a 12 h of light/12 h of dark cycle under an SPF environment and given free access to food and water. Before experiments, the rats were fed for 5 days to adapt to the environment. All animal experiments in this study were approved by the Committee of Experimental Animals of The First Affiliated Hospital Of Xinjiang Medical (T2019090805X) and also performed in the First Affiliated Hospital Of Xinjiang Medical.
Establishment of aneurysm model and animal grouping
To determine the effect of miR-17-5p in aneurysm, the aneurysm rat model was established and further treated with AgomiR-17-5p . The twenty-six male SD rats (age: 10 weeks, weight: 280–300 g) were used for the establishment of aortic aneurysm model: two rats were used as abdominal aorta donors, and the other 24 rats were used in the later establishment of aneurysm model. All traumatic operations were performed after the rats were anesthetized by isoflurane (R510-22-4, RWD, Shenzhen, China) inhalation.
The aortic aneurysm rat model was established according to the previous research [Citation13]. In brief, the abdominal aorta segment was obtained from two healthy SD rats and used as a donor segment. Then the surgery was performed: the proximal area of the aneurysm was constricted by partial ligation to create an aneurysm neck, and the distal region of the anastomosing vessel segment was completely ligated. After distal ligation, a coiled aneurysm model was created via Guglielmi detachable coil embolization.
The other 24 male SD rats were randomly divided into four groups: the Sham group (n = 6), the Model group (n = 6), the Model+AgomiR-NC group (n = 6), and the Model+AgomiR-17-5p group (n = 6). The rats in the Sham group received inhalation anesthesia without surgical intervention, and were injected with normal saline (F11445; OKA, Beijing, China) via the tail vein on days 0, 7, 14, 21, and 28. The rats in the Model group were treated surgically to establish the aneurysm model, and were injected with normal saline via the tail vein on days 0, 7, 14, 21, and 28. The rats in the Model+AgomiR-NC were treated surgically to establish the aneurysm model, and were injected with 10 mg/kg AgomiR-NC via the tail vein on days 0, 7, 14, 21, and 28. The rats in the Model+AgomiR-17-5p were treated surgically to establish the aneurysm model, and were injected with 10 mg/kg AgomiR-17-5p via the tail vein on days 0, 7, 14, 21, and 28. AgomiR-NC (5ʹ-CAGUCCUUUUGUGUAGUACAA-3ʹ) and AgomiR-17-5p (5ʹ-CUACCUGCACUGUAAGCACUUUG-3ʹ) were synthesized by RIBOBIO (Guangzhou, China).
On days 10, 20, and 30, the peripheral blood of the rats was collected for the detection of circulating EPCs by flow cytometry. On day 30, after the rats were anesthetized with 150 mg/kg of 0.2% phenobarbital sodium and sacrificed by cervical dislocation, the aneurysm tissue of the rats was harvested for hematoxylin-eosin staining and immunofluorescence.
Hematoxylin-eosin staining
The aneurysm tissue was incubated with paraformaldehyde fixative (30,525–89-4, Rhawn, Shanghai) and embedded in paraffin (A13912, OKA). After being sectioned (5 μm) and dewaxed, the tissue was stained with hematoxylin (E12218, OKA) for 10 min and soaked in hydrochloric acid alcohol (C0163M, Beyotime, Shanghai) for 10 s. Then, the tissue was dyed with eosin (R20589, OKA) for 1 min. Subsequently, the tissue was transparentized by xylene (ALFL13317, OKA) and sealed with neutral gum (D11029, OKA). Finally, the tissue was observed and photographed using an automatic microscope (DMLA; Leica, Solms, Germany) at a magnification of ×200.
Immunofluorescence
The aneurysm tissue was incubated with paraformaldehyde fixative and embedded in paraffin. After being sectioned (5 μm) and dewaxed, the tissue was permeabilized with 0.5% Triton X-100 (T8787, Sigma-Aldrich, St. Louis, Missouri, USA). Then, the tissue was incubated with vWF antibody (11,778-1-AP, Proteintech, Wuhan, China) or KDR antibody (26,415-1-AP, Proteintech) overnight at 4°C. The second day, after being washed with PBS (10,010,049, Gibco, Waltham, Massachusetts, USA), the tissue was incubated with Alexa Fluor 488 goat anti-rabbit IgG (ab150077, Abcam, Cambridge, UK) for 1 h. Finally, the tissue was counterstained with DAPI (D9542, Sigma-Aldrich) and the images of cells were observed and documented with a BSF-40 fluorescence microscope (Batuo Instrument, Shanghai, China).
Isolation and culture of rat EPCs
EPCs used in this study were isolated from the four male SD rats (age: 3 weeks, weight: 80–100 g) referring to the previous publication [Citation13]. In brief, after the rats were anesthetized with 0.2% phenobarbital sodium at a dose of 150 mg/kg and sacrificed by cervical dislocation, the limb bones of the rats were collected. Ten ml of PBS was then used to rinse the bone marrow cavity repeatedly. After the bone marrow suspension was mixed with rat lymphocyte isolation buffer (M048494, Mreda, Beijing, China) and centrifuged (1,900 × g) for 20 min, the mononuclear cells were collected and cultured in a 25 cm2 culture flask with EGM-2 MV medium (CC-2505, Bitab Biotecch, Beijing, China) containing 10% FBS (16,140,071, Gibco) at 37°C in a humidified environment with 5% CO2. After culture for 72 h, the adherent cells were EPCs and cultured with fresh EGM-2 MV medium with 10% FBS. During culture, the medium was replaced by fresh medium every 2 days and the morphology of the cells were observed under an optical microscope (BX53; Olympus, Japan) at a magnification of ×200. After culture for 17 days, when the primary EPCs reached >95% confluence, they were harvested for identification and subsequent functional experiments.
Flow cytometry
Flow cytometry was used to analyze the proportion of EPCs in the peripheral blood of rats and identify the isolated EPCs. In brief, after being incubated with the CD34-PE antibody (ab223930, Abcam) for 1 h, the blood samples, and the isolated EPCs were further incubated with KDR-APC antibody (10,012-MM06-A, Sino Biological, Beijing, China) for 1 h separately. Finally, the percentages of EPCs in the mononuclear cells of rat peripheral blood and the EPCsCD34+/KDR+ were measured using the flow cytometer system (FACS-LSR II; Becton-Dickinson, NJ, USA). Data were finally analyzed using FCS Express software 3.0 (Dickinson Biosciences).
Cell transfection
MiR-17-5p mimic (5ʹ-CAAAGUGCUUACAGUGCAGGUAG-3ʹ), mimic control (MC; 5ʹ-UUCUCCGAACGUGUCACGUUU-3ʹ), miR-17-5p inhibitor (5ʹ-CUACCUGCACUGUAAGCACUUUG-3ʹ), and inhibitor control (IC; 5ʹ-CAGUCCUUUUGUGUAGUACAA-3ʹ) were purchased from RIBOBIO (Guangzhou, China). Plasmids constructed with pcDNA3.1 vector (VT1001) which contained PTEN overexpression sequence were synthesized by YouBio (Hunan, China); and plasmids constructed with pcDNA3.1 vector which contained no target sequence were used as a negative control (NC) for overexpression. Before transfection, 2 ml of EGM-2 MV medium containing 10% FBS and 1.0 × 106 EPCs were cultured in each well of a 6-well plate. After the cell confluence reached about 80%, the medium was refreshed. Meanwhile, 2 μg of miR-17-5p mimic, miR-17-5p inhibitor, overexpression plasmids, or their control was transfected into the cells using 2 μl of lipofectamine 3000 (BMB1385, Bomei Biotechnology, Hefei, China) and further cultured for 48 h.
Dual-luciferase reporter assay
The binding relationship between miR-17-5p and PTEN was predicted using Targetscan7.2 (http://www.targetscan.org/vert_72/). Then, the sequence of PTEN 3ʹUTR wild-type (PTEN-WT) containing miR-17-5p binding sites (5ʹ-GGATTAATAAAGATGGCACTTTC-3ʹ) and the PTEN 3ʹUTR mutant sequence (PTEN-MUT; 5ʹ-GGATTAATAAAGATGTAGCTAGC-3ʹ) were separately cloned into the pGL3-basic vectors (VT1554, YouBio). After the EPCs were co-transfected with the vectors and miR-17-5p mimic or MC for 48 h, the transfected EPCs were processed with a dual-luciferase reporter experiment kit (ab228530, Abcam, CA, USA). Finally, the absorbance of the 48-well plates was detected with a SpectraMax reader (Molecular Devices, Shanghai, China) for determining luciferase activity.
RNA extraction and quantitative real-time PCR (qPCR)
Total mRNA and miRNA of the cultured EPCs were extracted for determining the expressions of PTEN and miR-17-5p. For total mRNA extraction, after being lysed with TRIzol (15,596, Invitrogen, Waltham, Massachusetts, USA), the EPCs were mixed with chloroform (A53235, OKA) and centrifuged (14,000 × g) for 30 min. The supernatant was collected and then incubated with an equal volume of isopropanol (E15794, OKA) for 15 min. After centrifuging (14,000 × g) the supernatant for 5 min, the total mRNA sediments were obtained. MiRNA was directly isolated from the EPCs using a miRNA isolation regent (DP501, TianGEN, Beijing, China) according to the instructions.
Then, the mRNA and miRNA were reverse-transcribed into cDNA by using an Omniscript RT Kit (205,111, Qiagen, Dusseldorf, Germany). The cDNA was then mixed with qPCR Qupermix (AQ601-01, TransGen, Beijing, China) and relative primers to perform the amplification reaction on the QuantStudio6 system (Applied Biosystems, CA, USA). The RNA expression was finally quantified using the 2−ΔΔCT method on the QuantStudio6 system. The primers used for amplification were as follows: PTEN-F: 5ʹ-CAGCCAAGTCTGTGACTFGCCGTAC-3ʹ, PTEN-R: 5ʹ-CGCTCGAGCAGTCGCTGCAACCATCCA-3ʹ; miR-17-5p-F: 5ʹ-GTGCTTACAGTGCAGGTAGGT-3ʹ, miR-17-5p-R: 5ʹ-TGTCGTGGAGTCGGCAATTG-3ʹ; GAPDH-F: 5ʹ-CAAGCTCATTTCCTGGTATGAC-3ʹ, GAPDH-R: 5ʹ-CAGTGAGGGTCTCTCTCTTCCT-3ʹ; U6-F: 5ʹ-CTCGCTTCGGCAGCACATATACTA-3ʹ, U6-R: 5ʹ-ACGAATTTGCGTGTCATCCTTGCG-3ʹ. GAPDH and U6 were used as internal controls for PTEN and miR-17-5p, respectively.
MTT assay
After transfection, 1.0 × 104 EPCs were added into each well of a 96-well plate in 100 μl of EGM-2 MV medium with 10% FBS. After cell growth for 24 h, the medium was replaced by 100 μl of 0.5 mg/ml MTT solution (475,989, Sigma-Aldrich) for further incubation for 4 h. Then the MTT solution was replaced by 100 μl of dimethyl sulfoxide (116,743, Sigma-Aldrich) and the 96-well plate was shaken for 1 min. Finally, the 96-well plate was laid into an Imark microplate reader (Bio-Rad, Hercules, California, USA) to measure the absorbance at 570 nm.
Wound healing assay
After transfection, 3.0 × 105 EPCs were added into each well of a 6-well plate in 2 ml of EGM-2 MV medium with containing 10% FBS. After the cell confluence reached about 95%, wounds of the same width were created by scratching in each well and the medium was then replaced by EGM-2 MV medium without FBS. The wound in each well was photographed at 0 and 24 h using an optical microscope at a magnification of ×100. The data were analyzed using Image J 1.8.0 software.
Tube formation assay
ECMatix gel (ECM625, Millipore, Billica, Massachusetts, USA) was pre-coated into a 48-well plate and 300 μl of medium was added into each well of the 48 well plate for pre-incubation at 37°C for 1 h. Then, 2.0 × 105 EPCs were added into each well of the 48-well plate in 300 μl of EGM-2 MV medium with 10% FBS. After 4 h incubation, the tube-like structures formed in EPCs were photographed using an optical microscope at a magnification of ×100. The data were analyzed using Image J 1.8.0 software.
ELISA
The rat ELISA kit for VEGFA (GN-R31187, Gaining Biological, Shanghai, China) was used to determine the secretion level of VEGFA in the EPCs. In brief, the cultured supernatant (50 μl) of EPCs was added into the wells of the 96-well plate provided in the ELISA kit. Then, 50 µl of target antibody was added into the wells containing the supernatant and incubated for 1 h. After being washed twice, each well was added with 80 μl of HRP and incubated for 30 min at 37°C. Equal volumes of solution A and solution B were mixed together to a total volume of 100 μl and added into the wells for 20-min incubation. After the reaction in the wells was stopped by using 50 µl of stop buffer, the absorbance of each well was read by using the Imark microplate reader at 450 nm.
Western blot
Total protein was extracted from EPCs using the NP-40 (R21234, OKA) buffer. Then, the protein concentration was determined using a BCA Protein Assay Kit (BCA1, Sigma-Aldrich). After electrophoresis in 8% SDS-PAGE gel (P0688, Beyotime, Shanghai, China), the protein was transferred to a PVDF membrane (FFP32, Beyotime) and blocked with a blocking buffer (P0231, Beyotime). Then primary antibodies were applied to binding to the protein on the membrane overnight at 4°C. The primary antibodies were: PTEN (1:10,000, 54kDa, ab32199, Abcam), p-PI3K (1:1000, 84kDa, ab182651, Abcam), PI3K (1:1000, 84kDa, ab191606, Abcam), p-AKT (1:800, 56kDa, ab38449, Abcam), AKT (1:6000, 56kDa, ab179463, Abcam), VEGFA (1:2000, 23kDa, ab46154, Abcam), and β-actin (1:8000, 42kDa, ab8226, Abcam). After that, the mouse secondary antibody (1:10,000, ab205719, Abcam) or the rabbit secondary antibody (1:10,000, ab205718, Abcam) was further used to bind to the primary antibodies on the membrane. Lastly, the protein signal on the membrane was measured by using the Image Lab 3.0 Software (Bio-Rad).
Statistical analysis
One-way ANOVA was applied to analyze all the data in the current study. Tukey served as a post-hoc test. All the analyses in this study were performed in SPSS 20.0 software. Statistical data were finally presented as Mean ± SD. Statistically significance was defined as P< 0.05.
Results
MiR-17-5p increased the numbers of local EPCs and circulating EPCs and facilitated the vascular repair of aneurysm
To determine the effect of miR-17-5p in aneurysm, the aneurysm rat model was established and further treated with AgomiR-17-5p. After AgomiR-17-5p treatment for five times, the aneurysm tissues were collected and their histopathology was examined by hematoxylin-eosin staining. As depicted in , there were few endothelial cells and only sparse fibrocytes in the aneurysm tissues in the Model and Model+AgomiR-NC groups; while in the Model+AgomiR-17-5p group, a more complete aneurysm neck was formed with more spindle-shaped elongated cells in it, which indicated that miR-17-5p facilitated the vascular repair of aneurysm. Furthermore, the EPCs in the aneurysm tissues were identified using immunofluorescence. As exhibited in ), the expressions of the EPC markers (vWF and KDR) were significantly increased in the aneurysm tissues of the Model+AgomiR-17-5p group. Meanwhile, the circulating EPCs were also detected using flow cytometry ), and the results showed that the EPCs which were positive for CD34 and KDR were increased in the Model group (P< 0.001) as compared to the Sham group; besides, the EPCs which were positive for CD34 and KDR were further increased in the Model+AgomiR-17-5p group (P< 0.001) as compared to the Model+AgomiR-NC group, which proved that EPCs participate in the vascular repair of aneurysm, and miR-17-5p increased the number of EPCs in aneurysm tissues.
Figure 2. MiR-17-5p increased the numbers of local EPCs and circulating EPCs and facilitated the vascular repair of aneurysm. (a) The histopathology of aneurysm tissues was examined by hematoxylin-eosin staining (magnification ×200). (b) The vWF expression in the aneurysm tissues was determined using immunofluorescence (magnification ×200). (c) The KDR expression in the aneurysm tissues was determined using immunofluorescence (magnification ×200). (d-e) The percentage of EPCs in the mononuclear cells of rat peripheral blood was detected using flow cytometry (^^^P < 0.001, vs. Sham; ***P < 0.001, vs. Model+AgomiR-NC). (EPCs: endothelial progenitor cells, NC: negative control)
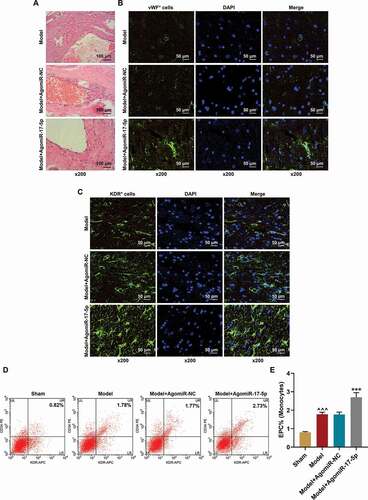
EPCs from healthy rats were successfully isolated and cultured
To determine the effect of miR-17-5p on EPCs in vitro, EPCs were isolated from healthy rats. The morphology of the isolated cells is depicted in . Also, the cells were further identified using flow cytometry after binding with KDR and CD34 antibodies. As the results in ) showed, the percentage of KDR+ cells was 47.40%±3.2%, the percentage of CD34+ cells was 40.54%±3.6%, and the percentage of double-positive cells was 38.08% ±1.8%, which indicated that the cells isolated and cultured were EPCs.
MiR-17-5p regulated the viability, migration, and tube formation of EPCs
After the miR-17-5p mimic and inhibitor were used to overexpress and silence miR-17-5p in EPCs respectively , the viability, migration, and tube formation of EPCs were further detected. As shown in , the viability of EPCs was enhanced by miR-17-5p mimic (P< 0.001), yet inhibited by miR-17-5p inhibitor (P< 0.001) when compared with their negative controls, respectively. Besides, the migration , e) and tube formation , f) of EPCs were also enhanced by miR-17-5p mimic (P< 0.001), but inhibited by miR-17-5p inhibitor (P< 0.05) when compared with the MC and IC groups, respectively. All the evidence indicated that miR-17-5p regulated the viability, migration, and tube formation of EPCs.
Figure 4. MiR-17-5p regulated the viability, migration, and tube formation of EPCs. (a) The transfection efficiency of miR-17-5p was evaluated using qPCR, with U6 as an internal control. (b) The viability of EPCs transfected with miR-17-5p mimic and inhibitor was detected by MTT assays. (c-e) The migration ability of EPCs transfected with miR-17-5p mimic and inhibitor was detected by wound healing assays (magnification ×100). (d-f) The tube formation ability of EPCs transfected with miR-17-5p mimic and inhibitor was detected by tube formation assays (magnification ×100). (***P < 0.001, vs. MC; ^P < 0.05, ^^^P < 0.001, vs. IC) (EPCs: endothelial progenitor cells, M: miR-17-5p mimic, I: miR-17-5p inhibitor, MC: mimic control, IC; inhibitor control)
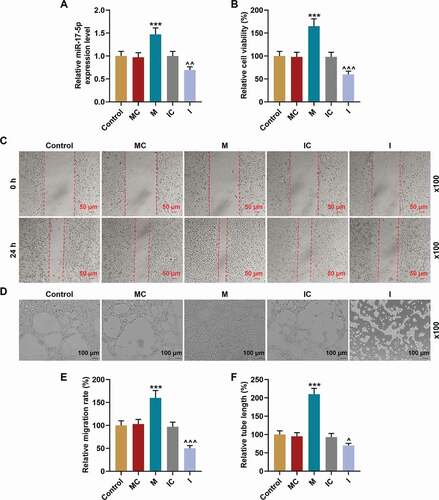
MiR-17-5p regulated the expression of VEGFA and the activation of PTEN-mediated PI3K/AKT pathway
Then, the VEGFA secretion in EPCs was examined , and the results revealed that the secretion of VEGFA was promoted by miR-17-5p mimic (P< 0.001) and inhibited by miR-17-5p inhibitor (P< 0.001) when compared with their negative controls, respectively. In addition, the expressions of VEGFA and proteins related to PTEN-mediated PI3K/AKT pathway were further evaluated through Western blot. As demonstrated in ), miR-17-5p mimic inhibited the expression of PTEN (P< 0.001) and promoted the expressions of p-PI3K (P< 0.001), p-AKT (P< 0.001), and VEGFA (P< 0.001) when compared with the MC group; while miR-17-5p inhibitor up-regulated the expression of PTEN (P< 0.001) and down-regulated the expressions of p-PI3K (P< 0.001), p-AKT (P< 0.001), and VEGFA (P< 0.001) when compared with the IC group. In addition, the ratios of p-PI3K to PI3K and p-AKT to AKT were also increased by miR-17-5p mimic (P< 0.001) and decreased by miR-17-5p inhibitor (P< 0.001), which indicated that miR-17-5p regulated the activation of PTEN-mediated PI3K/AKT pathway.
Figure 5. MiR-17-5p regulated the expression of VEGFA and the activation of PTEN-mediated PI3K/AKT pathway. (a) The secretion of VEGFA in EPCs transfected with miR-17-5p mimic and inhibitor was detected by ELISA. (b-c) The expressions of PTEN, p-PI3K, PI3K, p-AKT, AKT, and VEGFA in EPCs transfected with miR-17-5p mimic and inhibitor were detected by Western blot. β-actin was used as an internal control. (d-e) The ratios of p-PI3K to PI3K (d) and p-AKT to AKT (e) were calculated based on the data of Western blot. (***P < 0.001, vs. MC; ^^^P < 0.001, vs. IC) (EPCs: endothelial progenitor cells, M: miR-17-5p mimic, I: miR-17-5p inhibitor, MC: mimic control, IC; inhibitor control)
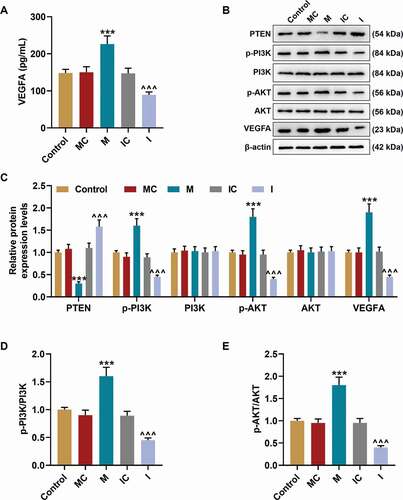
MiR-17-5p specifically targeted PTEN
After TargetScan predicted that PTEN was targeted by miR-17-5p , we performed dual-luciferase reporter assay to verify this prediction. As presented in , the luciferase activity in cells was decreased by co-transfection of PTEN-WT and miR-17-5p mimic (P< 0.001), while no difference was discovered in the cells co-transfected with PTEN-MUT and miR-17-5p mimic, which suggested that PTEN was targeted by miR-17-5p.
Figure 6. PTEN was targeted by miR-17-5p and overturned the effects of miR-17-5p mimic on the expression of PTEN and the viability of ECPs. (a) The binding sites between PTEN and miR-17-5p were predicted by Targetscan7.2. (b) The targeted relationship between PTEN and miR-17-5p was verified by dual-luciferase reporter assay. (c-d) The expression of PTEN in EPCs after overexpressing miR-17-5p and PTEN was detected by Western blot. β-actin was used as an internal control. (e) The expression of PTEN in EPCs after overexpressing miR-17-5p and PTEN was detected by qPCR. β-actin was used as an internal control. (f) The viability of EPCs after overexpressing miR-17-5p and PTEN was detected by MTT assay. (+++P < 0.001, vs. MC; ***P < 0.001, vs. NC; ^^^P < 0.001, vs. Control; ###P < 0.001, vs. PTEN; &&&P < 0.001, vs. M+ NC) (EPCs: endothelial progenitor cells, M: miR-17-5p mimic, MC: mimic control, NC; negative control)
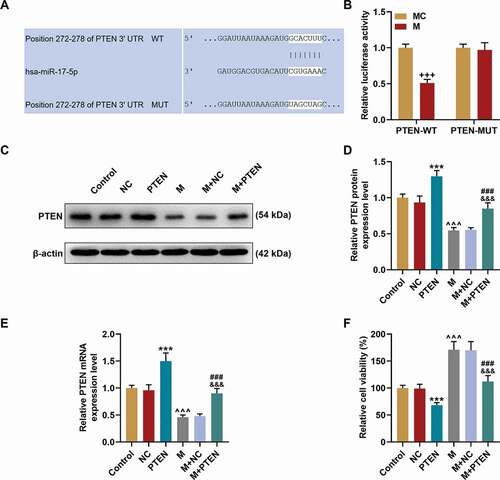
PTEN overexpression overturned the effects of miR-17-5p mimic on the expression of PTEN, cell viability, migration, and tube formation of EPCs
Given that PTEN was targeted by miR-17-5p, the expression of PTEN was further detected at both the transcription ) and translation ) levels. The expression of PTEN was up-regulated by PTEN overexpression (P< 0.001) but down-regulated by miR-17-5p mimic (P< 0.001); and after co-overexpression of PTEN and miR-17-5p, the effects of miR-17-5p mimic and PTEN overexpression on PTEN expression were overturned by each other. Also, the cel viability was decreased by PTEN overexpression (P< 0.001) and increased by miR-17-5p mimic (P< 0.001), and after co-overexpressing PTEN and miR-17-5p, the effects of miR-17-5p mimic and PTEN overexpression on cell viability were counteracted by each other. Similarly, the cell migration ability ) and tube formation ability ) were inhibited by PTEN overexpression (P< 0.001) and enhanced by miR-17-5p mimic (P< 0.001), and after co-overexpressing PTEN and miR-17-5p, the effects of miR-17-5p mimic and PTEN overexpression on cell migration and tube formation were overturned by each other. All these phenomena suggested that miR-17-5p regulated the viability, migration, and tube formation of EPCs by regulating PTEN.
Figure 7. PTEN overexpression overturned the effects of miR-17-5p mimic on the migration and tube formation of EPCs. (a, c) The migration ability of EPCs after overexpressing miR-17-5p and PTEN was detected by wound healing assay (magnification ×100). (B. D) The tube formation ability of EPCs after overexpressing miR-17-5p and PTEN was detected by tube formation assay (magnification ×100). (***P < 0.001, vs. NC; ^^^P < 0.001, vs. Control; ###P < 0.001, vs. PTEN; &&&P < 0.001, vs. M+ NC) (EPCs: endothelial progenitor cells, M: miR-17-5p mimic, NC; negative control)
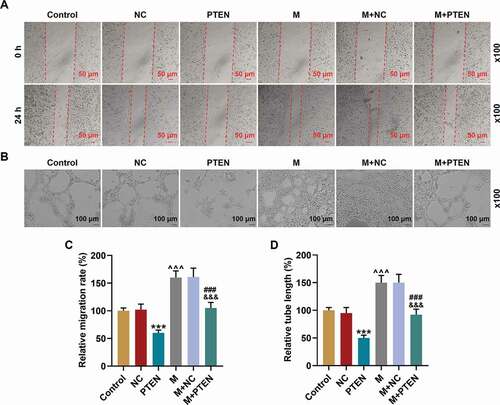
PTEN overexpression overturned the effects of miR-17-5p mimic on the expression of VEGFA and the activation of PTEN-mediated PI3K/AKT pathway
The secretion of VEGF was decreased by PTEN overexpression (P< 0.001) and increased by miR-17-5p mimic (P< 0.001); and after co-overexpression of PTEN and miR-17-5p, the effects of PTEN overexpression and miR-17-5p mimic were reversed by each other. Similarly, the protein expressions of p-PI3K, p-AKT, and VEGFA ), and the ratios of p-PI3K to PI3K and p-AKT to AKT were down-regulated by PTEN overexpression (P< 0.001) and up-regulated by miR-17-5p mimic (P< 0.001); and after co-overexpressing PTEN and miR-17-5p, the effects of PTEN overexpression and miR-17-5p mimic were reversed by each other. These results indicated that miR-17-5p regulated the expression of VEGFA and the activation of PTEN-mediated PI3K/AKT pathway. The working model of this study was shown in .
Figure 8. PTEN overexpression overturned the effects of miR-17-5p mimic on the expression of VEGFA and the activation of PTEN-mediated PI3K/AKT pathway. (a) The secretion of VEGFA in EPCs after overexpressing miR-17-5p and PTEN was detected by ELISA. (b-c) The expressions of p-PI3K, PI3K, p-AKT, AKT, and VEGFA in EPCs after overexpressing miR-17-5p and PTEN were detected by Western blot. β-actin was used as an internal control. (d-e) The ratios of p-PI3K to PI3K (d) and p-AKT to AKT (e) were calculated based on the data of Western blot. (***P < 0.001, vs. NC; ^^^P < 0.001, vs. Control; ###P < 0.001, vs. PTEN; &&&P < 0.001, vs. M+ NC) (EPCs: endothelial progenitor cells, M: miR-17-5p mimic, NC; negative control)
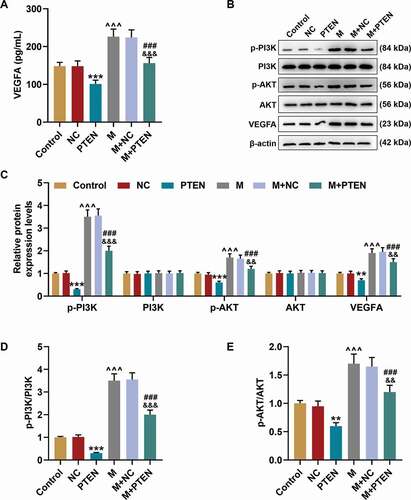
Discussion
Although miR-17-5p was previously discovered to be abnormally expressed in vascular diseases such as thoracic aortic dissection and aneurysm [Citation14–16], the specific role of miR-17-5p in aneurysm is still unclear. Therefore, in this study, we established a rat aneurysm model and treated the rats with overexpressed miR-17-5p. After treatment for almost a month, we discovered that miR-17-5p overexpression promoted the vascular repair of aneurysm. Previous research has proved that in various types of vascular diseases, EPCs in bone marrow can enter the peripheral circulation, home to the injured area, and differentiate into mature vascular endothelial cells to promote the endothelial repair of injured vessels [Citation4,Citation6]. To verify whether the effect of miR-17-5p on the vascular repair of aneurysm was associated with the function of EPCs, we further evaluated the level of EPCs by detecting the expressions of the EPCs markers including KDR, vWF, and CD34 [Citation17–19]. Interestingly, miR-17-5p overexpression up-regulated the level of EPCs in both aneurysm tissues and peripheral circulation, which indicated that miR-17-5p up-regulated the levels of local EPCs and circulating EPCs to promote the vascular repair of aneurysm. Besides, we further conjectured that the specific effect mechanism of miR-17-5p on the vascular repair of aneurysm was realized by facilitating the endothelialization of EPCs.
To further verify our conjecture, the primary EPCs were isolated and cultured. The functions of ECPs including endothelialization are dependant on its biological activities such as proliferation, migration, and angiogenesis [Citation13]. In this study, we for the first time discovered that the viability, migration, and tube formation ability of ECPs were promoted by miR-17-5p overexpression and inhibited by miR-17-5p silencing, which indicated that miR-17-5p increased the viability of ECPs, enhanced their migration to the aneurysm local tissue, and promoted their endothelialization and angiogenesis, thereby facilitating the vascular repair of aneurysm. VEGFA is recognized as a crucial mediator of blood vessel growth and can enhance angiogenesis by inducing the proliferation, migration, and capillary-like tube formation of EPCs [Citation13,Citation20]. In this study, the secretion of VEGFA in EPCs was up-regulated by miR-17-5p overexpression and down-regulated by miR-17-5p silencing, which further verified our above discoveries.
Accumulating evidence reveals that miRNA can target certain mRNA to modulate the down-stream signaling [Citation21–23]. Besides, previous studies have discovered that miR-17-5p can target PTEN in various diseases, such as gastric cancer, thyroid cancer, and hypoxia/reoxygenation injury [Citation21–23]. Consistently, our results also proved that PTEN was targeted by miR-17-5p. PTEN is one of the most commonly mutated tumor suppressors and has been proven to regulate the biological activities of different cancer cells [Citation24–26]. In this study, we also discovered that PTEN overexpression not only suppressed the viability, migration, and tube formation of EPCs and the secretion of VEGFA in EPCs, but also overturned the effect of miR-17-5p overexpression on EPCs, which indicated that miR-17-5p targeted PTEN to regulate the biological functions of ECPs.
Previous research demonstrated that PTEN is a negative regulator of the PI3K/AKT pathway which can further mediate VEGFA [Citation20,Citation27,Citation28]. In addition, the activation of PI3K/AKT signaling contributes to the suppression of the development of abdominal aortic aneurysm [Citation11,Citation29,Citation30]. To further verify whether the effect of miR-17-5p on EPCs and the vascular repair of aneurysm was realized by activating PTEN-mediated PI3K/AKT signaling pathway, we further evaluated the activation of the PI3K/AKT signaling pathway in the EPCs that overexpressed miR-17-5p and PTEN. To our delight, the activation of PI3K/AKT signaling pathway was enhanced by miR-17-5p overexpression and suppressed by PTEN overexpression, and overexpressed PTEN could further abrogate the effect of miR-17-5p.
The limitation of this research was not using Transwell assay to confirm the result of wound healing assay for migration detection.
All in all, the present study revealed that miR-17-5p promoted the endothelialization of EPCs to facilitate the vascular repair of aneurysm by regulating PTEN-mediated PI3K/AKT/VEGFA pathway. Our research provided a novel biological therapy for the prevention and treatment of aortic aneurysm.
Acknowledgments
Not applicable
Disclosure statement
The authors declare no conflicts of interest.
Additional information
Funding
References
- Davis FM, Daugherty A, Lu HS. Updates of recent aortic aneurysm research. Arterioscler Thromb Vasc Biol. 2019 Mar;39(3):e83–e90. PubMed PMID: 30811252; PubMed Central PMCID: PMCPMC6394847.
- Mathur A, Mohan V, Ameta D, et al. Aortic aneurysm. J Transl Int Med. 2016 Apr 1;4(1):35–41. PubMed PMID: 28191516; PubMed Central PMCID: PMCPMC5290913.
- Das UN. Is aortic aneurysm preventable? J Transl Int Med. 2017 Jun;5(2):72–78. PubMed PMID: 28721338; PubMed Central PMCID: PMCPMC5506405. eng.
- Balsam LB. Endothelial progenitor cells to the rescue? J Thorac Cardiovasc Surg. 2019 Feb;157(2):667–668. PubMed PMID: 30269973.
- Bayraktutan U. Endothelial progenitor cells: potential novel therapeutics for ischaemic stroke. Pharmacol Res. 2019 Jun;144:181–191. PubMed PMID: 31004788; eng.
- Peters EB. Endothelial progenitor cells for the vascularization of engineered tissues. Tissue Eng Part B Rev. 2018 Feb;24(1):1–24. PubMed PMID: 28548628; PubMed Central PMCID: PMCPMC5797330. eng.
- Garikipati VNS, Kishore R. Endothelial progenitor cells: procedure for cell isolation and applications. Methods Mol Biol. 2017;1553:85–89. PubMed PMID: 28229409.
- Yu G, Liu P, Shi Y, et al. Sitagliptin stimulates endothelial progenitor cells to induce endothelialization in aneurysm necks through the SDF-1/CXCR4/NRF2 signaling pathway. Front Endocrinol (Lausanne). 2019;10:823. PubMed PMID: 32038475; PubMed Central PMCID: PMCPMC6988800. eng.
- Boileau A, Lino Cardenas CL, Courtois A, et al. MiR-574-5p: a circulating marker of thoracic aortic aneurysm. Int J Mol Sci. 2019 Aug 12;20(16):3924. PubMed PMID: 31409059; PubMed Central PMCID: PMCPMC6720007. eng.
- Gan S, Pan Y, Mao J. miR-30a-GNG2 and miR-15b-ACSS2 interaction pairs may be potentially crucial for development of abdominal aortic aneurysm by influencing inflammation. DNA Cell Biol. 2019 Dec;38(12):1540–1556. PubMed PMID: 31730405; eng.
- Ma X, Yao H, Yang Y, et al. miR-195 suppresses abdominal aortic aneurysm through the TNF-α/NF-κB and VEGF/PI3K/Akt pathway. Int J Mol Med. 2018 Apr;41(4):2350–2358. PubMed PMID: 29393364; eng.
- Maegdefessel L, Spin JM, Raaz U, et al. miR-24 limits aortic vascular inflammation and murine abdominal aneurysm development. Nat Commun. 2014 Oct;31(5):5214. PubMed PMID: 25358394; PubMed Central PMCID: PMCPMC4217126. eng.
- Yu G, Liu P, Shi Y, et al. Stimulation of endothelial progenitor cells by microRNA-31a-5p to induce endothelialization in an aneurysm neck after coil embolization by modulating the Axin1-mediated beta-catenin/vascular endothelial growth factor pathway. J Neurosurg. 2019 Aug 9:1–9. PubMed PMID: 31398705. DOI:10.3171/2019.5.JNS182901
- Sun J, Chen G, Jing Y, et al. LncRNA expression profile of human thoracic aortic dissection by high-throughput sequencing. Cell Physiol Biochem. 2018;46(3):1027–1041. PubMed PMID: 29669321.
- Liu D, Han L, Wu X, et al. Genome-wide microRNA changes in human intracranial aneurysms. BMC Neurol. 2014 Oct;10(14):188. PubMed PMID: 25300531; PubMed Central PMCID: PMCPMC4210474. eng.
- Yang P, Cai L, Zhang G, et al. The role of the miR-17-92 cluster in neurogenesis and angiogenesis in the central nervous system of adults. J Neurosci Res. 2017 Aug;95(8):1574–1581. PubMed PMID: 27869313.
- Liu P, An Q, Chen X, et al. Rosuvastatin for enhancement of aneurysm neck endothelialization after coil embolization: promotion of endothelial progenitor cells in a rodent model. J Neurosurg. 2016 May;124(5):1265–1274. PubMed PMID: 26406802.
- Zan T, Li Q, Dong J, et al. Transplanted endothelial progenitor cells increase neo-vascularisation of rat pre-fabricated flaps. J Plast Reconst Aesthetic Surg. 2010 Mar;63(3):474–481. PubMed PMID: 19117823; eng.
- Zhang HR, Chen FL, Xu CP, et al. Incorporation of endothelial progenitor cells into the neovasculature of malignant glioma xenograft. J Neurooncol. 2009 Jun;93(2):165–174. PubMed PMID: 19052696; eng.
- Lu JM, Zhang ZZ, Ma X, et al. Repression of microRNA-21 inhibits retinal vascular endothelial cell growth and angiogenesis via PTEN dependent-PI3K/Akt/VEGF signaling pathway in diabetic retinopathy. Exp Eye Res. 2020 Jan;190:107886. PubMed PMID: 31759996.
- Guan YJ, Ma JY, Song W. Identification of circRNA-miRNA-mRNA regulatory network in gastric cancer by analysis of microarray data. Cancer Cell Int. 2019;19:183. PubMed PMID: 31346318; PubMed Central PMCID: PMCPMC6636116. eng.
- Guo L, Zhang Q, Ma X, et al. miRNA and mRNA expression analysis reveals potential sex-biased miRNA expression. Sci Rep. 2017 Jan;3(7):39812. PubMed PMID: 28045090; PubMed Central PMCID: PMCPMC5206641. eng.
- Huang Y. The novel regulatory role of lncRNA-miRNA-mRNA axis in cardiovascular diseases. J Cell Mol Med. 2018 Dec;22(12):5768–5775. PubMed PMID: 30188595; PubMed Central PMCID: PMCPMC6237607. eng.
- Boosani CS, Gunasekar P, Agrawal DK. An update on PTEN modulators - a patent review. Expert Opin Ther Pat. 2019 Nov;29(11):881–889. PubMed PMID: 31530116; PubMed Central PMCID: PMCPMC6817417. eng.
- Luongo F, Colonna F, Calapà F, et al. PTEN Tumor-Suppressor: the Dam of Stemness in Cancer. Cancers (Basel). 2019 Jul 30;11(8). PubMed PMID: 31366089; PubMed Central PMCID: PMCPMC6721423. eng. DOI:10.3390/cancers11081076.
- Malaney P, Uversky VN, Davé V. PTEN proteoforms in biology and disease. Cell Mol Life Sci. 2017 Aug;74(15):2783–2794. PubMed PMID: 28289760; eng.
- Wang Y, Lu YH, Tang C, et al. Calcium dobesilate restores autophagy by inhibiting the VEGF/PI3K/AKT/mTOR signaling pathway. Front Pharmacol. 2019;10:886. PubMed PMID: 31447680; PubMed Central PMCID: PMCPMC6696883. eng.
- Zhang Y, Cheng H, Li W, et al. Highly-expressed P2X7 receptor promotes growth and metastasis of human HOS/MNNG osteosarcoma cells via PI3K/Akt/GSK3β/β-catenin and mTOR/HIF1α/VEGF signaling. Int J Cancer. 2019 Aug 15;145(4):1068–1082. PubMed PMID: 30761524; PubMed Central PMCID: PMCPMC6618011. eng.
- Liu Q, Shan P, Li H. Gambogic acid prevents angiotensin II-induced abdominal aortic aneurysm through inflammatory and oxidative stress dependent targeting the PI3K/Akt/mTOR and NF-κB signaling pathways. Mol Med Rep. 2019 Feb;19(2):1396–1402. PubMed PMID: 30535428; eng.
- Zhao L, Huang J, Zhu Y, et al. miR-33-5p knockdown attenuates abdominal aortic aneurysm progression via promoting target adenosine triphosphate-binding cassette transporter A1 expression and activating the PI3K/Akt signaling pathway. Perfusion. 2020 Jan;35(1):57–65. PubMed PMID: 31170866; eng.