ABSTRACT
Intervertebral disc degeneration (IDD) is characterized by excessive inflammatory reaction, and neuropeptide Y (NPY) was reported to have anti-inflammatory effect. However, the effect of NPY on NP cells has not been investigated up to date. This study aimed to clarify the role of NPY on the process of IDD. Fourteen fresh human lumbar intervertebral discs were harvested, and degeneration-related proteins were examined. Pfirrmann grading system was used to evaluate IDD. Rat nucleus pulposus (NP) cells were used to investigate the effect of NPY on the proliferation, apoptosis, and extracellular matrix (ECM) in NP cell induced by IL-1βin vitro. The expression levels of NPY and its receptors (type 1 receptor, Y1R, and type 2 receptor, Y2R) were detected via immunohistochemical analysis, western blot, and quantitative real-time polymerase chain reaction (qRT-PCR). Cell viability and proliferation were explored using cell counting kit-8 assay, western blot, and immunofluorescence analysis. Cell apoptosis was investigated by Hoechst staining, JC-1 Staining, annexin V-FITC/PI double staining, and western blot. The secretion of NPY from NP cells was determined via enzyme-linked immunosorbent assay (ELISA). The expression of anabolic and catabolic gene was analyzed by qRT-PCR, western blot, immunofluorescence analysis, and ELISA. The expression of Y2R was significantly increased in both human degenerative intervertebral discs and IL-1β-induced NP cells. Although no positive results for NPY indicated by western blot both in vivo and in vitro, ELISA results demonstrated that the secretion of NPY from NP cells was increased by low-concentration IL-1β, but was decreased when the concentration of IL-1β was 30 ng/ml and above. In addition, NPY could promote NP cells proliferation and protect NP cells against IL‑1β‑induced apoptosis via suppressing mitochondrial-mediated apoptosis pathway. What’s more, NPY can suppress the expression of catabolic gene and ameliorate IL-1β- induced matrix degeneration in NP cells. In conclusion, NPY could promote NP cell proliferation and alleviate IL‑1β‑induced cell apoptosis via mitochondrial pathway. In addition, NPY can suppress the expression of ECM‑catabolic proteinases and ameliorate IL-1β- induced ECM degeneration in vitro.
Introduction
Low back pain (LBP) has ranked as one of the major public concerns for musculoskeletal disorders, with approximately 80% of the populations experiencing LBP in their lifetime [Citation1]. Despite the unknown pathogenesis, intervertebral disc degeneration (IDD) has been considered as one of the primary contributors to LBP [Citation2]. Although patients with early IDD can be treated conservatively, surgical intervention is frequently the preferred option. However, given poor understanding about the pathogenesis of IDD, current therapies for IDD are still not satisfactory [Citation3]. In addition, current treatment perspectives gradually focus on the symptom relief rather than only decelerating or retarding the degenerative process itself. Consequently, it is imperative to precisely elucidate the pathogenic mechanism of IDD, which would provide a novel insight into future treatment for IDD.
Automatically, the intervertebral disc (IVD) is composed of three main structures: the inner nucleus pulposus (NP), circumferential fibrocartilaginous annulus fibrosus (AF), and cartilaginous endplates (EP) that cap the NP and AF [Citation4]. Under healthy condition, NP tissue is rich in NP cells and extracellular matrix (ECM) (collagen type II and aggrecan) [Citation5,Citation6]. However, as the disc degenerates with age or disease, the abnormally activated inflammatory reactions, increased cell apoptosis, elevated production of ECM‑catabolic proteinases mainly including matrix metalloproteinases (MMPs) and a disintegrin and metalloproteinase with thrombospondin motifs (ADAMTS), have been frequently observed [Citation7]. These pathogenic changes would compromise the integrity of intervertebral disc and lead to abnormal distribution of mechanical load imposed on spine and consequently destroy the mechanical properties of the spine.
At present, increasing studies have suggested that excessive production of proinflammatory factors has been the key contributor to IDD [Citation8,Citation9]. Interleukin‐1β (IL‐1β) has been the most commonly studied inflammatory cytokine regarding IDD, which widely involves in inflammatory responses, oxidative stress, cell apoptosis, cell senescence, and abnormal degradation of ECM [Citation9]. As a result, inhibiting the damaging effects of IL‐1β on NP cells has been proposed to be the therapeutic target for IDD.
Neuropeptide Y (NPY) is a 36-amino acid neurotransmitter that belongs to the family of neuroendocrine peptides [Citation10]. NPY is primarily distributed in the central and peripheral nervous systems in mammals, and has been widely involved in various physiological and homeostatic processes, such as brain activity, stress coping, blood pressure, heart rate, and body metabolism [Citation11]. In the central nervous system (CNS), NPY is expressed in regions such as the hypothalamus [Citation10]. In peripheral nervous systems, NPY has been reported to mainly co-localize with noradrenaline in sympathetic terminals [Citation12]. Previous studies frequently focus on the regulation of energy homeostasis by the NPY system [Citation13]. However, the regulative role of NPY in immune function has gradually become the hotspot in research [Citation14].
In fact, in addition to generated by nerve system, NPY could be also produced by immune cells, such as monocytes and macrophages [Citation15]. A growing body of literatures, both in humans and rodent models, have revealed that NPY levels are altered in some neurodegenerative and neuroimmune diseases [Citation11,Citation14–17]. Mitic et al. ever reported that NPY could inhibit the LPS-induced accumulation of inflammatory cells in the peripheral blood of lipopolysaccharide- (LPS-) induced inflammation rat model [Citation16]. Similarly, De la Fuente et al. found that NPY could decrease the phagocytosis capacity of macrophages, and the release of tumor necrosis factor α (TNF-α), IL-1β and IL-217. In addition, NPY has been reported to involve in joint neuropathic pain and back pain [Citation18,Citation19]. Recently, several studies have revealed that NPY locates in the sympathetic nerve fibers in rat lumbar annulus fibrosus (AF) and human degenerated annulus fibrosus (AF), making NPY of particular interest in IDD [Citation20,Citation21]. In addition, a recent study in 2020 has revealed that NPY could enhance proliferation and chondrogenic differentiation [Citation22]. In fact, several previous studies found associations between serum NPY and LBP among older adults [Citation23,Citation24]. Furthermore, NPY has been reported to significantly correlate with radiographic disc space narrowing in a recent study by Adam et al [Citation25]. However, the further mechanism of NPY involving in IDD remains elusive and the effect of NPY on NP cells has not been investigated up to date. In fact, the mature NP cells have morphological similarities with chondrocytes, even being termed “chondrocyte-like cells”. Therefore, in this study we hypothesized that NPY may have similar protective effect on the NP cells during the process of IDD.
Therefore, this study aimed to investigate the effects of NPY on the proliferation and apoptosis of NP cells, and also to examine the expression changes of ECM-catabolic proteinases in NP cells under NPY treatment.
Methods and materials
Patient tissue samples
NP tissues from 14 patients who underwent lumbar discectomy surgery in our institution from June 2018 to May 2019 were collected (four females and ten males, mean age, 45.00 ± 9.07 years; age range, 35‑61 years). All patients accepted preoperative MRI of lumbar spine, and the Pfirrmann grading system was used to evaluate the condition of affected disc. The disc with grade I/II was defined as normal, whereas disc with grade III/above was defined as degenerated [Citation26]. According to the evaluation of intervertebral disc, patients were divided into non-degeneration group and degeneration group. The non–degeneration group consisted of four patients with idiopathic scoliosis and lumbar trauma who required lumbar surgery, and all 10 patients in degeneration group were treated due to IDD at lumbar spine.
This study was approved by the institution review board (IRB) of our hospital, and written informed consent was obtained from all participants involved in the study.
Rat NP cells isolation, culture and identification
Animal experiments in this study were performed according to a protocol approved by the Animal Experimentation Committee of our institution.
The procedure has been reported previously [Citation27]. Twenty Sprague–Dawley male rats weighed 100–150 g were euthanized with an overdose of sevoflurane. The tails were removed carefully and immersed in 75% disinfection alcohol for 5-10 min. The skin around the tail was removed under aseptic conditions. We separated gel-like NP tissues from coccyx discs and washed the tissues twice with aseptic PBS. NP cells were released and isolated with 0.25% Trypsin-EDTA (Gibco, Invitrogen) for 30 min and 0.1% collagenase type II (Invitrogen, Carlsbad, CA, USA) for 4 h at 37°C in DMEM/F12 (Gibco; Thermo Fisher Scientific, Inc., Waltham, MA, USA) combined with 0.1% of fetal bovine serum (Gibco; Thermo Fisher Scientific, Inc.) and 1% of a penicillin‑streptomycin solution (Gibco; Thermo Fisher Scientific, Inc.). After isolation, NP cells were resuspended in DMEM/F12 containing 20% of FBS and 1% penicillin‑streptomycin solution and cultured at 37°C in a humidified atmosphere of 95% air and 5% CO2. The medium was changed every 2 days. Frequently, NP cells would move out of the explants after 1 week, which we called passage 0. When confluent, the cells were harvested by using 0.25% Trypsin-EDTA (Gibco, Invitrogen) for 5 min at 37°C. Next, cells were counted and replanted into 25 cm3 culture flasks at the appropriate density. Due to no significant changes in morphology of NP cells between primary cells (passage 1, P1) and later passage cells (P4), the low-passage (<4) cells cultured in monolayers were used for the following experiments, and the rat NP cell phenotype was confirmed by identifying the expression of type II collagen and aggrecan.
Establishment of rat NP cells injury model in vitro
Rat NP cells were plated at 4 × 105 cells/well in 2 ml of culture medium in 6 well plates. After 24 h, cells were cultured with IL-1β (30 ng/ml; PeproTech, Rocky Hill, NJ, USA) for the indicated times. The cell viability (24 h) and catabolic gene (0 h, 6 h, 12 h, 24 h, 48 h) were analyzed.
Cell viability analysis
Cell Counting Kit‑8 (CCK‑8, Beyotime Biotechnology, Inc., Shanghai, China) was used to determine the effect of NPY on the cell viability of NP cells. Briefly, the rat NP cells were seeded in a 96‑well plate with a density of 4 × 103 cells/well and cultured as described above. After cell adhesion of 24 hours, cells were intervened by various concentrations of NPY (10−8–10−12 mol/L) were for 24 h, 48 h, 72 h at 37°C, respectively. Subsequently, 10 μl of the CCK-8 solution combined with 90 ul of complete medium was added into each well, followed by incubation at 37°C for 3 h. The absorbance was then measured at a wavelength of 450 nm on an ELX800 absorbance microplate reader (Bio-Tek, USA).
Quantitative Real-Time Polymerase Chain Reaction (qRT-PCR)
The expression of anabolic and catabolic gene, proliferation- and apoptosis-related gene, and Y2R gene was analyzed by qRT-PCR.
Firstly, total RNA was isolated from the rat NP cells with RNA extraction kit (Magen, Inc. Guangzhou, China) following the manufacturer’s instructions, and cDNA was then reversed from 1 ug of total RNA using reverse transcriptase (TaKaRa Biotechnology, Otsu, Japan). Subsequently, the mRNA expression levels were quantified by qRT-PCR with the SYBR Premix Ex Tag kit (TaKaRa, Biotechnology, Otsu, Japan) on a ABI 7500 Real‑Time PCR system (Applied Biosystems, Foster City, CA, USA). The reaction conditions were as follows: 40 cycles of denaturation at 95°C for 5 s and amplification at 60°C for 24 s. All reactions were run in triplicate, and the gene expression levels were normalized to that of GAPDH. The primer sequences used for qRT‑qPCR analysis are shown in .
Table 1. Sequence of Primers for RT-PCR
Immunohistochemical analysis
Immunohistochemical analysis was used to investigate the expression of Y1R and Y2R in human intervertebral disc.
After intervertebral disc sections embedded in paraffin were deparaffinized, rehydrated endogenous peroxidase was blocked by 3% hydrogen peroxide. Then, the sections were incubated with 0.4% pepsin (Sangon Biotech, Shanghai, China) in 5 mM HCl at 37°C for 30 min for antigen retrieval. The sections were incubated with 10% goat blocking serum for 30 min at room temperature, then with primary antibody against Y1R (Santa Cruz Biotechnology, Inc., Dallas, TX, USA, 1:100) and Y2R (Zenbio, Chengdu, China, 1:100) overnight at 4°C, and finally with HRP-conjugated secondary antibody. Rinsed sections were counterstained with hematoxylin. Bright-field images were acquired by using light microscopy (Olympus, Japan).
Hoechst staining
Cell apoptosis was quantified by Hoechst 33,342 staining (Beyotime Biotechnology, Inc., Shanghai, China) according the instructions. After being treated with NPY at various concentrations, the cells were washed with PBS for three times and then incubated with 1 mL of Hoechst 33,342 dye in the dark for 20 min. The morphologic changes in the apoptotic cell nuclei were detected by a fluorescence microscope (Olympus, BX53; Melville, NY, USA).
Analysis of mitochondrial membrane potential
Mitochondrial membrane potential could reveal the functional condition of mitochondrion. JC-1 Staining was performed via JC-1 staining kit (Beyotime Biotechnology, Inc., Shanghai, China) according to the instruction. Briefly, after treated with NPY and IL-1β, NP cells were washed by cool PBS for twice and were incubated with JC-1 staining fluid in 37°C for 2 h. Then, the NP cells were washed twice with JC-1 dyeing buffer solution (1X), followed by adding 2 ml of cell complete medium. Finally, a fluorescence microscope (Olympus, BX53; Melville, NY, USA) was used to analyzed the JC-1 staining of NP cells.
Analysis of apoptosis by flow cytometry
Cell apoptosis was also determined using an Annexin V-FITC Apoptosis Detection Kit (C1062M, Beyotime Biotechnology, Inc., Shanghai, China). Briefly, the NP cells in different groups (Control, IL-1β, IL-1βL+NPY) were trypsinized (Ca2 + free), washed three times with PBS and resuspended in 500 μL of binding buffer at a density of 5.0 × 105 cells/mL. Next, annexin V-FITC (5 μL) and PI solution (5 μL) were added to the cells for 5 min at room temperature in the dark, while two control groups were stained with either annexin V-FITC or PI as negative controls, followed by examination via flow cytometry.
Enzyme-linked immunosorbent assay
Enzyme-linked immunosorbent assay was used to sensitively examine the ECM catabolic proteinases and NPY secreted by NP cells.
To obtain the conditioned media for ECM catabolic proteinases array analysis, NPY with different concentrations was applied to NP cells for 24 without culture medium change. Then, the conditioned media was collected from each group and centrifuged (5000 rpm at 4°C for 15 min) to remove debris. The supernatants were stored at −80°C in separate until use and diluted with the appropriate standard diluents before measurement. Measurements for collagen-I/II, MMP-1/3/13, and ADAMTS5 were performed on the supernatants with Enzyme-Linked Immunosorbent Assay (ELISA) kits according to manufacturer’s instructions (Westang, Shanghai, China). The optical density at 450 OD was tested using an absorbance microplate reader (Bio-Tek, USA).
To obtain the conditioned media for NPY analysis, NP cells were treated by IL-1β with different concentrations (10, 30, 50 ng/mL) in serum-free medium for 24 h, and then the cell cultural supernatant was collected. The secretion level of NPY was determined using the similar method above (F16317, Westang, Shanghai, China).
Western blot
The Western blot procedure was performed to analyze the expression of proteins. Different treated NP cells were completely lysed in ice-cold RIPA, and protein concentration was measured using the BCA Protein Assay kit (Beyotime Institute of Technology, Shanghai, China). A total of 40 μg protein per lane was separated by sodium dodecyl sulfate polyacrylamide gel electrophoresis on a 12% gel and transferred onto a polyvinylidene fluoride membrane (EMD Millipore, Billerica, MA, USA). Subsequently, blocking was performed by 5% non‑fat milk in Tris-buffered saline-Tween for 2 hours at room temperature (Invitrogen, San Diego, CA, USA), and the membranes were incubated with primary antibodies against NPY (821,051, Zenbio, Chengdu, China, 1:100), Y1R (sc-393,192, Santa Cruz Biotechnology, Inc., Dallas, TX, USA, 1:100), Y2R(510,857, Zenbio, Chengdu, China, 1:100), Aggrecan ((ab3778; 50‐60 kDa; Abcam, Cambridge, UK; 1:100), type I collagen (399,525; 142 kDa; cell signaling tecnology, Inc., 3 Trask Lane Danvers, MA 01923; 1:1000); type II collagen (Collagen II (1:1000, ab34712, Abcam), MMP‑3 (380,816; 60kDa; Zenbio, Chengdu, China, 1:1,000), MMP‑13 (820,098; 54 kDa; Zenbio, Chengdu, China, 1:1000), PCAN (sc–56, Santa Cruz Biotechnology, Inc., Dallas, TX, USA, 1:100-1:1000), and GAPDH (5174, cell signaling technology, Inc., 3 Trask Lane Denver, MA 01923) at 4°C temperature for overnight. The membranes were then incubated with the indicated secondary antibodies (goat against rabbit or mouse, 1:5000) for 2 h at room temperature. The protein bands were detected using a Tanon Imaging System (version 5200, Tanon Science & Technology Co., Ltd., High-tech Park, Shanghai, China) and analyzed quantitatively using Image J software (National Institutes of Health, USA).
Immunofluorescence analysis
For aggrecan, type II collagen, MMP3, Ki-67 immunofluorescent staining, NP cells with different treatments were fixed with 4% PFA for 15 min at room temperature (RT) and were permeated for 5 min with 0.1% v/v Triton X-100. Cells were incubated with Aggrecan (GB11373, 1:500–1:1000, Servicebio, Wuhan, China), type II collagen (GB11021, 1:100–1:500, Servicebio, Wuhan, China), MMP3 (GB11131, 1:400-1:1600, Servicebio, Wuhan, China), Ki-67 (GB111141, 1:1200, Servicebio, Wuhan, China) diluted in 0.2% w/v bovine serum albumin (BSA)-PBS for 1 h at RT and then washed extensively with 0.2% w/v BSA-PBS and PBS. Cells were then incubated with DAPI (G1012-100ML, Servicebio, Wuhan, China) for visualization of nuclei, washed with PBS, and mounted with the buffer of FITC-conjugated goat anti-rabbit IgG and Cy3-conjugated goat anti-rabbit IgG at 37°C for 30 min in the dark, rinsed with PBS. Fluorescence detection was performed under a fluorescence microscope (Olympus, BX53; Melville, NY, USA).
Statistical analysis
All data are presented as the mean±standard deviation (SD) from at least three independent experiments. Differences between groups were assessed by the Student’s t test and ANOVA with appropriate post hoc analysis using GraphPad Prism (GraphPad Software Inc; La Jolla, CA). P value less than 0.05, 0.01, and 0.001 can be considered statistically significant * suggested statistically significant. * or # indicated P value was less than 0.05, ** or # # indicated P value was less than 0.01, *** or # # # indicated P value was less than 0.001, and **** or # # ## indicated P value was less than 0.0001.
Results
Expression of Y2R is increased in human degenerative intervertebral discs
To detect the role of NPY, Y1R, and Y2R in IDD, we firstly examined the expression level of those proteins above in normal and degenerated disc tissue. Representative Pfirrmann score on MRI images are shown in : grade II and grade Ⅳ, respectively. The results of immunohistochemical analysis (, red arrow) revealed that the expression of Y2R was elevated in human degenerative intervertebral discs. Under healthy condition, NP tissue is rich in NP cells and extracellular matrix (ECM) (type II collagen and aggrecan), which contributes to the highly hydrated nature of the NP, helps maintain IVD height, and distributes loading across the endplates. However, increasing ECM‑catabolic proteinases (such as MMP3 and MMP13) have been the most key catabolic proteins to degenerate type II collagen and aggrecan when the disc degeneration is initiated. Therefore, in this present study, we used the expression of type II collagen and aggrecan to reflect the health condition of NP tissue, and used the expression of MMP3 and MMP13 to reveal the degeneration condition of NP tissue. Western blot results demonstrated that the degenerated intervertebral disc showed higher expression of MMP3, and MMP13, and lower expression of type II collagen and aggrecan compared to normal intervertebral disc, which was consistent with previous study [Citation3] (-). In addition, western blot of human disc tissue also confirmed the relatively higher expression of Y2R in degenerated discs (). Further, we investigated the expression of Y2R in disc tissue with different Pfirrmann scores and found that the expression of Y2R increased with the severity of IDD ( and ). In conclusion, Y2R may be the key receptor for NPY during IDD. However, we did not find expression of NPY and Y1R via immunohistochemical analysis or Western bolt in this study. We deduced that NPY as a local exocrine protein within disc tissue may be extremely low.
Figure 1 Expression of Y2R is increased in human degenerated discs. (a) Representative MRI images and immunohistochemistry for Y2R of the degenerated human discs between Pfirrmann grading II and IV; (b) Western blotting analysis for the expression of Y2R and IDD-related proteins in different samples (Normal group includes the former three ones, and the latter three ones belong to degeneration group). (c-g) The quantitative and statistical analysis of the western blot band (Y2R, MMP3, MMP13, Aggrecan, and Collagen type II); (h) Western blotting analysis for the expression of Y2R in human nucleus pulposus with different Pfirrmann grading; (i) The quantitative and statistical analysis of the western blot band (Y2R)
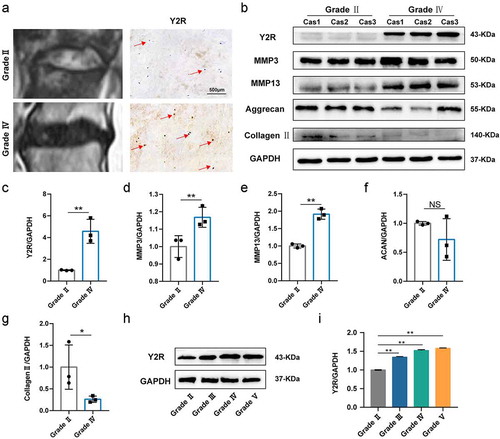
Identification of rat NP cells and establishment of NP cells injury model in vitro
shows no significant changes in cell morphology between passage 1 and passage 4 cells (). Firstly, the isolated cells (Passage 1 and 4) were validated via evaluating the expression of collagen type II and aggrecan via immunofluorescence analysis and toluidine blue stanning, respectively, which were two key markers for NP cells (and ). In addition, we explored the expression of these two key proteins in NP cells with different passages and found no difference regarding the expression of collagen type II and aggrecan via western blot among passage 1, 2 and 4, except passage 0 (D-F). Therefore, the low-passage (<4) cells cultured in monolayers were used for the following experiments. In order to establish injury model of NP cells in vitro, we used IL-1β (30 ng/ml) to stimulate cells. demonstrates that cells treated by IL-1β lost the normal spindle shape and became flat. In addition, cell viability test suggested the cell viability of NP cells with IL-1βwas significantly decreased (. Furthermore, qRT-PCR results showed that IL-1β increased the expression of IDD-related gene, especially at 24 h after IL-1βstimulation. The results above indicated the NP cells injury model can be successfully induced by IL-1β. In addition, to further confirm the expression of NPY and its receptors in NP cells, we used IL-1β with indicated concentrations (0, 10, 30, 50 ng/ml) to intervene NP cells. The results of qRT-PCR revealed that the mRNA expression of NPY in NP cells was increased by IL-1β (10 ng/ml) but was reduced when the concentration of IL-1β was 30 ng/ml and 50 ng/ml (. Furthermore, ELISA analysis of cell cultural supernatant also revealed that the secretion of NPY by NP cells was increased by IL-1β (10 ng/ml) but reduced when the concentration of IL-1β was 30 ng/ml or 50 ng/ml, consistent with the mRNA expression of NPY (. However, the expression of Y2R increased with the elevating concentrations of IL-1β (. Western blot also showed the similar changes of Y2R to qRT-PCR. Because the expression of NPY mRNA decreased and the expression of Y2R was increased significantly (p < 0.001) when the concentration of IL-1β was 30 ng/ml and above, we believe this concentration could result in more severe injury and changes of physiological function to NP cells, which became the reason that why we used the specific concentration of the IL-1β in this study.
Figure 2. Culture and identification of rat nucleus pulposus (NP) cells. (a) The NP cells of passage 1 and 4; (b) Immunofluorescence analysis for collagen type II of NP cells of passage 1 and 4; (c) Toluidine blue staining for aggrecan of NP cells of passage 1 and 4; (d) Western blotting analysis for the expression of collagen type II and aggrecan of NP cells of passage 1 and 4; (e-f) The quantitative and statistical analysis of the western blot band (Aggrecan, and Collagen type II)
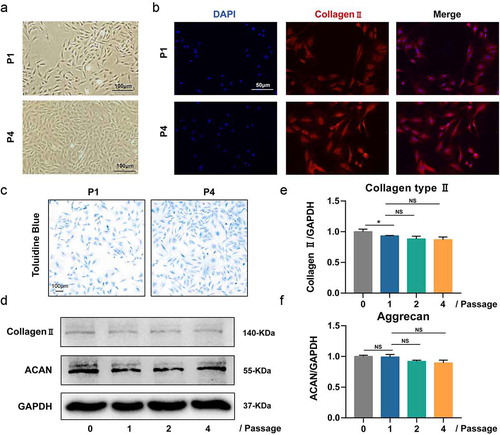
Figure 3. Establishment of nucleus pulposus (NP) cells injury model in vitro. (a) Representative images of cells in normal medium and cells treated by IL-1β (30 ng/ml); (b) Analysis of the cell viability between cells in normal medium and cells treated by IL-1β (30 ng/ml); (c-f) Quantitative analysis of mRNA expression of IDD-related proteins in NP cells treated by IL-1β (30 ng/ml) at indicated time points; (h) qRT-PCR for the expression of NPY mRNA in Rat NP cells treated by IL-1β with indicated concentrations; (i) qRT-PCR for the expression of Y2R mRNA in Rat NP cells treated by IL-1β with indicated concentrations; (j) ELISA analysis for the secretion the content of NPY in cell cultural supernatant of Rat NP cells treated by IL-1β with indicated concentrations; (k) Western blotting analysis for the expression of Y2R protein in Rat NP cells treated by IL-1β with indicated concentrations; (l) The quantitative and statistical analysis of the western blot band (Y2R)
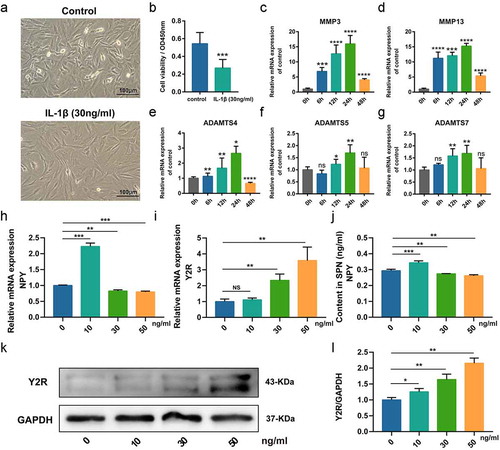
NPY promotes the proliferation of NP cells and protects NP cells against IL‑1β‑induced apoptosis
The present study evaluated the effect of NPY on the proliferation of NP cells and the expression of Ki-67, a marker of cell proliferation, indicated by immunofluorescence analysis that NPY could concentration-dependently promote the proliferation of NP cells (. In addition, the cell viability test results showed that NPY could increase the viability of NP cells in a time- and dose-dependent manner, especially at the concentration of 10−10 mol/L (. qRT-PCR found the increased expression of Cyclin D1 in NP cells treated by NPY (. What’s more, the protein expression of proliferating cell nuclear antigen (PCAN), another key marker of cell proliferation, was also increased in NP cells treated by NPY ( and ). We further used JC-1 staining to evaluate the mitochondrial membrane potential (MMP) of NP cells and found that cells treated by NPY (10−10 mol/L) could reverse the decreased MMP induced by IL‑1β (, and the changes of MMP was also presented as diagram ( . Hoechst staining also indicated that NPY (10−10 mol/L) could reduce the nuclear condensation, abnormally morphological changes(arrows), and apoptosis rate induced of NP cells by IL‑1β (and).
Figure 4 NPY promotes the proliferation of NP cells (a) Immunofluorescence analysis for the expression of Ki-67 in NP cells treated by NPY with different concentrations; (b) Analysis of the cell viability of NP cells treated by NPY (10−8-10−12 mol/L); (c) Quantitative analysis of mRNA expression of Cyclin D1 in NP cells; (d) Western blot for the expression of PCAN in NP cells treated by NPY with different concentrations; (e) The quantitative and statistical analysis of the western blot band (PCAN); (f) JC-1 stanning of NP cells in normal group and NPY-treated group; (g) Diagram of ratio of JC-1 aggregate/monomer; (h) Hoechst stanning of NP cells in normal group and NPY-treated group; (i) The apoptosis rate of NP cells indicated by diagram for Hoechst stanning
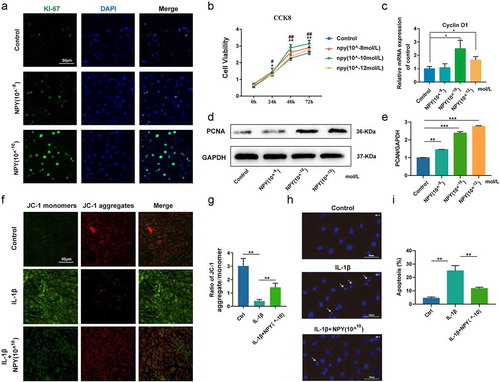
Furthermore, the results of qRT-PCR showed that NPY could lower the mRNA expression of Caspase 3/8 and Bax (A-C). Annexin V-FITC/PI double staining showed that NPY (10−10 mol/L) could protect NP cells against IL‑1β‑induced apoptosis, including early and late apoptosis (). In addition, Western blot results also suggested the inhibitory effect of NPY on the expression of Bax and Cleaved-caspase 3, and reversed the reduced expression of Bcl-2 induced by IL-1β (F-J). In addition, the ratio of Bax/Bcl-2 in IL-1βNP cells was also restored following NPY treatment (.
Figure 5. NPY protects NP cells against IL‑1β‑induced cell apoptosis. (a) Quantitative analysis of the mRNA expression of Bax; (b) Quantitative analysis of the mRNA expression of Caspase 3; (c) Quantitative analysis of the mRNA expression of Caspase 8; (d) Annexin V-FITC/PI double staining of NP cells; (e) Apoptosis rate of NP cells; (f) Western blot for the expression of apoptosis-related proteins (Bax, Bcl-2, and Cleaved caspase 3); (g-j) The quantitative and statistical analysis of the western blot band (Bax, Bcl-2, Bax/Bcl-2, and Cleaved caspase 3)
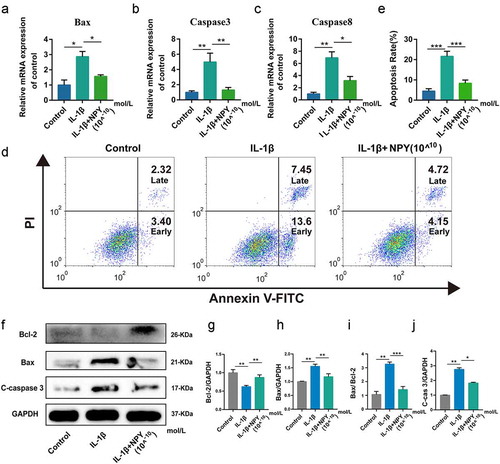
Taken together, NPY could promote the proliferation of NP cells and protect NP cells against IL‑1β‑induced cell apoptosis, and the inhibitory effect of NPY on cell apoptosis may be mediated by mitochondrial pathway.
NPY ameliorates IL-1β-induced extracellular matrix degeneration in vitro
To determine whether NPY could affect IL-1β-induced ECM degradation of NP cells, we firstly investigated the mRNA and protein expression levels of major NP ECM catabolic proteinases, including MMP3, MMP13, ADAMTS4, ADAMTS5, and ADAMTS7. After pre-treatment with NPY for 24 h, IL-1β (30 ng/mol) was added into the cell medium for another 24 h. Then, the mRNA levels were assessed by qRT‑qPCR, and the results revealed that NPY could significantly attenuate IL-1β-induced expression of MMP3, MMP13, ADAMTS4, ADAMTS5, and ADAMTS7 (A-E). Western blot also suggested similar results. In addition, NPY could increase the expression of aggrecan and collagen type II (F-I).
Figure 6. NPY ameliorates IL-1β- induced matrix degeneration in NP Cells. (a-e) Quantitative analysis of mRNA expression of IDD-related genes in NP cells treated by different concentrations of NPY; (f) Western blotting analysis of the expression of IDD-related proteins in NP cells treated by different concentrations of NPY; (g-i) The quantitative and statistical analysis of the western blot band (MMP13, Collagen type II, and aggrecan)
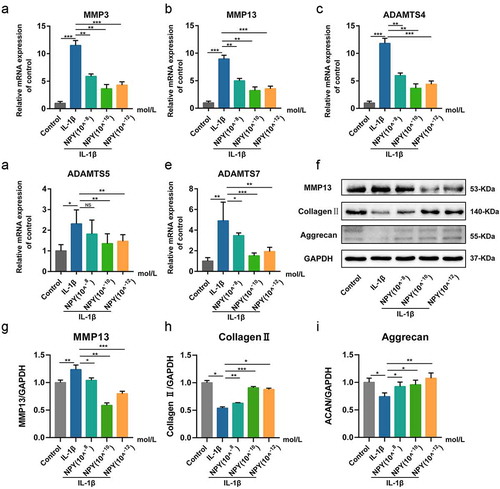
To explore the ECM change by phenotype, we firstly examined the expression of MMP3 and collagen type II in NP cells via immunofluorescence analysis and the results revealed that NPY could significantly decrease the increasing expression of MMP3 induced by IL-1β, while the decreased expression of collagen type II could also be reversed by NPY (. we then examined the secretion of catabolic proteinases in IL-1β-induced NP cells via ELISA, and the results revealed that NPY could also mitigate the increased expression of MMP1/3/13, ADAMTS5, and Collagen type 1 induced by IL-1β, and that the expression of Collagen type II was also increased by NPY while NP cells were injured by IL-1β (B-F). Conclusively, NPY ameliorates IL-1β- induced extracellular matrix degeneration in vitro.
Figure 7. NPY suppresses IL-1β- induced expression and secretion of ECM catabolic proteinases in NP Cells. (a) Immunofluorescence analysis for collagen type II and MMP3 of NP cells treated by IL-1β with or without NPY; (b-g) ELISA analysis for the secretion of ECM catabolic proteinases
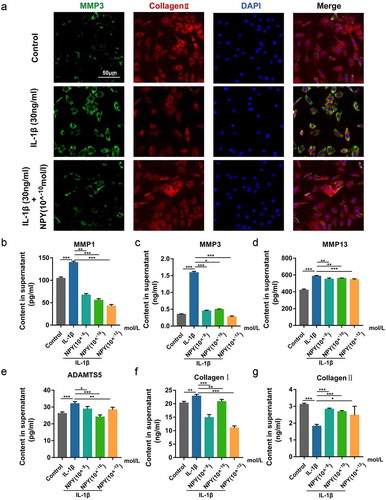
Discussion
IDD is a multifactorial disease in which extracellular matrix degradation and a reduction in the number of NP cells are major characteristics [Citation28]. Therefore, ameliorating the degradation of ECM and reducing the cell apoptosis may postpone the progression of IDD. Increasing studies have suggested excessive inflammatory response may be the major cause for IDD [Citation4,Citation5,Citation9]. Abnormal production and accumulation of local inflammatory mediators, such as IL–1β, could trigger NP cells injury [Citation29–31]. In addition, injured NP cells could also produce pro-inflammatory cytokines which further exacerbate the progression of IDD via a positive feedback loop [Citation32]. IL-1β is reported to be involved in multiple pathological process during IDD [Citation33]. Christine et al. also reported that IL-1β was expressed at higher levels than TNF-αin degenerated IVDs [Citation9]. Therefore, in this present study, we established IL-1β-induced IDD model in vitro, and the results suggested the successful establishment of the model, as indicated by increasing NP cell apoptosis and the expression of ECM catabolic proteinases.
Neuropeptides have been reported to involve in inflammatory responses in IDD, including substance P, vasoactive intestinal peptide (VIP), and calcitonin gene-related peptide (CGRP) [Citation34–36]. However, different from other neuropeptides, NPY have been reported to have a negative effect on immune response [Citation16,Citation17,Citation37,Citation38]. A rodent study revealed that rats with NPY intraperitoneally injected showed a decrease in the pro-inflammatory cytokines IL-1β, TNF-α, and IL-6 in the serum [Citation37]. An in vitro study also showed that NPY could inhibit the IL-1β signaling pathway via nitric oxide production in microglia cells, which also indicated that NPY may inhibit the release of IL-1β [Citation38]. Similarly, Mitic et al. found NPY could inhibit the LPS-induced accumulation of inflammatory cells [Citation16]. What’s more, De la Fuente et al. found that NPY could decrease the phagocytosis capacity of macrophages, and LPS-induced release of TNF-α and IL-1β[Citation17]. NPY can also increase the production of anti-inflammatory cytokines including transforming growth factorβ [Citation39]. These studies above suggested that the inhibitory effect of NPY on inflammatory response. Therefore, this present study aimed to investigate the effects of NPY on NP cells in vitro.
NPY and its receptors are mainly located in nerve systems. However, whether NPY and its receptors can be expressed by NP cells remains unknown. In the present study, we first explored the expression of NPY and its receptors in human intervertebral discs and found that the expression of Y2R increased with the severity of IDD. Furthermore, in vitro experiment also revealed that the expression of Y2R was increased by IL-1β in a dose-dependent manner. Notably, in vitro ELISA test demonstrated that the secretion level of NPY was increased by slight inflammation injury from IL-1β but reduced when the inflammation injury was moderate and above. A recent study by Malcolm E et al. also found that rabbit AF cells could produce a significantly greater amount of NPY in response to inflammatory conditions via ELISA test [Citation40]. These results above suggested a endogenous NPY-Y2R axis existing in rat NP cells, and an increase of NPY induced by IL-1β(10 ng/ml) may suggest that in a condition with moderate inflammatory stress, the NP cells could activate self-protection via increasing the secretion of NPY by means of an autocrine or paracrine manner. A recent study by Wang et al. suggested that NPY could suppress the activation of the LPS-induced inflammasome via Y2Rs [Citation41]. In addition, the anti-inflammatory effect of Y2R agonist has also been reported in endo-toxemic animals [Citation42]. On the other hand, NPY has been reported to be correlate with chronic inflammatory pain [Citation43,Citation44]. Pain usually is considered as a single of self-protection of the body. Therefore, we deduced that during early-stage of IDD, the secretion of NPY is initiated and may be involved in the discogenic pain, which could be considered as an early warning to reveal forthcoming symptomatic IDD, whereas with the deterioration of IDD, the excessive inflammatory stress may disturb the secretion of NPY. However, the increased expression of Y2R in degenerated intervertebral disc or Il-1β-induced NP cells may be the compensatory action for local decreasing NPY level. However, the mechanisms underlying the immune-modulating and stress-buffering actions of NPY-Y2R axis contributing to the attenuation of IDD are complicated and further studies are further required.
Abnormal NP cell apoptosis has been proposed as an important pathological mechanism of IDD [Citation45,Citation46]. Mitochondrial pathway-induced apoptosis includes the decrease of anti-apoptotic members of the Bcl-2 family as well as the release of pro-apoptotic members of Bax, and finally triggers activation of caspase family, such as caspase 3/8 [Citation47]. It is well-known that mitochondrial pathway-induced apoptosis frequently serves as a critical factor in degenerative diseases [Citation48,Citation49]. In this present study, we found that treatment with NPY could enhance the viability and proliferation of NP cells, as indicated by the expression of Cyclin D1, PCNA, and Ki-67. Simultaneously, NPY could reverse IL-1β-induced the injury of mitochondrion, a marker for early cell apoptosis. On the other hand, NPY could also alleviate IL-1β-induced NP cell apoptosis indicated by suppressing the expression of mitochondrial pathway-induced cell apoptosis indicators (Bax, Bcl–2, and Casepase 3), consistent with previous study [Citation50–52]. Taken together, this present study confirmed that the mitochondrial pathway might be involved in the anti-apoptosis effect of NPY on NP cells. In addition, mitogen-activated protein kinase (MAPK) plays an important role in the regulation of cellular proliferation, differentiation, and survival. Previous studies have reported that the cellular protection of NPY mediated via Y2Rs may involve the activation of MAPK (ERK1/2) pathways [Citation53]. However, the exact singling pathways of NPY affecting the proliferation and apoptosis of NP cells need future investigation.
The imbalance of anabolism and catabolism of ECM within NP tissue has been another typical characteristic during the development of IDD [Citation5]. When IDD occurs owing to age or other pathological factors, the overall aggrecan content of the IVD declines, and collagen type II fibers are replaced with collagen type I fibers [Citation5]. Those pathological changes can be attributed to increased production of ECM‑catabolic proteinases, including MMPs (3/9) and ADAMTSs (4/5/7). To investigate the effect of NPY on the expression of those ECM‑catabolic proteinases above, the NP cells were treated by IL-1β after pretreatment with NPY with varying concentrations. The qRT-PCR and western blot results demonstrated NPY could significantly suppress the increased gene expression of ECM‑catabolic proteinases induced by IL-1β. What’s more, the immunofluorescence assay showed that NPY could significantly decrease the increasing expression of MMP3 induced by IL-1β, whereas the decreased expression of collagen type II could also be reversed by NPY. In addition, considering that these catabolic proteinases frequently functioning at extracellular environment, we further examined the secretion of catabolic proteinases in IL-1β-induced NP cells via ELISA, and the results revealed that NPY could also mitigate the increased secretion of MMP1/3/13, ADAMTS5, and collagen type I induced by IL-1β, whereas the reduced secretion of collagen type II was also reversed by NPY, which can also be considered as indicators for the protective effect of NPY on ECM in NP cells. Therefore, NPY has the ability to ameliorate the progression of IDD by suppressing the expression of ECM catabolic proteinases.
However, this present study has several limitations. Firstly, this study was mainly conducted in vitro, and its conclusions may not necessarily be indicative of that which occurs in vivo. Whether NPY within the disc tissue has the protective impact in vivo remains to be fully elucidated. Secondly, the in vitro experiment was conducted using rat NP cells. As described previous studies, there may be biological difference between human NP cells and rat NP cells. Therefore, despite the gene homology between the human and rat NP cells, studies with human NP cells will be better to reflex the exact mechanism of NPY on IDD. Thirdly, NPY has been reported to be correlate with chronic inflammatory pain, and more complex in vivo analysis with local NPY-Y2R axis inhibition and assessment for the presence or absence of pain-related behaviors are warranted for future investigation.
Conclusion
In conclusion, our study firstly reported the existence of NPY-Y2R axis in rat NP tissue and provided the evidence that treatment with NPY significantly promoted rat NP cells proliferation and suppressed rat NP cells apoptosis in vitro via mitochondrial pathway. In addition, NPY could ameliorate IL-1β-induced ECM degeneration in vitro and increase the expression of aggrecan and collagen type II. Therefore, based on the results of this present study, we concluded that the role of NPY may be not only involved in discogenic pain, but also ameliorate the progression of IDD by protecting NP cells against apoptosis and suppressing ECM degeneration.
Acknowledgments
We would like to thank Professor Weizhong Wang and Professor Yangkai Wang from Second Military Medical University for their experimental platform and useful suggestions on my research.
Disclosure statement
The authors have declared no any conflict of interest.
Additional information
Funding
References
- Walker BF. The prevalence of low back pain: a systematic review of the literature from 1966 to 1998. Clin Spine Surg. 2000;13:205–217.
- Peng B, Wu W, Hou S, et al. The pathogenesis of discogenic low back pain. J Bone Joint Surg Br Vol. 2005;87:62–67.
- Guo J, Shao M, Lu F, et al. Role of Sirt1 plays in nucleus pulposus cells and intervertebral disc degeneration. Spine (Phila Pa 1976). 2017;42(13):E757–E766. .
- Roughley PJ. Biology of intervertebral disc aging and degeneration: involvement of the extracellular matrix. Spine (Phila Pa 1976). 2004;29(23):2691–2699.
- Dowdell J, Erwin M, Choma T, et al. Intervertebral disk degeneration and repair. Neurosurgery. 2017;80(3S):S46–S54. .
- Sakai D, Grad S. Advancing the cellular and molecular therapy for intervertebral disc disease. Adv Drug Deliv Rev. 2015;84:159–171.
- Gorth DJ, Shapiro IM, Risbud MV. Transgenic mice overexpressing human TNF-α experience early onset spontaneous intervertebral disc herniation in the absence of overt degeneration. Cell Death Dis. 2018;10:1–14.
- Nakazawa KR, Walter BA, Laudier DM, et al. Accumulation and localization of macrophage phenotypes with human intervertebral disc degeneration. Spine J. 2018;18(2):343–356. .
- Le Maitre CL, Hoyland JA, Freemont AJ. Catabolic cytokine expression in degenerate and herniated human intervertebral discs: IL-1β and TNFα expression profile. Arthritis Res Ther. 2007;9(4):R77.
- Tatemoto K, Carlquist M, Mutt V. Neuropeptide Y—a novel brain peptide with structural similarities to peptide YY and pancreatic polypeptide. Nature. 1982;296(5858):659–660.
- Li C, Wu X, Liu S, et al. Roles of neuropeptide Y in neurodegenerative and neuroimmune diseases. Front Neurosci. 2019;13:869. .
- Amano S, Arai M, Goto S, et al. Inhibitory effect of NPY on isoprenaline-induced osteoclastogenesis in mouse bone marrow cells. Biochim Biophys Acta. 2007;1770(6):966–973. .
- Loh K, Herzog H, Shi Y-C. Regulation of energy homeostasis by the NPY system. Trends Endocrinol Metab. 2015;26(3):125–135.
- Farzi A, Reichmann F, Holzer P. The homeostatic role of neuropeptide Y in immune function and its impact on mood and behaviour. Acta Physiol. 2015;213(3):603–627.
- Schwarz H, Villiger PM, Von Kempis J, et al. Neuropeptide Y is an inducible gene in the human immune system. J Neuroimmunol. 1994;51(1):53–61. .
- Mitić K, Stanojević S, Kuštrimović N, et al. Neuropeptide Y modulates functions of inflammatory cells in the rat: distinct role for Y1, Y2 and Y5 receptors. Peptides. 2011;32(8):1626–1633. .
- De La Fuente M, Del Rıo M, Medina S. Changes with aging in the modulation by neuropeptide Y of murine peritoneal macrophage functions. J Neuroimmunol. 2001;116:156–167.
- Wang L, Zhang L, Pan H, et al. Levels of neuropeptide Y in synovial fluid relate to pain in patients with knee osteoarthritis. BMC Musculoskelet Disord. 2014;15(1):319. .
- Brismée J-M, Sizer PS Jr, Dedrick GS, et al. Immunohistochemical and histological study of human uncovertebral joints: a preliminary investigation. Spine (Phila Pa 1976). 2009;34(12):1257–1263. .
- Ahmed M, Bjurholm A, Kreicbergs A, et al. Neuropeptide Y, tyrosine hydroxylase and vasoactive intestinal polypeptideimmunoreactive nerve fibers in the vertebral bodies, discs, dura mater, and spinal ligaments of the rat lumbar spine. Spine (Phila Pa 1976). 1993;18:268–273.
- Ashton IK, Roberts S, Jaffray DC, et al. Neuropeptides in the human intervertebral disc. J Orthop Res. 1994;12(2):186–192. .
- Chen R, Hao Z, Chen X, et al. Neuropeptide Y enhances proliferation and chondrogenic differentiation of ATDC5 cells. Neuropeptides. 2020;80:102022.
- Sowa GA, Perera S, Bechara B, et al. Associations between serum biomarkers and pain and pain-related function in older adults with low back pain: a pilot study. J Am Geriatr Soc. 2014;62(11):2047–2055. .
- Schell E, Theorell T, Hasson D, et al. Stress biomarkers’ associations to pain in the neck, shoulder and back in healthy media workers: 12-month prospective follow-up. Eur Spine J. 2008;17:393–405.
- Goode AP, Schwartz TA, Kraus VB, et al. Inflammatory, structural, and pain biochemical biomarkers may reflect radiographic disc space narrowing: the Johnston county osteoarthritis project. J Orthop Res. 2020;38(5):1027–1037. .
- Chen F, Liu H, Li Z, et al. Paraoxonase 1 was negatively associated with intervertebral disc degeneration. Spine (Phila Pa 1976). 2019;44(18):E1053–E1062. .
- Wang J, Chen H, Cao P, et al. Inflammatory cytokines induce caveolin‐1/β‐catenin signalling in rat nucleus pulposus cell apoptosis through the p38 MAPK pathway. Cell Prolif. 2016;49:362–372.
- Vergroesen -P-P, Kingma I, Emanuel KS, et al. Mechanics and biology in intervertebral disc degeneration: a vicious circle. Osteoarthritis Cartilage. 2015;23(7):1057–1070. .
- Kedong H, Wang D, Sagaram M, et al. Anti-inflammatory effects of interleukin-4 on intervertebral disc cells. Spine J. 2020;20(1):60–68. .
- Andrade P, Hoogland G, Garcia MA, et al. Elevated IL-1β and IL-6 levels in lumbar herniated discs in patients with sciatic pain. Eur Spine J. 2013;22(4):714–720. .
- Weiler C, Nerlich AG, Bachmeier BE, et al. Expression and distribution of tumor necrosis factor alpha in human lumbar intervertebral discs: a study in surgical specimen and autopsy controls. Spine (Phila Pa 1976). 2005;30(1):44–53. .
- Shamji MF, Setton LA, Jarvis W, et al. Proinflammatory cytokine expression profile in degenerated and herniated human intervertebral disc tissues. Arthritis Rheumatism. 2010;62:1974–1982.
- Johnson ZI, Schoepflin ZR, Choi H, et al. Disc in flames: roles of TNF-α and IL-1β in intervertebral disc degeneration. Eur Cell Mater. 2015;30:104.
- Kepler CK, Markova DZ, Hilibrand AS, et al. Substance P stimulates production of inflammatory cytokines in human disc cells. Spine (Phila Pa 1976). 2013;38(21):E1291–E1299. .
- Kepler CK, Markova DZ, Koerner JD, et al. Substance P receptor antagonist suppresses inflammatory cytokine expression in human disc cells. Spine (Phila Pa 1976). 2015;40(16):1261–1269.
- McCarthy P, Carruthers B, Martin D, et al. Immunohistochemical demonstration of sensory nerve fibers and endings in lumbar intervertebral discs of the rat. Spine (Phila Pa 1976). 1991;16(6):653–655. .
- Oztas B, Sahin D, Kir H, et al. The effect of leptin, ghrelin, and neuropeptide-Y on serum Tnf-alpha, Il-1beta, Il-6, Fgf-2, galanin levels and oxidative stress in an experimental generalized convulsive seizure model. Neuropeptides. 2017;61:31–37.
- Ferreira R, Santos T, Viegas M, et al. Neuropeptide Y inhibits interleukin-1beta-induced phagocytosis by microglial cells. J Neuroinflammation. 2011;8:169.
- Zhou JR, Xu Z, Jiang CL. Neuropeptide Y promotes TGF-beta1 production in RAW264.7 cells by activating PI3K pathway via Y1 receptor. Neurosci Bull. 2008 Jun;24(3):155–1559.
- Dombrowski ME, Olsen AS, Vaudreuil N, et al. Rabbit annulus fibrosus cells express neuropeptide Y, which is influenced by mechanical and inflammatory stress. Neurospine. 2020;17(1):69–76. .
- Wang W, Xu T, Chen X, et al. NPY receptor 2 mediates NPY antidepressant effect in the mPFC of LPS rat by suppressing NLRP3 signaling pathway. Mediators Inflamm. 2019;2019:7898095. .
- Stadler J, Le TP, Haas P, et al. Distinct effects of NPY13-36, a specific NPY Y2 agonist, in a model of rodent endotoxemia on leukocyte subsets and cytokine levels. Ann Anat. 2011;193(6):486–493.
- Diaz-delcastillo M, Woldbye DPD, Heegaard AM. Neuropeptide Y and its involvement in chronic pain. Neuroscience. 2018;387:162–169. .
- Fu W, Nelson TS, Santos DF, et al. An NPY Y1 receptor antagonist unmasks latent sensitization and reveals the contribution of protein kinase A and Epac to chronic inflammatory pain. Pain. 2019;160(8):1754–1765. .
- Han Y, Li X, Yan M, et al. Oxidative damage induces apoptosis and promotes calcification in disc cartilage endplate cell through ROS/MAPK/NF-κB pathway: implications for disc degeneration. Biochem Biophys Res Commun. 2019;516(3):1026–1032. .
- Cheng X, Zhang L, Zhang K, et al. Circular RNA VMA21 protects against intervertebral disc degeneration through targeting miR-200c and X linked inhibitor-of-apoptosis protein. Ann Rheum Dis. 2018;77(5):770–779. .
- Zhao C-Q, Jiang L-S, Dai L-Y. Programmed cell death in intervertebral disc degeneration. Apoptosis. 2006;11(12):2079–2088.
- Green DR, Reed JC. Mitochondria and apoptosis. science. 1998;281(5381):1309–1312.
- Nunnari J, Suomalainen A. Mitochondria: in sickness and in health. Cell. 2012;148(6):1145–1159.
- Coleman MC, Goetz JE, Brouillette MJ, et al. Targeting mitochondrial responses to intra-articular fracture to prevent posttraumatic osteoarthritis. Sci Transl Med. 2018;10(427):eaan5372. .
- Hu Y, Shao Z, Cai X, et al. Mitochondrial pathway is involved in advanced glycation end products-induced apoptosis of rabbit annulus fibrosus cells. Spine (Phila Pa 1976). 2019;44(10):E585. .
- Wu J, Liu S, Meng H, et al. Neuropeptide Y enhances proliferation and prevents apoptosis in rat bone marrow stromal cells in association with activation of the Wnt/β-catenin pathway in vitro. Stem Cell Res. 2017;21:74–84.
- Fatoba O, Kloster E, Reick C, et al. Activation of NPY-Y2 receptors ameliorates disease pathology in the R6/2 mouse and PC12 cell models of Huntington’s disease. Exp Neurol. 2018;302:112–128.