ABSTRACT
Hyperthermia has been used for cancer therapy for a long period of time, but has shown limited clinical efficacy. Induction-heating hyperthermia using the combination of magnetic nanoparticles (MNPs) and an alternating magnetic field (AMF), termed magnetic hyperthermia (MHT), has previously shown efficacy in an orthotopic mouse model of disseminated gastric cancer. In the present study, superparamagnetic iron oxide nanoparticles (SPIONs), a type of MNP, were conjugated with an anti-HER2 antibody, trastuzumab and termed anti-HER2-antibody-linked SPION nanoparticles (anti-HER2 SPIONs). Anti-HER2 SPIONs selectively targeted HER2-expressing cancer cells co-cultured along with normal fibroblasts and HER2-negative cancer cells and caused apoptosis only in the HER2-expressing individual cancer cells. The results of the present study show proof-of-concept of a novel hyperthermia technology, immuno-MHT for selective cancer therapy, that targets individual cancer cells.
Abbreviations: AMF: alternating magnetic field; DDW: double distilled water; DMEM: Dulbecco’s Modified Eagle’s; Medium; f: frequency; FBS: fetal bovine serum; FITC: Fluorescein isothiocyanate; GFP: green fluorescent protein; H: amplitude; Hsp: heat shock protein; MHT: magnetic hyperthermia; MNPs: magnetic nanoparticles; PI: propidium iodide; RFP: red fluorescent protein; SPION: superparamagnetic iron oxide (Fe3O4) nanoparticle.
Introduction
Hyperthermia has a long history for cancer treatment [Citation1]. However, conventional hyperthermia treatments heat both tumor tissues as well as normal tissues and therefore have dose-limiting toxicity.
Magnetic nanoparticles (MNPs) have been used to target tumor tissues followed by applying an alternating magnetic field (AMF) from outside of the body to selectively heat tumors [Citation2]. This treatment is called magnetic hyperthermia (MHT) [Citation3]. MHT has been approved for glioblastoma in Europe [Citation4]. MHT has been conducted mainly by direct injection of MNPs into tumor tissue. Our laboratory recently showed the efficacy of MHT in an orthotopic mouse model of disseminated gastric cancer using superparamagnetic iron oxide nanoparticles (SPIONs) as MNPs [Citation5]. In the present study, we have developed cancer-targeting immuno-SPIONs conjugated with an anti-HER2-antibody and demonstrated that these immuno-SPIONs caused selective targeting and eradication of individual HER2-expressing cancer cells as a novel therapy termed immuno-hyperthermia.
Materials and methods
Cell lines
The human breast cancer cell line AU565 was purchased from the American Type Culture Collection (ATCC, Manassas, VA, USA). The human gastric-cancer cell line NUGC-4 was purchased from JCRB Cell Bank (National Institute of Biomedical Innovation, Osaka, Japan). The human colon-cancer cell line HCT-116-RFP (AntiCancer Inc., San Diego, CA, USA), which expresses the red fluorescent protein (RFP) gene, was established previously [Citation6–8]. The human fetal esophageal fibroblast cell line FEF3-GFP, which expresses the green fluorescent protein (GFP) gene, was established previously [Citation9,Citation10]. SKOV-3 was purchased from Cell Biolabs, Inc. (San Diego, CA, USA). AU565, NUGC-4, and HCT-116-RFP cells were cultured in RPMI-1640 medium (Sigma-Aldrich, St.Louis, MO, USA) with 10% fetal bovine serum (FBS; Gibco, Thermo Fisher Scientific, Waltham, MA, USA); FEF3-GFP and SKOV-3 cells were cultured in Dulbecco’s Modified Eagle’s medium (DMEM; Sigma-Aldrich, St.Louis, MO, USA) with 10% FBS. All media were supplemented with 100 U/ml penicillin and 100 mg/ml streptomycin (Sigma-Aldrich) and 10% FBS. Cells were maintained at 37°C in a humidified atmosphere with 5% CO2.
SPIONs
Superparamagnetic iron oxide (Fe3O4) nanoparticles (SPIONs) comprise a core of iron oxide nanoparticles surrounded by surface coatings (). SPIONs of 10 nm core diameter with two types of surface coatings were used in the present study. Carboxydextran-coated SPIONs were kindly supplied by Meito Sangyo Co., Ltd. (Nagoya, Japan). Amphiphilic polymer-coated SPIONs were purchased from Ocean NanoTech, LLC (San Diego, CA, USA). Both surface coatings have carboxyl groups which react with EDC/NHS. The iron concentration of both types of surface coatings was adjusted to 5 mg Fe/ml. The SPION solution was sterilized through a 0.2 μm membrane filter before use.
Figure 1. Production and delivery of HER2-targeted antibody-conjugated superparamagnetic iron oxide nanoparticles (SPIONs)
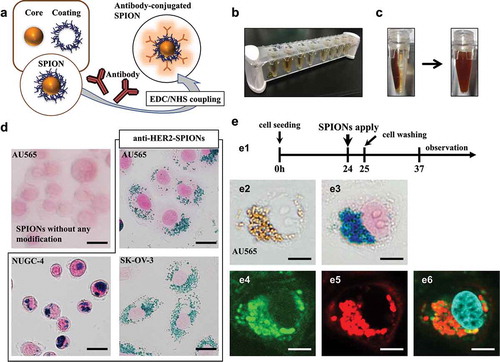
Antibody-conjugated SPIONs
For production of antibody-conjugated SPIONs, the EDC/NHS reaction was used to couple the carboxyl group (–COOH) of the surface coating of the SPIONs with the amine group (–NH2) of the antibody [Citation11] (). The conjugation was performed with the Iron Oxide Nanoparticle Conjugation Kit (ICK-10-005; Ocean NanoTech, LLC, San Diego, CA, USA) according to the manufacturer’s protocol. After SPIONs (1 mg) were diluted in sodium borate, the EDC/sulfo-NHS mixture (2 mg/ml EDC and 1 mg/ml sulfo-NHS) were added to the SPIONs. After 30 minutes reaction at room temperature, 1 mg of antibody, suspended in sodium borate at 2 mg/ml concentration, was added to the SPION solution, and allowed to react for 2 hours at room temperature. The solution was quenched by adding 2-(2-aminoethoxy) ethanol. Products were magnetically collected and washed twice with double-distilled water (DDW) using a magnetic separator (DynaMag-2TM; Life Technologies, Carlsbad, CA, USA) (). The suspension was stored in DDW at a concentration of 1 mg/ml at 4°C. Since the suspension aggregates easily, it was re-suspended in a bath sonicator for 1 minute before use (). A humanized monoclonal antibody targeting HER2, trastuzumab (Chugai Pharmaceutical, Tokyo, Japan) [Citation12], was bound to the SPIONs via a surface coating (carboxydextran or an amphiphilic polymer). The resultant immuno-SPIONs were termed anti-HER2-carboxydextran-coated SPIONs and anti-HER2-amphiphilic polymer-coated SPIONs, respectively.
To evaluate the anti-HER2-SPIONs tropism toward HER2-overexpressing cancer cells, anti-HER2-carboxydextran-coated SPIONs or SPIONs without any modification were administrated to HER2-overexpressing cancer cell lines (AU565, NUGC-4 and SKOV-3). The cells were fixed with 4% paraformaldehyde (FUJIFILM Wako Pure Chemical Corporation, Osaka, Japan, #163-20145) for 15 min, permeabilized and blocked with 0.2% Triton® X-100 (Nacalai Tesque Inc., Kyoto, Japan, #35501-02) diluted by Blocking One (Nacalai Tesque Inc., Kyoto, Japan, #03953-95) for 10 min, and then were stained with Prussian blue which detects ferric iron. Prussian-blue staining was performed according to the manufacturer’s protocol (Iron Stain Kit, ScyTek Laboratories, Inc., Logan, UT, USA, #IRN-1-IFU).
Anti-HER2-SPION delivery to HER2-over-expressing cells
HER2-over-expressing cells (AU565, NUGC-4, and SKOV-3) were seeded in a 35 mm glass-based dish (Iwaki, Tokyo, Japan) at a density of 1 × 105 cells per well containing 3 ml culture medium and grown for 24 hours. After 1 hour, anti-HER2-carboxydextran-coated SPIONs at 100 µg/ml concentration were added to the cells. The cells were washed twice with fresh medium to remove excess SPIONs, and the cells were incubated for another 12 hours. AU565 cells were immunostained with Alexa Fluor® 647-labeled goat anti-human IgG (H+L) antibody (Thermo Fisher Scientific, Waltham, MA, #A-21445) to visualize the Fc fragment of trastuzumab, and with fluorescein isothiocyanate (FITC) coupled anti-dextran antibody (Clone DX1; Stem Cell Technologies, Vancouver, Canada, #60026FI.1) to visualize the carboxydextran surface coating of the anti-HER2-carboxydextran-coated SPIONs. Cellular images were acquired using an inverted microscope (IX71; Olympus Optical, Tokyo, Japan) or a confocal laser-scanning microscope (FV10i; Olympus) and were analyzed using Image J software (National Institutes of Health, Bethesda, MD, USA).
Determination of HER2-targeted delivery and cellular uptake of anti-HER2-SPIONs
One hour after AU565 cells were administrated with anti-HER2-carboxydextran-coated SPIONs, the cells were washed to remove excess SPIONs and cultured in fresh medium. The anti-HER2-SPIONs attached to the cells were observed with an inverted microscope (IX71) at different time points. To evaluate cancer antigen-specific delivery of the anti-HER2-SPIONs, the SPIONs were administrated to co-cultured AU565 (HER2-positive cancer cells), HCT-116-RFP (HER2-negative cancer cells) and HER2-negative GFP-FEF3 cells (normal cells). After 1 hour incubation, the cells are washed to remove excess SPIONs and cultured in fresh medium. The cells were observed 12 hours after administration of the anti-HER2-SPIONs.
Immuno-magnetic hyperthermia (MHT) system
An alternating magnetic field (AMF) was produced by a 2.4 kW radio-frequency generator (EASYHEAT 2.4; A meritherm Inc, Scottsville, KY, USA) containing a water-cooled, three-turn spiral coil with an internal diameter of 38 mm. Magnetic hyperthermia (MHT) was performed by placing a cell-containing dish over the coil that generates an AMF, with amplitude (H) = 31 kA/m and frequency (f) = 280 kHz for 30 minutes ().
Cell viability assay
A Cell Proliferation Kit II (Roche Molecular Biochemicals, Indianapolis, IN, USA), which is based on a sodium 3'-[1-(phenylaminocarbonyl)-3,4-tetrazolium]-bis(4-methoxy-6-nitro) benzene sulfonic acid hydrate (XTT) assay, was used according to the manufacturer’s protocol.
Determination of therapeutic efficacy of immuno-magnetic hyperthermia
AU565 or NUGC-4 cells were seeded in 35 mm glass-based dishes at a density of 1 × 105 cells per well containing 3 ml culture medium and grown for 24 hours. To evaluate cytotoxicity of anti-HER2-carboxydextran-coated SPIONs, the cells were cultured with 100 µg SPIONs at 100 µg/ml for 1 hour and washed twice with fresh medium to remove excess SPIONs. AMF with an H= 31 kA/m and f= 280 kHz was applied to the cells for 30 minutes.
The XTT cell-staining assay was performed at 48 hours after each treatment. Image analysis was also performed with a confocal microscope (FV10i), for determination of the cell-death index using dual staining with propidium iodide (PI) (Sigma-Aldrich, #P4864) for nonviable cells, and Hoechst 33342 (Thermo Fisher Scientific, #H-1399) for all cells in . The percentage of cell death in each treatment group was calculated by counting the number of PI-positive nuclei and normalizing it with the number of Hoechst 33342-positive nuclei. The efficacy of MHT using SPIONs with different surface coatings as well as anti-HER2-amphiphilic polymer-coated SPIONs, was also evaluated on AU565 and NUGC-4 cells in the same way as anti-HER2-carboxydextran-coated SPIONs described above.
Determination of the cell-death mechanism and heat-shock-protein expression after immuno-magnetic hyperthermia
AU565 cells were seeded in 35 mm glass-based dishes at a density of 1 × 104 cells per well containing 3 ml culture medium and grown for 3 days. Anti-HER2-carboxydextran-coated SPIONs (100 µg) at 100 µg/ml were applied to the cells. Five hours after administration of the anti-HER2-SPIONs, cells were washed twice and supplied with fresh medium. Immuno-MHT was performed by applying AMF for 30 minutes. 48 hours later AU565 cells were stained using the GFP-CERTIFIED® Apoptosis/Necrosis Detection Kit (Enzo Life Science, Farmingdale, NY, USA) for 30 minutes and observed using a confocal laser-scanning microscope (FV10i). The Apoptosis/Necrosis Detection kit stains early apoptotic cells with the apoptosis detection reagent Annexin V-EnzoGold (Ex/Em: 550/570 nm, red fluorescence, Enzo Life Science). Early necrotic cells were stained positive with a necrosis detection reagent (7-AAD, Ex/Em: 546/647 nm, Enzo Life Science) [Citation13]. To evaluate the expression of heat shock protein 70 (Hsp70) after immuno-MHT, the cells were stained with an anti-Hsp70 antibody (Alexa Fluor® 488, Abcam, Cambridge, UK, #ab197870).
Time-lapse imaging after immuno-magnetic hyperthermia
HER2-overexpressing AU565 cancer cells (3 × 104) and normal FEF3-GFP fibroblasts (2 × 104) were seeded together in 35 mm glass-based dishes containing 3 ml culture medium. The co-cultured cells were administrated anti-HER2-conjugated amphiphilic polymer-coated SPIONs (200 µg) and AMF was applied for 30 minutes. Starting at 30 minutes after application of AMF, time-lapse images were acquired using a confocal microscope (FV10i, Olympus).
Statistical analysis
Statistical analysis was performed using the Student’s t-test in Excel (Microsoft, Redmond, USA). All values are presented as mean ± standard deviation (SD) of at least three independent experiments. p-values of 0.05 or less between groups were considered statistically significant.
Results
Targeted delivery of anti-HER2-conjugated SPIONs to HER2-expressing cancer cells
When administered with anti-HER2-conjugated-carboxydextran-coated SPIONs, Prussian-blue-stained particles were observed in the peri-nuclear area of HER2-expressing AU565, NUGC-4 and SKOV-3 cells as early as 12 hours (), indicating the SPIONs have entered the cancer cells. No blue particles were observed in the cells administrated SPIONs without antibody conjugation, indicating the selective targeting of anti-HER2-conjugated-carboxydextran-coated SPIONs.
Blue-stained particles were confirmed as anti-HER2-conjugated-carboxydextran-coated SPIONs by immunostaining with Alexa Fluor® 647-labeled goat anti-human trastuzumab IgG of the anti-HER2-conjugated SPIONs, and with FITC-labeled anti-dextran antibody for detecting surface coating of the SPIONs ().
The localization of anti-HER2-conjugated-carboxydextran-coated SPIONs after administration to HER2-overexpressing AU565 cells was determined at different time points by Prussian-blue staining, before and after cell permeabilization. Prussian-blue staining, after cell permeabilization, detected intracellular ferric iron by the brown SPIONs turning blue, indicating that the SPIONs were in the cytoplasm ().
Figure 2. Cancer-associated-antigen-dependent delivery and cellular internalization of anti-HER2-conjugated SPIONs
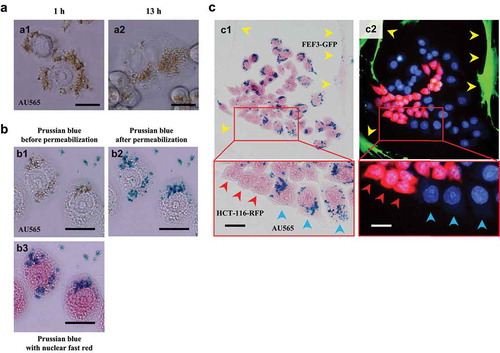
Figure 3. Immuno-magnetic hyperthermia (MHT) system with anti-HER2-conjugated SPIONs heated by an alternating magnetic field (AMF)
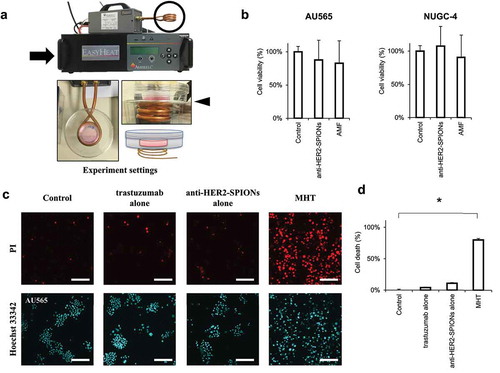
Cancer-associated-antigen-dependent delivery of anti-HER2-conjugated SPIONs
To demonstrate the specific targeting of the anti-HER2-SPIONs, they were administrated to a co-culture of AU565 (HER2-positive cancer cells), HCT-116-RFP (HER2-negative cancer cells) and FEF3-GFP cells (HER2-negative normal cells). Prussian-blue staining at 12 hours after administration showed that the anti-HER2-conjugated SPIONs were specifically delivered only to AU565 cells, demonstrating they were specific for HER2 ().
Cytotoxicity of immuno-MHT
To evaluate the cytotoxicity of immuno-MHT, AU565 and NUGC-4 were first administrated anti-HER2 antibody or anti-HER2-conjugated-carboxydextran-coated SPIONs without applying AMF, or AMF alone. There was not a significant decrease of cell viability with anti-HER2 antibody alone, anti-HER2-conjugated SPIONs-administration alone, or AMF alone, in either cell line (). AMF with anti-HER2-conjugated SPIONs caused a significant increase of death of AU565 cells (). AMF with anti-HER2-conjugated-amphiphilic polymer-coated SPIONs also caused AU565 cell death ().
Figure 4. Intra-cellular magnetic hyperthermia (MHT) using antibody-conjugated SPIONs with an amphiphilic-polymer coating
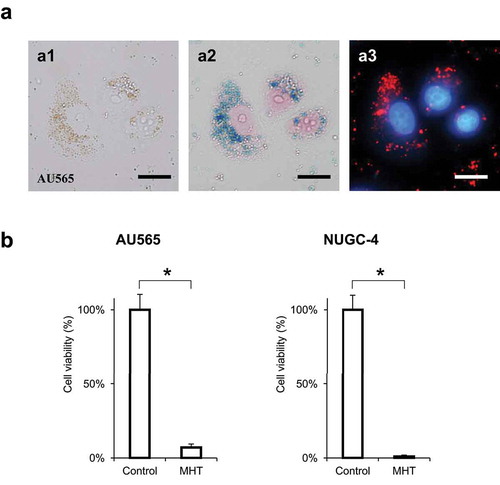
Immuno-MHT induces apoptosis of HER2-expressing AU565 cells
AU565 cells were treated with immuno-MHT. The cells were observed using the Apoptosis/Necrosis Detection Kit with an anti-Hsp70 antibody 48 hours after MHT. Immuno-MHT-treated cells showed predominant red fluorescence, indicating early apoptosis, rather than green fluorescence, which indicates early necrosis (). Induction of heat-shock protein 70 (Hsp70), which is an anti-apoptotic protein, was also observed in the cells treated with immuno-MHT by immunostaining with anti-Hsp 70 antibody (). These results suggest that immuno-MHT induces apoptosis by generating intra-cellular heating.
Kinetic response to immuno-magnetic hyperthermia
In the untreated control group, AU565 cancer cells showed active cell growth. Treatment of AU565 cells with immuno-MHT resulted in cell death by 6 hours as shown by round, highly light-refractile cells, under phase-contrast microscopy, with the co-cultured FEF3-GFP cells over-growing the culture ().
Figure 5. Immuno-MHT induces apoptosis of HER2-expressing AU565 cells, indicated by an anti-Hsp70 antibody
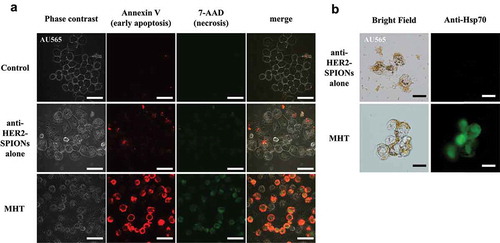
Figure 6. Kinetic response to cancer-specific immuno-MHT
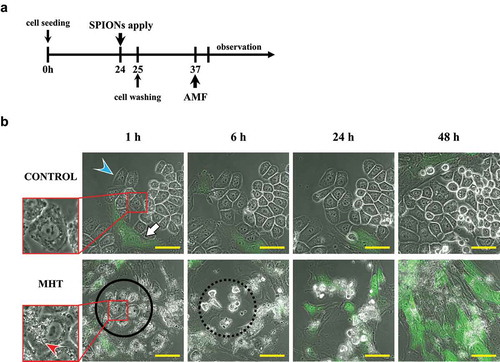
Discussion
In the present study, we demonstrate the new concept of immuno-hyperthermia whereby MNPs with a specific tumor-targeting antibody directed to individual cells with over-expressed HER2, showed high targeting-specificity. SPIONs as MNPs, were used for their high heating capacity and safety [Citation14]. SPION-alone treatment did not decrease viability of any cell type tested, indicating their nontoxicity. Trastuzumab is an antibody with high binding affinity to HER2 [Citation15]. We bound trastuzumab to the outer surface (–COOH) of the SPIONs using EDC/NHS coupling. The resultant anti-HER2 SPIONs selectively targeted HER2-positive cancer cells when administrated in vitro, and were internalized inside the cells as early as 12 hours after administration, as shown in . Non-conjugated SPIONs did not targeted HER2-positive cancer cells and were not internalized. Anti-HER2 SPIONs alone were not cytotoxic.
In the present study, we proved the novel concept of immuno-hyperthermia. Future studies will use other cancer-specific antibodies bound to SPIONs to demonstrate the generality of the new concept of immuno-hyperthermia. Our previous study showed SPION-based hyperthermia was effective against disseminated gastric cancer in a mouse model [Citation5]. Future studies will test immuno-SPIONs in vivo as a bridge to the clinic.
Acknowledgments
We thank Dr. Takeshi Nagasaka for helpful discussions, and Tae Yamanishi and Tomoko Sueishi for their excellent technical support.
Disclosure statement
No potential conflict of interest was reported by the author(s).
Correction Statement
This article has been republished with minor changes. These changes do not impact the academic content of the article.
Additional information
Funding
References
- Roussakow S. The history of hyperthermia rise and decline. Conference of the International Clinical Hyperthermia Society 2012, Budapest, Hungary. Conference Papers in Medicine 2013; 2013:1–40.
- Gilchrist RK, Medal R, Shorey WD, et al. Selective inductive heating of lymph nodes. Ann Surg. 1957;146(4):596–606.
- Liu X, Zhang Y, Wang Y, et al. Comprehensive understanding of magnetic hyperthermia for improving antitumor therapeutic efficacy. Theranostics. 2020;10(8):3793–3815.
- Maier-Hauff K, Rothe R, Scholz R, et al. Intracranial thermotherapy using magnetic nanoparticles combined with external beam radiotherapy: results of a feasibility study on patients with glioblastoma multiforme. JNeurooncol. 2007;81(1):53–60.
- Matsumi Y, Kagawa T, Yano S, et al. Hyperthermia generated by magnetic nanoparticles for effective treatment of disseminated peritoneal cancer in an orthotopic nude-mouse model. Cell Cycle. 2021;20(12):112–1133.
- Kishimoto H, Urata Y, Tanaka N, et al. Selective metastatic tumor labeling with green fluorescent protein and killing by systemic administration of telomerase-dependent adenoviruses. Mol Cancer Ther. 2009;8(11):3001–3008.
- Bouvet M, Tsuji K, Yang M, et al. In vivo color-coded imaging of the interaction of colon cancer cells and splenocytes in the formation of liver metastases. Cancer Res. 2006;66(23):11293–11297.
- Kishimoto H, Aki R, Urata Y, et al. Tumor-selective,adenoviral-mediated GFP genetic labeling of human cancer in the live mouse reports future recurrence after resection. Cell Cycle. 2011;10(16):2737–2741.
- Noma K, Smalley KS, Lioni M, et al. The essential role of fibroblasts in esophageal squamous cell carcinoma-induced angiogenesis. Gastroenterology. 2008;134(7):1981–1993.
- Watanabe S, Noma K, Ohara T, et al. Photoimmunother-apy for cancer-associated fibroblasts targeting fibroblast activation protein in human esophageal squamous cell carcinoma. Cancer Biol Ther. 2019;20(9):1234–1248.
- Grabarek Z, Gergely J. Zero-length crosslinking procedure with the use of active esters. Anal Biochem. 1990;185(1):131–135.
- Goldenberg MM. Trastuzumab, a recombinant DNA-derived humanized monoclonal antibody, a novel agent for the treatment of metastatic breast cancer. Clin Ther. 1999;21(2):309–318.
- Yamane M, Moriya S, Kokuba H. Visualization of ceramide channels in lysosomes following endogenous palmitoyl-ceramide accumulation as an initial step in the induction of necrosis. Biochem Biophys Rep. 2017;11:174–181.
- Banobre-Lopez M, Teijeiro A, Rivas J. Magnetic nanoparticle-based hyperthermia for cancer treatment. Rep Pract Oncol Radiother. 2013;18(6):397–400.
- Vogel CL, Cobleigh MA, Tripathy D, et al. Efficacy and safety of trastuzumab as a single agent in first-line treatment of HER2-overexpressing metastatic breast cancer. Jclin oncol. 2002;20(3):719–726.