ABSTRACT
Increasing proofs have declared that liver cancer stem cells (CSCs) are the main contributors to tumor initiation, metastasis, therapy resistance, and recurrence of hepatocellular carcinoma (HCC). However, the molecular mechanisms underlying CSCs regulation remain largely unclear. Recently, PCNA-associated factor (PAF) was identified to play a key role in maintaining breast cancer cell stemness, but its role in liver cancer stem cells has not been declared yet. Herein, we found that both mRNA and protein expression levels of PAF were significantly higher in HCC tissues and cell lines than normal controls. CSC-enriched hepatoma spheres displayed an increase in PAF expression compared to monolayer-cultured cells. Both loss-of-function and gain-of-function experiments revealed that PAF enhanced sphere formation and the percentage of CD133+ or EpCAM+ cells in HCCLM3 and Huh7 cells. In the xenograft HCC tumor model, tumor initiation rates and tumor growth were suppressed by knockdown of PAF. Mechanistically, PAF can amplify the self-renewal of liver CSCs by activating β-catenin signaling. Taken together, our results demonstrate that PAF plays a crucial role in maintaining the hepatoma cell stemness by β-catenin signaling.
Abbreviations: CSCs: cancer stem cells; HCC: hepatocellular carcinoma; PAF: pCNA-associated factor.
1. Introduction
Hepatocellular carcinoma (HCC), comprising over than 70% of primary liver cancer cases, has a high fatality rate [Citation1–3]. In spite of the rapid progresses that have been achieved in HCC detection and treatment, the overall survival rate is still poor due to frequent recurrence and therapy resistance [Citation4].
A great number of studies have declared that a small cluster of cells with increasing capabilities of tumor-initiating and self-renewal, known as cancer stem cells (CSCs), located in various kinds of tumor including hematologic [Citation5], brain [Citation6], breast [Citation7], prostate [Citation8], liver [Citation9], pancreas [Citation10] and colon cancer [Citation11]. These cells are considered to be the primary cause of metastasis, relapse, and therapy resistance of HCC [Citation12,Citation13]. Based on the differentiation stage, liver CSCs express specific markers, such as epithelial cell adhesion molecule (EpCAM), cluster of differentiation 133 (CD133), CD44, CD13 and CD24 [Citation14–17]. Therefore, liver CSCs are becoming attractive targets for liver cancer therapy and prevention strategies. Kaori et al. recently reported that engineered biological nanoparticles containing small interfering RNA targeting β-catenin significantly reduced the tumor growth rate by suppressing the proliferation of hepatic cancer stem cells [Citation18]. However, because the mechanisms underlying maintaining the self-renewal of liver CSCs are poorly understood, therapeutic strategies directly targeting stem cells in HCC are few until now.
PAF (also known as KIAA0101 and OEATC-1) is defined as a proliferating cell nuclear antigen (PCNA)–interacting protein [Citation19]. PAF participates in an extensive range of biological activities like DNA replication and cell cycle progression by interacting with PCNA [Citation20]. Overexpression of PAF was closely related with tumor development and weak prognosis in a broad spectrum of human cancers, which acted as an oncogene by enhancing cancer cell proliferation, migration and invasion [Citation21–24]. A recent study has reported that PAF exerts functions in maintaining the stemness properties of breast cancer cell by positively modulating Wnt signaling [Citation25]. Nevertheless, the exact role of PAF in liver CSCs remains largely unknown.
The present study aimed to determine whether PAF regulates the stemness of hepatocellular carcinoma cells. Our results demonstrated that PAF mRNA and protein expression were both up-regulated in HCC tumors and liver CSCs. To evaluate the effects of changes in PAF expression on the stemness of CSCs, we constructed lentiviral vectors able to inhibit or increase PAF gene expression in HCC cell lines. By knockdown or overexpression of PAF, our results revealed that PAF enhanced the self-renewal ability in liver CSCs via activating β-catenin signaling.
2. Materials and methods
2.1 HCC specimens
HCC and normal control liver tissues were acquired from 20 HCC patients who accepted surgery at Shaanxi Provincial Cancer Hospital, from 2010 to 2016. After histopathology confirmation, these tissues were stored at −80°C for further use. This study was authorized by the Ethics Committee of Shaanxi Provincial Cancer Hospital, and all patients or their guardians signed the informed consent form.
2.2 Cells
The human HCC cell lines: HCCLM3, PLC/PRF/5, Huh7, and Hep3B, and normal control cell line THLE-2 were purchased from Cell Bank of Type Culture Collection of the Chinese Academy of Sciences (Shanghai Institute of Cell Biology), and maintained in high-glucose Dulbecco’s modified Eagle’s medium (DMEM, Gibco-BRL, USA) including 10% fetal bovine serum (AccuRef Scientific, Xi’an, China) at 37°C in an atmosphere containing 5% CO2.
2.3 Plasmid construction, lentivirus production, and siRNA transfection
The CDS sequence of PAF was generated by PCR and inserted into pcDNA3.1 vector (Invitrogen) to construct PAF overexpression vector. HCCLM3 and Huh7 cells with 60% confluence were transfected with lentivirus-PAF-shRNA or lentivirus- PAF-shNC for 24 h and then selected for a week in a medium supplemented with puromycin (Sigma, USA) to obtain stable expression cells. Small interfering RNA (siRNA) for silencing β-catenin was purchased from GenePharma (Shanghai, China). The sequence was as follows: si-β-catenin sense: 5ʹ-UGGUUGCCUUGCUCAACAA-3ʹ; si-β-catenin anti-sense: 5ʹ-ACCAA CGGAACGAGUUGUU-3ʹ; si-NC sense: 5ʹ-UUCUCCGAACGUGUCACGUUU-3ʹ; si-NC anti-sense, 5ʹ-ACGUGACACGUUCGGAGAAUU-3ʹ.
2.4 RNA extraction and qRT-PCR
Total RNAs were separated from HCC cells or tissues using Trizol reagent (Invitrogen, USA) and reverse transcription reaction was conducted by using Super M-MLV reverse transcriptase (Tiangen, Beijing, China) and random primers. The BestarTM Real-time PCR Master Mix was used for quantitative PCR analysis using the SYER Green Method. β-actin was used as the internal control. The primers were obtained from Sangon Biotech and listed as following: PAF, 5ʹ-ATGGTGCGGACTAAAGCAGAC-3ʹ (forward) and 5ʹ-CCTCGATGAAACTGATGTCGAAT-3ʹ (reverse); β-actin, 5ʹ-GGGACCTGACTGACTACCTC-3ʹ (forward) and 5ʹ-TCATACTCCTGCTTGCTGAT-3ʹ (reverse).
2.5 Western blot analysis
The levels of protein expression were analyzed by western-blot analysis as previous described [Citation26]. Briefly, proteins were collected from cells or tissues using RIPA (AccuRef Scientific). After quantification by the BCA Protein Assay kit (EXPrecision, Xi’an, China), equal amounts of protein were isolated by 12% SDS-PAGE gels, transferred to the PVDF membrane. The PVDF membranes were cut into small pieces containing target protein bands as designated by a protein ladder (AccuRef Scientific). After blocking with 1% BSA (Sigma-Aldrich) for 1 h at room temperature, the membranes were incubated with anti-PAF (1:2000; ab226255; Abcam), anti-t-Akt (1:500; ab8805; Abcam), anti-p-Akt (1:500; ab38449; Abcam), antip-GSK3β (1:500; ab68476; Abcam), GSK-3β (1:1,000; ab32391; Abcam), anti-β-catenin (1:5000; ab32572; Abcam), or anti-β-actin (1:5,000; ab179467; Abcam), respectively, overnight at 4°C. Following incubating with horseradish peroxidase-labeled secondary antibodies at room temperature for 1 h, the blots were visualized by chemiluminescent gel imaging system of ChemiDoc XR (Bio-RAD). β-actin was acted as an internal control.
2.6 Spheroid formation assay
HCC cell lines HCCLM3 and Huh7 at a density of 1x103/ml were seeded in an ultra-low attachment 6-well plate (Corning Incorporated, USA) including 2% B27 (Invitrogen), 20 ng/ml basic fibroblast growth factor (bFGF, Sigma), and 20 ng/ml rhEGF (PeproTech, USA). After 7 days, the tumor spheres with a diameter larger than 50 μm were photographed and calculated.
2.7 Flow cytometry
HCCLM3 and Huh7 cells washing with PBS containing 2% fetal bovine serum were centrifuged at 1000 rpm for 5 min. Then, cell suspension with a concentration of 107 cells/mL was incubated with APC-conjugated-EpCAM antibody or PE-conjug-CD133 antibody (BD Biosciences, San Diego, CA, United States) for 30 min at 4°C. IgG antibody labeled with APC or PE was served as isotype control. Following that, the proportions of CD133+ or EpCAM+ HCC cells were analyzed on a Becton-Dickinson FACS Calibur flow cytometer.
2.8 Experiment animal models
To evaluate the tumor formation ability, cells were mixed with Matrigel (Becton Dickinson) at a volume ratio of 1:1 and injected subcutaneously into NOD/SCID mice (6-week-old, Shanghai, China) with indicated number. The mice were sacrificed 7-week post-inoculation, and the incidence of tumor was recorded.
To observe whether PAF promoted sphere cell growth in vivo, ten of mice were randomly divided into two groups with Huh7 sh-NC or Huh7 sh-PAF sphere cell injection subcutaneously. After that, the mice were measured every 3 days and tumor volumes were calculated by V = (a2 × b)/2. The mice were sacrificed on day 40 after first injection. The xenograft tissues were then removed and weighed. All experiments using animals were obeyed the guidelines of the animal care and use committees of Shaanxi Provincial Cancer Hospital.
2.9 Statistics analysis
All results were displayed as mean ± SEM and analyzed using Prism 6.0 software. Significant between two-group differences was evaluated by Student’s t-test. When comparing the differences more than two groups, one-way ANOVA was performed. The significance was set as p < 0.05.
3. Results
3.1 PAF was up-regulated in HCC tissues and cells
The level of PAF in 20 HCC tumor specimens and adjacent control specimens was measured by qRT-PCR analysis. Our result demonstrates that the mRNA level of PAF is remarkably increased in HCC tissues compared to controls (). Western blot results also revealed that PAF protein level was significantly up-regulated in four cases of HCC tumor tissues compared with the relevant normal controls (). Moreover, the expression of PAF in normal control cell THLE-2 and HCC cell lines was evaluated by qRT-PCR and western blot analysis. A notable elevation of PAF level was observed in Huh7, HCCLM3, Hep3B and PLC/PRF/5 cells () by comparison with THLE-2 cells,
Figure 1. PAF expression is up-regulated in HCC samples and cell lines
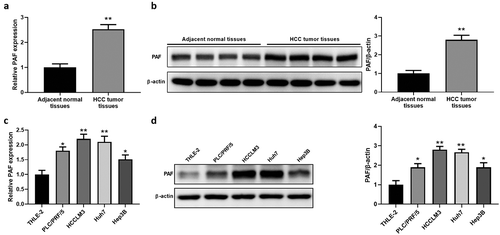
3.2 PAF expression was elevated in liver CSCs
Next, we cultured spheres of Huh7 and HCCLM3, and detected the expression of stemness markers of hepatoma spheres. The representative photos of spheres from two HCC cell lines are shown in . CD133 and EpCAM), the two markers of stemness, were significantly higher in spheroids generated from HCCLM3 and HUH7 cells compared to adherent states (), suggesting that sphere culture could enrich liver CSCs. We further detected PAF expression in spheroids from HCC cells. We found that both mRNA and protein levels of PAF were markedly elevated in spheres in contrast to adherent cells (), indicating that PAF might play a role in liver CSCs.
Figure 2. PAF expression levels are elevated in spheres derived from HCC cell lines
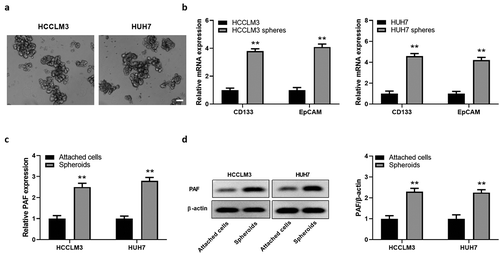
3.3 PAF enhanced the self-renewal ability of liver CSCs
To further determine the function of PAF in CSCs regulation, HCCLM3 and HUH7 cells were transfected with sh-PAF to knock down their expression. The sh-PAF stable transfectants were established, as verified by examining PAF mRNA and protein levels (). Compared with control, depletion of PAF resulted in an obvious decrease in the number of spheroids (). FCM results displayed a reduced percentage of CD133+ or EpCAM+ cells (which represented CSCs) in PAF-deleted HCCLM3 and Huh7 cells (). Next, we established HCC cell lines over-expressing PAF by transfecting PAF-expression vector into HCCLM3 and Huh7 cells, which is confirmed by measuring PAF expression with qRT-PCR and western-blot analysis (). HCC cells overexpressing PAF caused an obvious increase in the number of spheroids and percentage of liver CSCs by comparison with control cells (). These data revealed that PAF may be involved in HCC development via enhancing the self-renewal ability of CSCs.
Figure 3. PAF knockdown significantly impairs self-renewal ability of liver CSCs
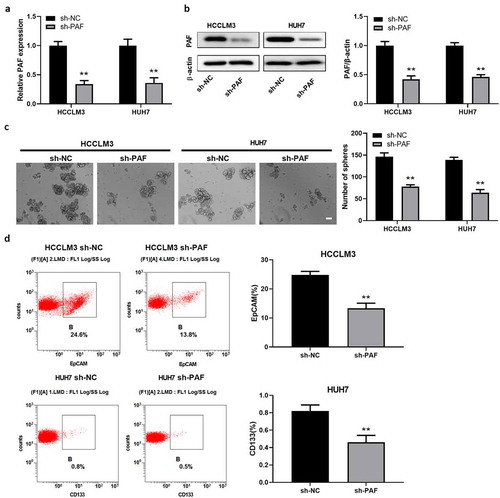
Figure 4. PAF over-expression promotes sphere formation of HCC cells
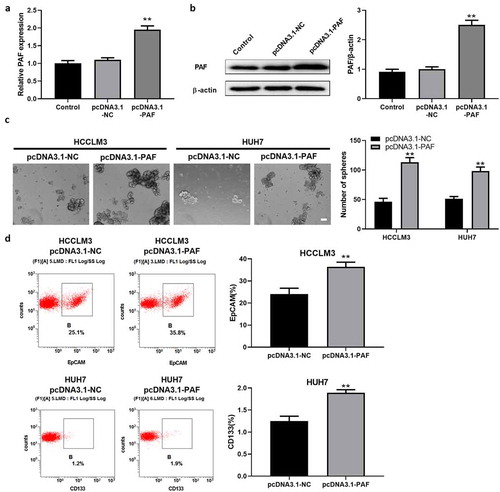
3.4 PAF promoted tumor initiation and growth in vivo
One of the key features of CSCs is their tumor formation capability. To further test the effect of PAF on tumorigenicity, Huh7 cells expressing sh-PAF or sh-NC were injected into NOD-SCID mice subcutaneously followed by evaluating tumor occurrence after inoculation. We found that Huh7 cells expressing sh-PAF displayed lower tumor incidences than control cells, whether mice were inoculated with 1 × 103, 1 × 104, or 1 × 105 cells (). Next, the action of PAF on sphere cell growth in vivo was evaluated by implanting the Huh7 sphere cells expressing sh-PAF into NOD-SCID mice. The representative photos of xenografts are shown in . PAF deletion significantly reduced the average tumor volume and weight of xenografts (). QRT-PCR analysis revealed that PAF level in xenografts was much lower in sh-PAF group compared to control group ().
Table 1. Tumor initiation of HUH7 cells in NOD-SCID mice
Figure 5. PAF knockdown significantly represses the tumor formation and growth of HCC in vivo. NOD-SCID mice were subcutaneously injected with Huh7 sh-PAF or control sphere cells (1 × 104)
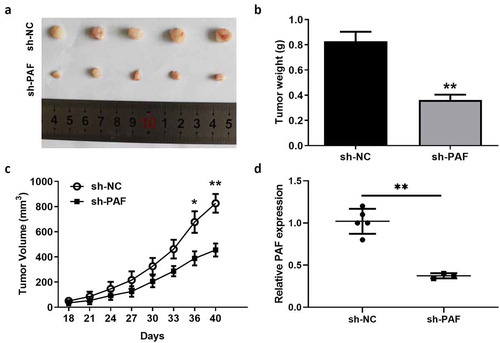
3.5 The enhancement of self-renewal ability in liver CSCs was β-catenin dependent
It has been reported that PAF activates Wnt/β-catenin signaling to maintain the stemness of breast cancer; thus, we detected β-catenin level in liver CSCs. We found that PAF ablation significantly reduced the expression of β-catenin in spheroids from HCCLM3 and Huh7 cells (). Moreover, depletion of β-catenin by specific siRNA abolished the difference in the number of spheroids between sh-PAF transfected HCCLM3 and Huh7 cells and their corresponding control cells (). In accordance with the above observation, we found that the discrepancy in the proportion of liver CSCs between sh-PAF transfected HCC cells and control cells was also eliminated (). Therefore, these findings suggested that PAF could regulate self-renewal of liver CSCs via a β-catenin dependent way.
Figure 6. PAF facilitates self-renewal ability of liver CSCs via activating β-catenin
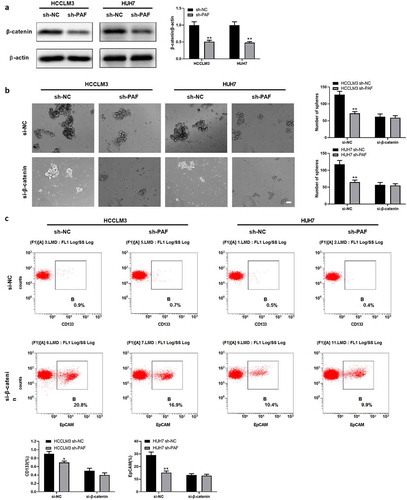
3.6 PAF promotes the expression of β-catenin via activation of Akt/GSK3β signaling
Previous study had reported that Akt signaling was involved in the regulation of β-catenin [Citation27]. To investigate the potential molecular mechanism by which PAF is able to modulate β-catenin signaling, we performed western blot assay to detect the levels of Akt and its downstream protein GSK3β. As shown in , depletion of PAF significantly decreased the phosphorylated Akt and phosphorylated GSK3β, while total Akt and total GSK3β remained unchanged when compared with negative control. As expected, the downregulation of PAF also decreased the protein level of β-catenin (). Taken together, these data suggested that PAF modulated the expression of β-catenin by regulating Akt/GSK3β signaling.
Figure 7. Downregulation of PAF deactivates Akt/GSK3β signaling
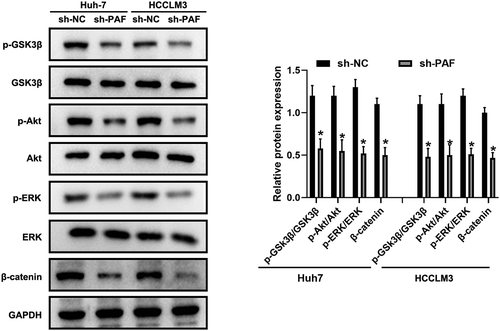
4. Discussion
Hepatocellular carcinoma (HCC) is the most common type of primary liver cancer. Of all cancers, HCC ranks the second most lethal tumor worldwide. However, with the advances in methods of diagnosis and treatment, the survival rate of HCC patients is poor. Hepatocellular carcinoma with a high rate of recurrence and heterogeneity comprised heterogeneous cell populations [Citation28,Citation29], among which cancer stem cells (CSCs) are key drive force for tumorigenesis, chemo-resistance, and recurrence of HCC [Citation30]. HCC patients with higher numbers of CSCs predicted poorer outcomes. Thus, the discovery of a novel molecular modulating stemness of CSCs could contribute to the advancement of HCC treatment. In the present study, we demonstrated that PAF exerted important functions in liver CSCs self-renewal and could be a novel target in HCC therapy.
PAF was initially identified as a PCNA-associated factor by using a yeast two-hybrid screen system [Citation20], which acted as an oncogene in several types of tumors including hepatocellular carcinoma. In this study, PAF levels in HCC tissues and cells were measured. Both mRNA and protein expression of PAF were notably up-regulated, which was consistent with the previous studies. Due to the key role of CSCs in tumor development and recurrence, we explored the effect of PAF on liver CSCs. CD133 and EpCAM are two cell surface markers expressed in CSCs that modulate stem cell properties in liver CSCs. Using two HCC cell lines, HCCLM3 and Huh7, we set up suspension spheroid culture condition and detected the expression of CD133 and EpCAM. Our results showed that the level of CD133 and EpCAM were both higher in spheroids compared to adherent states, verifying that liver CSCs were enriched by spheroid culture of cancer cells. Moreover, we observed that PAF mRNA and protein expression were obviously higher in spheroids. These findings indicated that PAF might be involved in liver cancer stem cell regulation.
PAF has been reported to play a crucial role in maintaining the stemness features of breast cancer cells via regulating cell plasticity [Citation25]. However, its function in liver CSCs is largely obscure. In the present study, through knockdown or overexpression of PAF, we demonstrated that PAF increased spheroid formation and the percentage of CD133+ or EpCAM+ hepatoma cells. Xenograft model further supported that PAF promoted sphere propagation in vivo. These data indicate that PAF is needed for liver CSC expansion by enhancing liver CSCs self-renewal and may provide a hopeful target for HCC therapy.
Wnt/β-catenin was identified as an important signaling pathway to regulate self-renewal of liver CSCs in human HCC. It has been reported that anti-miR-181 inhibitors could inhibit stemness-related gene level and tumorigenicity in EpCAM+ HCC via blocking Wnt/β-catenin pathway [Citation31]. To explore the downstream signaling pathway underlying the modulation of CSCs by PAF, we assessed the level of β-catenin in hepatoma spheroids. Consistent with previous studies, we found that PAF upregulated β-catenin expression, thus resulting in the enhanced self-renewal of liver CSCs. Numerous studies have reported that the activation of Akt signaling plays a critical role in the pathogenesis of hepatic cancer [Citation32–34]. Activation of Akt suppresses GSK3β by phosphorylation at Ser9 [Citation35], resulting the increment of β-catenin [Citation36]. Using RNA interference, we found that downregulation of PAF significantly reduced phosphorylated Akt and phosphorylated GSK3β, by which the expression of β-catenin was inhibited (), indicating Akt signaling is potentially involved in the PAF-regulated β-catenin expression. Consistently, Liu et al. found that Akt activation promotes the expression of β-catenin, resulting in the progression of hepatocellular carcinoma [Citation37]. A recent study demonstrated that PAF was significantly increased in the high-grade serous ovarian cancer and silencing PAF could markedly decrease the phosphorylation of PI3K, AKT, and mTOR and promoting cisplatin sensitivity of ovarian cancer cells [Citation38]. This evidence suggests that PAF might regulate the GSK3β/β-catenin pathway in HCC via Akt activation.
In conclusion, the findings of our study demonstrated that it is a novel regulator of PAF. PAF levels were obviously increased in HCC tumors and liver CSCs. In addition, PAF overexpression enhanced liver CSC properties via activating β-catenin signaling. Therefore, targeting PAF could be a hopeful strategy for CSC therapy in HCC.
Acknowledge
Not applicable
Data availability
The datasets used and/or analyzed during the current study are available from the corresponding authors on reasonable requests.
Authors’ contributions
LM and LZB conceived and designed the experiments. MXD, SJR and ZPT performed the experiments. CY and LY analyzed and interpreted the data. MXD wrote the manuscript. LM and LZB revised the manuscript. All authors read and approved the final manuscript.
Ethics approval and informed consent
Written informed consent was obtained from all patients and the study protocol was approved by the Ethics Committee of Shaanxi Provincial Cancer Hospital. Animal care and study were approved by the Institutional Animal Care and Use Committee of Shaanxi Provincial Cancer Hospital.
Patient consent for publication
Not applicable.
Disclosure statement
No potential conflict of interest was reported by the authors.
Additional information
Funding
References
- Bray F, Ferlay J, Soerjomataram I, et al. Global cancer statistics 2018: GLOBOCAN estimates of incidence and mortality worldwide for 36 cancers in 185 countries. CA Cancer J Clin. 2018;68:394–424.
- Ferlay J, Soerjomataram I, Dikshit R, et al. Cancer incidence and mortality worldwide: sources, methods and major patterns in GLOBOCAN 2012. Int J Cancer. 2015;136:E359–E86.
- Mittal S, El-Serag HB. Epidemiology of hepatocellular carcinoma: consider the population. J Clin Gastroenterol. 2013;47(Suppl):S2–S6.
- M C, Terashita K, Sakamoto N, et al. New molecularly targeted therapies against advanced hepatocellular carcinoma: from molecular pathogenesis to clinical trials and future directions. Hepatol Res. 2015;45:E1–E11.
- Lapidot TSC, Vormoor J, Murdoch B, et al. A cell initiating human acute myeloid leukaemia after transplantation into SCID mice. Nature. 1994;367:645–648.
- Singh SKCI, Terasaki M, Bonn VE, et al. Identification of a cancer stem cell in human brain tumors. Cancer Res. 2003;63:5821–5828.
- Al-Hajj MWM, Benito-Hernandez A, Morrison SJ, et al. Prospective identification of tumorigenic breast cancer cells. Proc Natl Acad Sci USA. 2003;100::3983–8.
- Collins ATBP, Hyde C, Stower MJ, et al. Prospective identification of tumorigenic prostate cancer stem cells. Cancer Res. 2005;65:10946–10951.
- Dalerba PCR, Clarke MF. Cancer stem cells: models and concepts. Annu Rev Med. 2007;58:267–284.
- Wicha MSLS, Dontu G, Dontu G. Cancer stem cells: an old idea—a paradigm shift. Cancer Res. 2006;66(4):1883–1890.
- Dalerba PDS, Park IK, Liu R, et al. Phenotypic characterization of human colorectal cancer stem cells. Proc Natl Acad Sci USA. 2007;104:10158–10163.
- Choi K-JB IH, Ye S-K, Lee Y-H. Molecular targeted therapy for hepatocellular carcinoma: present status and future directions. Biol Pharm Bull. 2015;38:986–991.
- Oishi N, Wang XW. Novel therapeutic strategies for targeting liver cancer stem cells. Int J Biol Sci. 2011;7:517–535.
- Haraguchi N, Ishii H, Mimori K, et al. CD13 is a therapeutic target in human liver cancer stem cells. J Clin Invest. 2010;120:3326–3339.
- Lee TKW, Castilho A, Cheung VCH, et al. CD24(+) liver tumor-initiating cells drive self-renewal and tumor initiation through STAT3-mediated NANOG regulation. Cell Stem Cell. 2011;9:50–63.
- Ma S, Chan K-W, Hu L, et al. Identification and characterization of tumorigenic liver cancer stem/progenitor cells. Gastroenterology. 2007;132:2542–2556.
- Zhao W, Wang L, Han H, et al. 1B50-1, a mAb raised against recurrent tumor cells, targets liver tumor-initiating cells by binding to the calcium channel α2δ1 subunit. Cancer Cell. 2013;23:541–556.
- Ishiguro KYI, Lewis-Tuffin L, Patel T. Targeting liver cancer stem cells using engineered biological nanoparticles for the treatment of hepatocellular cancer. Hepatol Commun. 2020;4:298–313.
- Karg ESM, Ryan J, Forné I, et al. Ubiquitome analysis reveals PCNA-associated factor 15 (PAF15) as a specific ubiquitination target of UHRF1 in embryonic stem cells. J Mol Biol. 2007;429:3814–3824.
- De Biasio A, De Opakua AI, Mortuza GB, et al. Structure of p15(PAF)-PCNA complex and implications for clamp sliding during DNA replication and repair. Nat Commun. 2015;6:6439.
- Kais Z, Barsky SH, Mathsyaraja H, et al. KIAA0101 interacts with BRCA1 and regulates centrosome number. Mol Cancer Res. 2011;9:1091–1099.
- Cheng Y, Li K, Diao D, et al. Expression of KIAA0101 protein is associated with poor survival of esophageal cancer patients and resistance to cisplatin treatment in vitro. Lab Invest. 2013;93:1276–1287.
- Yuan R-H, Jeng Y-M, Pan H-W, et al. Overexpression of KIAA0101 predicts high stage, early tumor recurrence, and poor prognosis of hepatocellular carcinoma. Clin Cancer Res. 2007;13:5368–5376.
- Kato T, Daigo Y, Aragaki M, et al. Overexpression of KIAA0101 predicts poor prognosis in primary lung cancer patients. Lung Cancer. 2012;75:110–118.
- Wang XJY, Jun S, Lee S, et al. PAF-Wnt signaling-induced cell plasticity is required for maintenance of breast cancer cell stemness. Nat Commun. 2016;7:10633.
- Zhuo Z, Hu J, Yang X, et al. Ailanthone inhibits Huh7 cancer cell growth via cell cycle arrest and apoptosis in vitro and in vivo. Sci Rep. 2015;5:16185.
- Duda P, Akula SM, Abrams SL, et al. Targeting GSK3 and associated signaling pathways involved in cancer. Cells. 2020;9:1110.
- Magee JA, Piskounova E, Morrison SJ. Cancer stem cells: impact, heterogeneity, and uncertainty. Cancer Cell. 2012;21:283–296.
- Shackleton MQE, Fearon ER, Morrison SJ. Heterogeneity in cancer: cancer stem cells versus clonal evolution. Cell. 2009;138:822–829.
- Visvader JELG, Lindeman GJ. Cancer stem cells in solid tumours: accumulating evidence and unresolved questions. Nat Rev Cancer. 2008;8:755–768.
- Ji JYT, Budhu A, Forgues M, et al. Identification of microRNA-181 by genome-wide screening as a critical player in EpCAM-positive hepatic cancer stem cells. Hepatology. 2009;50:472–480.
- Zhuang L, Wang X, Wang Z, et al. MicroRNA-23b functions as an oncogene and activates AKT/GSK3β/β-catenin signaling by targeting ST7L in hepatocellular carcinoma. Cell Death Dis. 2017;8:e2804.
- Xie Y, Du J, Liu Z, et al. MiR-6875-3p promotes the proliferation, invasion and metastasis of hepatocellular carcinoma via BTG2/FAK/Akt pathway. J Exp Clin Cancer Res. 2019;38:7.
- Kahraman DC, Kahraman T, Cetin-Atalay R. Targeting PI3K/Akt/mTOR pathway identifies differential expression and functional role of IL8 in liver cancer stem cell enrichment. Mol Cancer Ther. 2019;18:2146–2157.
- Krishnankutty A, Kimura T, Saito T, et al. In vivo regulation of glycogen synthase kinase 3β activity in neurons and brains. Sci Rep. 2017;7:8602.
- Liu X, Yao Z. Chronic over-nutrition and dysregulation of GSK3 in diseases. Nutr Metab (Lond). 2016;13:49.
- Liu G, Ouyang X, Sun Y, et al. The miR-92a-2-5p in exosomes from macrophages increases liver cancer cells invasion via altering the AR/PHLPP/p-AKT/β-catenin signaling. Cell Death Differ. 2020;27:3258–3272.
- Jin C, Liu Z, Li Y, et al. PCNA-associated factor P15(PAF), targeted by FOXM1, predicts poor prognosis in high-grade serous ovarian cancer patients. Int J Cancer. 2018;143:2973–2984.