ABSTRACT
As the main exchanger of electroneutral NaCl absorption, sodium-hydrogen exchanger isoform 3 (NHE3) circulates in the epithelial brush border (BB) and intracellular compartments in a multi-protein complex. The size of the NHE3 complex changes during rapid regulation events. Recycling regulation of NHE3 in epithelial cells can be roughly divided into three stages. First, when stimulated by Ca2+, cGMP, and cAMP-dependent signaling pathways, NHE3 is converted from an immobile complex found at the apical microvilli (MV) into an easily internalized and mobile form that relocates to a compartment near the base of the MV. Second, NHE3 is internalized by clathrin and albumin-dependent pathways into cytoplasmic endosomal compartments, where the complex is reprocessed and reassembled. Finally, NHE3 is translocated from the recycling endosomes (REs) to the apex of epithelial cells, a process that can be stimulated by an increase in sodium-glucose cotransporter 1 (SGLT1) activity, epidermal growth factor receptor (EGFR) signaling, Ca2+ signaling, and binding to βPix and SH3 and multiple ankyrin repeat domains 2 (Shank2) proteins. This review describes the molecular steps and protein interactions involved in the recycling movement of NHE3 from the apex of epithelial cells, into vesicles, where it is reprocessed and reassembled, and returned to its original location on the plasma membrane, where it exerts its physiological function.
Introduction
Sodium-hydrogen exchanger isoform 3 (NHE3, also known as SLC9A3), one of the nine subtypes of the Na+/H+ exchanger (NHE) in mammals, is part of the main pathway for the Na+ absorption and NaHCO3 reabsorption in the intestinal and kidney brush border (BB) epithelial cells. NHE3 plays a key role in the absorption of salt and liquid, and pH stability, and is highly expressed in the apical membrane of the small intestine, colon and proximal tubules of the kidney [Citation1,Citation2]. Under physiological conditions, NHE3 exchanges intracellular H+ and extracellular Na+ at a 1:1 ratio of electrical neutrality for reverse transport and transmembrane exchange, maintaining the dynamic balance of intracellular pH1. In addition, NHE3, is combined with a Cl−/base exchanger and a Cl–formate or Cl–oxalate exchanger to exert its salt reabsorption function [Citation3], which accounts for approximately 50% of the glomerular filtrate reabsorbed by NaCl and 70% reabsorbed by NaHCO3 in the proximal tubule [Citation4]. In addition to its role in Na+ and water absorption, NHE3 also regulates the absorption of other nutrients, such as dipeptides and amino acids, through H+-gradients [Citation5].
Long term downregulation of NHE3 leads to a variety of pathophysiological conditions [Citation6,Citation7]. Research has shown that Nhe3−/−mice had intestinal and kidney absorption defects, mild acidosis, and shortened lifespan [Citation8]. In addition, the activity of NHE3 is closely related to the development of diarrhea [Citation9–11]. Studies on NHE3 function and/or expression changes in patients with ulcerative colitis indicated that decreased NHE3 activity in the inflammatory mucosa might be the basis of ulcerative colitis diarrhea. A lack of NHE3 might be a key factor in immune regulation disorders, such as inflammatory bowel disease (IBD), loss of barrier function, and biological dysfunction, for example, in spontaneous colitis accompanied by mild diarrhea, occasional rectal prolapse, and weight loss in Nhe3 KO mice [Citation12]. Therefore, it is of great importance to determine the mechanism of NHE3 regulation associated with intestinal infection and inflammation, and water and salt absorption disorders in the gastrointestinal system and renal system.
The acute regulatory activity of brush border NHE3 contributes to the gastrointestinal and renal system water and Na+ homeostasis by changing the level of plasma membrane NHE3 via the rate of endocytosis and regulation of exocytosis [Citation13]. Paradoxically, NHE3, which can perform the physiological function of sodium-hydrogen exchange, is fixed on the MV actin skeleton, with weak mobility. This fixation requires of NHE3 to combine with multiple PDZ domain scaffold protein Na+/H+ exchanger regulatory factors (NHERFs, including NHERF1, NHERF2, NHERF3, and NHERF4) and ezrin [Citation14]. NHE3 is regulated by a large number of second messengers, including cAMP-dependent protein kinase (PKA), cGMP-dependent protein kinase II (cGKII), intracellular calcium ([Ca2+]i), and protein kinase C alpha (PKCα) at the molecular and cellular level [Citation15]. The regulation of NHE3 can occur through a variety of mechanisms, including vesicle endocytosis mediated by clathrin and albumin [Citation16,Citation17], intracellular protein transport, interactions with auxiliary regulatory proteins, and direct protein phosphorylation [Citation18]. Although these regulatory processes are delicate and intricate, the recycling regulation of NHE3 is systematic, coherent, and complete. However, the importance and role of each molecular mechanism in regulating the activity of NHE3 has proven to be difficult to determine accurately. Therefore, this review provides a systematic description of the regulation of NHE3 activity, integrating the conclusions from on multiple previous studies related to the regulation of NHE3 activity.
NHE3 and its binding partners
NHE3 consists of two domains, a relatively conservative NH2 terminus (~450 aa) and a decentralized COOH terminus (~400 aa). The N-terminus of NHE3 contains 13 transmembrane domains (), which carries out the Na and H exchange, The C-terminus regulates the transport rate [Citation1,Citation19].Almost all the rapid regulation of NHE3 activity requires the intracellular C-terminus (455–832 aa) [Citation20]. Therefore, we discuss the C-terminus in detail in this review (). The NHE3 C-terminus can be divided into four regions: (I) C-terminus domain 1 (F1 domain, 455–585 aa): There are two inosine phosphate binding regions (Tyr501–Arg512 and Arg520–Arg552) in the F1 domain, which are important for serum stimulation. Mutations in these regions alter the binding and basic NHE3 activity of phosphatidyl diphosphate PI (4,5) P2 and phosphatidyl triphosphate PI (3,4,5) P3. The latter alters the surface expression of NHE3, while the former does not [Citation21]. In addition, there is a predicted helix structure between residues 511 and 528 [Citation21,Citation22]. On one side of the α helix, there is a cluster of three positive amino acids (K516, R520, and R527), including a conserved triserine cluster (Ser515, Ser522 and Ser526), which has a high isoelectric point (pI = 12.1). Point mutations of these positively charged amino acids eliminated Ezrin binding, which is a member of the Ezrin-radixin-moesin (ERM) protein family, connecting NHE3 to the cytoskeleton, and reduced the basic activity and surface of NHE3 [Citation23]. The significant changes in phosphorylation of NHE3 at serine 552 and 605 were related to protein kinase A (PKA) activation, but did not directly affect the activity of NHE3 [Citation18]. NHE3-Ser526 was predicted to be the phosphorylation site of serum/glucocorticoid-induced kinase 3 (GSK-3) [Citation21,Citation24]. (II) C-terminal domain 2 (F2 domain, 586–660 aa): Based on in vitro interactions, NHE3 binds NHERFs through internal PDZ domain binding motifs (589–660 aa) [Citation25]. In mouse intestines and kidneys, cAMP acts by phosphorylating NHE3 primarily at two amino acids: Ser552 and Ser605 (in rabbit they are Ser554 and Ser607) [Citation18,Citation26,Citation27]. Other consensus sequences for cAMP in NHE3 have been reported, including Ser330, Ser514, Ser576, Ser662, Ser691, Ser692, and Ser805; however, NHE3 does not require NHE3-ser663 phosphorylation [Citation18,Citation27]. The phosphorylation of Ser552 and Ser605 sites in NHE3 precede the inhibition of NHE3 activity by PKA [Citation18], because mutation of Ser552 or Ser605 eliminated the inhibition of NHE3 by 8-Br-cAMP activated by PKA [Citation18,Citation28]. Calmodulin kinase II (CaMKII) is associated with the C-terminal 586–605 aa of NHE3 in a Ca2+-dependent manner, and CaMKII requires 690 amino acids downstream of NHE3 to inhibit NHE3 phosphorylation and NHE3-related basic activities, while mutations in three recognized phosphorylation sites downstream of amino acid 690 prevented KN-93 (a CaMKII-inhibitor) from stimulating NHE3 activity [Citation29]. (III) C-terminal domain 3 (F3 domain, 661–756 aa): Previously, residue S663 was proven to be necessary for acute stimulation of NHE3 by serum and glucocorticoid-inducible kinase (SGK) and glucocorticoid. Then, it was found that SGK1 could phosphorylate NHE3 at Ser665. Mutation of Ser665 blocked glucocorticoid regulation of NHE3. SGK1 phosphorylation regulates the activity of NHE3 by acidifying NHE3 via a large phosphate side chain-induced allosteric displacement [Citation26]. Tandem liquid chromatography (LC-MS/MS) of trypsinized NHE3 identified the phosphorylation site of casein kinase 2 (CK2) at the C-terminal S719 residue using an in vitro kinase assay. CK2 binds and phosphorylates the S719 site of the NHE3 C-terminus, and then stimulates the basic NHE3 activity of the C-terminus, thus affecting the transport of NHE3 [Citation30]. (IV) C-terminal domain 4 (F4 domain, 757–832 aa): The interaction between NHE3 and megalin occurs in the MV gap, in which megalin-bound NHE3 is inactive [Citation31]. By contrast, the association between NHE3 and dipeptidyl peptidase 4 (DPPIV) occurs mainly in the MV region, where NHE3 is active [Citation31]. However, Kocisky et al. showed that there was a time separation between the phosphorylation and activity of NHE3, suggesting that phosphorylation might not directly affect the transport activity of NHE3 [Citation18].
Figure 1. Simulation of NHE3 and its binding partners. Amino acids 1–477 of NHE3 (AAB48990.1) were subjected to prediction online using PHYRE2 (http://www.sbg.bio.ic.ac.uk/phyre2/phyre2_output/6a92509e13ede827/summary.html). Amino acids 1–459 are the N-terminus NHE3, of which aa 1–15 form the signal peptide and aa 56–459 form the 13 transmembrane structure. Phyre2 predicted that 1–459aa belongs the NH2 terminal of NHE3, in which 1–15aa was the signal peptide and 56–459aa was its 13 transmembrane structures.Y56-H73aa, P83-W100aa, V114-Y129aa, T142-I167aa, F181-V196aa,E216-E232aa,K249-S273aa, E286-E302aa, I306-K321aa M339-S357aa, A369-I392aa, V407-V423aa and F437-I459aa belonges S1, S2, S3, S4, S5, S6, S7, S8, S9,S10,S11,S12, S13 transmembrane region, respectively. Its C-terminus can be divided into four domains: (a) The F1 domain (455–585 aa), in which aa 475 is the CHP binding site, and aa 526 is the SGK3 binding site. Amino acids 511–528 form the site where Ezrin binds directly to NHE3, and aa 552 is the PKA/cAMP/cGMP binding site; (b) The F2 domain 5 (86–660 aa): aa 586–605 are the CaM II/CaMK II binding site, and aa 605 is the PKA/cAMP/cGMP binding site; (c) The F3 domain (661–756 aa): aa 663 is the SGK binding site, aa 665 is the SGK1 binding site, and aa 719 is the CK2 binding site. The NHERFs (i.e. NHERF1, NHERF2, NHERF3, and NHERF4) binding sites spans the F2 and F3 domains (585–689 aa); (d) The F4 domain (757–834 aa), which contains the protein phosphatase 2A (PP2A) and megalin binding sites; however, their precise locations are unknown
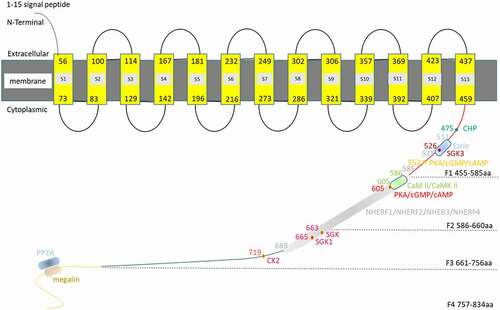
The recycling regulation of hydrogen and sodium exchange of NHE3
Many membrane proteins, including transporters (such as NHE3), are involved in a recycling mechanism between intracellular compartments, including transgolgi, endosomes, and lysosomes in epithelial cells [Citation32]. In fact, 70% of NHE3 subgroups seem to be tied to the cytoskeleton, resulting in limited movement and an inability to participate in endocytosis. Only those proteins that can diffuse into the pits covered by inclusion proteins can be internalized [Citation33]. Generally, NHE3 recycles between the epithelial BB and intracellular compartments in a multi-protein complex, and the size of the complex changes with the rapid regulation of binding proteins, over a life span of about 16 h [Citation22,Citation34,Citation35]. Recycling regulation of NHE3 in epithelial cells can be roughly divided into three stages: (I) When stimulated by Ca2+, cGMP, and cAMP-dependent signaling pathways, NHE3 is converted from an immobile complex found at the apical MV into an easily internalized and mobile form that relocates to a compartment near the base of the intermicrovillar space; (II) NHE3 is internalized by clathrin and albumin-dependent pathways into cytoplasmic endosomal compartments, where the complex is reprocessed and reassembled [Citation36]; (III) NHE3 is translocated from the RE back to the apex of the plasma membrane, a process that can be stimulated by an increase in SGLT1 activity, EGFR signaling, Ca2+ signaling, and the binding to βPix (also known as ARHGEF7 (Rho guanine nucleotide exchange factor 7)) and SH3 and multiple ankyrin repeat domains 2 (Shank2) proteins, interacting with the actin cytoskeleton and retaining almost immobile subgroups on the plasma membrane in a manner dependent on the persistent activity of Rho GTPases.
Transfer of NHE3 from the apical membrane toward the intermicrovillar space
Normally, NHE3 is anchored on the actin scaffold, where it exchanges hydrogen and sodium with an immobile complex on the epithelial BB. Only when it transfers to the intermicrovillar space can it be internalized. McDonough et al. have shown that NHE3 retracts from MV to the microvillar base in a two-step process in intact proximal tubules and then enters a denser membrane pool, which seems to be a reproducible storage pool [Citation37,Citation38]. Circulating cAMP, cGMP, elevated [Ca2+]i, as well as neurohumoral substances and bacterial toxins that cause increases in these second messengers, inhibit the activity of NHE3, thus triggering the transformation of NHE3 from an almost immobile complex retained on the apical membrane, in a manner dependent on the persistent activity of Rho GTPases, to an internalized and mobile NHE3 subgroup on the apical membrane, which stimulates NHE3 (). Meanwhile, cAMP, and cGMP can also stimulate the secretion of Cl−, increase the level of [Ca2+]i, and inhibit the activity of NHE3 [Citation39]. Phosphorylated NHE3 forms membrane complexes and interacts with Ezrin, NHERFs, and actin to sense and maintain a constant direction during cell migration.
Figure 2. Transfer of NHE3 from the apical membrane toward the intermicrovillar space. (a) Elevated [Ca2+]i inhibits NHE3 activity. Carbachol (CCH) raises [Ca2+]i through the basolateral membrane (BLM) muscarinic receptor (M3), and the resulting activated protein kinase C alpha (PKCα) moves to the top of the plasma membrane. Elevated [Ca2+]i oligomerizes through the formation of the NHE3-NHERF2-actin-4-PKCα complex, resulting in endocytosis of the plasma membrane-located NHE3, thereby inhibiting NHE3 activity; (b) Inhibition of NHE3 activity mediated by cGMP. Upon activation of guanylate cyclase, both guanylin and heat-stable enterotoxins (STa) bind to the same BB receptor, guanine cyclase-C, resulting in a rapid increase in intestinal cGMP levels. This activates BB cGKII, which passes its guanyl moiety N-terminally to the plasma membrane and the second PDZ domain of C-terminal NHERF2, to increase NHE3 phosphorylation at three sites (rabbit Ser554, Ser607, Ser663, equivalent to mouse Ser552, Ser605, Ser659), resulting in rapid inhibition of NHE3 activity; (c) cAMP-mediated inhibition of NHE3. Dopamine (DA), parathyroid hormone (PTH), vasoactive intestinal peptide, secretin, and enterotoxins, such as cholera toxin, activate adenylate cyclase, which can rapidly increase the level of intestinal cAMP, resulting phosphorylation of NHE3 at Ser552 and Ser605, which then forms a complex containing NHE3, NHERF1/2, Ezrin, F-actin, and PKAII (cAMP-dependent protein kinase type II), thereby inhibiting the activity of NHE3
![Figure 2. Transfer of NHE3 from the apical membrane toward the intermicrovillar space. (a) Elevated [Ca2+]i inhibits NHE3 activity. Carbachol (CCH) raises [Ca2+]i through the basolateral membrane (BLM) muscarinic receptor (M3), and the resulting activated protein kinase C alpha (PKCα) moves to the top of the plasma membrane. Elevated [Ca2+]i oligomerizes through the formation of the NHE3-NHERF2-actin-4-PKCα complex, resulting in endocytosis of the plasma membrane-located NHE3, thereby inhibiting NHE3 activity; (b) Inhibition of NHE3 activity mediated by cGMP. Upon activation of guanylate cyclase, both guanylin and heat-stable enterotoxins (STa) bind to the same BB receptor, guanine cyclase-C, resulting in a rapid increase in intestinal cGMP levels. This activates BB cGKII, which passes its guanyl moiety N-terminally to the plasma membrane and the second PDZ domain of C-terminal NHERF2, to increase NHE3 phosphorylation at three sites (rabbit Ser554, Ser607, Ser663, equivalent to mouse Ser552, Ser605, Ser659), resulting in rapid inhibition of NHE3 activity; (c) cAMP-mediated inhibition of NHE3. Dopamine (DA), parathyroid hormone (PTH), vasoactive intestinal peptide, secretin, and enterotoxins, such as cholera toxin, activate adenylate cyclase, which can rapidly increase the level of intestinal cAMP, resulting phosphorylation of NHE3 at Ser552 and Ser605, which then forms a complex containing NHE3, NHERF1/2, Ezrin, F-actin, and PKAII (cAMP-dependent protein kinase type II), thereby inhibiting the activity of NHE3](/cms/asset/db3c09dd-eaf0-4544-946e-dc46ac41eb9d/kccy_a_2005274_f0002_oc.jpg)
[Ca2+]i regulates the phosphorylation of NHE3 activity, and the formation of multiprotein complexes
Carbachol (CCH) treatment of the intact rabbit ileum caused the rapid translocation of phospholipase C-γ (PLC-γ) to the apical membrane [Citation40], resulting in activated PKCα moving to the apical plasma membrane to increase [Ca2+]i through a muscarinic receptor (M3) in the basolateral membrane (BLM). This decreased the surface expression and activity of NHE3, and increased the volume of the NHE3 multiprotein complex [Citation41], which inhibited NHE3 activity by 40%, and stimulated the endocytosis of NHE3. In addition, serotonin (5-HT), heat-stable Escherichia coli (STa) toxin B, and the rotavirus enterotoxin NSP5 directly upregulate [Ca2+]I, together with an ionophore. Previous studies have shown that [Ca2+]i elevation in an NHERF2 (not-NHERF1)-dependent manner inhibits the activity of NHE3 at the apical plasma membrane [Citation42]. NHERF2-NHE3 regulation involves the formation of polyprotein complexes (including NHE3, NHERF2, α-actinin-4, and PKCα). Thus, elevated [Ca2+]i inhibits NHE3 activity by forming an oligomeric NHE3- NHERF2-actin-4-PKCα complex, resulting in endocytosis of NHE3 into the intermicrovillar space [Citation42–44]. Interestingly, overexpression of α-actin-4 can enhance the inhibition of NHE3 activity by accelerating the oligomerization and endocytosis of NHE3. However, overexpression of the actin-binding domain plus spectrin repeat domain acted as a dominant negative mutation, which prevented the inhibition of NHE3 activity by a calcium ionophore and the oligomerization and endocytosis of NHE3 [Citation42]. At the same time, elevated [Ca2+]i decreases the intracellular NHE3-NHERF4 linkages to reduce NHE3-dependent NHERF4 targeting to the apex of the plasma membrane, and released NHERF3 from NHE3, resulting in decreased activity and surface expression of NHE3 [Citation45]. It should be noted that the [Ca2+]i-dependent inhibition mediated by NHE3 and NHERF2 has the opposite effect on the interaction between NHE3 and inositol 1,4,5-triphosphate (IP3) receptor-binding protein (IRBIT), which enhances the translocation of NHE3 to the surface membrane through a mechanism involving calmodulin-dependent protein (CaM) and CaMKII [Citation46].
Inhibition of NHE3 Activity Mediated by cGMP
After activation of guanylate cyclase, or the binding of guanylin and STa to the same BB receptor, guanosine cyclase-C, the intestinal cGMP level increases rapidly, and inhibits the absorption of NaCl by NHE3. The effect of cGMP on NHE3 seems to occur entirely through activation of cGKII in the BB [Citation47]. The inhibition of NHE3 involves a complex, NHE3-NHERF2-cGKII/cGMP/cGKII, which increases the phosphorylation of NHE3 at three sites (rabbit Ser554, Ser607, Ser663, equivalent to mouse Ser552, Ser605, Ser659) and rapidly inhibits NHE3, resulting in a decrease in its surface expression. To inhibit NHE3, the N-terminus of myristoylated cGKII is attached to the plasma membrane, and its C-terminus is bound to NHERF2 (via PDZ domain 2) to allow cyclic GMP to inhibit NHE3, which effect involves the complex of NHE3- NHERF2-cGKII [Citation48]. Both cGMP and cGKII must be present simultaneously to enable cGMP to inhibit NHE3 [Citation27]. Even if cGKII was co-expressed, it did not inhibit the stable transfection of NHE3 in PS120 cells. As Donowitz’s research showed, most cell culture models (including PS120 cells and opossum kidney (OK) cells) lack cGKII, but have NHERF2, and thus cannot inhibit the activity of NHE3, showing that the presence of cGKII, rather than its absence, could inhibit NHE3 activity acutely. In addition, NHERF2 (but not NHERF1) PDZ2 C terminal binding to cGKII was identified under in vitro conditions. Although cGKII also binds NHERF2 in vitro, it will not cause NHE3 inhibition unless the myristoylation site is present, which is necessary for plasma membrane binding; the non-myristoylation mutant of cGKII does not support cGMP inhibition of NHE3 [Citation49]. Guanylated cGKI can also reconstruct the inhibition of cGMP on NHE3, probably because NHERF2 is a specific cGMP kinase anchoring protein (GKAP) that binds to GKI and cGKII [Citation48]. Neither cyclic cGKI nor cGKII lacking the myristoylation site could reconstitute cGMP inhibition of NHE3. In contrast, a chimera of wild-type cGKI and the first 29 amino acids of cGKII, which was myristoylated and thus bound to the plasma membrane, did reconstitute cGMP inhibition of NHE3. Thus, cGKII must be myristoylated and attached to the plasma membrane and bound to NHERF2 to inhibit NHE3 [Citation49]. Therefore, cGMP’s inhibition of NHE3 expression in fibroblasts and polarized epithelial cells requires both NHERF2 and cGKII. When NHERF2 was replaced by NHERF1, cGMP did not change the activity of NHE3 [Citation49,Citation50], indicating the strict requirement for NHERF2. The specific ligand of cGMP kinase also inhibits NHE3; therefore, a specific inhibitor of cGMP kinase blocked the effect of NHE3. In addition, because guanylate cyclase C binds to NHERF4, larger complexes in the BB might be involved in signal complexes associated with cGMP inhibition of NHE3.
cAMP-mediated inhibition of NHE3
Hormones (such as vasoactive intestinal peptide and secretin) and enterotoxins (such as cholera toxin) activate adenylate cyclase to rapidly increase intestinal cAMP levels, which mediates the inhibition of NHE3 at Ser552 and Ser605 to decrease plasma membrane NHE3 levels, seemingly through another phosphorylation process involving PKA, which stimulates endocytosis [Citation18]. Mutation of Ser605, the main target of PKA in NHE3, reduced the acute inhibition by cAMP by 50%[Citation51]. In fibroblasts and polarized proximal tubular OK cell lines, cAMP inhibits NHE3 in the presence of NHERF1 or NHERF2, which involves the PDZ domain of NHERF1 or NHERF2 [Citation52–54]. cAMP inhibition regulation includes the complex of NHE3, NHERF1 or NHERF2, Ezrin, F-actin, and protein kinase II [Citation29,Citation55]. Specifically, Ezrin located in F-actin binds to both NHERF1/NHERF2 and PKAII (cAMP-dependent protein kinase type II), positioning PKAII so it can phosphorylate NHE3, thus inhibiting its activity [Citation55,Citation56]. Therefore, the phosphorylation of NHE3 could directly inhibit its transport rate and also act as a signal for its endocytosis [Citation3]. Kinetic analysis of NHE3 inhibition mediated by cAMP in OK cells showed that the number of transporters in the plasma membrane remained unchanged during the initial stage of cAMP-elevation [Citation57], although a decrease was observed at subsequent time points [Citation58].
In addition, Moe et al. found that parathyroid hormone (PTH) and Dopamine (DA) inhibited NHE3 activity in OK cells [Citation59]. The short-term acute inhibition by PTH on NHE3 activity was not related to the change of abundance of NHE3 on the membrane; however, its long-term regulation of NHE3, which requires PKA-mediated C-terminal specific serine phosphorylation, decreased the amount of NHE3 [Citation55]. DA significantly inhibits the activity of sodium and hydrogen exchange by reducing the surface abundance of NHE3, a process that also involves the phosphorylation of PKA-mediated transporters [Citation58].
NHE3 enters the cytoplasm through internalization from the intermicrovillar space
Generally, NHE3 circulates between the plasma membrane and the intracellular compartments, which is regulated by changes in endocytosis and exocytosis rates or both to balance the amount of NHE3 on the surface of the plasma membrane [Citation60,Citation61]. Most basic NHE3 endocytosis occurs through clathrin and albumin-dependent processes, partially involving lipid rafts (e.g. CCH-mediated Ca2+ dependent NHE3 internalization) [Citation34,Citation62] ().
Figure 3. NHE3 enters the cytoplasm through internalization from the intermicrovillar space. (a) Endocytosis of NHE3 mediated by clathrin (CME). Synaptotagmin I (Syt I) binds to NHE3 in the intestinal epithelium and initiates the assembly of clathrin required for endocytosis through the recruitment of AP2 and clathrin, mediating endocytosis induced by cAMP (mediated by NHERF1) and Ca2+ (mediated by NHERF2). In addition, DA phosphorylates NHE3 (Ser605) mediated by PKA, leading to NHE3 endocytosis through reticulin-mediated vesicles; (b) Albumin mediated NHE3 endocytosis. Albumin-dependent NHE3 endocytosis requires the scavenger receptor megalin to bind to albumin in the intravillar cleft to form a large protein complex, which is then regulated by many protein complexes of transmembrane proteins and auxiliary proteins (e.g. scaffold proteins NHERF1 and NHERF2). The type 2 PDZ binding domain of NHERF1 binds directly to C-terminus of megalin and NHE3. NHERF2 nucleates endocytotic complexes in villi by interacting with NHE3 and CLC-5; (c) Reprocessing, assembly, and cycling of NHE3 in the cytoplasm. The PDZ domain of SNX27 binds VPS26 of the retromer complex (including VPS26-VPS29-VPS35 and regulates NHE3 exocytosis from early endosomes (EEs) to the plasma membrane
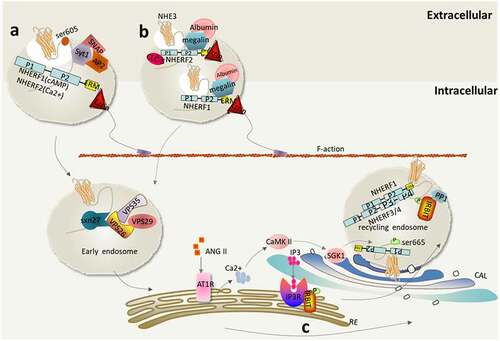
Endocytosis of NHE3 via clathrin-mediated endocytosis
Clathrin-mediated endocytosis (CME) is the most distinctive endocytosis mechanism, and is the main way for epithelial cells to absorb macromolecules [Citation63,Citation64]. NHE3 is internalized in a manner similar to the multiple receptors of receptor-mediated, endocytosis via clathrin-mediated pits and vesicles, which are then transported to early endosomes (EEs) through endocytotic vesicles, involving a coatomer protein complex subunit epsilon (ε-COP)-dependent step [Citation61,Citation63]. The internalization pathway of the NHE3-clathrin-dependent complex mainly involves the following proteins: (I) Synaptotagmin I (Syt I), a Ca2+ binding protein, which is expressed in the apex and subapical areas of the intestinal epithelial cells, with its highest expression in villous epithelial cells and the surface of crypt epithelial cells in the intestine. Syt I is a pivotal mediator of Ca2+ and cAMP-induced membrane endocytosis of NHE3, and is a member of the large class of Syt proteins that are involved in synaptic vesicle fusion as well as the delivery of vesicles from the Golgi to the synaptic bulb [Citation65]. The endocytosis process of NHE3 by Syt I involves many different transport proteins, such as vesicle-associated membrane proteins, synaptic fusion proteins (syntaxin), S-nitroso-N-acetyl penicillamine (SNAP), and adaptor protein 2 (AP2) [Citation66,Citation67]. Mechanistically, Syt I binds to intestinal epithelial NHE3 and activates the assembly of clathrin required for endocytosis through the recruitment of AP2 and clathrin [Citation65], mediating the endocytosis induced by cAMP and Ca2+ [Citation58,Citation65]. It should be noted that NHERF1 mediates cAMP regulation [Citation68], while NHERF2 mediates Ca2+ regulation of NHE3 [Citation42]. The binding and internalization of NHE3 serine 605 (S605) and Syt I were identified using fluorescence resonance energy transfer (FRET) analysis and NHE3 site directed mutation. (II) DA, a key hormone for sodium balance in mammals, significantly reduces the amount of surface NHE3 and inhibits the exchange activity of Na+/H+ at the apex of proximal tubules [Citation69], mainly through PKA-dependent and PKA-independent mechanisms involving DR1-like receptors, one of the molecular subtypes of the DA receptor, which results in NHE3 endocytosis via clathrin-coated vesicles [Citation58,Citation70]. In addition, studies have shown that DA-stimulated NHE3 endocytosis can be blocked by drugs, genetic inhibition of PKA, or mutation of two PKA-binding residues (Ser-560 and Ser-613) on NHE3. Another important process of endocytosis mediated by clathrin in the early stage is the pH homeostasis and intracellular fusion events in the endosomal compartment, which might affect ligand-receptor separation, vesicle transport, endosomal fusion events, recycling to the plasma membrane, and the formation of outer membrane proteins. However, pH homeostasis is partly maintained by vacuolar H+-ATPase and NHE3 [Citation71].
NHE3-dependent albumin endocytosis
NHE3-dependent albumin endocytosis requires macrophage scavenger receptors to bind to albumin in the intravillar cleft to form a large protein complex containing many transmembrane proteins and accessory proteins. This complex may include accessory proteins, such as the type I PDZ motif (S/T-X-Φ) of the scaffold proteins NHERF1 and NHERF2, which is then subjected to endocytosis [Citation17,Citation62,Citation72]. Previous studies have shown that the interaction between NHERF scaffolds and members of the albumin endocytosis complex is as follows: Firstly, megalin is important in regulating albumin uptake, especially its ability to translocate albumin into Villar fissures [Citation73]; Secondly, the PDZ binding motif of megalin at its C-terminus has been proven to bind proteins containing MAGI-1 and postsynaptic density (PSD)-95 PDZ domains [Citation74], such that the effect of NHERF1 may be achieved through a direct link with scavenger receptors. Fortunately, Slattery et al. found that NHERF1 directly bound to NHE3 and the C-terminus of megalin through the type 2 PDZ binding domain of NHERF1, without any auxiliary proteins, i.e. NHE3 was bound to this region [Citation17]. In contrast, NHERF2 nucleates endocytic complexes in the villi through an interaction with NHE3 and chloride voltage-gated channel 5 (CLC-5), to stimulate the endocytosis of NHE3 [Citation73]. Silencing NHERF1 increased the endocytosis of albumin, probably because the absence of NHERF1 increased the mobility of NHE3 in the MV, thereby increasing the formation of endocytosis complexes in the villous fissure. Silencing NHERF2 reduced albumin endocytosis [Citation62], presumably by increasing and reducing CLC-5 cell surface availability, respectively.
Endocytosis mediated by cell division cycle 42 involves lipid rafts independent of clathrin
Nearly half of surface NHE3 is located in lipid rafts, and the activity and transport of NHE3 depends on these lipid rafts [Citation60], which might be very important for the timing of membrane signaling, transport, and transport activities of NHE3. It is worth noting that the endocytosis of NHE3 mediated by CCH occurs via lipid rafts, activated by the cell division cycle 42 (Cdc42)-dependent pathway, which does not involve clathrin. Cdc42 is necessary for a clathrin-independent endocytic process, termed the clathrin Independent Carrier (CLIC)/GPI anchored protein-enriched Early Endocytic Compartment (GEEC) pathway [Citation75,Citation76]. Research shows that NHE3 binds to NHERF3 in the plasma membrane, and is then internalized to a compartment near the base of the MV after CCH treatment, while NHERF3 remains in the plasma membrane [Citation77].
Reprocessing, assembly, and cycling of NHE3 in the cytoplasm
By comparing the size of the surface and endosomal compartment-located NHE3, it was found that the NHE3 complex in the plasma membrane was larger than that in the cell, suggesting that not all the complexes are formed in the ER or GOLGI, and it could be assumed that NHE3 is associated with several different proteins as it passes between the BB and the recovered endosome. Therefore, the complex containing NHE3 is dynamic (). During the cyclic processing of receptors and ligands, internalized receptors and ligands either enter REs for reprocessing or are transported into late endosomes and then degraded by lysosomes. An important process of the endocytosis pathway is acidification of the endosomal compartments [Citation64,Citation78].
SNX27 is necessary for the transfer of NHE3 from early endosomes to the extracellular region
Sorting nexin 27 (SNX27), a member of the sorting nexin family, contains a PDZ domain that has high homology with the sequence of the NHERF family’s PDZ domain, which is necessary for the transfer of NHE3 from the EE to extracellular region of epithelial cells. The multimeric complex of NHE3 regulates its activity by interacting with PDZ domain proteins associated with NHERF proteins [Citation20,Citation79]; therefore, it is possible that other proteins also interact with the SNX27-NHE3 complex to regulate the transport of NHE3 in vivo. NHE3 mainly interacts with the SNX27 N-terminal PDZ domain, which lies upstream of the PX domain, unique in SNX27 [Citation80,Citation81]. In addition, SNX27 is annotated as having a Ras-association domain and an extended C-terminal domain [Citation82,Citation83]. SNX27 was initially thought to mediate intracellular transport to late endosomes leading to degradation [Citation84,Citation85]. Then, later research suggested that SNX27 is more likely to mediate the transport of Rab5 to Rab11-positive vesicles, while it remains in Rab5-positive EE vesicles [Citation86]. Singh et al. have revealed the mechanism of SNX27’s regulation of NHE3: SNX27 binds to and regulates the exocytosis of NHE3 from EEs to plasma membranes, and plays an important role in regulating the transport of multiple protein membranes in the EEs, which occurs through interaction with a retromer complex [Citation35]. The retromer complex comprises a vacuolar protein sorting (VPS) trimer core subcomplex (VPS26-VPS29-VPS35) and the membrane-related sorting nexin (SNX) dimer (SNX1-SNX2) [Citation87]. In this process, SNX27 binds VPS26 directly via SNX27’s PDZ domain, which enhances serine phosphorylation of the SNX27 PDZ domain, inhibits the binding of SNX27 to NHE3, and reduces the extracellular secretion of NHE3 to the plasma membrane. In summary, the PDZ adapter protein SNX27 and other nucleosomes may appear along the endosomal-recycling pathway [Citation88]. In addition, the retention of NHE3 on plasma membrane requires SNX27 regulation, and deletion of SNX27 reduced the apical localization and basic activity of NHE3 in intestinal epithelial cells. On the other hand, NHE3 and NHERF3 and/or NHERF4 were co-localized in Rab11 positive RE vesicles, and NHE3-NHERF3 translocated from this chamber to the plasma membrane after the Ca2+ concentration increased, while NHERF4 remained in the Rab11 vesicles, indicating the motivational role of Rab11-positive REs in the regulation of NHE3 [Citation45,Citation77,Citation88].
Transcriptional regulation of NHE3: glucocorticoid/ SGK1
Most NHE3 regulation is the acute regulation that occurs within a few minutes to a few hours after activation, which is rapid and reversible, often involving changes in phosphorylation, transport, and dynamic interactions with regulatory proteins. By contrast, the chronic regulation of NHE3 involves the transcription and translation modification of NHE3. In short, the regulation of NHE3 is complex, with myriad signals converging on a single protein at different levels. A major example of the complex and integrated regulation of NHE3 is the regulation of glucocorticoids, which increase the level of NHE3 mRNA and often affect NHE3 via multiple pathways, a process that requires SGK1 as a key kinase targeting the S663 site of NHE3 [Citation89–92]. In vitro, after activation by serum glucocorticoids, SGK1 induced the translation activity of NHE3 requiring NHERF2 (not NHERF1) [Citation93], and NHERF2 interacts with actin via ezrin [Citation94], causing the translocation of NHE3 to the cell surface [Citation91]. These findings are supported in mice lacking SGK1 expression: Glucocorticoid-mediated stimulation activity and translocation of NHE3 to the apical membrane of mice was significantly reduced [Citation95]. The upregulation of NHE3 function mediated by SGK1 also includes phosphatidylinositol 3 (PI3) kinase, which is known to affect the surface expression of NHE3 [Citation92].
NHE3 translocation from recycling endosomes (REs) to the apex of epithelial cells
There are several types of apical membranes containing NHE3 in epithelial cells, including NHE3 in the lipid raft and outside the lipid raft on the plasma membrane. NHE3 binds to megalin (denser, less active) but not to megalin (lighter, more active). Intracellular NHE3 also exists in two pools, one of which is rapidly discharged, while the other moves to the apical membrane with slower kinetics [Citation33,Citation36]. When stimulated by certain signals, such as increased SGLT1 activity, EGFR signaling, elevated Ca2+ concentration, and binding to βPix & Shank2 proteins, NHE3 will be translocated from the REs back to the apex of the plasma membrane ().
Figure 4. NHE3 translocation from REs to the apical region of epithelial cells. This mainly includes the following activation mechanisms: (a) The SGLT1/P38AMP/MAPMAPK2/PI3K/AKT2/Ezrin pathway activates NHE3; (b) IRBIT mediates the activation of NHE3 by ANG II via a Ca2+/calmodulin-dependent protein kinase II-dependent pathway; (c) Regulation of NHE3 by PLCs; (d) Regulation of NHE3 by the (2AR)/LPA5/EGFR/RSK2 pathway;(e) βPix upregulates NHE3 through protein–protein interaction mediated by Shank2
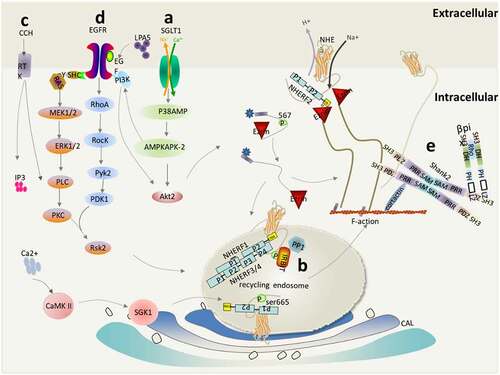
The SGLT1/P38AMP/MAPKAPK2/PI3K/AKT2/Ezrin pathway activates NHE3
Glucose transport triggered by SGLT1 will lead to the activation of the apical hydrogen-sodium exchanger NHE3 [Citation96]: Na+-glucose co-transport mediated by SGLT1 activates phosphatidylinositol 3-kinase (PI3K), Serine/Threonine Kinase 2 (AKT2) and NHERF2-dependent signaling pathways via p38 mitogen-activated protein kinase (p38AMPK)/MAP kinase-activated protein kinase 2 (MAPKAPK2) sequentially to stimulate NHE3 activity, and finally AKT2 phosphorylates Ezrin [Citation2]. PI3K is a cytoplasmic signaling molecule that is recruited to the activated receptor under stimulation, which leads to an increase in the intracellular 3-phosphoinositide phospholipid level and induces a signal response, including AKT2 activation [Citation94]. AKT2 phosphorylates Ezrin to trigger the translocation and activation of NHE3 after the initiation of Na-glucose transport [Citation97]. Ezrin connects with the actin cytoskeleton in MVs and is necessary to regulate the rate of endocytosis of NHE3 and changes to stimulated extracellular proliferation [Citation97]. In addition to directly binding to NHE3, Ezrin interacts with the regulatory subunit p85 of PI3K at two different sites, one is the N-terminal amino acid 1–309, and the other is its C-terminal phosphorylated tyr353 Binding of residues to the SH2 [Citation98]. In addition, Ezrin regulates NHE3 activation through a Rho-dependent mechanism. The Rho-dependent formation of an MV-like structure requires the ERM protein (standing for Ezrin, radixin, and moesin), which is controlled by the small GTPase Rho and phosphorylated by RhoK on a C-terminal threonine to interfere with their head-tail interaction [Citation98]. Therefore, the lateral migration of NHE3 regulated by Ezrin activation on the apical plasma membrane is Rho/RhoA- RhoA-Rho associated kinase (ROCK)-dependent (not shown in the picture) rather than PI3K/AKT2-dependent [Citation23]. In addition, the N-terminal region binding sites between Rho-GDI and NHERF1 proteins have been identified [Citation99].
IRBIT/PLCs mediate the activation of NHE3 by ANG II via a CaMKII-dependent pathway
The interaction between NHE3 and inositol 1,4,5-triphosphate (IP3) receptor binding protein (IRBIT) is opposite to the [Ca2+]i-dependent inhibition mediated by the combination of NHE3 and NHERF2. IRBIT, dependent on CaM and CaMK II, mediates the activation of NHE3 by ANG II and enhances the translocation of NHE3 to the surface membrane [Citation46]. IRBIT binds to the carboxyl terminal region of NHE3, which is necessary for the acute regulation of NHE3, responding to the increase in Ca2+ induced by thapsigargin or ionomycin in PS120 fibroblasts and activating NHE3 [Citation46]. In addition, extracellular stimulation, including hormones, growth factors, and neurotransmitters activate receptor tyrosine kinase (RTK), and then activate PLCs (PLCβ and PLCγ) through their phospholipase catalytic domains. The activated PLCs hydrolyze the membrane and bind phosphatidylinositol 4,5-bisphosphate (PIP2) to produce two second messengers: Diacylglycerol (DAG) and IP3. The former is an activator of PKCs, and the activated PKCα translocates to the BB [Citation100]; while the IP3 binds to the IP3 receptor (IP3R) to induce a transient increase in Ca2+ through its release from storage in the endoplasmic reticulum [Citation101–104]. At the same time, the PLCγ lipase-dependent increased in Ca2+ also activates the calmodulin/calmodulin kinase II (CaM/CaMKII) complex.
CaMKII is a multifunctional kinase that is involved in many biological processes regulated by [Ca2+]i, including synaptic transmission, gene transcription, and channel regulation [Citation105]. Reversal of phosphorylation indicated that NHE3 was phosphorylated by CaMKII under basic conditions, suggesting that CaMKII is a novel NHE3 binding protein, and that this association was reduced by [Ca2+]i elevation, which occurs in fibroblasts and the BB [Citation106]. The angiotensin II (ANG II) system, which is mainly involved in regulating blood pressure and fluid balance, has been confirmed to stimulate the activity of NHE3 in renal proximal tubular epithelial cells [Citation107]. The regulation of NHE3 activity by ANG II is mediated by activation of the G protein-dependent angiotensin II type I receptor (AT1R)/PLC/calcium/calmodulin pathway: ANG II activates and phosphorylates CaMKII directly or indirectly through AT1R and the CaM complex, inducing increased [Ca2+]i to stimulate NHE3 activity. Ang II (1 nm) did not change the total expression of NHE3, but induced CaMKII to stimulate NHE3 phosphorylation and CD40 receptor associated factor 1 (CRAF-1)/mitogen-activated protein kinase (MEK)/ERK1/2 to stimulate 90 KDa ribosomal protein S6 kinase 1 (p90RSK) activity [Citation108]. In addition, ANG II stimulates NHE3 activity by increasing the extracellular insertion of NHE3 in a phosphoinositide 3-kinase (PI3K)-dependent manner [Citation109]. Recently, Peijian et al. found that ANG II induced an increased interaction among NHERF1, NHE3, and IRBIT, and they were co-expressed in the same macro-complex. NHERF1 was essential for the positive RE transport and activation of ANG II-mediated NHE3 in cultured cells. When the NHERF1 PDZ1 domain was removed, the interaction between NHERF1 and IRBIT was eliminated. In addition, the phosphorylation of IRBIT at Ser68 is necessary for the assembly of the NHEF1-IRBIT-NHE3 complex [Citation110].
Regulation of NHE3 by the LPA5/EGFR/RSK2 Pathway
Lysophosphatidic acid (LPA), a small phospholipid that is produced by various kinds of cells or derived from food, stimulates NHE3 activity via lysophosphatidic acid receptor 5 (LPA5) transactivation of epidermal growth factor receptor (EGFR) in the apical membrane [Citation111,Citation112]. The activation of NHE3 by the LPA5/EGFR/RSK2 pathway occurs as follows: Transactivation of EGFR activates the proline kinase 2 (PYK2)-RhoA-RocK pathway and the downstream MEK-ERK pathway, and the two pathways eventually converge on the central protein RSK2 (also known as p90RSK). Then, LPA5 phosphorylates RSK2 at T573 and S363 via ERK, and then RSK2 self-phosphorylates at S380. At the same time, RhoA and RocK are involved in the activation of PYK2, which phosphorylates and activates pyruvate dehydrogenase kinase 1 (PDK1) bound to S380 and S221-phosphorylated RSK2. Finally, fully activated RSK2 directly interacts with NHE3 and phosphorylates it at S663. In conclusion, these results reveal the key role of EGFR in LPA’s regulation of NHE3, and indicate that the RhoA-RocK-PYK2 and MEK-ERK pathways converge on NHE3 [Citation113]. RSK2 is a member of the Ser/Thr protein kinase P90 ribosomal S6 kinase (RSK) family, which responds to certain growth factors and mitogens, including epidermal growth factor, insulin, and insulin-like growth factor-1 [Citation114]. PYK2, as a pH sensor to initiate an acid regulation cascade in NHE3 regulation, which acts as a scaffold for SRC-phosphorylated PDK1, is necessary for c-SRC kinase and NHE3 acid activation [Citation115]. In addition, PI3K is necessary for EGF/fibroblast growth factor (FGF) stimulation of NHE3 in fibroblasts and EGF in the ileal BB to stimulate NHE3.
βPix up-regulates NHE3 through protein–protein interaction mediated by Shank2
βPix, a small GTPase in the Rho family of guanine nucleotide exchange factors (GEFs), is a strong binding partner of Shank2 [Citation116]. Shank2 is a newly identified scaffold protein and is considered as the central coordinating protein of the postsynaptic density (PSD) membrane protein complex [Citation117], containing many specific protein–protein interaction sites, including an anchor protein repeat sequence, an SH3 domain, a PDZ domain, a proline-rich region, and a sterile motif (SAM) [Citation118]. Shank interacts with βPix to promote the accumulation of βPix-related signal molecules in the synapses of excitatory postsynaptic density membrane, regulates the membrane expression and activity of NHE3 by activating ρGTPase, small GTPases of the Rho family, and Shank2 protein in the apical region of epithelial cells [Citation119,Citation120]. In addition, the interaction between the PDZ domain of the Shank family and RSK2 (also containing a PDZ binding motif at its C-terminus), has been proven to regulate synaptic transmission through the amino-3-hydroxy-5-methyl-4-isoxazopropionic acid receptor [Citation121]. Thus, it is likely that RSK2-mediated phosphorylation of NHE3 might enhance its interaction with other proteins, such as Shank2 and Ezrin, which can link to NHE3 in the apical membrane, because NHERF2 itself is not sufficient to address the specificity of RSK2 [Citation122]. In addition, in Caco-2 epithelial cells, decreased Shank2 expression by RNA interference led to decreased expression and activity of NHE3 and enhanced the inhibition of NHE3 by cAMP [Citation120]. These results suggested that Shank2 is a novel NHE3 interacting protein that participates in the fine regulation of transepithelial salt and water transport by affecting the expression and activity of NHE3.
Conclusions and Perspectives
NHE3, as an important exchanger of Na+/H+ in the gastrointestinal tract, does not exist in the form of monomers, but in the form of complex and dynamic macromolecular complexes. The complexes, mainly including the PDZ domain proteins (NHERF1–4) of the NHERF family and the ligand protein Ezrin, are involved in phosphorylation, transport of multiple proteins, and multiple cellular pathways that interact with cellular proteins, which are integrated to regulate NHE3 activity. Acute regulation of NHE3 activity is achieved by redistribution of NHE3 between the plasma membrane and the intracellular compartments. In this review, we presented the regulatory mechanism of NHE3 (), i.e. (1) Normally, when stimulated by Ca2+, cGMP, and cAMP-dependent signaling pathways, NHE3 binds to the cytoskeleton in an immobile complex state to participate in hydrogen-sodium exchange at the apical MV, and is then converted from the immobile complex into an easily internalized and mobile form that relocates to a compartment near the base of the MV; (2) Subsequently, it is endocytosed into intracellular vesicles mainly via clathrin/albumin mediation pathways, passing through the Rab5-positive early endosomes (EE) and reorganized/reprocessed in the Golgi and endoplasmic reticulum, before being transferred to the Rab11a- positive RE; (3) NHE3 is translocated from the RE back to the apex of the plasma membrane, a process that can be stimulated by the SGLT1/P38AMP/MAPKAPK2/PI3K/AKT2/Ezrin pathway, IRBIT/PLCs mediating ANG II via a CaMKII-dependent pathway, LPA5/EGFR/RSK2 signaling, and by binding to βPix & Shank2 proteins. Our understanding of the relationship between water and salt absorption disorder in the gastrointestinal system and renal system and NHE3 activity, the protective mechanism of NHE3 on diarrhea, and the mechanism of microbial or inflammatory stimuli inhibiting NHE3 that leads to diarrhea remains limited; therefore, the complex dynamic changes and synergistic properties of NHE3-interacting proteins should be the subject of future research. It is of great practical significance to study the regulation mechanism of NHE3 activity for the treatment of diarrhea, intestinal infection and inflammation, and water and salt absorption disorders in the gastrointestinal system and renal system in the future.
Figure 5. Cyclic physiological regulation of NHE3 for sodium and hydrogen exchange in epithelial cells. (a) Transfer of NHE3 from the apical region of epithelial cells to the interstitial space. Under normal physiological conditions, NHE3 circulates between the plasma membrane and intracellular compartments. In fact, about 70% of the NHE3 subgroups seem to be tied to the cytoskeleton and play a stable role in the exchange of hydrogen and sodium. When stimulated by plasma membrane Ca2+/cAMP/cGMP and other signals, the NHE3-binding complex undergoes a series of complex dynamic changes, which transforms the stable NHE3 subgroups into movable subgroups; (b) NHE3 enters the cytoplasm through internalization from the intermicrovillar space. The movable NHE3 subgroups transfer to the intestinal intermicrovilli space. Only those NHE3 subgroups transferring to the intermicrovilli space can enter the inclusion protein through such pathways as clathrin/albumin/lipid rafts. Encapsulated vesicles can then be internalized. NHERF1 mediates cAMP regulation of NHE3, while NHERF2 mediates Ca2+ regulation of NHE3. Subsequently, internalized NHE3 is reprocessed, assembled, and recycled in the cytoplasm and assembled into circulating nucleosomes via SNX27; (c) NHE3 translocation from the RE to the apical region of the epithelial cells. When NHE3 in circulating nucleosomes is stimulated by plasma membrane activation signals, such as Ca2+/Ezrin/RSK2/Shank2, it will migrate to the plasma membrane and be re-fixed on the cytoskeleton to form stable subgroups of NHE3, thus exerting the physiological activity of hydrogen and sodium exchange
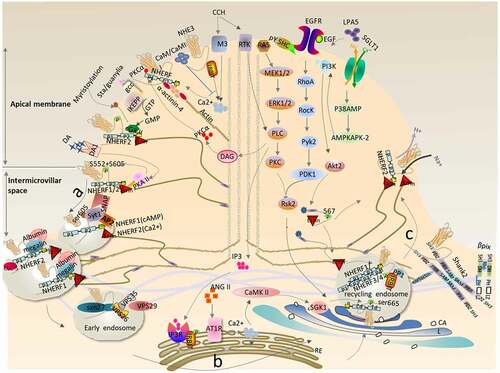
Acknowledgments
The authors gratefully acknowledge Yang Yang, Kai Wang, and other veterinary medicine students from Southwest University for valuable suggestions and assistance.
Disclosure statement
No potential conflict of interest was reported by the author(s).
Additional information
Funding
References
- He P, Yun CC. Mechanisms of the regulation of the intestinal Na+/H+ exchanger NHE3. J Biomed Biotechnol. 2010;2010:238080.
- Donowitz M, Li X. Regulatory binding partners and complexes of NHE3. Physiol Rev. 2007;87(3):825–872.
- Aronson PS. Role of SLC26-mediated Cl-/base exchange in proximal tubule NaCl transport. Novartis Found Symp. 2006;273:148–158. discussion 58-63, 261-4.
- Bobulescu IA, Moe OW. Na+/H+ exchangers in renal regulation of acid-base balance. Semin Nephrol. 2006;26(5):334–344.
- Daniel H. Molecular and integrative physiology of intestinal peptide transport. Annu Rev Physiol. 2004;66(1):361–384.
- Enns CB, Keith BA, Challa N, et al. Impairment of electroneutral Na+ transport and associated downregulation of NHE3 contributes to the development of diarrhea following in vivo challenge with Brachyspira spp. Am J Physiol Gastrointest Liver Physiol. 2020;318(2):G288–G97.
- Kumar A, Anbazhagan AN, Coffing H, et al. Lactobacillus acidophilus counteracts inhibition of NHE3 and DRA expression and alleviates diarrheal phenotype in mice infected with Citrobacter rodentium. Am J Physiol Gastrointest Liver Physiol. 2016;311(5):G817–G26.
- Sultan A, Luo M, Yu Q, et al. Differential association of the Na+/H+ exchanger regulatory factor (NHERF) family of adaptor proteins with the raft- and the non-raft brush border membrane fractions of NHE3. Cell Physiol Biochem. 2013;32(5):1386–1402.
- Xue J, Thomas L, Tahmasbi M, et al. An inducible intestinal epithelial cell-specific NHE3 knockout mouse model mimicking congenital sodium diarrhea. Clin Sci (Lond). 2020;134(8):941–953.
- Janecke AR, Heinz-Erian P, Muller T. Reduced NHE3 activity results in congenital diarrhea and can predispose to inflammatory bowel disease. Am J Physiol Regul Integr Comp Physiol. 2017;312(3):R311.
- Xue H, Zhang M, Ma J, et al. Lactose-induced chronic diarrhea results from abnormal luminal microbial fermentation and disorder of ion transport in the colon. Front Physiol. 2020;11:877.
- Priyamvada S, Gomes R, Gill RK, et al. Mechanisms underlying dysregulation of electrolyte absorption in inflammatory bowel disease-associated diarrhea. Inflamm Bowel Dis. 2015;21(12):2926–2935.
- Chen T, Hubbard A, Murtazina R, et al. Myosin VI mediates the movement of NHE3 down the microvillus in intestinal epithelial cells. J Cell Sci. 2014;127(Pt 16):3535–3545.
- Cha B, Chen T, Sarker R, et al. Lysophosphatidic acid stimulation of NHE3 exocytosis in polarized epithelial cells occurs with release from NHERF2 via ERK-PLC-PKCdelta signaling. Am J Physiol Cell Physiol. 2014;307(1):C55–65.
- Musch MW, Arvans DL, Wang Y, et al. Cyclic AMP-mediated endocytosis of intestinal epithelial NHE3 requires binding to synaptotagmin 1. Am J Physiol Gastrointest Liver Physiol. 2010;298(2):G203–11.
- Zachos NC, Alamelumangpuram B, Lee LJ, et al. Carbachol-mediated endocytosis of NHE3 involves a clathrin-independent mechanism requiring lipid rafts and Cdc42. Cell Physiol Biochem. 2014;33(3):869–881.
- Slattery C, Jenkin KA, Lee A, et al. Na+-H+ exchanger regulatory factor 1 (NHERF1) PDZ scaffold binds an internal binding site in the scavenger receptor megalin. Cell Physiol Biochem. 2011;27(2):171–178.
- Kocinsky HS, Dynia DW, Wang T, et al. NHE3 phosphorylation at serines 552 and 605 does not directly affect NHE3 activity. Am J Physiol Renal Physiol. 2007;293(1):F212–8.
- Donowitz M, Mohan S, Zhu CX, et al. NHE3 regulatory complexes. J Exp Biol. 2009;212(11):1638–1646.
- Cha B, Kenworthy A, Murtazina R, et al. The lateral mobility of NHE3 on the apical membrane of renal epithelial OK cells is limited by the PDZ domain proteins NHERF1/2, but is dependent on an intact actin cytoskeleton as determined by FRAP. J Cell Sci. 2004;117(15):3353–3365.
- Donowitz M, Ming Tse C, Fuster D. SLC9/NHE gene family, a plasma membrane and organellar family of Na(+)/H(+) exchangers. Mol Aspects Med. 2013;34(2–3):236–251.
- Sarker R, Cha B, Kovbasnjuk O, et al. Phosphorylation of NHE3-S719 regulates NHE3 activity through the formation of multiple signaling complexes. Mol Biol Cell. 2017;28(13):1754–1767.
- Cha B, Donowitz M. The epithelial brush border Na/H Exchanger NHE3 associates with the actin cytoskeleton by binding to ezrin directly and via PDZ domain-containing Na/H exchanger regulatory factor (NHERF) proteins. Clin Exp Pharmacol Physiol. 2008;35(8):863–871.
- Singh V, Lin R, Yang J, et al. AKT and GSK-3 are necessary for direct ezrin binding to NHE3 as part of a C-terminal stimulatory complex: role of a novel Ser-rich NHE3 C-terminal motif in NHE3 activity and trafficking. J Biol Chem. 2014;289(9):5449–5461.
- Cha B, Yang J, Singh V, et al. PDZ domain-dependent regulation of NHE3 protein by both internal Class II and C-terminal Class I PDZ-binding motifs. J Biol Chem. 2017;292(20):8279–8290.
- Wang D, Sun H, Lang F, et al. Activation of NHE3 by dexamethasone requires phosphorylation of NHE3 at Ser663 by SGK1. Am J Physiol Cell Physiol. 2005;289(4):C802–10.
- Chen T, Kocinsky HS, Cha B, et al. Cyclic GMP kinase II (cGKII) inhibits NHE3 by altering its trafficking and phosphorylating NHE3 at three required sites: identification of a multifunctional phosphorylation site. J Biol Chem. 2015;290(4):1952–1965.
- Zhao H, Wiederkehr MR, Fan L, et al. Acute inhibition of Na/H exchanger NHE-3 by cAMP. Role of protein kinase a and NHE-3 phosphoserines 552 and 605. J Biol Chem. 1999;274(7):3978–3987.
- Zizak M, Cavet ME, Bayle D, et al. Na+/H+ exchanger NHE3 has 11 membrane spanning domains and a cleaved signal peptide: topology analysis using in vitro transcription/translation. Biochemistry. 2000;39(27):8102–8112.
- Sarker R, Gronborg M, Cha B, et al. Casein kinase 2 binds to the C terminus of Na+/H+ exchanger 3 (NHE3) and stimulates NHE3 basal activity by phosphorylating a separate site in NHE3. Mol Biol Cell. 2008;19(9):3859–3870.
- Biemesderfer D, Nagy T, DeGray B, et al. Specific association of megalin and the Na+/H+ exchanger isoform NHE3 in the proximal tubule. J Biol Chem. 1999;274(25):17518–17524.
- D’Souza S, Garcia-Cabado A, Yu F, et al. The epithelial sodium-hydrogen antiporter Na+/H+ exchanger 3 accumulates and is functional in recycling endosomes. J Biol Chem. 1998;273(4):2035–2043.
- Alexander RT, Malevanets A, Durkan AM, et al. Membrane curvature alters the activation kinetics of the epithelial Na+/H+ exchanger, NHE3. J Biol Chem. 2007;282(10):7376–7384.
- Li X, Galli T, Leu S, et al. Na+-H+ exchanger 3 (NHE3) is present in lipid rafts in the rabbit ileal brush border: a role for rafts in trafficking and rapid stimulation of NHE3. J Physiol. 2001;537(2):537–552.
- Singh V, Yang J, Chen TE, et al. Translating molecular physiology of intestinal transport into pharmacologic treatment of diarrhea: stimulation of Na+ absorption. Clin Gastroenterol Hepatol. 2014;12(1):27–31.
- Alexander RT, Furuya W, Szaszi K, et al. Rho GTPases dictate the mobility of the Na/H exchanger NHE3 in epithelia: role in apical retention and targeting. Proc Natl Acad Sci U S A. 2005;102(34):12253–12258.
- Yang L, Leong PK, Chen JO, et al. Acute hypertension provokes internalization of proximal tubule NHE3 without inhibition of transport activity. Am J Physiol Renal Physiol. 2002;282(4):F730–40.
- Yang LE, Maunsbach AB, Leong PK, et al. Differential traffic of proximal tubule Na+ transporters during hypertension or PTH: NHE3 to base of microvilli vs. NaPi2 to endosomes. Am J Physiol Renal Physiol. 2004;287(5):F896–906.
- Zachos NC, Tse M, Donowitz M. Molecular physiology of intestinal N+/H+ exchange. Annu Rev Physiol. 2005;67(1):411–443.
- Khurana S, Kreydiyyeh S, Aronzon A, et al. Asymmetric signal transduction in polarized ileal Na(+)-absorbing cells: carbachol activates brush-border but not basolateral-membrane PIP2-PLC and translocates PLC-gamma 1 only to the brush border. Biochem J. 1996;313(Pt 2):509–518.
- Li X, Zhang H, Cheong A, et al. Carbachol regulation of rabbit ileal brush border Na+-H+ exchanger 3 (NHE3) occurs through changes in NHE3 trafficking and complex formation and is Src dependent. J Physiol. 2004;556(3):791–804.
- Kim JH, Lee-Kwon W, Park JB, et al. Ca(2+)-dependent inhibition of Na+/H+ exchanger 3 (NHE3) requires an NHE3-E3KARP-alpha-actinin-4 complex for oligomerization and endocytosis. J Biol Chem. 2002;277(26):23714–23724.
- Lee-Kwon W, Kawano K, Choi JW, et al. Lysophosphatidic acid stimulates brush border Na+/H+ exchanger 3 (NHE3) activity by increasing its exocytosis by an NHE3 kinase A regulatory protein- dependent mechanism. J Biol Chem. 2003;278(19):16494–16501.
- Lee-Kwon W, Kim JH, Choi JW, et al. Ca2+-dependent inhibition of NHE3 requires PKCα which binds to E3KARP to decrease surface NHE3 containing plasma membrane complexes. Am J Physiol Cell Physiol. 2003;285(6):C1527–36.
- Zachos NC, Hodson C, Kovbasnjuk O, et al. Elevated intracellular calcium stimulates NHE3 activity by an IKEPP (NHERF4) dependent mechanism. Cell Physiol Biochem. 2008;22(5–6):693–704.
- He D, Natarajan V, Stern R, et al. Lysophosphatidic acid-induced transactivation of epidermal growth factor receptor regulates cyclo-oxygenase-2 expression and prostaglandin E(2) release via C/EBPbeta in human bronchial epithelial cells. Biochem J. 2008;412(1):153–162.
- Vaandrager AB. Structure and function of the heat-stable enterotoxin receptor/guanylyl cyclase C. Mol Cell Biochem. 2002;230(1/2):73–83.
- Cha B, Kim JH, Hut H, et al. cGMP inhibition of Na+/H+ antiporter 3 (NHE3) requires PDZ domain adapter NHERF2, a broad specificity protein kinase G-anchoring protein. J Biol Chem. 2005;280(17):16642–16650.
- Donowitz M, Cha B, Zachos NC, et al. NHERF family and NHE3 regulation. J Physiol. 2005;567(1):3–11.
- Yun CC. Concerted roles of SGK1 and the Na+/H+ exchanger regulatory factor 2 (NHERF2) in Regulation of NHE3. Cell Physiol Biochem. 2003;13(1):29–40.
- Kurashima K, Yu FH, Cabado AG, et al. Identification of sites required for down-regulation of Na+/H+ exchanger NHE3 activity by cAMP-dependent protein kinase. phosphorylation-dependent and -independent mechanisms. J Biol Chem. 1997;272(45):28672–28679.
- Murtazina R, Kovbasnjuk O, Chen TE, et al. NHERF2 is necessary for basal activity, second messenger inhibition, and LPA stimulation of NHE3 in mouse distal ileum. Am J Physiol Cell Physiol. 2011;301(1):C126–36.
- Yun CH, Oh S, Zizak M, et al. cAMP-mediated inhibition of the epithelial brush border Na+/H+ exchanger, NHE3, requires an associated regulatory protein. Proc Natl Acad Sci U S A. 1997;94(7):3010–3015.
- Martin ER, Barbieri A, Ford RC, et al. In vivo crystals reveal critical features of the interaction between cystic fibrosis transmembrane conductance regulator (CFTR) and the PDZ2 domain of Na(+)/H(+) exchange cofactor NHERF1. J Biol Chem. 2020;295(14):4464–4476.
- Yun CH, Lamprecht G, Forster DV, et al. NHE3 kinase A regulatory protein E3KARP binds the epithelial brush border Na+/H+ exchanger NHE3 and the cytoskeletal protein ezrin. J Biol Chem. 1998;273(40):25856–25863.
- Dransfield DT, Bradford AJ, Smith J, et al. Ezrin is a cyclic AMP-dependent protein kinase anchoring protein. EMBO J. 1997;16(1):35–43.
- Lamprecht G, Weinman EJ, Yun CH. The role of NHERF and E3KARP in the cAMP-mediated inhibition of NHE3. J Biol Chem. 1998;273(45):29972–29978.
- Hu MC, Fan L, Crowder LA, et al. Dopamine acutely stimulates Na+/H+ exchanger (NHE3) endocytosis via clathrin-coated vesicles: dependence on protein kinase A-mediated NHE3 phosphorylation. J Biol Chem. 2001;276(29):26906–26915.
- Collazo R, Fan L, Hu MC, et al. Acute regulation of Na+/H+ exchanger NHE3 by parathyroid hormone via NHE3 phosphorylation and dynamin-dependent endocytosis. J Biol Chem. 2000;275(41):31601–31608.
- Murtazina R, Kovbasnjuk O, Donowitz M, et al. Na+/H+ exchanger NHE3 activity and trafficking are lipid Raft-dependent. J Biol Chem. 2006;281(26):17845–17855.
- Gekle M, Serrano OK, Drumm K, et al. NHE3 serves as a molecular tool for cAMP-mediated regulation of receptor-mediated endocytosis. Am J Physiol Renal Physiol. 2002;283(3):F549–58.
- Hryciw DH, Ekberg J, Ferguson C, et al. Regulation of albumin endocytosis by PSD95/Dlg/ZO-1 (PDZ) scaffolds. Interaction of Na+-H+ exchange regulatory factor-2 with ClC-5. J Biol Chem. 2006;281(23):16068–16077.
- Chow CW, Khurana S, Woodside M, et al. The epithelial Na(+)/H(+) exchanger, NHE3, is internalized through a clathrin-mediated pathway. J Biol Chem. 1999;274(53):37551–37558.
- Mukherjee S, Ghosh RN, Maxfield FR. Endocytosis. Physiol Rev. 1997;77(3):759–803.
- Musch MW, Arvans DL, Walsh-Reitz MM, et al. Synaptotagmin I binds intestinal epithelial NHE3 and mediates cAMP- and Ca2+-induced endocytosis by recruitment of AP2 and clathrin. Am J Physiol Gastrointest Liver Physiol. 2007;292(6):G1549–58.
- Jarousse N, Wilson JD, Arac D, et al. Endocytosis of synaptotagmin 1 is mediated by a novel, tryptophan-containing motif. Traffic. 2003;4(7):468–478.
- Sudhof TC. Synaptotagmins: why so many?. J Biol Chem. 2002;277(10):7629–7632.
- Weinman EJ, Steplock D, Donowitz M, et al. NHERF associations with sodium-hydrogen exchanger isoform 3 (NHE3) and ezrin are essential for cAMP-mediated phosphorylation and inhibition of NHE3. Biochemistry. 2000;39(20):6123–6129.
- Felder CC, Campbell T, Albrecht F, et al. Dopamine inhibits Na(+)-H+ exchanger activity in renal BBMV by stimulation of adenylate cyclase. Am J Physiol. 1990;259(2 Pt 2):F297–303.
- Wiederkehr MR, Di Sole F, Collazo R, et al. Characterization of acute inhibition of Na/H exchanger NHE-3 by dopamine in opossum kidney cells. Kidney Int. 2001;59(1):197–209.
- Kapus A, Grinstein S, Wasan S, et al. Functional characterization of three isoforms of the Na+/H+ exchanger stably expressed in Chinese hamster ovary cells. ATP dependence, osmotic sensitivity, and role in cell proliferation. J Biol Chem. 1994;269:23544–23552.
- Gekle M. Renal tubule albumin transport. Annu Rev Physiol. 2005;67(1):573–594.
- Christensen EI, Birn H. Megalin and cubilin: synergistic endocytic receptors in renal proximal tubule. Am J Physiol Renal Physiol. 2001;280(4):F562–73.
- Larsson M, Hjalm G, Sakwe AM, et al. Selective interaction of megalin with postsynaptic density-95 (PSD-95)-like membrane-associated guanylate kinase (MAGUK) proteins. Biochem J. 2003;373(2):381–391.
- Doherty GJ, McMahon HT. Mechanisms of endocytosis. Annu Rev Biochem. 2009;78(1):857–902.
- Lundmark R, Doherty GJ, Howes MT, et al. The GTPase-activating protein GRAF1 regulates the CLIC/GEEC endocytic pathway. Curr Biol. 2008;18(22):1802–1808.
- Zachos NC, Li X, Kovbasnjuk O, et al. NHERF3 (PDZK1) contributes to basal and calcium inhibition of NHE3 activity in Caco-2BBe cells. J Biol Chem. 2009;284(35):23708–23718.
- Gruenberg J, Maxfield FR. Membrane transport in the endocytic pathway. Curr Opin Cell Biol. 1995;7(4):552–563.
- Cha B, Zhu XC, Chen W, et al. NHE3 mobility in brush borders increases upon NHERF2-dependent stimulation by lyophosphatidic acid. J Cell Sci. 2010;123(14):2434–2443.
- Cai L, Loo LS, Atlashkin V, et al. Deficiency of sorting nexin 27 (SNX27) leads to growth retardation and elevated levels of N-Methyl-d-Aspartate Receptor 2C (NR2C). Mol Cell Biol. 2011;31(8):1734–1747.
- Valdes JL, Tang J, McDermott MI, et al. Sorting nexin 27 protein regulates trafficking of a p21-activated kinase (PAK) interacting exchange factor (beta-Pix)-G protein-coupled receptor kinase interacting protein (GIT) complex via a PDZ domain interaction. J Biol Chem. 2011;286(45):39403–39416.
- Cullen PJ. Endosomal sorting and signalling: an emerging role for sorting nexins. Nat Rev Mol Cell Biol. 2008;9(7):574–582.
- Seet LF, Hong W. The Phox (PX) domain proteins and membrane traffic. Biochim Biophys Acta. 2006;1761(8):878–896.
- Joubert L, Hanson B, Barthet G, et al. New sorting nexin (SNX27) and NHERF specifically interact with the 5-HT4a receptor splice variant: roles in receptor targeting. J Cell Sci. 2004;117(22):5367–5379.
- Lunn M-L, Nassirpour R, Arrabit C, et al. A unique sorting nexin regulates trafficking of potassium channels via a PDZ domain interaction. Nat Neurosci. 2007;10(10):1249–1259.
- Lauffer BE, Melero C, Temkin P, et al. SNX27 mediates PDZ-directed sorting from endosomes to the plasma membrane. J Cell Biol. 2010;190(4):565–574.
- Seaman MN. Recycle your receptors with retromer. Trends Cell Biol. 2005;15(2):68–75.
- Ler S, Hsieh CJ, Nold L, et al. The PDZ-interaction of the intestinal anion exchanger downregulated in adenoma (DRA; SLC26A3) facilitates its movement into Rab11a-positive recycling endosomes. Am J Physiol Gastrointest Liver Physiol. 2013;304(11):G980–90.
- Bobulescu IA, Dwarakanath V, Zou L, et al. Glucocorticoids acutely increase cell surface Na+/H+ exchanger-3 (NHE3) by activation of NHE3 exocytosis. Am J Physiol Renal Physiol. 2005;289(4):F685–91.
- Klisic J, Hu MC, Nief V, et al. Insulin activates Na+/H+ exchanger 3: biphasic response and glucocorticoid dependence. Am J Physiol Renal Physiol. 2002;283(3):F532–9.
- Wang D, Zhang H, Lang F, et al. Acute activation of NHE3 by dexamethasone correlates with activation of SGK1 and requires a functional glucocorticoid receptor. Am J Physiol Cell Physiol. 2007;292(1):C396–404.
- Yun CC, Chen Y, Lang F. Glucocorticoid activation of Na(+)/H(+) exchanger isoform 3 revisited. The roles of SGK1 and NHERF2. J Biol Chem. 2002;277(10):7676–7683.
- Chun J, Kwon T, Lee E, et al. The Na(+)/H(+) exchanger regulatory factor 2 mediates phosphorylation of serum- and glucocorticoid-induced protein kinase 1 by 3-phosphoinositide-dependent protein kinase 1. Biochem Biophys Res Commun. 2002;298(2):207–215.
- He P, Lee SJ, Lin S, et al. Serum- and glucocorticoid-induced kinase 3 in recycling endosomes mediates acute activation of Na+/H+ exchanger NHE3 by glucocorticoids. Mol Biol Cell. 2011;22(20):3812–3825.
- Grahammer F, Henke G, Sandu C, et al. Intestinal function of gene-targeted mice lacking serum- and glucocorticoid-inducible kinase 1. Am J Physiol Gastrointest Liver Physiol. 2006;290(6):G1114–23.
- Zhao H, Shiue H, Palkon S, et al. Ezrin regulates NHE3 translocation and activation after Na+-glucose cotransport. Proc Natl Acad Sci U S A. 2004;101(25):9485–9490.
- Shiue H, Musch MW, Wang Y, et al. Akt2 phosphorylates ezrin to trigger NHE3 translocation and activation. J Biol Chem. 2005;280(2):1688–1695.
- Gautreau A, Poullet P, Louvard D, et al. Ezrin, a plasma membrane-microfilament linker, signals cell survival through the phosphatidylinositol 3-kinase/Akt pathway. Proc Natl Acad Sci U S A. 1999;96(13):7300–7305.
- Takahashi K, Sasaki T, Mammoto A, et al. Direct interaction of the Rho GDP dissociation inhibitor with ezrin/radixin/moesin initiates the activation of the Rho small G protein. J Biol Chem. 1997;272(37):23371–23375.
- Cohen ME, Wesolek J, McCullen J, et al. Carbachol- and elevated Ca(2+)-induced translocation of functionally active protein kinase C to the brush border of rabbit ileal Na+ absorbing cells. J Clin Invest. 1991;88(3):855–863.
- Caraveo G, van Rossum DB, Patterson RL, et al. Action of TFII-I outside the nucleus as an inhibitor of agonist-induced calcium entry. Science. 2006;314(5796):122–125.
- Clapham DE. TRP channels as cellular sensors. Nature. 2003;426(6966):517–524.
- Patterson RL, van Rossum DB, Nikolaidis N, et al. Phospholipase C-gamma: diverse roles in receptor-mediated calcium signaling. Trends Biochem Sci. 2005;30(12):688–697.
- van Rossum DB, Patterson RL, Sharma S, et al. Phospholipase Cgamma1 controls surface expression of TRPC3 through an intermolecular PH domain. Nature. 2005;434(7029):99–104.
- Hudmon A, Schulman H. Structure-function of the multifunctional Ca2+/calmodulin-dependent protein kinase II. Biochem J. 2002;364(3):593–611.
- Cohen ME, Reinlib L, Watson AJ, et al. Rabbit ileal villus cell brush border Na+/H+ exchange is regulated by Ca2+/calmodulin-dependent protein kinase II, a brush border membrane protein. Proc Natl Acad Sci U S A. 1990;87(22):8990–8994.
- Houillier P, Chambrey R, Achard JM, et al. Signaling pathways in the biphasic effect of angiotensin II on apical Na/H antiport activity in proximal tubule. Kidney Int. 1996;50(5):1496–1505.
- Costa-Pessoa JM, Figueiredo CF, Thieme K, et al. The regulation of NHE(1) and NHE(3) activity by angiotensin II is mediated by the activation of the angiotensin II type I receptor/phospholipase C/calcium/calmodulin pathway in distal nephron cells. Eur J Pharmacol. 2013;721(1–3):322–331.
- Du Cheyron D, Chalumeau C, Defontaine N, et al. Angiotensin II stimulates NHE3 activity by exocytic insertion of the transporter: role of PI 3-kinase. Kidney Int. 2003;64(3):939–949.
- He P, Zhao L, No YR, et al. The NHERF1 PDZ1 domain and IRBIT interact and mediate the activation of Na+/H+ exchanger 3 by ANG II. Am J Physiol Renal Physiol. 2016;311(2):F343–51.
- Mills GB, Moolenaar WH. The emerging role of lysophosphatidic acid in cancer. Nat Rev Cancer. 2003;3(8):582–591.
- Yun CC, Kumar A. Diverse roles of LPA signaling in the intestinal epithelium. Exp Cell Res. 2015;333(2):201–207.
- Yoo BK, He P, Lee SJ, et al. Lysophosphatidic acid 5 receptor induces activation of Na+/H+ exchanger 3 via apical epidermal growth factor receptor in intestinal epithelial cells. Am J Physiol Cell Physiol. 2011;301(5):C1008–16.
- Anjum R, Blenis J. The RSK family of kinases: emerging roles in cellular signalling. Nat Rev Mol Cell Biol. 2008;9(10):747–758.
- Li S, Sato S, Yang X, et al. Pyk2 activation is integral to acid stimulation of sodium/hydrogen exchanger 3. J Clin Invest. 2004;114(12):1782–1789.
- Manser E, Loo TH, Koh CG, et al. PAK kinases are directly coupled to the PIX family of nucleotide exchange factors. Mol Cell. 1998;1(2):183–192.
- Lim S, Naisbitt S, Yoon J, et al. Characterization of the Shank family of synaptic proteins. Multiple genes, alternative splicing, and differential expression in brain and development. J Biol Chem. 1999;274(41):29510–29518.
- Sheng M, Kim E. The Shank family of scaffold proteins. J Cell Sci. 2000;113(Pt 11):1851–1856.
- Park E, Na M, Choi J, et al. The Shank family of postsynaptic density proteins interacts with and promotes synaptic accumulation of the beta PIX guanine nucleotide exchange factor for Rac1 and Cdc42. J Biol Chem. 2003;278(21):19220–19229.
- Han W, Kim KH, Jo MJ, et al. Shank2 associates with and regulates Na+/H+ exchanger 3. J Biol Chem. 2006;281(3):1461–1469.
- Thomas GM, Rumbaugh GR, Harrar DB, et al. Ribosomal S6 kinase 2 interacts with and phosphorylates PDZ domain-containing proteins and regulates AMPA receptor transmission. Proc Natl Acad Sci U S A. 2005;102(42):15006–15011.
- Cha B, Tse M, Yun C, et al. The NHE3 juxtamembrane cytoplasmic domain directly binds ezrin: dual role in NHE3 trafficking and mobility in the brush border. Mol Biol Cell. 2006;17(6):2661–2673.