ABSTRACT
The present study aimed to investigate the role of neohesperidin (NH) in mice with steroid-induced femoral head necrosis (SONFH) and in bone marrow stromal cells (BMSCs). The SONFH model was established. The effects of NH on SONFH mice were detected by hematoxylin–eosin (HE) staining and micro-CT, while those on proliferation, osteogenic differentiation and associated pathways of BMSCs were detected by molecular experiments. Besides, the effects of NH on β-catenin nuclear translocation and the H3K27me3 abundance on the transcriptional start site of Bone Morphogenetic Protein 2 (BMP2) were also determined by immunofluorescence staining and Chromatin Immunoprecipitation. Results indicated that NH not only reduced histopathological changes and improved the structures of the femoral heads of the SONFH mice but also promoted the proliferation and osteogenic differentiation of mouse BMSCs, enhanced alkaline phosphatase (ALP) activity, and upregulated expressions of osteoblast markers in a dose-dependent manner. Moreover, NH was also confirmed to upregulate the expressions of genes related to osteogenesis and Wnt/β-catenin pathway of BMSCs, which, however, were all noticeably downregulated by Noggin and DKK1. Additionally, Noggin and DKK1 in combination further promoted the suppressive effect on genes related to osteogenesis and Wnt/β-catenin pathway than alone. Besides, NH induced nuclear translocation of β-catenin in BMSCs and further reduced H3K27me3-triggered enrichment of BMP2. In conclusion, NH could promote proliferation and osteogenic differentiation of BMSCs via BMP2-Wnt/β-catenin pathway.
Introduction
Osteonecrosis of the femoral head (ONFH), also known as avascular necrosis of the femoral head, is a disease caused by interruption or damage of blood supply to the femoral head. ONFH will lead to ischemia and necrosis of the bone cells, causing structural changes of the femoral head, collapse, and joint dysfunction [Citation1]. The treatment of ONFH is confronted with various obstacles [Citation1] as long-term or high-dose use of glucocorticoids will increase the incidence of steroid-induced femoral head necrosis (SONFH) [Citation2,Citation3]. In recent years, studies have verified that the pathogenesis of SONFH is related to the factors such as intravascular coagulation, osteoporosis, fat metabolism disorder, and intraosseous hypertension [Citation4]. However, the exact pathogenesis is still unclear.
Bone marrow mesenchymal stem cells (BMSCs), which are important stem cells located in the bone marrow [Citation5], have functions of multi-directional differentiation and regulation of hematopoiesis [Citation6]. BMSCs can differentiate into diverse cells, such as osteoblasts and myoblasts under appropriate culture conditions [Citation7], which can also be used as seed cells for repairing and reconstructing injured or damaged tissues and organs [Citation8]. However, hormones can affect the activity or differentiation of BMSCs, cause necrosis and induce ischemic necrosis to the femoral head [Citation9]. Thus, it is of great significance to study the action mechanisms underlying the regulation of the direction, proliferation and differentiation of BMSCs, as well as the restoration of bone metabolism.
Traditional Chinese medicine has also been used to treat SONFH [Citation10]. As a widely used Chinese medicine, neohesperidin (NH), a plant polyphenol, is found abundantly in citrus plants [Citation11]. Studies revealed that citrus flavonoids have anti-cancer, anti-oxidant, anti-inflammatory effects on cardiovascular diseases [Citation12], which have been tested to be safe for humans [Citation13]. Additionally, NH is a natural antioxidant with anti-tumor effects [Citation14]. However, the role and mechanism of NH in SONFH are less illustrated. In this study, SONFH model in mice was constructed, and mouse BMSCs were cultured in vitro. Different doses of NH were administered to study its effects on apoptosis and osteogenic differentiation of mouse BMSCs and its role in repairing SONFH.
Materials and methods
Ethics statement
All the animal experiments were performed in accordance with the Guidelines of the China Council on Animal Care and Use. This study was approved by the Ethical Committee of Experimental Animals of Changzheng Hospital of Naval Military Medical University (S201901006). All efforts have been made to minimize the pain and discomfort caused to the animals. The animal experiments were performed in Changzheng Hospital of Naval Military Medical University.
Animal grouping and modeling
All 105 male C57 mice (6–8 weeks old, 20 ± 1 g) were raised in a specific pathogen-free environment at room temperature (at 21–23°C) under 12-h light–dark cycle with relative humidity (44%–70%). NH (1458042) was purchased from Sigma Company. The 105 mice were randomly divided into following groups by digital table method: Control group (n = 20, only saline was injected); Model group (n = 25, subcutaneous injection of 20 mg/kg methylprednisolone (MPSL) for 6 weeks to induce osteonecrosis); Model+10NH group (n = 20, after being injected with MPSL for 1 h, mice received intragastric administration of NH at 10 g/kg/d for 6 weeks); Model+15NH group (n = 20, after being injected with MPSL for 1 h, mice received intragastric administration of NH at 15 g/kg/d for 6 weeks) and Model+22.5NH group (n = 20, after being injected with MPSL for 1 h, mice received intragastric administration of NH at 22.5 g/kg/d for 6 weeks).
Twelve weeks after the model establishment, the mice were sacrificed under general anesthesia by injecting pentobarbital (B5646-50 mg, ApexBio, USA) at 40 mg/kg and xylidinothiazole (BD161432, Bidepharm, CA, IM) at 0.1 mg/kg. Then, the hind limb femurs were dissected under sterile conditions. Parts of the femur tissue harvested in each group were dehydrated, paraffin-embedded, and sliced for HE staining and immunohistochemistry, while the remaining samples were used for Western blot [Citation15].
Cell culture and treatments
As previously described, the BMSCs were isolated by density gradient centrifugation [Citation16]. The BMSCs were isolated from the tibia and fibula of the healthy mice (aged 2 to 3 weeks old). During the operation, the bone marrow of the femurs was collected to separate BMSCs by density gradient centrifugation. Mononuclear cells in an equal amount of Percoll solution (P4937, Sigma, USA) were isolated by centrifuging at 1000 × g for 30 min and then cultured in DMEM (12491015, ThermoFisher, USA) in a humidified atmosphere at 37°C with 5% CO2. After 4 days of culture, fresh medium was added by discarding the original medium and the cells were grown to 60% to 80% confluence and then passaged. The surface marker phenotypes of CD29, CD90 and CD34 were identified by flow cytometry, and the cells confirmed to be BMSCs from passage 1 to passage 3 were used for subsequent experiments.
NH (1458042), DKK1 (371207) and Noggin (N6784) were purchased from Sigma Company. The BMSCs were cultured in an osteogenic induction medium (OIM) containing 10−8 mol/L dexamethasone, 50 mM l-ascorbic acid-2-phosphate and 10−2 mol/L β-glycerophosphate. The cells were treated with NH at different concentrations (12.5, 25, 50 and 100 μM). The optimal concentration of NH that could induce the proliferation and osteogenic differentiation of BMSCs was chosen for subsequent experiments. To observe the effects of NH, NH + Noggin and NH + DKK1 on osteogenic differentiation of BMSCs, the cells were divided into the following five groups: Control group (cultured with PBS and OIM); NH group (cultured with 50 μM NH and OIM); NH + Noggin group (cultured with a mixture of OIM, 50 μM NH and 10−7mol/L Noggin); NH + DKK1 group (cultured with a mixture of OIM, 50 μM NH and 2 mg/mL DKK1) and NH+ Noggin + DKK1 group (cultured with a mixture of OIM, 50 μM NH, 10−7mol/L Noggin and 2 mg/mL DKK1).
Hematoxylin-eosin (HE) staining
After dewaxing the paraffin-embedded sections, the sample sections were stained by hematoxylin for 5 min, separated by hydrochloric acid ethanol for 5 s, rinsed with ammonia water for 5 s, and stained by eosin for 20 s in sequence. Then, the tissue samples were immersed in 70% ethanol for 5 min, 90% ethanol for 5 min, anhydrous alcohol for 5 min, xylene I for 5 min, xylene II for 15 min, and xylene III for 5 min. After sealing, the pathological changes of femur tissue around the hematoma were observed under a microscope and photographed. Bone cell cavities, concentrated nuclei, and osteonecrosis were examined after necrosis was found in bone marrow hematopoietic cells or adipocytes. The ratio of empty bone dimples (the number of empty bone lacuna/bone cells) in each femoral head was calculated using the coronal section at the maximum width of the femur.
Micro-CT
Micro-CT (μCT, GE Healthcare Biosciences, USA) was used to examine the changes occurred in the femoral head specimen and trabecular bone. Calculation was based on the following parameters: bone volume (BV), bone surface (BS), trabecular bone pattern factor (Tb.Pf), structure model index (SMI), trabecular thickness (Tb.Th), trabecular number (Tb.N), trabecular separation (Tb.Sp), and bone mineral density (BMD).
CCK-8 assay
The BMSCs at a density of 5 × 104 cells/mL were added to a 96-well plate and incubated for 24 h at 37°C with 5% CO2, and 2 × 104 cells were cultured with different concentrations of NH (12.5, 25, 50, 100 μM, respectively) for 24 h. After the treatment for 1, 2, 3, 7, and 14 day(s), 10 μL CCK-8 solution (HY-K0301, MedChemExpress, USA) was added to the cells. The absorbance at 450 nm was measured using a microplate reader (24072800, ThermoFisher, USA) at 37°C.
Alkaline phosphatase (ALP) activity assay
The BMSCs were cultured in a 96-well plate at 37°C with 5% CO2 to reach 80% confluence and then treated with OIM or OIM containing different concentrations of NH (12.5, 25, or 50 μM) for 1, 2, 3, 7, 14 day(s). ALP activities of the BMSC lysates were determined using ALP assay kit (D720338-0096, AMEKO, CA).
Alizarin Red S staining (ARS)
The cells were fixed by 70% ice-cold ethanol at room temperature for 1 h, washed by ddH2O for 5 times, and then stained by 40 mM Alizarin Red S (ECM815, Millipore, and USA) for 10–15 min with gentle stirring. Next, the cells were washed again by ddH2O for 5 times, and the orange-red spots were formed by calcified nodules.
Real-time quantitative polymerase chain reaction (RT-qPCR)
The lysis of the cells and tissues, and extraction of total RNAs were performed using Trizol method at 4°C. All the consumables and reagents used in the extraction process were subjected to DEPC treatment. Reverse transcription of mRNAs was conducted using TaqMan Reverse Transcription Reagents (N8080234, ThermoFisher, USA). RT-qPCR was conducted using Verso 1-step RT-qPCR Kit (A15300; Thermo Scientific, MA, USA) in the Opticon RT-PCR detection system (ABI 7500, Life Technology, USA) under the following conditions: pretreatment at 95°C for 10 min, followed by 45 cycles at 95°C for 30 sec, at 60°C for 1 min, and finally at 60°C for 1 min, and then preserved at 4°C. The relative expressions were calculated by the 2−ΔΔCt method [Citation17]. β-actin served as an internal control for mRNAs. The primer sequences are listed in .
Table 1. Primers for RT-qPCR
Western blot
The lysis buffer containing protease inhibitor was used to treat cells for 30 min at 4°C. Instantly, protein lysates were collected by centrifugation at 12000 g, 4°C. Subsequently, the lysates (30 µg) were separated by sodium dodecyl sulfate polyacrylamide gel electrophoresis and then transferred onto polyvinylidene fluoride membranes. Next, the membranes were incubated with TBST containing 5% nonfat milk for 60 min at room temperature, and then incubated with the following primary antibodies: anti-Runx2 (ab76956, 37 kDa, 1:1000, Abcam, UK), anti-Bone Morphogenetic Protein 2 (BMP2; ab214821, 15 kDa, 1:1000, Abcam, UK), anti-TCF7 (ab30961, 49 kDa, 1:1000, Abcam), anti-Lef1 (ab137872, 44 kDa, 1:1000, Abcam), anti-c-myc (ab32072, 57 kDa, 1:1000, Abcam), anti-c-jun (ab40766, 39 kDa, 1:1000, Abcam), anti-cyclin D (ab16663, 36 kDa, 1:200, Abcam) and anti-β-actin (ab8227, 45 kDa, 1:1000, Abcam) antibodies at 4°C for 12 h. After that, the membranes were further incubated with the secondary antibody (Goat Anti-Mouse IgG or Goat Anti-Rabbit IgG as needed, 1:5000, Abcam) at room temperature for 2 h. Finally, the chemiluminescent horseradish peroxidase (HRP) substrate (Millipore) was used for chromogenic reaction.
Immunofluorescence staining
After 14 days of culture, the BMSCs were fixed by cold acetone (W332607, Sigma, USA) at room temperature for 15 min and then allowed to infiltrate through 0.1% Triton X (V900502, Sigma, USA) for 20 min. Next, the cells were incubated with 1% bovine serum albumin (15260037, ThermoFisher, USA) for 20 min to block the nonspecific antibody binding sites and then with primary anti-β-catenin antibody (1:200, ABE208, Sigma, USA) overnight at 4°C. The BMSCs were then washed three times by PBS and incubated with a 1:500 dilution of an APC-labeled rabbit secondary antibody (A10931, ThermoFisher, USA) for 1 h. The cells were washed with PBS again for three times and stained by 4-6-diamidino-2-phenylindole (DAPI) to observe the nuclear translocation of β-catenin. Finally, the BMSCs were observed under a fluorescence microscope, and the images were taken using image management software.
Chromatin immunoprecipitation (ChIP) coupled to quantitative polymerase chain reaction (qPCR)
ChIP assay was performed to assess the binding density of H3K27Me3 on BMP2 gene promoter. BMSCs were fixed with 1% formaldehyde for 10 min at room temperature, and then the fixation was terminated by the addition of 0.125 M glycine for 10 min at room temperature. After that, cell nuclei were collected and sonicated for 10 min at 4°C. Next, chromatin was de-crosslinked and then incubated with anti-histone H3 (0.0133 µg/µg chromatin and trimethyl K27 (ab6002, Abcam, UK)) overnight at 4°C. The immunoprecipitated DNA was extracted and then quantified by qPCR. The PCR primer for BMP2 transcription start site (TSS) was designed as follows: 5ʹ- GCTTCACACTCATCCGGGAC-3ʹ, 5ʹ-TTTTAAAGGAGACGCCGCCT-3ʹ. The relative level of BMP2 fragment in each group was calculated and then normalized to the input. ChIP assay was independently performed in triplicate.
Statistical analysis
The data were shown as the mean ± standard deviation (SD). Group comparison was performed using one-way analysis of variance (ANOVA), followed by Dunnett-t test in GraphPad Prism 7.0 (Graph-Pad Software Inc). Statistical significance was defined as P < 0.05.
Results
NH reduced histopathological changes of SONFH mice
To investigate the role of NH in SONFH, the SONFH mouse model was successfully established, and the effects of NH with different concentrations (10, 15, 22.5 g/kg/d) on the pathological changes of mouse femurs were detected by HE staining. The results uncovered that bone marrow cell debris was accumulated in the Model group compared with the Control group, the tendency of which was attenuated notably by different concentrations of NH. In addition, the trabecular cavity rate of the Model group was obviously higher than that of the Control group, but that was dose-dependently reduced in Model + NH groups (P< 0.001, ).
Figure 1. NH treatment reduced the histopathological changes of the SONFH mice and improved the structure of the femoral head. (a) HE staining was used to observe the effects of different concentrations of NH (10, 15, and 22.5 g/kg/d) on the pathological changes of femurs. Magnification, 100×; Scale bar = 30 µm. (b) Changes in femoral head tissue and trabecular bone were detected using μ-CT. The bone volume fraction (BV/TV) was calculated after SONFH mice treated with NH. (c) The trabecular bone morphology factor (Tb.Pf) was calculated after SONFH mice treated with NH. (d) The trabecular thickness (Tb.Th) was calculated after SONFH mice treated with NH. (e) The trabecular bone number (Tb.N) was calculated after SONFH mice treated with NH. (f) The trabecular spacing (Tb.Sp) was calculated after SONFH mice treated with NH. (g) The bone mineral density (BMD) was calculated after SONFH mice treated with NH. SONFH: steroid-induced femoral head necrosis; NH: Neohesperidin; ***P < 0.001 vs. control; #P< 0.05, ##P< 0.01, ###P< 0.001 vs. model).
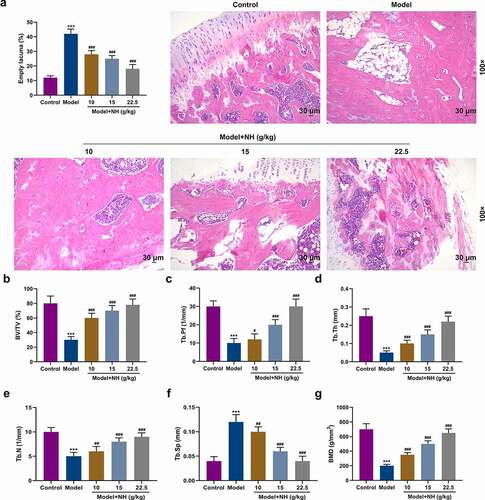
NH improved the structure of the femoral head of SONFH mice
To examine the effects of NH on the structure of mouse femoral head, the SONFH mouse model was treated with different concentrations of NH (10, 15, 22.5 g/kg/d) for constructing bone loss in vivo. Compared with the Control group, the levels of BV/TV, Tb.Pf, Tb.Th, Tb.N and BMD in the Model group were markedly reduced, but that of Tb.Sp was greatly elevated. The effects of steroids on the above-mentioned levels of microstructure parameters were reversed in Model + NH groups as compared with those in the Model group (P< 0.05, ).
NH promoted proliferation and osteogenic differentiation of the mouse BMSCs
Flow cytometry was conducted to detect P2 cells, most of which were positive for CD29 and CD90, and negative for CD34 (), demonstrating that a majority of the isolated and purified cells were BMSCs. The cells were treated with different concentrations of NH (12.5, 25, 50 or 100 μM) in OIM to further investigate the effects of NH on the proliferation and osteogenic differentiation of BMSCs. CCK-8 assay validated that the proliferation of BMSCs was augmented by NH at varying time points, with the most remarkable increment induced by 50 μM NH and the most obvious reduction induced by 100 μM NH (P< 0.05, ). Therefore, 50 μM of NH was selected for subsequent experiments. ALP activity assay revealed that NH enhanced ALP activity (P< 0.05, ) in a dose-dependent manner, which was also consistent with the results of Alizarin Red S staining because more mineralized matrix deposition was observed as the NH dose increased (). RT-qPCR was carried out to determine the expressions of osteoblast markers (Runx2, osteocalcin, β-catenin, and BMP2), unearthing that such expressions were upregulated greatly by NH in a dose-dependent manner (P< 0.05, ).
Figure 2. NH promoted proliferation and osteogenic differentiation of BMSCs. (a) Flow cytometry was used to identify standard markers (CD29, CD90 and CD34) for BMSCs. (b) The effects of NH on BMSCs viability were detected by CCK-8 on day 1, 2, 3, 7, and 14 after the treatment with different concentrations of NH. (c) ALP assay kit was used to detect the ALP activity of each group on day (s) 1, 2, 3, 7 and 14 after treatment with different concentrations of NH. (d) Alizarin Red S staining was used for mineralized matrix deposition. (e-h) RT-qPCR was used for the detection of osteoblast markers (Runx2, Osteocalcin, β-catenin, BMP2) after BMSCs treated with different concentrations of NH. (BMSCs: bone mesenchymal stem; RT-qPCR: real-time quantitative polymerase chain reaction; NH: Neohesperidin; CCK-8: Cell Counting Kit-8; *P < 0.05, **P < 0.01, ***P < 0.001 vs. control).
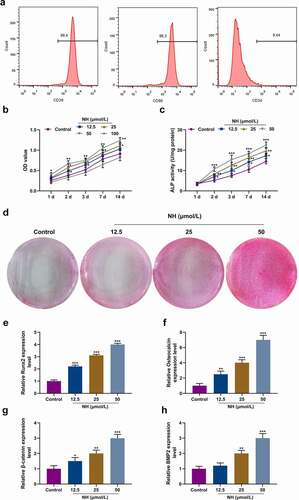
NH upregulated the expressions of osteogenic genes and Wnt/β-catenin pathway in BMSCs
BMP2 inhibitor Noggin, Wnt inhibitor DKK1, and NH were used to treat the BMSCs to further figure out the effects of NH on the expressions of osteogenic genes [Runx2, BMP2, Osteopontin (OPN), DLX5, Collagen I and Osteocalcin (OCN)]. In contrast with the Control group, RT-qPCR proved that NH prominently upregulated the aforementioned osteogenic genes expressions on days 3, 7, and 14. Moreover, the NH-induced upregulated expressions of the mRNAs were noticeably reversed by Noggin and DKK1. In addition, the expression level of BMP2 was reduced markedly by Noggin than DKK1 (P< 0.05, ). Furthermore, the expressions of these aforementioned osteogenic genes except for BMP2 were further downregulated in NH + Noggin + DKK1 group compared with those in the NH + DKK1 group and NH + Noggin group (P< 0.05, ). We also found that the expression of BMP2 was higher in NH + DKK1 group than that in NH + Noggin + DKK1 group (P< 0.05, ), further confirming that Noggin instead of DKK1 suppressed the expression of BMP2.
Figure 3. NH upregulated osteogenic gene expressions in BMSCs. (a-f) RT-qPCR was performed to detect the effects of NH on the expressions of osteogenic genes [Runx2, BMP2, Osteopontin (OPN), DLX5, Collagen I and Osteocalcin (OCN)] in Control group, NH group, NH+Noggin group, NH+DKK1 group and NH+Noggin+DKK1 group on days 3, 7 and 14. Each experiment was repeated three times, and β-actin served as an internal control (BMSCs: bone mesenchymal stem cells; RT-qPCR: real-time quantitative polymerase chain reaction; NH: Neohesperidin; *P < 0.05, **P< 0.01, ***P< 0.001, vs. control, ##P < 0.01, ###P < 0.001 vs. NH, ^^^P< 0.001 vs. NH+Noggin+DKK1).
![Figure 3. NH upregulated osteogenic gene expressions in BMSCs. (a-f) RT-qPCR was performed to detect the effects of NH on the expressions of osteogenic genes [Runx2, BMP2, Osteopontin (OPN), DLX5, Collagen I and Osteocalcin (OCN)] in Control group, NH group, NH+Noggin group, NH+DKK1 group and NH+Noggin+DKK1 group on days 3, 7 and 14. Each experiment was repeated three times, and β-actin served as an internal control (BMSCs: bone mesenchymal stem cells; RT-qPCR: real-time quantitative polymerase chain reaction; NH: Neohesperidin; *P < 0.05, **P< 0.01, ***P< 0.001, vs. control, ##P < 0.01, ###P < 0.001 vs. NH, ^^^P< 0.001 vs. NH+Noggin+DKK1).](/cms/asset/65796993-2eea-4c9a-bd4f-3624443adfe5/kccy_a_2015668_f0003_oc.jpg)
To further explore the molecular mechanisms underlying the effects of NH on BMSCs, we detected the mRNA and protein expressions of Wnt/β-catenin pathway-related genes, including TCF7, Lef1, c-myc, c-jun, and cyclin D. The data revealed that compared with the Control group, NH evidently upregulated Lef1 and c-jun mRNA and protein expressions on days 3, 7 and 14, as well as TCF7 and cyclin D mRNA and protein expressions on days 3 and 7 (P< 0.05, , ). In addition, NH evidently upregulated the mRNA c-myc on days 7 and 14, and increased the protein expression of on days 3, 7 and 14 (P< 0.05, ). Moreover, the increased mRNA levels of TCF7 and cyclin D were reversed by the pretreatment of DKK1 and Noggin (P< 0.05, , ), whereas similar results emerged in Western blot analysis of Runx2, BMP2 and TCF7 (P < 0.05, ).
Figure 4. Effects of NH treatment on the expressions of Wnt/β-catenin signaling pathway-related and osteogenic genes. (a-i) RT-qPCR and Western blot was used for the detection of the expressions of Wnt/β-catenin signaling pathway-related proteins (TCF7, Lef1, c-myc) in Control group, NH group, NH+Noggin group, NH+DKK1 group, and NH+Noggin+DKK1 group on day 3, 7 and 14. Each experiment was repeated three times, and β-actin served as an internal control (BMSCs: bone mesenchymal stem cells; RT-qPCR: real-time quantitative polymerase chain reaction; NH: Neohesperidin; *P < 0.05 ***P < 0.001, vs. control, ##P < 0.01, ###P < 0.001 vs. NH, ^^P< 0.01, ^^^P< 0.001 vs. NH+Noggin+DKK1).
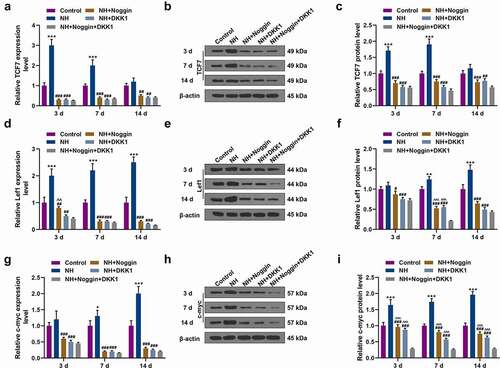
Figure 5. Effects of NH treatment on the expressions of c-jun, cyclin D, Runx2, BMP2 and TCF7. (a-f) RT-qPCR and Western blot was used for the detection of the expressions of c-jun and cyclin D in Control group, NH group, NH+Noggin group, NH+DKK1 group, and NH+Noggin+DKK1 group on day 3, 7 and 14. (g-j) Western blot was used for the detection of the expressions of Runx2, BMP2 and TCF7 in Control group, NH group, NH+Noggin group, NH+DKK1 group, and NH+Noggin+DKK1 group. Each experiment was repeated three times, and β-actin served as an internal control (BMSCs: bone mesenchymal stem cells; RT-qPCR: real-time quantitative polymerase chain reaction; NH: Neohesperidin; *P < 0.05 ***P < 0.001, vs. control, ##P < 0.01, ###P < 0.001 vs. NH, ^^P< 0.01, ^^^P< 0.001 vs. NH+Noggin+DKK1).
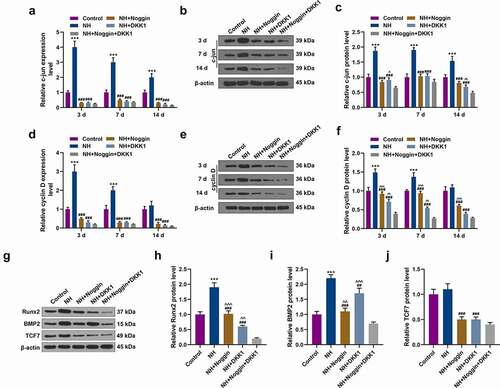
Immunofluorescence was performed to detect the effects of NH on β-catenin expression in the BMSCs, with the results revealing that NH induced nuclear translocation of β-catenin in the BMSCs ().
Figure 6. NH regulated the nuclear translocation of β-catenin and the H3K27me3 abundance on BMP2. (a) The BMSCs were treated with OIM in Control group, NH group, NH+Noggin group, and NH+DKK1 group. After incubation for 14 days, immunofluorescence staining of β-catenin (red) and DAPI (nuclear staining, blue) was used to stain the BMSCs (Scale bar = 40 µM; magnification, 200×; BMSCs: bone mesenchymal stem cells; NH: Neohesperidin). (b) ChIP-qPCR was performed to assess the H3K27me3 abundance on TSS of BMP2 gene. This assay was repeated independently for three times. ***P < 0.001 vs. control.
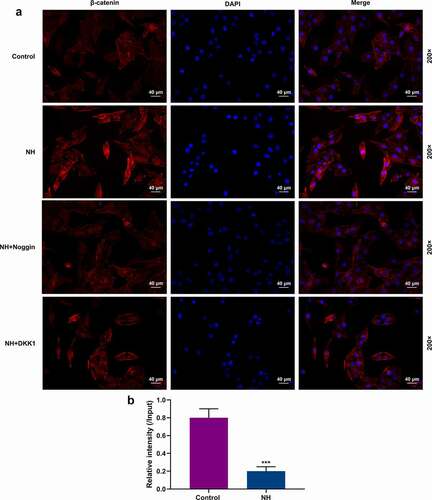
Epigenetic modulator H3K27me3 can act as an indicator of gene transcription [Citation18]. Thus, we examined whether NH modulated BMP2 expression through epigenetic modification pathway. A ChIP-qPCR assay was performed to assess the H3K27me3 abundance on BMP2 promoter, unveiling that NH overtly reduced the relative enrichment of H3K27me3 on BMP2 promoter compared with that in the Control group (, p< 0.001).
Discussion
SONFH is a glucocorticoid-induced refractory disease [Citation1]. BMSCs, which have been proven to be effective in rescuing femoral head necrosis [Citation19,Citation20], could differentiate into multiple bone stromal cells and are therefore used in cell-based therapies toward ONFH [Citation21]. Glucocorticoids can cause fat metabolism disorders, reduce osteogenic differentiation, and induce differentiation of BMSCs into adipogenic direction, thus promoting blood lipid levels, fatty acid blockage of tiny blood vessels, and microcirculatory disorders [Citation4,Citation21]. NH, a natural antioxidant with anti-inflammatory and anti-tumor effects [Citation11], inhibits the differentiation of osteoclasts and the functions of osteoclasts during bone metabolism [Citation14]. Our previous research found that neohesperidin ameliorated steroid-induced osteonecrosis of the femoral head by inhibiting the histone modification of lncRNA HOTAIR [Citation22]. In addition, it has been reported that ganluyin, a Traditional Chinese medicine containing NH, can treat chronic pharyngitis [Citation23,Citation24]. The main components of ethanol extract isolated from Weiqi Decoction include naringin, cinnamin, neohesperidin and hesperidin, and Weiqi Decoction, contain was prescribed for patients with functional dyspepsia and chronic atrophic gastritis to improve their health by raising qi, smoothing the blood and harmonizing the Stomach (Wei) [Citation25].
Traditional Chinese medicine has been used in treating SONFH [Citation10,Citation26]. Liu et al. observed that Epimedium extracts increase bone mineral density of SONFH mice [Citation27] through enhancing anti-apoptosis protein expression of BMSCs and reducing autophagy-related protein expressions. NH is mainly used in food science and medicine [Citation27]. Lee et al. isolated a neohesperidin from the ethanolic extracts of Poncirus trifoliata, which markedly inhibited the growth of human gastric cancer cell lines [Citation28]. Mandalari et al. proposed that NH generated certain inhibitory effects on Gram-negative bacteria and Saccharomyces cerevisiae [Citation29]. Through HE staining, we observed abnormally increased intracavity lesions in SONFH mice and the accumulation of adipocytes in their bone marrow cavities, whilst NH treatment can ameliorate such lesions, implying that NH can improve fat metabolism disorders. In addition, a new μ-CT examination was performed in the current study, disclosing that NH could enhance the bone density of SONFH mice and improve the structure of the femoral head, which is similar to the effects of Epimedium extracts.
The activity of BMSCs is closely related to SONFH [Citation19,Citation30]. Therefore, we isolated BMSCs from the healthy mice for further study. Both CD29 and CD90 are expressed on the surface of BMSCs [Citation31,Citation32]. CD34 is an adhesion molecule expressed in the hematopoietic stem cells and gradually disappears as the cells become mature [Citation31]. Our results showed that BMSCs were in long-spindle and fibroblast-like shape and that the characteristics of CD29 (+), CD90 (+), and CD34 (-) were consistent with the characteristics of surface molecules of mesenchymal stem cells. The results of the CCK-8 assay demonstrated that 50 μM NH promoted the proliferation of BMSCs, which, however, was inhibited by 100 μM NH; thus, the 100 μM NH-treatment group was excluded from the subsequent experiment. ALP, which is an early marker of osteogenesis, promotes matrix mineralization during osteogenesis [Citation33]. The results of Alizarin Red S staining and ALP activity assays indicated that NH promoted osteogenic differentiation of BMSCs. Runx2 is the main regulator of osteogenesis in the early stage, and it plays an important role in the intersection of bone-related genes, such as bone morphogenetic protein (BMP) signaling pathway [Citation34,Citation35]. Our further results revealed that NH can promote the expressions of osteoblast markers (Runx2, Osteocalcin, β-catenin, and BMP2).
Yougui pills promote the formation of SONFH through activating β-catenin [Citation36]. Zhai et al. [Citation37] confirmed that resveratrol can effectively prevent the occurrence of early SONFH through increasing the blood supply in SONFH mice model, and its anti-inflammatory effects are also conductive to protecting the vascular endothelium and reducing thrombosis of the mice [Citation37]. However, the underlying mechanisms of NH in repairing SONFH still remained unclear. Our study revealed that NH upregulated the expressions of RUNX2, BMP2, OPN, DLX5, Collagen I and OCN, which, however, were notably inhibited by the combination of Noggin and DKK1. Both BMP and Wnt/β-catenin pathways make profound impacts upon osteogenic differentiation and formation [Citation38,Citation39]. It has been found that a variety of natural products can promote the differentiation of BMSCS, such as polydatin, promotes the osteogenic differentiation of human bone mesenchymal stem cells by activating the BMP2-Wnt/β-catenin signaling pathway [Citation38]. Puerarin promotes BMSC differentiation and bone formation and increased bone mass in rats with bone grafts through microRNA-155-3p-mediated p53/TNF-α/STAT1 signaling pathway [Citation40]; it also could stimulate osteogenic differentiation and bone formation through the ERK1/2 and p38-MAPK signaling pathways [Citation41]. Chrysophanic acid shifts the differentiation tendency of BMSCs to prevent alcohol-induced osteonecrosis of the femoral head [Citation42]. Icariin promotes osteogenic differentiation of BMSCs by upregulating BMAL1 expression via BMP signaling [Citation43]. The present results affirmed that DKK1 exerted no significant effect on the mRNA level of BMP2. β-catenin, a key factor in the Wnt/β-catenin pathway, is involved in cell proliferation and differentiation [Citation44]. The concentration of β-catenin in the cytoplasm determines the activation and closure of the canonical Wnt pathway [Citation44,Citation45]. The cytoplasmic β-catenin is stable but can accumulate in the nuclei to form a complex with the transcription factor LEF/TCF, thereby upregulating the expressions of downstream genes (C-myc, cyclin D1 and c-jun) [Citation45,Citation46]. This study demonstrated that NH upregulated the expressions of genes related to Wnt/β-catenin signaling pathway to varying degrees, which, however, can be reduced by the pretreatment of DKK1 and Noggin. Furthermore, NH induced nuclear translocation of β-catenin in BMSCs, unearthing that NH participated in the osteogenic differentiation through inducing BMP2 to activate the Wnt/β-catenin signaling pathway. H3K27me3 is a well-known repressive modulator that triggers chromatin inactivation and gene transcription [Citation47], which is also involved in osteogenic differentiation of human MSCs [Citation48,Citation49]. Our findings corroborated that the enrichment of H3K27me3 on TSS of BMP2 gene was induced by NH, signifying that NH might promote transcriptional activity of BMP2 gene, which was consistent with our previous data [Citation22].
However, it should be noted that several limitations existed in this research. For instance, the underlying mechanism of NH remains to be elucidated. In addition, the mechanism through which NH regulated enrichment of H3K27me3 on BMP2 TSS and modulated the Wnt/β-catenin signaling pathway should be further investigated. Moreover, in vivo experiment should be conducted in future studies.
In conclusion, NH improves the histopathology and structure of the femoral head in the SONFH mice, and promotes the proliferation and osteogenic differentiation of mouse BMSCs. The potential mechanism may be the activation of the expressions of genes related to the BMP2-Wnt/β-catenin signaling pathway and the inhibition of the expressions of adipogenic factors.
Disclosure statement
No potential conflict of interest was reported by the author(s).
Additional information
Funding
References
- Wang XS, Zhuang QY, Weng XS, et al. Etiological and clinical analysis of osteonecrosis of the femoral head in Chinese patients. Chin Med J (Engl). 2013.Jan;126(2):290–295. PubMed PMID: 23324279.
- Kubo T, Ueshima K, Saito M, et al. Clinical and basic research on steroid-induced osteonecrosis of the femoral head in Japan. J Orthop Sci. 2016 Jul;21(4):407–413. PubMed PMID: 27062553.
- Wei Q, Yang F, Chen X, et al. [Microarchitecture features and pathology of necrotic region in patients with steroid-induced and alcohol-induced osteonecrosis of femoral head]. Zhongguo Xiu Fu Chong Jian Wai Ke Za Zhi. 2018 Jul 15;32(7):866–872. PubMed PMID: 30129310.
- Wang A, Ren M, Wang J. The pathogenesis of steroid-induced osteonecrosis of the femoral head: a systematic review of the literature. Gene. 2018 Sep 10;671:103–109. PubMed PMID: 29859289.
- Pontikoglou C, Deschaseaux F, Sensebe L, et al. Bone marrow mesenchymal stem cells: biological properties and their role in hematopoiesis and hematopoietic stem cell transplantation. Stem Cell Rev Rep. 2011 Sep 7;7(3):569–589. PubMed PMID: 21249477.
- Miao C, Lei M, Hu W, et al. A brief review: the therapeutic potential of bone marrow mesenchymal stem cells in myocardial infarction. Stem Cell Res Ther. 2017 Nov2;8(1):242. PubMed PMID: 29096705; PubMed Central PMCID: PMCPMC5667518.
- Mohamed-Ahmed S, Fristad I, Lie SA, et al. Adipose-derived and bone marrow mesenchymal stem cells: a donor-matched comparison. Stem Cell Res Ther. 2018 Jun 19;9(1):168. PubMed PMID: 29921311; PubMed Central PMCID: PMCPMC6008936.
- Zhao P, Xiao L, Peng J, et al. Exosomes derived from bone marrow mesenchymal stem cells improve osteoporosis through promoting osteoblast proliferation via MAPK pathway. Eur Rev Med Pharmacol Sci. 2018 Jun;22(12):3962–3970. PubMed PMID: 29949171.
- Liu X, Li Q, Niu X, et al. Exosomes secreted from human-induced pluripotent stem cell-derived mesenchymal stem cells prevent osteonecrosis of the femoral head by promoting angiogenesis. Int J Biol Sci. 2017;13(2):232–244. PubMed PMID: 28255275; PubMed Central PMCID: PMCPMC5332877.
- Qiang H, Liu H, Ling M, et al. Early steroid-induced osteonecrosis of rabbit femoral head and panax notoginseng saponins: mechanism and protective effects. Evid Based Complement Alternat Med. 2015;2015:719370. PubMed PMID: 25866538; PubMed Central PMCID: PMCPMC4378605.
- Wu H, Liu Y, Chen X, et al. Neohesperidin exerts lipid-regulating effects in vitro and in vivo via fibroblast growth factor 21 and AMP-activated protein kinase/sirtuin type 1/peroxisome proliferator-activated receptor gamma coactivator 1alpha signaling axis. Pharmacology. 2017;100(3–4):115–126. PubMed PMID: 28554169.
- Han GE, Kang HT, Chung S, et al. Novel neohesperidin dihydrochalcone analogue inhibits adipogenic differentiation of human adipose-derived stem cells through the Nrf2 pathway. Int J Mol Sci. 2018 Jul 29;19(8):8. PubMed PMID: 30060630; PubMed Central PMCID: PMCPMC6121477.
- Wen L, Jiang Y, Yang J, et al. Structure, bioactivity, and synthesis of methylated flavonoids. Ann N Y Acad Sci. 2017 Jun;1398(1):120–129. PubMed PMID: 28436044.
- Tan Z, Cheng J, Liu Q, et al. Neohesperidin suppresses osteoclast differentiation, bone resorption and ovariectomised-induced osteoporosis in mice. Mol Cell Endocrinol. 2017 Jan 5;439:369–378. PubMed PMID: 27664516.
- Xu J, Gong H, Lu S, et al. Animal models of steroid-induced osteonecrosis of the femoral head-a comprehensive research review up to 2018. Int Orthop. 2018 Jul;42(7):1729–1737. PubMed PMID: 29705870.
- Boregowda SV, Krishnappa V, Phinney DG. Isolation of mouse bone marrow mesenchymal stem cells. Methods Mol Biol. 2016;1416:205–223. PubMed PMID: 27236673.
- Livak KJ, Schmittgen TD. Analysis of relative gene expression data using real-time quantitative PCR and the 2(-Delta Delta C(T)) Method. Methods. 2001Dec;25(4):402–408. PubMed PMID: 11846609; eng.
- Lan F, Bayliss PE, Rinn JL, et al. A histone H3 lysine 27 demethylase regulates animal posterior development. Nature. 2007 Oct 11;449(7163):689–694. PubMed PMID: 17851529; eng.
- Fang S, Li Y, Chen P. Osteogenic effect of bone marrow mesenchymal stem cell-derived exosomes on steroid-induced osteonecrosis of the femoral head. Drug Des Devel Ther. 2019;13:45–55. PubMed PMID: 30587927; PubMed Central PMCID: PMCPMC6305133.
- Wang Q, Yang Q, Chen G, et al. LncRNA expression profiling of BMSCs in osteonecrosis of the femoral head associated with increased adipogenic and decreased osteogenic differentiation. Sci Rep. 2018 Jun 14;8(1):9127. PubMed PMID: 29904151; PubMed Central PMCID: PMCPMC6002551.
- Yoon BH, Jones LC, Chen CH, et al. Etiologic classification criteria of ARCO on femoral head osteonecrosis part 1: glucocorticoid-associated osteonecrosis. J Arthroplasty. 2019 Jan;34(1):163–168e1. PubMed PMID: 30348552.
- Yuan S, Zhang C, Zhu Y, et al. Neohesperidin ameliorates steroid-induced osteonecrosis of the femoral head by inhibiting the histone modification of lncRNA HOTAIR. Drug Des Devel Ther. 2020;25:5419–5430. PubMed PMID: 33324039; PubMed Central PMCID: PMCPMC7733036. eng.
- Chen YH, Luo R, Lei SS, et al. Anti-inflammatory effect of Ganluyin, a Chinese classic prescription, in chronic pharyngitis rat model. BMC Compl med Ther. 2020 Aug 28;20(1):265. PubMed PMID: 32859182; PubMed Central PMCID: PMCPMC7456022. eng.
- Feng XLY. Clinical observation on the treatment of 78 cases of chronic pharyngitis with Ganluyin. Mongol J Tradit Chin Med. 2011;30(17):9–10.
- Shi HL, Tan B, Ji G, et al. The ethanol extract isolated from Weiqi Decoction induces G₂/M arrest and apoptosis in AGS cells. Chin J Integr Med. 2014 Jun;20(6):430–437. PubMed PMID: 23943506; eng.
- Jiang Y, Zhang Y, Chen W, et al. Achyranthes bidentata extract exerts osteoprotective effects on steroid-induced osteonecrosis of the femoral head in rats by regulating RANKL/RANK/OPG signaling. J Transl Med. 2014 Nov29;12(1):334. PubMed PMID: 25471933; PubMed Central PMCID: PMCPMC4256888.
- Liu S, Huang Y, Wang C, et al. Epimedium protects steroid-induced avascular necrosis of femoral head in rats by inhibiting autophagy. Exp Ther Med. 2018 Dec;16(6):5047–5052. PubMed PMID: 30542458; PubMed Central PMCID: PMCPMC6257266.
- Lee JH, Lee SH, Kim YS, et al. Protective effects of neohesperidin and poncirin isolated from the fruits of Poncirus trifoliata on potential gastric disease. Phytother Res. 2009 Dec;23(12):1748–1753. PubMed PMID: 19367677.
- Mandalari G, Bennett RN, Bisignano G, et al. Antimicrobial activity of flavonoids extracted from bergamot (Citrus bergamia Risso) peel, a byproduct of the essential oil industry. J Appl Microbiol. 2007 Dec;103(6):2056–2064. PubMed PMID: 18045389.
- Wang A, Ren M, Song Y, et al. MicroRNA expression profiling of bone marrow mesenchymal stem cells in steroid-induced osteonecrosis of the femoral head associated with osteogenesis. Med Sci Monit. 2018 Mar 28;24:1813–1825. PubMed PMID: 29590087; PubMed Central PMCID: PMCPMC5887684.
- Mildmay-White A, Khan W. Cell surface markers on adipose-derived stem cells: a systematic review. Curr Stem Cell Res Ther. 2017;12(6):484–492. PubMed PMID: 27133085.
- Togarrati PP, Dinglasan N, Desai S, et al. CD29 is highly expressed on epithelial, myoepithelial, and mesenchymal stromal cells of human salivary glands. Oral Dis. 2018 May;24(4):561–572. PubMed PMID: 29197149; PubMed Central PMCID: PMCPMC5902634.
- Sharma U, Pal D, Prasad R. Alkaline phosphatase: an overview. Indian J Clin Biochem. 2014Jul;29(3):269–278. PubMed PMID: 24966474; PubMed Central PMCID: PMCPMC4062654.
- Komori T. Roles of Runx2 in Skeletal Development. Adv Exp Med Biol. 2017;962:83–93. PubMed PMID: 28299652.
- Komori T. Runx2, an inducer of osteoblast and chondrocyte differentiation. Histochem Cell Biol. 2018Apr;149(4):313–323. PubMed PMID: 29356961.
- Zhang P, Xu H, Wang P, et al. Yougui pills exert osteoprotective effects on rabbit steroid-related osteonecrosis of the femoral head by activating beta-catenin. Biomed Pharmacother. 2019 Oct 16;120:109520. PubMed PMID: 31629251.
- Zhai JL, Weng XS, Wu ZH, et al. Effect of Resveratrol on Preventing Steroid-induced Osteonecrosis in a Rabbit Model. Chin Med J (Engl). 2016 Apr 5;129(7):824–830. PubMed PMID: 26996479; PubMed Central PMCID: PMCPMC4819304.
- Chen XJ, Shen YS, He MC, et al. Polydatin promotes the osteogenic differentiation of human bone mesenchymal stem cells by activating the BMP2-Wnt/beta-catenin signaling pathway. Biomed Pharmacother. 2019 Apr;112:108746. PubMed PMID: 30970530.
- Zhang R, Oyajobi BO, Harris SE, et al. Wnt/beta-catenin signaling activates bone morphogenetic protein 2 expression in osteoblasts. Bone. 2013 Jan;52(1):145–156. PubMed PMID: 23032104; PubMed Central PMCID: PMCPMC3712130.
- Zhou Y, Lian H, Liu K, et al. Puerarin improves graft bone defect through microRNA-155-3p-mediated p53/TNF‑α/STAT1 signaling pathway. Int J Mol Med. 2020 Jul;46(1):239–251. PubMed PMID: 32377717; PubMed Central PMCID: PMCPMC7255454. eng.
- Yang X, Yang Y, Zhou S, et al. Puerarin stimulates osteogenic differentiation and bone formation through the ERK1/2 and p38-MAPK signaling pathways. Curr Mol Med. 2018 Mar 9;17(7):488–496. PubMed PMID: 29256352; eng.
- Yu H, Liu P, Zhu D, et al. Chrysophanic acid shifts the differentiation tendency of BMSCs to prevent alcohol-induced osteonecrosis of the femoral head. Cell Prolif. 2020 Aug;53(8):e12871. PubMed PMID: 32597546; PubMed Central PMCID: PMCPMC7445404. eng.
- Huang Z, Wei H, Wang X, et al. Icariin promotes osteogenic differentiation of BMSCs by upregulating BMAL1 expression via BMP signaling. Mol Med Rep. 2020 Mar;21(3):1590–1596. PubMed PMID: 32016461; PubMed Central PMCID: PMCPMC7002972. eng.
- Ahmadzadeh A, Norozi F, Shahrabi S, et al. Wnt/beta-catenin signaling in bone marrow niche. Cell Tissue Res. 2016 Feb;363(2):321–335. PubMed PMID: 26475718.
- Kim W, Kim M, Jho EH. Wnt/beta-catenin signalling: from plasma membrane to nucleus. Biochem J. 2013 Feb 15;450(1):9–21. PubMed PMID: 23343194.
- Hwang JH, Cha PH, Han G, et al. Euodia sutchuenensis Dode extract stimulates osteoblast differentiation via Wnt/beta-catenin pathway activation. Exp Mol Med. 2015 Mar 20;47(3):e152. PubMed PMID: 25792220; PubMed Central PMCID: PMCPMC4351407.
- Bender S, Tang Y, Lindroth AM, et al. Reduced H3K27me3 and DNA hypomethylation are major drivers of gene expression in K27M mutant pediatric high-grade gliomas. Cancer Cell. 2013 Nov11;24(5):660–672. PubMed PMID: 24183680; eng.
- Ye L, Fan Z, Yu B, et al. Histone demethylases KDM4B and KDM6B promotes osteogenic differentiation of human MSCs. Cell Stem Cell. 2012 Jul 6;11(1):50–61. PubMed PMID: 22770241; PubMed Central PMCID: PMCPMC3392612. eng.
- Camilleri ET, Dudakovic A, Riester SM, et al. Loss of histone methyltransferase Ezh2 stimulates an osteogenic transcriptional program in chondrocytes but does not affect cartilage development. J Biol Chem. 2018 Dec 7;293(49):19001–19011. PubMed PMID: 30327434; PubMed Central PMCID: PMCPMC6295726. eng.