ABSTRACT
This study aimed to explore the role of a stimulator of interferon (IFN) gene (STING) agonist in breast cancer (BCa) immunotherapy. Clinical samples were collected from 37 patients with BCa. A tumor-bearing mouse model was established by injecting 4T1 cells into the mammary fat pad of mice. STING agonist and atezolizumab were injected in the mice twice a week for 2 weeks. Peripheral blood, tumor mass, lung, liver, brain cortex and kidney samples of the tumor-bearing mice were collected. Anti-IFN alpha receptor subunit 1 (IFNAR1) was used to treat 4T1 cells. Tumor tissues of patients with BCa exhibited lower STING and high programmed cell death protein 1 and programmed death-ligand 1 protein expressions. The STING agonist inhibited 4T1 cell growth in mice (P < 0.001) and increased the IFN-β level and phosphorylation of STING, TBK1, IRF3 and STAT1 in tumor mass of tumor-bearing mice (P < 0.001). It synergized with atezolizumab to inhibit 4T1 cell growth in mice and increased tumor necrosis factor-α, IFN-β, interleukin-10 and IFN-γ levels in the peripheral blood and tumor mass (P < 0.01). It synergized with atezolizumab to increase CD8+ cytotoxic T cells and decrease FOXP3+ Treg cells in the tumor-bearing mouse model. The STING agonist was nontoxic to the lung, liver, brain cortex and kidney. Anti-IFNAR1 reversed the STING agonist promotion on TBK1, IRF3 and STAT1 phosphorylation in 4T1 cells (P < 0.01). STING agonists enhance the efficacy of atezolizumab in BCa immunotherapy by activating the IFN-β signaling pathway.
Introduction
Breast cancer (BCa) is a main malignant tumor that often occurs in women and is a leading cause of cancer-related deaths in women worldwide [Citation1]. Approximately 30% of all new diagnosed cancer cases are identified as BCa, and 15% of all cancer-related deaths in women are caused by BCa [Citation2]. Surgical resection, chemotherapy and radiotherapy are traditional treatment options for BCa. However, the mortality rate remains high because of metastasis and relapse caused by treatment resistance [Citation3].
Immunotherapy is new treatment strategy for solid tumors, and its emergence is gradually changing the traditional treatment concept of solid tumors [Citation4]. Programmed death-1 (PD-1) and programmed death-ligand 1 (PD-L1) are identified as immunotherapy targets for cancers [Citation5,Citation6]. PD-1 is expressed by activated T cells, whereas PD-L1 can be expressed by tumor cells [Citation6]. PD-1, which acts as a cell surface receptor, can suppress T cell activation by binding to PD-L1, thereby promoting immune escape of tumor cells [Citation7]. Studies have indicated that the blockage between PD-1 and PD-L1 can enhance the response of T cells to exert anti-tumor effects [Citation8,Citation9]. A study indicated that PD-1/PD-L1 antagonists had clinical activity in some patients with BCa [Citation4]. In 2019, atezolizumab (a type of PD-L1 monoclonal antibody) became the first immune checkpoint inhibitor approved specifically for BCa [Citation10,Citation11]. It greatly improved the progression-free survival and overall survival of patients with BCa and was approved for the treatment of patients with PD-L1-positive triple-negative BCa [Citation10,Citation12,Citation13].
Stimulator of interferon (IFN) genes (STING), which is considered a cytosolic pattern recognition receptor, is very important for the spontaneous induction of anti-tumor T cell immunity [Citation14]. STING activation can activate anti-tumor T cells by stimulating type I IFN-mediated inflammation programme [Citation15]. Therefore, STING activation may be an effective way to enhance the immunotherapy effects of tumors. Studies have found that a STING agonist can enhance the immunotherapy effects of BCa [Citation16] and normalize the BCa microenvironment, which enhances the regression of immunotherapy-resistant BCa in the presence of immune checkpoint blockade [Citation17]. However, whether STING agonists can enhance the efficacy of atezolizumab in BCa is still rarely reported. Therefore, this study examined the function of STING agonists in BCa treated with atezolizumab. Importantly, the mechanism involved in this process was also explored. This study provides solid theoretical basis for BCa treatment by atezolizumab in clinical settings.
Methods
Clinical sample collection
In this study, a total of 37 BCa tumor tissues and paired adjacent normal tissues were collected. All patients were firstly diagnosed with BCa, without previous history of cancer-related diseases or treatment. The clinical tissues were stored at −80°C in a refrigerator.
This study was approved by the ethics committee of Anhui No. 2 Provincial People’s Hospital and complied with the Declaration of Helsinki. All patients signed written informed consent and voluntarily participated in the study.
Cells and culture
Mice BCa cell line (4T1) was commercially provided by the American Type Culture Collection (ATCC, Manassas, VA, USA). The cell line was cultured at 37°C and 5% CO2 in Dulbecco’s modified Eagle medium (DMEM) containing 10% fetal bovine serum (FBS).
Animals and construction of tumor-bearing mouse model
The animal study was approved by the animal ethics committee of Anhui No. 2 Provincial People’s Hospital. Female Balb/c mice (n = 20, 5 weeks old) were purchased from the Shanghai Laboratory Animal Center of Chinese Academy of Sciences (Shanghai, China). The animals were housed in a room with (22 ± 1)°C and 12-h day/night cycle. Food and water were freely available. The animals were randomly divided into four groups: vehicle, STING agonist, atezolizumab and STING agonist + atezolizumab groups. Each group included five mice. In this study, the STING agonist was cyclic diguanylate (c-di-GMP) (CAS number: 61093–23-0; MedBio, Shanghai, China). Treatments in each group were as follows:
First, 4T1 cells were collected when the confluence reached approximately 85%. Phosphate-buffered saline (PBS) was used to disperse 4T1 cells at a density of 1 × 107 cells/mL. Subsequently, 4T1 cell suspension (100 μL) was injected into the mammary fat pad of the mice in each group. Five days after 4T1 cell injection, a STING agonist (25 μg) was injected into the tumor mass of the mice in the STING agonist group. At the same time point, the PBS group was injected with a PBS vehicle. The atezolizumab group was injected intraperitoneally with atezolizumab (200 μg), whereas the STING agonist + atezolizumab group was injected not only with STING agonist (25 μg) into the tumor mass but also with atezolizumab intraperitoneally (200 μg). The injection frequency of the STING agonist and atezolizumab was twice a week for 2 weeks.
Furthermore, on days 1, 7 and 14 after injections of PBS, STING agonist, atezolizumab or STING agonist + atezolizumab, peripheral blood samples of mice in each group were collected from the orbit and stored at −80°C in a refrigerator. Peripheral blood samples were collected 4 h after injection.
From day 5 of 4T1 cell injection, the tumor volume was measured every 3 days with the following formula: V = 0.5 × length × width2. On day 29 after 4T1 cell injection, the mice were anaesthetised with 2% isoflurane and then sacrificed through cervical dislocation. The tumor mass was collected from the mice and stored at −80°C in a refrigerator [Citation18,Citation19]. In addition, the lung, liver, brain cortex and kidney of mice were collected and stored at −80°C in a refrigerator.
Anti-interferon alpha receptor (IFNAR1) treatment of 4T1 cells
When 85% confluence was reached, 4T1 cells were harvested and dispersed into DMEM containing 10% FBS (1 × 106 cells/mL). Subsequently, 1 mL of cell suspension was added to each well. Thereafter, the STING agonist was added into 4T1 cells to a final concentration of 100 μg/mL (STING agonist group). The STING agonist (final concentration, 100 μg/mL) and anti-IFNAR1 (final concentration, 10 ng/mL) were both used to treat 4T1 cells (STING agonist + anti-IFNAR1 group). 4T1 cells treated by PBS (final concentration, 100 μg/mL) were used as the vehicle group. Cells were cultured for 48 h at 37°C and 5% CO2 and were then collected for Western blot.
Enzyme-linked immunosorbent assay
The tumor mass of tumor-bearing mice was homogenized at 15,000 rpm for 40s, followed by centrifugation at 1000 rpm for 5 min, and the supernatant was collected. Using an enzyme-linked immunosorbent assay (ELISA) kit, the levels of TNF-α, IFN-β, IL-10 and IFN-γ in the peripheral blood samples and the supernatant were measured according to the manufacturer’s instructions.
Quantitative reverse transcription polymerase chain reaction (qRT-PCR)
Clinical samples and tumor mass of tumor-bearing mice were prepared as tissue powders in liquid nitrogen. Total RNA in tissue powder samples was extracted using TRIzol reagent (Beyotime Biotech Inc., Shanghai, China). The extraction procedures strictly followed the instructions. Thereafter, cDNA was synthesized using 2 μg of total RNA samples according to the instructions of the Roche Transcriptor First Strand cDNA Synthesis kit (Roche, Mannheim, Germany). The cDNA was subjected to PCR using the ABI7500 instrument (Applied Biosystems, Waltham, MA, USA) in line with the following procedure: 95°C for 5 min, 38 cycles of 95°C for 45s, 50°C for 45s and 72°C for 40s and 72°C for 3 min. Genes and their corresponding primers are as follows:
STING: forward 5’-CAAGGACCAACTACAACC-3’, reverse 5’-TGCCTCTTCTTTAATTG-3’.
PD-1: forward 5′-GGTGTGAGGCCATCCACAA-3′, reverse 5′-CCATTCTGTCGGAGCCTCTG-3′.
PD-L1: forward 5′-TATGGTGGTGCCGACTACAA-3′, reverse 5′-TGGCTCCCAGAATTACCAAG-3′.
TNF-α: forward 5′-CCCGCATCCCAGGACCTCTCT-3′, reverse 5′-CGGGGGACTGGCGA-3′.
IFN-β: forward 5′-TCCGAGCAGAGATCTTCAGGAA-3′, reverse 5′-TGCAACCA-CCACTCATTCTGAG-3′.
IL-10: forward 5’-CTTCGAGATCTCCGAGATGCCTTC-3’, reverse 5’-ATTCTTCACCTGCTCCACGGCCTT-3’.
Actin: forward 5′-TCCTGTGGCATCCACGAAAC-3′, reverse 5′-GAAGCATTTGCGGACGAT-3′.
Actin was used as control to normalize the expression of other mRNA expressions using the 2−ΔΔCT method.
Immunohistochemical (IHC) analysis
Clinical samples and tumor mass of tumor-bearing mice were treated by 10% formaldehyde solution for 24 h. Paraffin was used to embed the tissues, which were then cut into sections (4 μm). The sections were sequentially treated by xylene and gradient alcohol for dewaxing and rehydration. Antigen retrieval was performed by immersing sections into citrate buffer (10 mM, pH = 6.0). After 10 min of treatment with 3% H2O2, the sections were treated for 1 h with 5% goat serum. Primary antibodies (1:100) were added to treat the sections for 12 h at 4°C. The primary antibodies were rabbit anti-STING (ab252560, Abcam, Shanghai, China), rabbit anti-PD-L1 (ab233482, Abcam), mouse anti-PD-1 (ab52587, Abcam), rabbit anti-CD8+ (ab138727, Abcam) and rabbit anti-FOXP3+ (ab4728, Abcam). Goat anti-rabbit (ab150080, Abcam) or anti-mouse (ab6789, Abcam) secondary antibodies (1:200) were then added and incubated for 2 h after treatment of the sections. Diaminobenzidine (Beijing Solarbio Science & Technology Co., Ltd., China) was used to stain the sections, followed by hematoxylin–eosin (HE) staining (Beijing Solarbio Science & Technology Co., Ltd.). Dehydration of sections was performed using gradient alcohol. The transparency of the sections was caused by xylene. After sealing in neutral resin, the sections were observed using a microscope.
HE staining
As described above, the lung, liver, brain cortex and kidney of the mice were treated by 10% formaldehyde solution for 24 h. Tissues embedded in paraffin were cut into sections (4 μm), followed by treatment with xylene and gradient alcohol for dewaxing and rehydration. According to the manufacturer’s instructions, HE (Beijing Solarbio Science & Technology Co., Ltd.) was sequentially used for staining the sections. Dehydration and transparency were achieved by using gradient alcohol and xylene. After sealing in neutral resin, the sections were observed using a microscope.
Western blot
The tumor mass of tumor-bearing mice was prepared in a powder form in liquid nitrogen to extract total proteins using lysis buffer (Beyotime Biotech Inc.) on ice. Furthermore, 4T1 cells were lysed by lysis buffer. Total proteins in the supernatant were collected after centrifugation at 5000 × g for 20 min. The concentration of total proteins was determined using a BCA kit (Beyotime Biotech Inc.) according to the instructions. Subsequently, 20 µg of protein sample was separated using 10% sodium dodecyl sulfate-polyacrylamide gel electrophoresis. After being separated, proteins were transferred onto a polyvinylidene fluoride (PVDF) membrane. For blocking, 5% skimmed milk was used to incubate the PVDF membrane for 1 h. Tris-buffered saline containing 0.1% Tween-20 (TBST) was used to wash the PVDF membrane. Afterward, the PVDF membrane was probed by rabbit anti-mouse primary antibodies for 12 h at 4°C. Rabbit anti-mouse primary antibodies used are as follows: p-STING (1:500, AF7416, Fushen Biotechnology, Shanghai, China), p-TBK1 (1:500, NY-1725 R-Phospho, Anyan Biotechnology, Nanjing, China), TBK1 (1:1000, ab227182, Abcam), p-IRF3 (1:500, ab192796, Abcam), IRF3 (1:1000, ab25950, Abcam), p-STAT1 (1:500, ab30645), STAT1 (1:1000, ab47425, Abcam) and actin (1:1000, ab8227, Abcam). TBST was used to rewash the PVDF membrane. Horseradish peroxidase-conjugated goat anti-rabbit secondary antibody (1:2000, ab6271, Abcam) was added to incubate the PVDF membrane for 2 h at room temperature. Enhanced chemiluminescent reagent (Beyotime Biotech Inc.) was used for protein blot visualization. The density of the protein bands was quantified using the ImageJ software (National Institutes of Health, Bethesda, MD, USA). Actin served as the control to normalize the expressions of other proteins.
Statistical analysis
SPSS version 19.0 (IBM Corp., Armonk, NY, USA) was used for statistical analysis. Data were expressed as mean ± standard deviation. Paired Student’s t-test and analysis of variance (followed by Tukey’s post-hoc test) were used for comparisons between two groups and multiple groups, respectively. A P-value < 0.05 was considered significant. All data were obtained from three independent repeated experiments.
Results
Low STING and high PD-1 and PD-L1 protein expressions in BCa tissues
IHC analysis was performed to detect STING, PD-1 and PD-L1 protein expressions in BCa tissues and paired adjacent normal tissues of BCa patients. Reduced STING-positive particles and increased PD-1- and PD-L1-positive particles were observed in BCa tissues as compared with paired adjacent normal tissues (). This result demonstrated the down-modulated STING and up-modulated PD-1 and PD-L1 in tumor tissues of BCa patients. Pearson’s correlation analysis indicated that the STING mRNA level was negatively correlated with PD-1 mRNA level or PD-L1 mRNA level in BCa tissues (P < 0.0001) ().
Figure 1. STING was downregulated and PD-1 and PD-L1 were upregulated in BCa tissues (a) Immunohistochemical analysis was performed to detect STING, PD-1 and PD-L1 protein expressions in BCa tissues and paired adjacent normal tissues of patients. (b) Pearson’s correlation analysis was conducted to examine the correlation between STING level and PD-1 or PD-L1 protein levels in BCa tissues. BCa, breast cancer; STING, stimulator of interferon gene; PD-1, programmed cell death; PD-L1, programmed death-ligand 1.
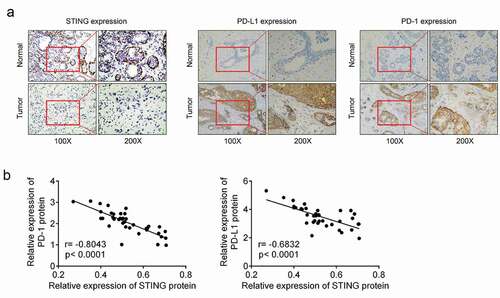
STING agonist inhibited BCa cell growth in mice and promoted IFN-β expression in the tumor mass of tumor-bearing mice
A tumor-bearing mouse model was constructed by injecting 4T1 cells into the mammary fat pad. STING agonist and PBS vehicle were respectively injected into the tumor mass. Data indicated that the STING agonist group showed prominently lower tumor volume than that of the vehicle group (P < 0.001) (). ELISA revealed that the tumor of the STING agonist group exhibited markedly higher IFN-β expression than that of the vehicle group (P < 0.001) (). Western blot indicated that the tumor of the STING agonist group showed significantly higher p-STING, p-TBK1/TBK1, p-IRF3/IRF3 and p-STAT1/STAT1 protein levels than those of the vehicle group (P < 0.001) (). All of these data implied that STING agonist could supress 4T1 cells growth in mice and activate IFN-β signaling pathway in the tumor mass of tumor-bearing mice.
Figure 2. STING agonist inhibited BCa cell growth in mice and promoted IFN-β expression in the tumor-bearing mouse model (a) The tumor volume was measured. (b) Enzyme-linked immunosorbent assay was used for the detection of IFN-β expression level in the tumor mass. (c) Western blot was applied to analyze protein expression in tumor mass. *** P < 0.001. STING, stimulator of interferon gene; PD-1, programmed cell death; IFN-β, interferon beta.
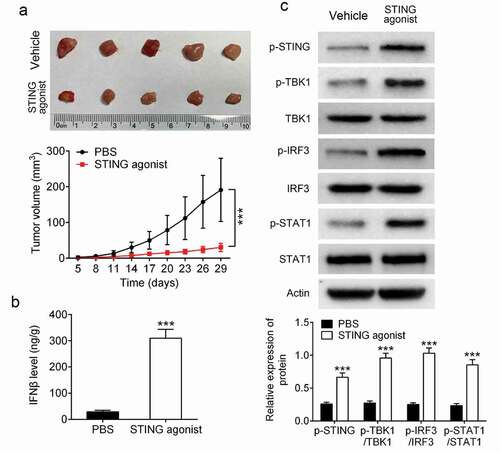
STING agonist synergized with atezolizumab to exert anti-tumor effects on the BCa mouse model
The tumorigenesis ability of 4T1 cells in mice was examined. As demonstrated in , in comparison with the vehicle group, the STING agonist and atezolizumab groups had decreased tumor volumes (P < 0.001). It suggested that STING agonist and atezolizumab could suppress 4T1 cells growth in mice. Interestingly, the tumor volume was significantly reduced in the STING agonist + atezolizumab group compared with the STING agonist group (P < 0.001). Therefore, STING agonist synergized with atezolizumab to inhibit 4T1 cells growth in mice.
Figure 3. STING agonist synergized with atezolizumab exerts anti-tumor effect on the BCa mouse model (a) The tumor volume was measured. *** P < 0.001. (b) Enzyme-linked immunosorbent assay (ELISA) was performed to detect TNF-α, IFN-β and IL-10 levels in the peripheral blood on days 1, 7 and 14 after administration of STING agonist and atezolizumab. (c) The levels of TNF-α, IFN-β and IL-10 in the tumor mass were examined by ELISA. (d) TNF-α, IFN-β and IL-10 mRNA expressions in the tumor mass were explored by qRT-PCR. (e) IFN-γ level in mice blood samples and tumor tissues by qRT-PCR and ELISA. ** P < 0.01 vs. vehicle group. # P < 0.05 and ## P < 0.01 vs. STING agonist group. BCa, breast cancer; STING, stimulator of interferon gene; qRT-PCR, quantitative reverse-transcription polymerase chain reaction; TNF-α, tumor necrosis factor; IFN-β, interferon; IL-10, interleukin-10.
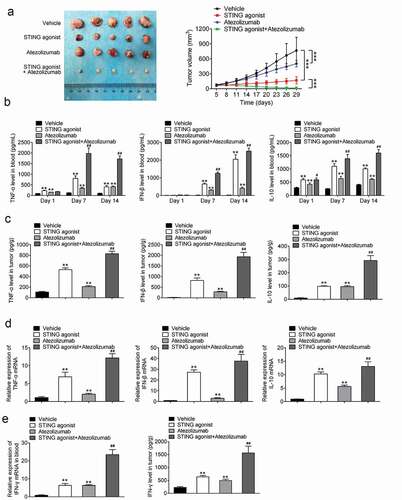
On days 1, 7 and 14 after the injection of the STING agonist and atezolizumab, peripheral blood of mice was collected from their orbits. The levels of TNF-α, IFN-β and IL-10 were measured using ELISA. As a result, compared with the vehicle group, the STING agonist and atezolizumab groups had higher TNF-α levels in the peripheral blood on days 1, 7 and 14 after administration (P < 0.01). On days 7 and 14 after administration, the STING agonist + atezolizumab group had much higher TNF-α level than that of the STING agonist group (P < 0.01). Moreover, the IFN-β level was markedly higher in the STING agonist and atezolizumab groups than in the vehicle group on days 7 and 14 after administration (P < 0.01). At the same time, a much higher IFN-β level was found in the STING agonist + atezolizumab group than in the STING agonist group (P < 0.01). In addition, compared with the vehicle group, the STING agonist and atezolizumab groups had remarkably higher IL-10 levels in the peripheral blood on days 1, 7 and 14 after administration. In addition, the STING agonist + atezolizumab group showed prominently higher IL-10 levels than that of the STING agonist group (P < 0.05 or P < 0.01) (). Hence, STING agonist synergized with atezolizumab to increase TNF-α, IFN-β and IL-10 level in peripheral blood of tumor-bearing mice.
The levels of TNF-α, IFN-β and IL-10 in the tumor were detected using ELISA. Much higher levels of TNF-α, IFN-β and IL-10 level were detected in the STING agonist and atezolizumab groups than in the vehicle group (P < 0.01). Unlike the STING agonist group, the STING agonist + atezolizumab group had significantly elevated levels of TNF-α, IFN-β and IL-10 (P < 0.01) (). The mRNA expressions of TNF-α, IFN-β and IL-10 in the tumor were further assessed using qRT-PCR. The data trend was similar to that shown in () (P < 0.01). Thereby, STING agonist synergized with atezolizumab to enhance TNF-α, IFN-β and IL-10 expression in tumor of tumor-bearing mice.
IFN-γ level in blood samples and tumor of tumor-bearing mice was monitored. As a result, matched to the vehicle group, much higher IFN-γ level was discovered in the blood sample and tumor mass of mice in the STING agonist and atezolizumab groups (P < 0.01). Relative to the STING agonist group, the IFN-γ level was pronounced elevated in the blood samples and tumor of mice in the STING agonist + atezolizumab group (P < 0.01) (). These data illustrated that STING agonist synergized with atezolizumab to promote IFN-γ expression in peripheral blood and tumor of tumor-bearing mice.
STING agonist synergized with atezolizumab to activate CD8+ cytotoxic T cells and suppress FOXP3+ Treg cells in tumor of the tumor-bearing mice
The levels of CD8+ cytotoxic T cells and FOXP3+ regulatory T (Treg) cells in the tumor mass of the tumor-bearing mice were investigated via IHC analysis. Results indicated that more CD8+ particles and less FOXP3+ Treg-positive particles were observed in the tumor-bearing mice of the STING agonist and atezolizumab groups as compared with the vehicle group. Compared with the STING agonist group, the STING agonist + atezolizumab group had increased CD8+ particles and decreased FOXP3+ Treg-positive particles in the tumor mass (). Thus, STING agonist synergized with atezolizumab to activate CD8+ cytotoxic T cells and suppress FOXP3+ Treg cells in tumor of the tumor-bearing mice.
Figure 4. STING agonist synergized with atezolizumab to activate CD8+ cytotoxic T cells and suppress FOXP3+ Treg cells in tumor of the tumor-bearing mice (a) CD8+ cytotoxic T cells and FOXP3+ Treg cells in the tumor mass were investigated by IHC. (b) The toxicity of the STING agonist and atezolizumab to the lung, liver, brain cortex and kidney of the mice was detected by hematoxylin and eosin staining. STING, stimulator of interferon gene.
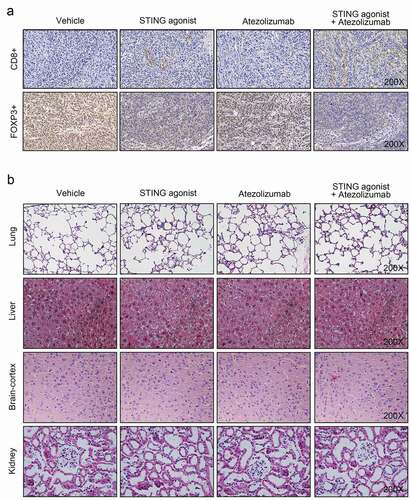
HE staining exhibited that the structures of the lung, liver, brain cortex and were comparable among the vehicle, STING agonist, atezolizumab and STING agonist + atezolizumab groups. The cell structure was complete, and tissues were arranged in an orderly manner (). Therefore, both STING agonist and atezolizumab were nontoxic to the lung, liver, brain cortex and kidney.
STING agonist exerted anti-tumor effects on the BCa mouse model by activating the IFN-β signaling pathway
STING agonist and anti-IFNAR1 were used to treat 4T1 cells in vitro. The phosphorylation levels of TBK1, IRF3 and STAT1 in 4T1 cells were investigated using Western blot. The results are displayed in . Compared with the cells of the vehicle group, 4T1 cells of the STING agonist group had remarkably higher p-TBK1/TBK1, p-IRF3/IRF3 and p-STAT1/STAT1 levels (P < 0.01). However, unlike the STING agonist group, 4T1 cells of the STING agonist + anti-IFNAR1 group had much reduced p-TBK1/TBK1, p-IRF3/IRF3 and p-STAT1/STAT1 levels (P < 0.01). Anti-IFNAR1 reversed the promotion of STING agonist on the IFN-β signaling pathway activity. Thus, STING agonist exerted anti-tumor effects on the BCa mouse model by activating the IFN-β signaling pathway.
Discussion
In this study, IHC analysis revealed STING downregulation and PD-1 and PD-L1 up-regulation in the clinical tissues of patients with BCa. Previous studies have been implied that STING agonist possessed well cancer immunotherapy effect [Citation20,Citation21]. Therefore, this study employed STING agonist to treat tumor-bearing mice in order to explore the availability of STING agonist in BCa treatment. The in vivo study demonstrated that the STING agonist reduced BCa cell growth in mice. In addition, the STING agonist synergized with atezolizumab to enhance anti-tumor efficacy in mice. The STING agonist and atezolizumab were nontoxic to the lung, liver, brain cortex and kidney of the BCa mouse model.
Specifically, the STING agonist synergized with atezolizumab to enhance the expressions of TNF-α and IL-10 in the peripheral blood and tumor mass of the BCa mice. BCa progression is promoted by inflammation driven by the tumor microenvironment [Citation22]. TNF-α can be expressed by tumor cells, which exerts a dual role in BCa progression [Citation22]. It acts as an essential pro-inflammatory cytokine in BCa [Citation23]. However, excessive TNF-α expression can suppress the proliferation and tumorigenesis of BCa cells [Citation24]. Therefore, in this study, the STING agonist synergized with atezolizumab to reduce BCa cell growth in mice through the overproduction of TNF-α. IL-10 is mainly secreted by activated T cells, which is an important anti-inflammatory cytokine [Citation25]. A study reported that a STING agonist promoted IL-10 expression to relieve mice with experimental autoimmune encephalomyelitis [Citation26]. This study indicated that the STING agonist synergized with atezolizumab to exert anti-tumor effects through the reduction of inflammation by increasing IL-10 expression.
The tumor microenvironment plays an important role in malignant progression, immune escape and treatment resistance [Citation27]. The existence of cytotoxic T cells in tumor lesions indicates a good prognosis [Citation28]. CD8+ cytotoxic T cells are considered preferred immune cells in tumor target treatment. During tumor progression, CD8+ cytotoxic T cells experience dysfunction and exhaustion because of immunosuppression [Citation29]. In a mice tumor model, a previous study reported that STING agonist treatment increased CD8+ cytotoxic T cells in tumor mass [Citation30]. In preclinical models, the STING agonist induced the local activation of CD8+ cytotoxic T cell in tumor lesions, thereby resulting in tumor regression and durable anti-tumor immunity [Citation31]. Recently, IL10 has been reported to directly modulating CD8 + T cells function by reactivating terminally exhausted CD8 + T cells, thereby to exerted antitumor effect [Citation32]. Our data revealed that STING agonist synergized with atezolizumab to reduce the number of FOXP3+ Treg cells in BCa tumor mass. FOXP3+ Tregs are important inhibitors of anti-tumor responses, which can maintain the immunological tolerance of host tissues [Citation33]. A high FoxP3+ Treg infiltration rate was associated with unfavorable prognosis of patients with tumor [Citation34]. It has been reported that STING agonist can suppress Tregs cells by activating the IFN signaling [Citation35]. Based on this, this research speculated that STING agonist might reduce FOXP3+ Treg cells by activating the IFN-β signaling. STING agonist and anti-IFNAR1 were then utilized to treat 4T1 cells in vitro. Results from revealed that, anti-IFNAR1 reversed the promotion of STING agonist on the phosphorylation of TBK1, IRF3 and STAT1 in 4T1 cells. As we know, the phosphorylation of TBK1, IRF3 and STAT1 are associated with the inactivation of the IFN-β signaling pathway [Citation36]. Therefore, this study firstly implied that STING agonist might reduce FOXP3+ Treg cells by activating the IFN-β signaling pathway. In BCa, CD8+ cytotoxic T cells and FoxP3+ Tregs are identified to be potential prognostic factors. High CD8+ cytotoxic T cell infiltration indicates a favorable prognosis, whereas high FoxP3+ Treg infiltration indicates a poor outcome of BCa [Citation37]. This study is novel because it demonstrated that a STING agonist synergized with atezolizumab to increase CD8+ cytotoxic T cells and decrease FoxP3+ Treg infiltration in the BCa tumor mass.
This study discovered that the STING agonist synergized with atezolizumab to increase the IFN-β and IFN-γ level in the peripheral blood and tumor mass of BCa mice. IFN-β was suggested to be used in BCa treatment because its stimulates cellular immunity [Citation38]. Patients with BCa with a high IFN-β level are associated with favorable clinical prognosis, such as the repressed cancer stem cell property [Citation39]. Regarding immunotherapy, IFN-β treatment can bridge the immune responses by targeting the tumor microenvironment [Citation40]. A previous study reported that STING agonists exerted anti-tumor effects on pancreatic cancer by activating the IFN-β pathway [Citation41]. IFN-γ possesses anti-proliferative, angiogenesis inhibition, as well as pro-apoptotic functions, which exerts important role in activate antitumor immune-response. Therefore, IFN-γ is regarded as a promising factor in adjuvant immunotherapy of multiple tumors [Citation42]. Furthermore, a STING agonist activated STING, TBK1, IRF3 and STAT1. In the tumor microenvironment, the activation of STING, TBK1, IRF3 and STAT1 had been reported to enhance the immune cell infiltration and the sensitivity of tumors to immune checkpoint blockade [Citation43,Citation44]. However, an in vitro study demonstrated that anti-IFNAR1 treatment significantly reversed the promoting effects of a STING agonist on the activation of TBK1, IRF3 and STAT1. Therefore, STING agonists might synergize with atezolizumab to enhance immunotherapy effects by activating the IFN-β signaling pathway.
Of course, this work has limitation. In this research, STING was monitored to be down-regulated in BCa tissues of patients. Based on previously reported anti-tumor effects of STING agonists, this study employed STING agonist to treat tumor-bearing mice in order to explore the availability of STING agonist in BCa treatment. It might be better to up-regulate STING first and then utilize STING agonist in BCa cells and tumor-bearing mice. However, due to laboratory limitations, these experiments cannot be carried out currently. This interesting issue will be the focus in our future research.
Conclusion
This study investigated the effects of a STING agonist on BCa immunotherapy by atezolizumab. The STING agonist exerted anti-tumor effects on BCa progression and enhanced atezolizumab immunotherapy efficacy by activating the IFN-β signaling pathway. Therefore, STING agonists can be used not only for BCa treatment but also in combination with atezolizumab to enhance the efficacy of immunotherapy.
Highlights
(1) Patients with BCa had low STING and high PD-1 and PD-L1 protein expressions.
(2) A STING agonist inhibited 4T1 cell growth and promoted IFN-β expression in mice.
(3) A STING agonist synergized with atezolizumab to inhibit 4T1 cell growth in mice.
(4) A STING agonist exerted anti-tumor effects on BCa by activating the IFN-β pathway.
Data availability
The datasets generated during and/or analyzed during the current study are available from the corresponding author on reasonable request.
Authors’ contributions
Mingming Yin, Jinlong Hu, Wenyong Wu, and Zhiqi Hu designed the study. All author performed the experiments. Jinlong Hu, Wenyong Wu, and Zhiqi Hu analyzed the data. Mingming Yin and Wenyong Wu wrote the manuscript. All authors read and approved the final manuscript.
Ethical approval
This research has been approved by the ethics committee of Anhui No. 2 Provincial People’s Hospital and complied with the Declaration of Helsinki. All patients have signed written informed consent and voluntarily joined the study.
Supplemental Material
Download Zip (2.1 MB)Acknowledgments
We thank the experts of department of pathology in Anhui No. 2 Provincial People’s Hospital for the help of the immunohistochemical analysis.
Supplementary material
Supplemental data for this article can be accessed here
Disclosure statement
No potential conflict of interest was reported by the author(s).
Additional information
Funding
References
- Zhu Q, Zhang X, Zai H-Y, et al. circSLC8A1 sponges miR-671 to regulate breast cancer tumorigenesis via PTEN/PI3k/Akt pathway. Genomics. 2021;113(1):398–410.
- Wm Nor W, Chung I, Said N. MicroRNA-548m Suppresses Cell Migration and Invasion by Targeting Aryl Hydrocarbon Receptor in Breast Cancer Cells. Oncol Res. 2021;28(6):615–629.
- Yuan X, Qian N, Ling S, et al. Breast cancer exosomes contribute to pre-metastatic niche formation and promote bone metastasis of tumor cells. Theranostics. 2021;11(3):1429–1445.
- Emens LA. Breast Cancer Immunotherapy: facts and Hopes. Clin Cancer Res. 2018;24(3):511–520.
- Tsoukalas N, Kiakou M, Tsapakidis K, et al. PD-1 and PD-L1 as immunotherapy targets and biomarkers in non-small cell lung cancer. J Buon. 2019;24(3):883–888.
- Dermani FK, Samadi P, Rahmani G, et al. PD-1/PD-L1 immune checkpoint: potential target for cancer therapy. Journal of Cellular Physiology. 2019;234(2):1313–1325.
- Jiang Y, Chen M, Nie H, et al. PD-1 and PD-L1 in cancer immunotherapy: clinical implications and future considerations. Human Vaccines & Immunotherapeutics. 2019;15(5):1111–1122.
- Li C, Zhang N, Zhou J, et al. Peptide Blocking of PD-1/PD-L1 Interaction for Cancer Immunotherapy. Cancer Immunol Res. 2018;6(2):178–188.
- Nowicki TS, Hu-Lieskovan S, Ribas A. Mechanisms of Resistance to PD-1 and PD-L1 Blockade. Cancer J. 2018;24(1):47–53.
- Reddy SM, Carroll E, Nanda R. Atezolizumab for the treatment of breast cancer. Expert rev anticancer ther. 2020;20(3):151–158.
- Herbst RS, Giaccone G, de Marinis F, et al. Atezolizumab for First-Line Treatment of PD-L1-Selected Patients with NSCLC. N Engl J Med. 2020;383(14):1328–1339.
- Noguchi E, Shien T, Iwata H. Current status of PD-1/PD-L1 blockade immunotherapy in breast cancer. Jpn J Clin Oncol. 2021;51(3):321–332.
- Heimes AS, Schmidt M. Atezolizumab for the treatment of triple-negative breast cancer. Expert Opin Investig Drugs. 2019;28(1):1–5.
- Shae D, Becker KW, Christov P. Endosomolytic polymersomes increase the activity of cyclic dinucleotide STING agonists to enhance cancer immunotherapy. Nature Nanotechnology. 2019;14(3):269–278.
- Corrales L, McWhirter SM, Dubensky TW, et al. The host STING pathway at the interface of cancer and immunity. J Clin Investig. 2016;126(7):2404.
- Chen YP, Xu L, Tang TW, et al. STING Activator c-di-GMP-Loaded Mesoporous Silica Nanoparticles Enhance Immunotherapy Against Breast Cancer. ACS Appl Mater Interfaces. 2020;12(51):56741–56752.
- Yang H, Lee WS, Kong SJ, et al. STING activation reprograms tumor vasculatures and synergizes with VEGFR2 blockade. J Clin Invest. 2019;129(10):4350–4364.
- Abd Razak N, Yeap SK, Alitheen NB. Eupatorin Suppressed Tumor Progression and Enhanced Immunity in a 4T1 Murine Breast Cancer Model. Integrative Cancer Therapies. 2020;19:1534735420935625.
- Roda E, De Luca F, Di Iorio C, et al. Novel Medicinal Mushroom Blend as a Promising Supplement in Integrative Oncology: a Multi-Tiered Study using 4T1 Triple-Negative Mouse Breast Cancer Model. Int J Mol Sci. 2020;21(10):3479.
- Motedayen Aval L, Pease JE, Sharma R, et al. Challenges and Opportunities in the Clinical Development of STING Agonists for Cancer Immunotherapy. J Clin Med. 2020;9(10):3323.
- Go EJ, Yang H, Chon HJ, et al. Combination of Irreversible Electroporation and STING Agonist for Effective Cancer Immunotherapy. Cancer. 2020;12(11):3123.
- Cruceriu D, Baldasici O, Balacescu O. The dual role of tumor necrosis factor-alpha (TNF-α) in breast cancer: molecular insights and therapeutic approaches. Cell oncol (Dordrecht). 2020;43(1):1–18
- Ma Y, Ren Y, Dai Z-J, et al. IL-6, IL-8 and TNF-α levels correlate with disease stage in breast cancer patients. Adv Clin Exp Med. 2017;26(3):421–426.
- Wang K, Ren Y, Liu Y, et al. Tumor Necrosis Factor (TNF)-α-Induced Protein 8-like-2 (TIPE2) Inhibits Proliferation and Tumorigenesis in Breast Cancer Cells. Oncol Res. 2017;25(1):55–63.
- Fujio K, Yamamoto K, Okamura T. Overview of LAG-3-Expressing, IL-10-Producing Regulatory T Cells. Curr Top Microbiol Immunol. 2017;410:29–45.
- Johnson BM, Uchimura T, Gallovic MD, et al. STING Agonist Mitigates Experimental Autoimmune Encephalomyelitis by Stimulating Type I IFN-Dependent and -Independent Immune-Regulatory Pathways. J immunolo (Baltimore, Md.: 1950). 2021;206(9):2015–2028.
- Liu M, Zhou J, Chen Z, et al. Understanding the epigenetic regulation of tumours and their microenvironments: opportunities and problems for epigenetic therapy. J Pathol. 2017;241(1):10–24.
- van der Leun AM, Thommen DS. CD8(+) T cell states in human cancer: insights from single-cell analysis. Nat Rev Cancer. 2020;20(4):218–232.
- Farhood B, Najafi M, Mortezaee K. CD8(+) cytotoxic T lymphocytes in cancer immunotherapy: a review. Journal of Cellular Physiology. 2019;234(6):8509–8521.
- Ghaffari A, Peterson N, Khalaj K, et al. STING agonist therapy in combination with PD-1 immune checkpoint blockade enhances response to carboplatin chemotherapy in high-grade serous ovarian cancer. Br J Cancer. 2018;119(4):440–449.
- Sivick KE, Desbien AL, Glickman LH, et al. Magnitude of Therapeutic STING Activation Determines CD8(+) T Cell-Mediated Anti-tumor Immunity. Cell Rep. 2018;25(11):3074–3085.e5.
- Guo Y, Xie YQ, Gao M. Metabolic reprogramming of terminally exhausted CD8(+) T cells by IL-10 enhances anti-tumor immunity. Nature Immunology. 2021;22(6):746–756.
- Sakaguchi S, Yamaguchi T, Nomura T, et al. Regulatory T cells and immune tolerance. Cell. 2008;133(5):775–787.
- Shang B, Liu Y, Jiang S-J, et al. Prognostic value of tumor-infiltrating FoxP3+ regulatory T cells in cancers: a systematic review and meta-analysis. Sci Rep. 2015;5(1):15179.
- Ohkuri T, Ghosh A, Kosaka A, et al. STING contributes to antiglioma immunity via triggering type I IFN signals in the tumor microenvironment. Cancer Immunol Res. 2014;2(12):1199–1208.
- Palmer C, Facchini FA, Jones RP, et al. Synthetic glycolipid-based TLR4 antagonists negatively regulate TRIF-dependent TLR4 signalling in human macrophages. Innate immun. 2021;27(3):275–284.
- Peng GL, Li L, Guo Y-W, et al. CD8(+) cytotoxic and FoxP3(+) regulatory T lymphocytes serve as prognostic factors in breast cancer. Am J Transl Res. 2019;11(8):5039–5053.
- Carpi A, Nicolini A, Antonelli A, et al. Cytokines in the management of high risk or advanced breast cancer: an update and expectation. Curr Cancer Drug Targets. 2009;9(8):888–903.
- Doherty MR, Parvani JG, Tamagno I, et al. The opposing effects of interferon-beta and oncostatin-M as regulators of cancer stem cell plasticity in triple-negative breast cancer. Breast Cancer Res. 2019;21(1):54.
- Yang X, Zhang X, Fu M, et al. Targeting the tumor microenvironment with interferon-β bridges innate and adaptive immune responses. Cancer Cell. 2014;25(1):37–48.
- Ren D, Qin G, Zhao J, et al. Metformin activates the STING/IRF3/IFN-β pathway by inhibiting AKT phosphorylation in pancreatic cancer. Am J Cancer Res. 2020;10(9):2851–2864.
- Jorgovanovic D, Song M, Wang L, et al. Roles of IFN-γ in tumor progression and regression: a review. Biomark Res. 2020;8(1):49.
- Sen T, Rodriguez BL, and Chen L, et al. Targeting DNA Damage Response Promotes Antitumor Immunity through STING-Mediated T-cell Activation in Small Cell Lung Cancer. 2019;9(5): 646–661 .
- Zemek RM, and De Jong E. Sensitization to immune checkpoint blockade through activation of a STAT1/NK axis in the tumor microenvironment. Sci Transl Med. 2019;11(501): eaav7816.