ABSTRACT
Radiotherapy has an essential role in breast cancer treatment. However, tumor cells may be resistant to radiotherapy. Noncoding RNAs are considered regulators of different pathways which modulate radiotherapy. This systematic review classifies long noncoding RNAs, and microRNAs precipitated in the radiation response of breast cancer patients. A total of 14 microRNAs and 8 long noncoding RNAs were studied in this review. MiR-22, miR-200 c, Let7, and LINP1 as tumor suppressors increase the effect of radiotherapy in BC. However, some noncoding RNAs such as HOTAIR, NEAT1, and miR-21 are precipitated in radio-resistance breast cancers. Significant changes in the pattern of noncoding RNAs expression before and after radiotherapy make them a good candidate for the prognosis and prediction of radiotherapy response. MiR-21 and miR-182 can promote radio-resistance via cancer stem cells. At last, the molecular mechanisms initiating radio-resistance were also examined to find the candidate noncoding RNAs for the development of radiation-sensitized agents.
1. Introduction
Despite advances in cancer therapy, breast cancer (BC) is reported as the leading cause of cancer-related death among women worldwide. Adjuvant radiotherapy (RT) improves the BC patient’s overall survival by reducing recurrence and mortality after the surgery[Citation1]. RT is an essential mode of treatment in many types of breast tumors that confronts tumor progression and metastasis.
Ionizing radiation (IR) causes various responses including cell cycle arrest, differentiation, signal transduction, mutations, altered gene expression, genomic instability, and many cellular structures damages [Citation2]. Radiation-induced DNA damage usually results in double-strand breaks (DSBs), prevents tumor cells proliferation, and initiates a DNA damage response (DDR). Extensive DNA damage leads cells to undergo programmed cell death. The cellular response to IR exposure is complex and involves many cellular pathways [Citation2,Citation3].
Studies show that the biological heterogeneity of tumors may fail RT [Citation4]. In these cases, tumors often have or acquire radio-resistance characteristics that enable them to escape radiation-induced cell death. So, the investigation of responsible factors for radiation response has become an essential issue in the cancer field [Citation5]. Although there are particular panels predicting chemotherapy benefits in BC, limited similar panels are available for RTs. For example, Oncotype DX can also predict the benefit of RT in some groups of BC. But, it cannot predict the resistance or response to RT [Citation6,Citation7]. Personalized radiotherapy and biomarker-based treatment are essential in cancer medicine. So, we need reliable predictors of radiation response or resistance, and noncoding RNAs (ncRNAs) may have promising roles for this purpose. It is critical to know radiation-related cellular events to improve the efficiency of RT and enable more individualized treatment plans[Citation1]. Tumor heterogeneity, due to the different genetic profile or presence of different cell subpopulations such as cancer-initiating cells (CICs) or cancer stem cells (CSCs), plays a major role in response to cancer therapy [Citation8]. Moreover, the molecular mechanisms initiating radio-resistance should be understood to develop radiation-sensitizing agents. As a result, ncRNAs are implicated in the regulation of various cellular and biological processes such as cell differentiation, proliferation, cell cycle regulation, and metastasis. Long noncoding RNAs (lncRNAs) and microRNAs (miRNAs) are two important types of ncRNAs.
Recent studies highlight the participation of miRNAs in the growth, metastasis, and tumor microenvironment which affect the radioresponse [Citation9]. MiRNAs are potential biomarkers; particularly in BC. Circulating miRNAs can be found in blood, plasma, serum, and saliva [Citation10]. In BC, several tumor suppressors or oncogene miRNAs have been recognized as critical regulators of CSCs [Citation11].
LncRNAs could affect radio-resistance by controlling DNA damage repair and apoptosis [Citation12].In recent years, awareness regarding lncRNAs has been raised, and it seems they could be a promising predictor of treatment response in the future.
Choosing appropriate doses and the number of RT sessions according to the specific BC biomarkers may prevent treatment failure. Developing a profile of miRNAs or lncRNAs related to RT response in BC could be considered in the liquid biopsy. The present study systematically reviews miRNAs and lncRNAs responsible for molecular and cellular mechanisms of radioresponse in BC ().
Table 1. The roles of ncRNAs in breast cancer
2. Method
2.1. Literature search
Appropriate studies were sought in PubMed, Scopus, and Cochrane databases; the end of the search date was August 2020. The following standardized terms were used: breast cancer AND microRNA as well as other synonyms: miR OR miRNA AND cancer stem cells (CSCs OR BCSCs) AND radiotherapy AND radio-resistance AND radiosensitivity. Long noncoding RNA OR lncRNAs, Noncoding RNAs OR ncRNAs were also searched. Studies were searched in English databases. After omitting duplicates, irreverent articles were removed based on title and abstract.
2.2. Selection process
A PRISMA chart of the literature is shown in . According to the criteria eligibility, 220 studies were identified. After removing duplicates, 85 abstracts were reviewed. Articles that did not simultaneously study breast cancer, radiotherapy and ncRNA were removed as irrelevant articles. Twenty-two articles were included in the systematic review. All the research investigating ncRNA and radiotherapy in breast cancer was included. The manuscripts may study cell lines or/and clinical samples.
3. Results
Due to an insufficient number of articles on a specific ncRNA and different types of biological samples included in studies, a meta-analysis was not performed, and only a systematic review was conducted.
3.1. MiRNAs and radioresponse
The cellular response to IR exposure is complex and involves many pathways [Citation3]. It is shown that multiple factors can influence RT, and among them, tumor genetic and epigenetic background may have an important role. One of the most significant modifiers of gene expression is miRNAs which are provided with a quick response to environmental changes. MiRNAs are evolutionarily conserved small ncRNA molecules (∼22 nt), which are involved in both negative and positive regulation of gene expression by post-transcriptional regulation of numerous different target mRNAs [Citation2,Citation13]. Most miRNAs are transcribed as primary (pri)-miRNAs by RNA polymerase II after being processed by the DROSHA-DGCR8 complex within the nucleus. Then, (pre)-miRNA is exported from the nucleus to the cytoplasm. In the cytoplasm, the DICER-TRBP complex degrades the non-incorporated passenger strand of miRNA. The remaining mature guide strand of the miRNA is assembled into the RNA-induced silencing complex (RISC) that includes the catalytic Argonaute proteins such as AGO2. MiRNAs bind the miRNA response elements (MREs) sequences to the 3ʹUTR of target mRNAs, followed by degradation/inhibition of mRNA molecules.
Targeted genes could be silenced from several distinct mechanisms. MiRNA can function as either oncogene or tumor suppressor and influence signaling pathways that could alter multiple cellular processes [Citation14]. MiRNAs have been shown to play important roles in the regulation of cell proliferation, cell death, tumorigenesis, and cell damages. Many researchers exhibited that the stress induced by IR could adjust the expression of miRNAs [Citation13]. The microarray technology, real-time quantitative PCR, and next-generation deep sequencing are used to measure the relative expression of miRNAs; following exposure to IR [Citation2]. Their results indicated various miRNA expression changes in BC tumors in the responses to RT [Citation14]. Also, in response to IR, malignant breast cells expressed miRNAs differently from normal breast cells. Based on recent studies, miRNAs could modify signaling pathways after RT. In this way, miRNAs have the potential to be a biomarker for predicting RT responses or be a therapeutic target for improving the efficacy of radiation treatment; especially in radio-resistance BC patients. MiRNAs that are shown in have been investigated in the radiotherapy of BC. In the following section, we are going to discuss some of the miRNAs involved in the RT of BC.
Figure 2. MiRNAs involved in radiotherapy of BC. After radiotherapy, miRNAs have effect on metastasis, growth and survival of BC cells by modulating their important targets in different signaling pathways.
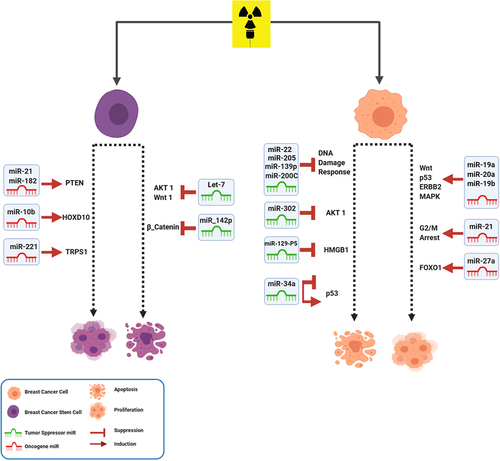
3.1.1. MiR-21
MiR-21 is a well-defined oncogenic miRNA related to cellular dysfunction, especially in apoptosis, cell cycle, and DNA damage repair [Citation15]. The upregulation of miR-21 after radiation is observed in different cell lines. Also, miR-21 overexpression is reported in almost all types of human cancers, including head and neck, breast, liver, pancreatic, and colorectal cancer [Citation16]. IR led to up-regulation of miR-21 through the activation of some oncogenic pathways such as epidermal growth factor receptor (EGFR) [Citation15]. MiR-21 is known to promote radio-resistance in various cancers; mainly BC. MiR-21 acts as an inhibitor in BC cells by inducing G2/M cell cycle arrest. Induction of DNA damage in the G2 checkpoint influences cell cycle progression. HALIMI et al. showed that miR-21 is increased in the serum of BC patients in response to IR [Citation15]. Anti-miR-21 can unblock G2/M arrest followed by apoptosis induction after radiation treatment. Additionally, inhibition of miR-21 significantly increased TE-1 cell radiosensitivity by modifying PTEN protein expression [Citation15].
3.1.2. MiR-34a
MiR-34a is a member of the miR-34 family. Recently, it is reported that BC patients have higher serum miR-34a expression than healthy women [Citation17]. Mir-34a has both positive and negative regulatory effects on the p53 function. MiR-34a is down-regulated in various solid tumors, and its high levels often correlate with patients’ poor prognosis [Citation18]. In vitro and in vivo studies in different cell lines and tissues showed upregulation of miR-34a after IR in some cancers. It has been reported that high doses of IR (up to 400 Gy) elevate miR-34a levels in BC cells [Citation19]. This upregulation leads to reduced radio-resistance. MiR-34a is also up-regulated after X-ray irradiation in p53 positive in both normal and BC cell lines. This result highlights the involvement of miR-34a in response to low-dose IR in BC cells. It is shown that miR-34 could enhance RT response in p53- mutant BC cells [Citation20]. It could be beneficial to use miR-34 mimics in BC patients to improve the effect of RT.
3.1.3. MiR-200 c
Recent studies indicated that miR-200 c; a member of the miR-200 family, could inhibit migration, invasion, tumorigenicity, and chemo-resistance. MiR-200 c can act as a predictor of response to RT in tumor progression [Citation21]. Downregulation of miR-200 c is associated with radio-resistance in BC cells. Its ectopic expression enhances radiosensitivity via different mechanisms. A study on BC cells with a higher expression of miR-200 c showed greater radiosensitivity [Citation22]. Furthermore, a higher level of miR-200 c could sensitize cancer cells to radiation by targeting TBK1 and VEGF-VEGFR2; resulting in apoptosis [Citation23]. Investigation of the level of miR-200 c before the RT procedure could help candidate patients benefit from this treatment.
3.1.4. Let-7 Family
The earliest identified family of ncRNAs was the Let-7 family. Let-7 had been previously reported to be significantly downregulated in BC. The Let-7 family regulates multiple cellular processes; specifically cell division and DNA repair pathways. This family of miRNAs regulates RAS oncogene expression and is downregulated in many cancers [Citation24]. This downregulation causes poor outcomes in most types of cancers. Let-7a and Let-7b expression increase following exposure to IR. It is shown that Let-7d transfection combined with 2 Gy radiation may increase the inhibition of the self-renewal ability of the two BC cell lines [Citation25,Citation26]. Also, restoration of Let-7d may inhibit cancer proliferation and metastasis and increase the sensitization to chemotherapy and RT. Wang L et al. demonstrated that the upregulation of Let-7 enhanced the radiation sensitivity in BC cells compared with the control cells. Based on their results, Lin28, which encodes a Lin28 family RNA-binding protein, induces radio-resistance by downregulation of Let-7 miRNA [Citation25]. Using Let-7 family and other miRNAs as a liquid biopsy for ascertaining RT dosage regimes could help treat BC patients.
3.1.5. MiR-17-92 cluster
Expression levels of the miR-17-92 cluster, particularly miR-19a, miR-20a, and miR-19b have obvious incensement in the MDA-MB-361 cell line after undergoing RT. This cluster has oncogenic role in tumorigenesis by regulating cell survival, proliferation, differentiation, and cell cycles. The expression levels of the miRNAs in the miR-17-92 cluster were gradually increased in a dose-dependent manner in BC cells after RT. In silico approach showed that target genes of the miR-17-92 cluster had a leading role in several radiation-related pathways, including the mitogen-activated protein kinase (MAPK), ErbB, p53, and Wnt [Citation27]. Targeting these oncomiRs and suppressing them with anti-miRNA oligonucleotides (AMOs) might help improve RT response.
3.2. MicroRNAs related to cancer stem cells and radio responses
One of the critical roles of miRNAs is cancer recurrence. MiRNAs are closely connected with the formation and development of breast cancer stem cells (BCSCs) [Citation11]. BCSCs are a subpopulation of cancer cells that are known to possess high tumorigenicity. In 2003, Al-Hajj and colleagues reported for the first time that BC could originate from BCSCs. They identified and isolated a small subset of cells within primary BC cell lines that expressed CD44 or CD44 and epithelial-specific antigen (ESA), but low or negative CD24. Some of the CSCs markers, such as CD44, CD29 (integrin β1), and CD9 (MRP-1) are expressed on both stem cells and normal tissue cells [Citation28,Citation29]. Pre-clinical data suggested that BCSCs were enriched after radiation. Among BCSCs, the CD44+ CD24-/low BCSCs are more radio-resistant than other types. It is shown that miRNAs are important in BCSCs by their essential roles as oncogenes or tumor suppressors; depending on their target genes [Citation30,Citation31].
It is shown that several miRNAs affect the apoptotic pathways and metastasis in BCSCs. Dysregulation of apoptosis, followed by improper expression of miRNAs in BCSCs, leads to metastasis and chemo/radio-resistance. A complex of miRNAs is uniquely expressed among BCSCs. Some of these miRNAs belong to the critical clusters including miR‐200 and Let‐7 family [Citation26,Citation32].
The Let-7 family as a tumor suppressor has an inhibitory role in the self-renewal of BCSCs. Let-7d modulates inhibition of BCSC’s renewal after RT in triple-negative breast cancer (TNBC) cell lines. It is shown that Let-7 affects the Akt1/Wnt1 pathway in BCSCs that is contributed to the suppression of self-renewal and elimination of the tumor [Citation26].
BCSCs have a powerful DNA repair system followed by cancer treatment. Repairing damages make these cells to be chemo/radio-resistance. It is shown that fewer DSBs are accumulated in BCSCs in response to irradiation [Citation8].
It is shown that miR-142-3p decreases the radio-resistance of BCSCs cells by alteration in the DDR pathways. MiR-142-3p regulates some essential genes such as Bod1 and BRCA1 and increases the sensitivity of BCSCs to different doses of radiation [Citation30]. A study on BC patients’ blood samples demonstrated a group of oncomiRs with altered expression after RT. MiR-21, miR-182, and miR-10b have increased after RT in ki67 positive patients. According to this research, miR-210 and miR-221 are up-regulated after IR; especially in p53-positive patients. These oncomiRs also show upregulation in different BCSCs. However, miR-142; as a tumor suppressor, has downregulated through RT. As we mentioned above, different expression patterns of miRNAs are shown between BCSCs and cancer cells [Citation33]. Regarding the changes in the miRNA profile in BCSCs, one could think of using miRNA therapeutics to eradicate this population of cells; resulting in an improved outcome in clinical settings.
3.3. LncRNAs and radiotherapy responses
The lncRNAs are between 200 to100 kb in lengths without open reading frames (ORFs). Based on their characteristics, they are divided into intergenic, intronic, bidirectional, overlapping sense, antisense, and miRNA cluster [Citation34]. LncRNAs participate in the biological pathway through transcriptional, post-transcriptional, and epigenetic mechanisms.
They could be a signal or decoy to enhance or suppress gene expression. They can also regulate gene expression by acting as scaffold molecules. They also sponge miRNA and reverse the effects of miRNA in gene regulation. The roles of lncRNAs in cell proliferation and apoptosis make them an exciting subject in cancer studies. It is shown that lncRNAs affect different treatment responses in cancer; especially radiotherapy. Radio-resistance causes recurrence and metastasis in cancer patients leading to death. The molecular mechanisms initiating radio-resistance should be understood to develop radiation-sensitizing agents.
Moreover, unraveling the expression patterns of lncRNAs may lead to prognostic or predictive patterns for radiotherapy. Most of the studies in this field are in triple-negative breast cancer (TNBC) [Citation35]. In BC, the triple-negative subtype has the worse outcome due to a lack of proper targets for therapy. So. It is expected that increasing the sensitivity to radiotherapy is a promising treatment option in the future. The studied lncRNAs in BC radiotherapy are summarized in .
Figure 3. LncRNAs involved in radiotherapy of BC. LncRNAs have important roles in the radiotherapy responses via regulating different oncogenes or tumor suppressors in BC cells.
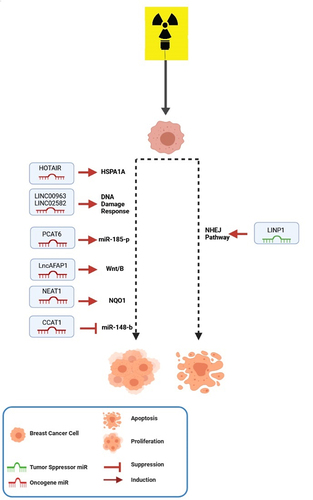
3.3.1. HOTAIR
HOTAIR is the most studied LncRNA in BC radio-resistance. Three studies investigated HOTAIR in BC patients [Citation36–39], but tissues were not radio-resistance or sensitive. They first found the significant expression of HOTAIR in BC tissue, and then cell line studies approved the role of HOTAIR in radiotherapy. However, meta-analysis was not possible. HOTAIR is transcribed from the antisense strand of the HOXC gene cluster. It is a crucial regulator of chromatin structure and is associated with growth, tumorigenesis, invasion, cancer stem cell differentiation, drug resistance, and metastasis in cancer [Citation40]. Studies showed that HOTAIR is expressed in metastatic BC tumors [Citation41]. Zhou et al. showed that HOTAIR could enhance radio-resistance in TNBC cells; proposing it as a therapeutic target to reverse radio-resistance in those cells. They also showed that its expression could predict radio-resistance risk in BC [Citation38]. Knock-down of HOTAIR in response to radiation inhibits apoptosis and cell survival. It also increases radiosensitivity through up-regulation of miR218 [Citation36].
LncRNAs can act as post-transcriptional regulators in radiation response in different cancers. For example, HOTAIR can also recruit epigenetic regulating factor EZH2, to MYC promoter; resulting in BC cell survival [Citation37]. Moreover, it is positively associated with heat shock protein family A (Hsp70) member 1A (HSPA1A) in irradiated BC patients. HOTAIR acts as a competing sponge for miR‐449b‐5p and thereby stops the miR‐449b‐5p‐mediated HSPA1A repression. As a result, radio-resistance in BC cells will be increased. However, HSPA1A knock-down or miR‐449b‐5p over-expression revokes HOTAIR‐related radio-resistance in-vivo and in-vitro. This finding is a promising perspective to overcome radio-resistance in the future [Citation39].
3.3.2. Other LncRNAs
LncRNAs in non-homologous end-joining pathway 1 (LINP1) increased triple-negative BC cells’ sensitivity to radiotherapy, augmenting the non-homologous end joining (NHEJ) pathway via DSB repair by acting as a scaffold that links two essential proteins in the pathway (Ku80, PKSs) [Citation42].
Colon cancer-associated transcript 1 (CCAT1) and its related miRNA; miR-148-b, were up and down-regulated, respectively, in 40 radio-resistant breast cancer patients. Mechanistic experiments showed that CCAT1 down-regulation and miR148-b up-regulation decrease invasiveness of the tumor cells. At the same time, it increases apoptosis and radiosensitivity [Citation43].
LINC00963 up-regulation was seen in BC; enhancing proliferation and tumorigenesis. Its knock-down sensitizes cancer cells to radiotherapy via enhancing DNA damage and oxidative stress. In-vitro studies showed a miR-324-3p response element within LINC00963 RNA which causes its down-regulation via miR-324-3p. Also, up-regulation of miR-324-3p sensitized BC cells to radiotherapy. It seems that activated Cdc42-associated kinase1 (ACK1) as a direct target of miR-324-3p will increase in the presence of LINC00963; resulting in radio-resistance [Citation44].
These ncRNAs could also be served as future targeted therapy for radio-resistance BC [Citation44]. Down-regulation of LINC02582 increases BC radiosensitivity, deubiquitinating, and stabilizing checkpoint kinase 1 (CHK1) in the DDR pathway. Radio-resistance enhancement occurs by the interaction between LINC02582 and deubiquitinating enzyme ubiquitin-specific peptidase 7 (USP7) augment. LINC02582 acts as a target for mir200c and links it to CHK1. So, mir200c increases radiosensitivity and can act as a radiosensitizer [Citation22].
Prostate cancer-associated transcript 6 (PCAT6) plays oncogenic roles in different cancer, and its expression is correlated with the aggressive nature of the tumor [Citation45]. The expression of this LncRNA is increased in TNBC tissue. PCAT6 sponge miR-185-p leads to up-regulation of TPD52 oncogene as a target of miR-185-p; explaining its aggressive effect in cancer. So, targeting PCAT6 might be a new therapeutic target to enhance radiosensitivity in TNBCs.
3.3.3. LncRNAs and therapeutic options
The potential power of lncRNAs in the treatment of cancer is inevitable. So, insight through the delivery of treatment choices such as nanoparticle delivery is increasing. Systemic delivery of LncAFAP1s antisense reverses the radio-resistance in BC cells. This nanoparticle’s reduction responsive nature synergically reduces the glutathione (GSH) effect produced under radiation conditions. LncAFAP1 activates the Wnt/β-catenin signaling pathway in TNBC, and its silencing through siRNA sensitizes these cells to radiotherapy [Citation46].
The use of other therapeutic options like Crisper-cas9 is increasing as well. Antioxidant molecules and enzymes, including superoxide dismutase or glutathione reductase, are responsible for the radio-resistance of cancer cells. Treatments involving their inhibition will sensitized cancer cells to radiotherapy. It is showed that quinone oxidoreductase 1 (NQO1) is increased in radio-resistant TNBC. LncRNA NEAT1 positively regulates the NQO1 expression in radio-resistant TNBC cells. NEAT1 positively regulates NQO1 in radio-resistance TNBC cells. Knock-down of NEAT1 by the Crisper-cas9 method sensitizes TNBC to radiotherapy. Moreover, some bioactive compounds can inhibit NQO1 and radiosensitizing cancer cells [Citation47].
4. Discussion
Here, we had summarized recent studies about miRNAs and lncRNAs involved in the modulation of RT responses. The number of researches in this area is limited. Most of the investigations have been done in cell lines, and studies on the tissue or blood of the patients are rare. High throughput studies on patients’ samples are essential to find relevant miRNAs. MiR27a, mir21, and mir17-92 clusters (miR-19a, miR-20a and miR-19b) are shown to have an oncogenic role in the RT response of BC [Citation48,Citation49]. Some important oncomiRs, including miR-21, miR-15b, miR-221, etc., have shown upregulation in BCSCs and BC patients after different doses of IR [Citation27].
There are important studies about miRs with tumor suppressor role in RT of BC. Most of these studies have investigated that tumor suppressor miRs modulate DDR pathways after RT. MiR22, mir139-p5, mir200c, mir205, and miR129-p5 can sensitize resistant cells to radiation by regulating different pathways. In vitro studies on BCSCs showed that the Let-7 family and miR142p inhibit some essential oncogenes in these cells [Citation21,Citation50–52].
It has been shown that lncRNAs as oncogenes or tumor suppressors could modify radiotherapy responses. Most of the studies are about lncRNAs with oncogenic roles. HOTAIR, CCAT1, NEAT1, LncAFAP1, LINC00963, and LINC02582 contribute to radio-resistance in BC. They have oncogenic effects on critical cellular pathways such as apoptosis and DNA break repair [Citation22,Citation36,Citation43,Citation44,Citation47]. In contrast, LINP as a tumor suppress or plays an essential role in the appropriate response to radiotherapy in BC patients [Citation42].
5. Conclusion
In conclusion, miRNA and lncRNAs are promising in predicting and manipulating the radiation response in the clinic to enhance RT. Quantification of potential ncRNAs in BC patients before and after RT may be used as a prognostic panel in the future. In this way, clinical trials are required to find, approve, and make a unique profile of miRNA and lncRNAs for BC patients who will use RT.
Authors’ contributions
Rezvan Esmaeili: Corresponding author, Conceptualization, Writing - Review & Editing
Tayebeh Oghabi Bakhshaiesh: Investigation, Writing - Original Draft preparation
Acknowledgments
Figure were created with BioRender.com.
Data availability statement for Share Upon Reasonable Request Data Sharing Policy
Data sharing is not applicable to this article as no new data were created or analyzed in this study.
Disclosure statement
No potential conflict of interest was reported by the author(s).
Additional information
Funding
References
- Azria D, Brengues M, Gourgou S, et al. Personalizing Breast Cancer Irradiation Using Biology: from Bench to the Accelerator. Front Oncol. 2018;8. DOI:10.3389/fonc.2018.00083
- Czochor JR, Glazer PM. microRNAs in cancer cell response to ionizing radiation. Antioxid Redox Signal. 2014;21:293–312.
- Chaudhry MA. Radiation-induced microRNA: discovery, functional analysis, and cancer radiotherapy. J Cell Biochem. 2014;115:436–449.
- Malik A, Sultana M, Qazi A, et al. Role of Natural Radiosensitizers and Cancer Cell Radioresistance: an Update. Anal Cell Pathol. 2016;2016:6146595.
- Zhao L, Lu X, Cao Y. MicroRNA and signal transduction pathways in tumor radiation response. Cell Signal. 2013;25:1625–1634.
- Slodkowska EA, Ross JS. MammaPrint 70-gene signature: another milestone in personalized medical care for breast cancer patients. Expert Rev Mol Diagn. 2009;9:417–422.
- McVeigh TP, Kerin MJ. Clinical use of the Oncotype DX genomic test to guide treatment decisions for patients with invasive breast cancer. Breast Cancer (Dove Med Press). 2017;9:393–400.
- Wei W, Lewis MT. Identifying and targeting tumor-initiating cells in the treatment of breast cancer. Endocr Relat Cancer. 2015;22:R135–55.
- Mueller AK, Lindner K, Hummel R, et al. MicroRNAs and Their Impact on Radiotherapy for Cancer. Radiat Res. 2016;185:668–677.
- Korpela E, Vesprini D, Liu SK. MicroRNA in radiotherapy: miRage or miRador? Br J Cancer. 2015;112:777–782.
- Krause M, Dubrovska A, Linge A, et al. Cancer stem cells: radioresistance, prediction of radiotherapy outcome and specific targets for combined treatments. Adv Drug Deliv Rev. 2017;109:63–73.
- Zhang X, Xie K, Zhou H, et al. Role of non-coding RNAs and RNA modifiers in cancer therapy resistance. Mol Cancer. 2020;19:47.
- Chaudhry MA, Kreger B, Omaruddin RA. Transcriptional modulation of micro-RNA in human cells differing in radiation sensitivity. Int J Radiat Biol. 2010;86:569–583.
- Yan Y, Zhang F, Fan Q, et al. Breast cancer-specific TRAIL expression mediated by miRNA response elements of let-7 and miR-122. Neoplasma. 2014;61:672–679.
- Halimi M, Parsian H, Asghari SM, et al. Clinical translation of human microRNA 21 as a potential biomarker for exposure to ionizing radiation. Transl Res. 2014;163:578–584.
- Liu J, Zhu H, Yang X, et al. MicroRNA-21 is a novel promising target in cancer radiation therapy. Tumour Biol. 2014;35:3975–3979.
- Imani S, Zhang X, Hosseinifard H, et al. The diagnostic role of microRNA-34a in breast cancer: a systematic review and meta-analysis. Oncotarget. 2017;8:23177–23187.
- Lacombe J, Zenhausern F. Emergence of miR-34a in radiation therapy. Crit Rev Oncol Hematol. 2017;109:69–78.
- Stankevicins L, Almeida Da Silva AP, Ventura Dos Passos F, et al. MiR-34a is up-regulated in response to low dose, low energy X-ray induced DNA damage in breast cells. Radiat Oncol. 2013;8:231.
- Kofman AV, Kim J, Park SY, et al. microRNA-34a promotes DNA damage and mitotic catastrophe. Cell Cycle (Georgetown, Tex). 2013;12:3500–3511.
- Bojmar L, Karlsson E, Ellegård S, et al. The role of microRNA-200 in progression of human colorectal and breast cancer. PLoS One. 2013;8:e84815–e.
- Wang B, Zheng J, Li R, et al. Long noncoding RNA LINC02582 acts downstream of miR-200c to promote radioresistance through CHK1 in breast cancer cells. Cell Death Dis. 2019;10:764.
- Sun Q, Liu T, Yuan Y, et al. MiR-200c inhibits autophagy and enhances radiosensitivity in breast cancer cells by targeting UBQLN1. Int J Cancer. 2015;136:1003–1012.
- Kolenda T, Przybyła W, Teresiak A, et al. The mystery of let-7d - a small RNA with great power. Contemp Oncol (Pozn). 2014;18:293–301.
- Wang L, Yuan C, Lv K, et al. Lin28 Mediates Radiation Resistance of Breast Cancer Cells via Regulation of Caspase, H2A.X and Let-7 Signaling. PLoS One. 2013;8:e67373.
- Sun H, Ding C, Zhang H, et al. Let‑7 miRNAs sensitize breast cancer stem cells to radiation‑induced repression through inhibition of the cyclin D1/Akt1/Wnt1 signaling pathway. Mol Med Rep. 2016;14:3285–3292.
- Zaleska K, Przybyła A, Kulcenty K, et al. Wound fluids affect miR-21, miR-155 and miR-221 expression in breast cancer cell lines, and this effect is partially abrogated by intraoperative radiation therapy treatment. Oncol Lett. 2017;14:4029–4036.
- Al-Hajj M, Clarke MF. Self-renewal and solid tumor stem cells. Oncogene. 2004;23:7274–7282.
- Eun K, Ham SW, Kim H. Cancer stem cell heterogeneity: origin and new perspectives on CSC targeting. BMB Rep. 2017;50:117–125.
- Troschel FM, Böhly N, and Borrmann K, et al. miR-142-3p attenuates breast cancer stem cell characteristics and decreases radioresistance in vitro. tumor biology . 2018;40(1010428318791887). doi:10.1177/1010428318791887
- Zhang D, Tang DG, Rycaj K. Cancer stem cells: regulation programs, immunological properties and immunotherapy. Semin Cancer Biol. 2018;52:94–106.
- Zhang Y, Xu B, Zhang X-P. Effects of miRNAs on functions of breast cancer stem cells and treatment of breast cancer. Onco Targets Ther. 2018;11:4263–4270.
- Khalighfard S, Alizadeh AM, Irani S, et al. Plasma miR-21, miR-155, miR-10b, and Let-7a as the potential biomarkers for the monitoring of breast cancer patients. Sci Rep. 2018;8:17981.
- Zhang T, Hu H, Yan G, et al. Long Non-Coding RNA and Breast Cancer. Technol Cancer Res Treat. 2019;18:1533033819843889.
- Aranza-Martínez A, Sánchez-Pérez J, Brito-Elias L, et al. Non-Coding RNAs Associated With Radioresistance in Triple-Negative Breast Cancer. Front Oncol. 2021;11:752270.
- Hu X, Ding D, Zhang J, Cui , J, et al. Knockdown of lncRNA HOTAIR sensitizes breast cancer cells to ionizing radiation through activating miR-218. Biosci Rep. 2019;39(4):BSR20181038. doi: 10.1042/BSR20181038. PMID: 30429228; PMCID: PMC6449517.
- Qian L, Fei Q, Zhang H, et al. lncRNA HOTAIR Promotes DNA Repair and Radioresistance of Breast Cancer via EZH2. DNA Cell Biol. 2020;39(12):2166–2173.
- Zhou Y, Wang C, Liu X, et al. Long non-coding RNA HOTAIR enhances radioresistance in MDA-MB231 breast cancer cells. Oncol Lett. 2017;13:1143–1148.
- Zhang S, Wang B, Xiao H, et al. LncRNA HOTAIR enhances breast cancer radioresistance through facilitating HSPA1A expression via sequestering miR-449b-5p. Thorac Cancer. 2020;11:1801–1816.
- Tang Q, Hann SS. HOTAIR: an Oncogenic Long Non-Coding RNA in Human Cancer. Cell Physiol Biochem. 2018;47:893–913.
- Chen L, Qian X, Wang Z, et al. The HOTAIR lncRNA: a remarkable oncogenic promoter in human cancer metastasis (Review). Oncol Lett. 2021;21:302.
- Zhang Y, He Q, Hu Z, et al. Long noncoding RNA LINP1 regulates repair of DNA double-strand breaks in triple-negative breast cancer. Nat Struct Mol Biol. 2016;23:522–530.
- Lai Y, Chen Y, Lin Y, et al. Down-regulation of LncRNA CCAT1 enhances radiosensitivity via regulating miR-148b in breast cancer. Cell Biol Int. 2018;42:227–236.
- Zhang N, Zeng X, Sun C, et al. LncRNA LINC00963 Promotes Tumorigenesis and Radioresistance in Breast Cancer by Sponging miR-324-3p and Inducing ACK1 Expression. Mol Ther Nucleic Acids. 2019;18:871–881.
- Lv XJ, Tang Q, Tu YQ, et al. Long noncoding RNA PCAT6 regulates cell growth and metastasis via Wnt/β-catenin pathway and is a prognosis marker in cervical cancer. Eur Rev Med Pharmacol Sci. 2019;23:1947–1956.
- Bi Z, Li Q, Dinglin X, et al. Nanoparticles (NPs)-Meditated LncRNA AFAP1-AS1 Silencing to Block Wnt/β-Catenin Signaling Pathway for Synergistic Reversal of Radioresistance and Effective Cancer Radiotherapy. Adv Sci. 2020;7:2000915.
- Lin L-C, Lee H-T, Chien P-J, et al. NAD(P)H:quinone oxidoreductase 1 determines radiosensitivity of triple negative breast cancer cells and is controlled by long non-coding RNA NEAT1. Int J Med Sci. 2020;17:2214–2224.
- Leung CM, Chen TW, Li SC, et al. MicroRNA expression profiles in human breast cancer cells after multifraction and single-dose radiation treatment. Oncol Rep. 2014;31:2147–2156.
- Ren YQ, Fu F, Han J. MiR-27a modulates radiosensitivity of triple-negative breast cancer (TNBC) cells by targeting CDC27. Med Sci Monit. 2015;21:1297–1303.
- Zhang X, Li Y, Wang D, et al. miR-22 suppresses tumorigenesis and improves radiosensitivity of breast cancer cells by targeting Sirt1. Biol Res. 2017;50:27.
- Pajic M, Froio D, Daly S, et al. miR-139-5p Modulates Radiotherapy Resistance in Breast Cancer by Repressing Multiple Gene Networks of DNA Repair and ROS Defense. Cancer Res. 2018;78:501–515.
- Zhang P, Wang L, Rodriguez-Aguayo C, et al. miR-205 acts as a tumour radiosensitizer by targeting ZEB1 and Ubc13. Nat Commun. 2014;5:5671.