ABSTRACT
Lumbar disc herniation (LDH) is a common spinal disease that endangers human health. Genetic factors play a vital role in the progression of LDH. This study aimed to explore the relationship of the MIR31HG polymorphism with LDH risk in the Chinese population. Seven candidate SNPs on MIR31HG in 504 patients with LDH and 503 healthy people were genotyped by Agena MassARRAY platform. Logistic regression was used to calculate the relationship between MIR31HG polymorphism and LDH risk under different genetic models. Multi-factor dimensionality reduction (MDR) analysis was performed to evaluate the SNP–SNP interaction. We found that rs10965059 was significantly associated with a decreased risk of LDH under the dominant (OR = 0.46, 95% CI: 0.34–0.62, P < 0.001), log-additive (OR = 0.59, 95%CI: 0.45–0.76, P < 0.001), and codominant (OR = 0.40, 95%CI: 0.29–0.55, P < 0.001) models in the overall analysis. In the subgroup analyses of age, male, and complications, we found that rs10965059 was associated with a reduced risk of LDH. However, there was no significant correlation between MiR-31HG polymorphisms and risk of LDH in females. In addition, the three SNPs (rs72703442-rs2025327-rs55683539) was mapped to a 26kb LD block with D’ >0.96, suggesting a significant linkage disequilibrium presence among each pair SNPs. MDR analysis showed that the best single-locus and multi-locus models for the prediction of LDH risk were rs10965059 and seven-locus models, respectively, and both of them increased LDH risk. Our results shown that in the Chinese Han population, the MIR31HG polymorphism rs10965059 was involved in a risk to symptomatic LDH, which provides a scientific basis for early screening, prevention, diagnosis and treatment of local LDH high-risk populations.
1. Introduction
Lumbar disc herniation (LDH) is a relatively common spinal diseases resulted by the degeneration and the displacement of nucleus pulposus [Citation1], has been identified as a health problem world-wide now [Citation2], and is the most general diagnosis of lumbar degenerative deformity. Initial lumbago that may eventually develop into persistent sciatica is typical of LDH [Citation3]. Although it is a benign disease, it still makes patients weak and disabled with lower quality of work and life and expensive medical expenses, as well as causes great physical and psychological distress to patients [Citation4]. Studies have shown that 37 markers on 8q24.21 (between CCDC26 and GSDMC) are related to sciatica caused by lumbar disc degeneration (LDHsurg) [Citation5]. A large number of research studies have determined that LDH is a complex and polyfactorial spinal disease caused by all kinds of factors, involving age, physical condition, gender, profession, sports activity, and other unlisted factors [Citation6–8]. However, the twin studies illuminated that greater than 70% of patients with LDH have a genetic origin [Citation9], which suggests that genes may have an important influence on the risk of LDH. Molecular epidemiological studies also pointed out that genetic polymorphisms were associated with LDH risk [Citation10–12].
Long non-coding RNAs (lncRNAs) are defined as transcriptions >200 nucleotides in length, and there is increasing evidences that lncRNAs are involved in regulating the growth and differentiation of various cell types [Citation13]. Zhuang-Wen Liao et al. showed that Lnc-MT1DP in combination with miR-365 disrupted cellular mitochondrial membranes, which in turn increased apoptosis, causing LDH [Citation14]. Ning Tang et al. show that LncRNA TUG1 is involved in promoting intervertebral disc degeneration [Citation15]. These results suggest that lncRNAs play an important role in LDH. MIR31HG is a newly discovered non-coding RNA, which is the host gene of MIR31 affecting the development process by regulating genes related to cell proliferation, apoptosis, cell differentiation and cell movement. Studies have shown that MIR31 plays an important regulatory role in embryo implantation, development, bone and muscle homeostasis, and immune system function [Citation16]. Yankun Dai et al. revealed that miR-31 affected chondrocyte viability and migration through methods, such as dual-luciferase reporter gene detection, MTT, and cell migration [Citation17]. Chanyuan Jin et al. showed that the interaction of MIR31HG with NF-B regulated bone formation and inflammation [Citation18]. These results indicate that MIR31HG is related to a variety of orthopedic diseases. However, the correlation between MIR31HG and LDH has not been reported. This study investigated the association between MIR31HG polymorphism and LDH risk in Chinese population by case-control strategy and aimed to provide a scientific basis for the early screening, diagnosis and treatment of LDH high-risk population.
2. Materials and methods
2.1 Ethics statement
Written informed consent was signed before all subjects participated in the research. The Institutional Ethical Committee of Xi’an Jiaotong University approved the study protocol, which was based on the ethical principles of medical research applicable to the human body in the Declaration of Helsinki.
2.2 Subjects
There are 504 patients with LDH (case group) and 503 healthy subjects (control group) in our study, and among them, patients with LDH was from Xi’an Jiaotong University. Patients with typical clinical signs, and LDH patients determined by imaging examination, including computed tomography and/or magnetic resonance imaging (MRI), were included in this trial as patients with LDH. MRI is the imaging gold standard for the diagnosis of suspected LDH, with a diagnostic accuracy of 97% and high inter-observer reliability [Citation19]. MRI findings of increased T2-weighted signal from the posterior 10% of the disc diameter are highly suggestive of disc herniation [Citation20]. LDH patients had the following symptoms: (i) lower back pain, (ii) lower lumbar pain and local typical sciatica; (iii) limited lumbar flexion amplitude; and (iv) more difficult straight leg elevation test and intensive test [Citation21]. Patients with complex hematological illnesses, trauma, autoimmune diseases, cancers, rheumatoid arthritis and relevant lumbar spine diseases were excluded in the scope of this study inside. Such a person having no family history of lumbago, no spinal instability, and no history of infection and tumor were classified into the control group, which was healthy subjects during the same period of physical examination. Inclusion criteria for LDH complications: (i) incomplete disc herniation; (ii) postoperative recurrence; (iii) nerve root injury; (iv) dural tear; (v) radicular hyperalgesia or burning radiculopathy; (vi) postoperative sensation or neck pain or infection of the surgical site occurs. Exclusion criteria for LDH complications: people who can live normally after the operation without the above-mentioned abnormalities [Citation22].
2.3 SNPs selection and genotyping
The selection of SNPs is based on haplotype data or genotype data and its location in the genome [Citation23–25]. Seven candidate tagging single-nucleotide polymorphisms (SNPs) of MIR31HG gene (rs1332184, rs72703442, rs2025327, rs55683539, rs2181559, rs10965059, and rs10965064) were obtained from the online HapMap database (https://www.internationalgenome.org/category/hapmap), and they are all located inside the MIR31HG region (). A minor allele frequency (MAF) of all selected SNPS was greater than 0.05. We obtained genomic DNA from whole blood samples using GoldMag-mini Whole Blood Genomic DNA Purification Kit (GoldMag Ltd, Xi’an, China) [Citation26]. NanoDrop 2000 was then used to measure the DNA concentration and to save eligible samples for further genotyping. Agena Bioscience Assay Design Suite Version 2.0 (https://agenacx.com/online-tools/)was used to proceed MassEXTEND test [Citation27,Citation28]. Genotyping was conducted by Agena MassARRAY platform with iPLEX gold chemistry (Agena Bioscience, San Diego, CA, USA (Sequenom, Inc)) in step with the manufacturer’s protocol [Citation29]. Finally, we performed data management and analysis.
Table 2. Basic information of candidate SNPs and its relationship with LDH risk.
2.4 Statistical analysis
For statistical analyses, we used Microsoft Excel (Microsoft Corp., Redmond, WA, United States) [7] as well as SPSS 19.0 Software (SPSS Inc., Chicago, IL, USA). To compare the differences in age and sex distribution between the case group and the control group, Welch’s t test and chi-square test were adopted [8]. To measure the genotype frequencies distribution of the polymorphism among the controls, chi-square test was in use [9]. The χ2 test was adopted to measure the difference of allele frequencies distribution between patients and the control group [Citation30]. OR values and 95% CIs used unconditional logistic regression analysis to measure the size of the risk allele effect [Citation31]. Genetic model analyses were adopted by PLINK software (http://www.cog-genom ics.org/plink 2/) to evaluate the relationship between SNPs and LDH risk [Citation32]. Linkage disequilibrium (LD) analysis and haplotype-based associations were carried out by the PLINK software and haploview software package [Citation33]. All the obtained P values were two-sided if the values were of statistical significance when P < 0.05 [Citation34] . In order to exclude the influences of age, sex, and complications on the experimental results, we adjusted for age, sex, and complications, respectively. We used G*power 3.1.9.2 software to calculate the sample size and proportion of the case group and the control group in this study [Citation35]. Multi-factor dimensionality reduction (MDR) analysis was performed through MDR_3.0.2 software to identify high-order interactive models of LDH risk.
3. Result
3.1 Characteristics of the participants
G*power 3.1.9.2 software analysis showed that the total sample size of a study needed to be greater than 210, while the sample size of the control and case groups needed to be greater than 105, where the effect size was 0.5, α error pro was 0.05, power (1 – β error pro) was 0.95, and allocation ratio N2/N1 was 0.998. The demographic information for this study is shown in . There were 504 patients with LDH and 503 healthy individuals possessed in this case-control research, indicating that the sample size in this study fully met the statistical requirements. There were 210 females (42%) and 294 males (58%) in the cases, while 294 men (58%) and 209 women (42%) in the controls. The average age of the control group and the case group were 49.25 ± 13.58 years and 49.30 ± 14.92 years, respectively. Furthermore, according to both groups regarding gender (P = 0.970) and age (P = 0.956), we found that there were no significantly statistical differences.
Table 1. Comparison of characteristics between control group and lumbar disc herniation.
3.2. Basic information of candidate SNPs and its relationship with LDH risk
The certain information about all the SNPs are shown in , such as alleles, the SNP_ID, OR (95%CI), gene name, chromosomal position, minor allele frequency (MAF), the P-value, and the Hardy-Weinberg equilibrium (HWE) results of the cases and controls. The MAF of the control group in this study is very close to the MAF in the 1000 Genome project () and both of them are greater than 0.05. The detection rate of all SNPs was >99% in the cases with LDH and the controls, which was considered sufficient for correlation analysis. The frequency distribution of all SNPs genotype was no difference from the HWE (P > 0.05) in the control group, which indicated that the seven SNPs were in equilibrium and representation. The ORs were calculated to evaluate the associations between SNPs and the risk of LDH. Overall, we learned that rs10965059 was significantly related to a reduced susceptibility of LDH (OR = 0.58, 95%CI = 0.44–0.75, P < 0.001). Nevertheless, we found no association between patients with LDH and the controls in the allele frequencies of the other six SNPs (rs1332184, rs72703442, rs2025327, rs55683539, rs2181559, and rs10965064).
3.3 Genetic model analyses of seven selected SNPs and the risk of LDH
Further, four types of multiple genetic models are used to analyze the correlation between SNPs and the risk of LDH by adjusting logistic regression analyses of gender and age (). A noteworthy association with a declining risk of LDH was found to be rs10965059 under the codominant (OR = 0.40, 95%CI = 0.29–0.55, P < 0.001), dominant (OR = 0.46, 95%CI = 0.34–0.62, P < 0.001) and log-additive (OR = 0.59, 95%CI = 0.45–0.76, P < 0.001) models. Before and after adjustment for gender and age, there was no significant association between SNP (rs1332184, rs72703442, rs2025327, rs55683539, rs2181559, and rs10965064) and the risk of LDH (P > 0.05).
Table 3. Genetic model analyses of seven selected SNPs and the risk of lumbar disc herniation.
3.4 Stratified analysis of the gender and age on association between selected SNPs and LDH risk
Additionally, in stratification analyses by gender and age, there are obviously significant correlations between rs10965059 and the LDH risk, while no correlation is found between the other six SNPs and LDH risk as shown in . At age >49 years, rs10965059 was associated with the reduced risk of LDH in the allele (OR = 0.55, 95%CI = 0.38–0.78, P = 0.001), codominant (OR = 0.32, 95%CI = 0.21–0.50, P < 0.001), dominant (OR = 0.39, 95%CI = 0.26–0.59, P < 0.001), log-additive (OR = 0.54, 95%CI = 0.38–0.78, P = 0.001) models. At the same time, we also found rs10965059 was relevant with a 0.61-fold reduced LDH risk in patients at age ≤49 years in the allele model (OR = 0.61, 95%CI = 0.41–0.89, P = 0.011), a 0.50-fold decreased susceptibility of LDH in the codominant model (TCvs.CC, OR = 0.50, 95%CI = 0.31–0.78, P = 0.002), a 0.53-fold decreased susceptibility of LDH in the dominant model (CT/TTvs.CC, OR = 0.53, 95%CI = 0.35–0.82, P = 0.004) and a 0.63-fold decreased susceptibility of LDH in the log-additive model (OR = 0.63, 95%CI = 0.43–0.91, P = 0.015).
Table 4. Stratified analysis of the gender and age on association between selected SNPs and LDH risk.
Furthermore, we found only rs10965059 was associated with LDH in the male subgroup, as illustrated in . The rs10965059 was associated with a descending risk of LDH under the log-additive (OR = 0.43, 95%CI = 0.31–0.61, P < 0.001), dominant (OR = 0.31, 95%CI = 0.21–0.46, P < 0.001) and allele (OR = 0.44, 95%CI = 0.31–0.61, P < 0.001) models, however, for female groups, no related SNPs were found.
3.5 Stratified analysis of the association between the selected SNP and the risk of complications caused by LDH
In addition, we carry out another stratified analysis of complication caused by LDH on association between the selected SNPs and the risk of complications caused by LDH, as displayed in . Compared to non-complication, the rs10965059 had related with an incremental munity of LDH in patients with complication under the allele (T vs. C, OR = 1.54, 95%CI = 1.03–2.31, P = 0.034), the recessive (T/T vs. C/C-C/T, OR = 3.55, 95%CI = 1.03–12.28, P = 0.046) and the log-additive (OR = 1.64, 95%CI = 1.07–2.51, P = 0.024) models. However, there was no significant association between six loci (rs1332184, rs72703442, rs2025327, rs55683539, rs2181559, and rs10965064) on MIR31HG gene and risk of LDH (P > 0.05) in the complication subgroup.
Table 5. Stratified analysis of the association between the selected SNP and the risk of complications caused by LDH.
3.6 Haplotype analyses
Based on genotype data of seven SNP, Haploview software was used to construct haplotypes. In the overall analysis, linkage disequilibrium analysis revealed a block in MIR31HG () and the LD block included rs72703442, rs2025327 and rs55683539. Furthermore, the correlation between the MIR31HG haplotype and the risk of LDH was analyzed, however, we did not discovery any statistical evidence for the risk of LDH in our research.
3.7 MDR analysis for the effect of MIR31HG SNP-SNP interaction on LDH risk
As shown in , the dendogram and Fockman-Reilingold describe the interactions between these SNPs that the short connections between nodes represent more redundant interactions (A) and that negative entropy of two locis indicates antagonistic effect (B), and positive entropy indicates synergistic effect. The MDR model analysis of SNP–SNP interaction are showed in . The results showed that rs10965059 was the best single-locus model to predict LDH (testing accuracy (TA), 0.589; cross-validation consistency (CVC), 10/10; OR = 2.78, 95%CI = 1.04–7.39, P = 0.037). Among the multi-locus models, the best combination of LDH risk prediction was the seven-locus model (TA, 0.596; CVC, 10/10; OR = 4.17, 95%CI = 3.17–5.49, P < 0.001).
Table 6. MDR analysis for the effect of MIR31HG SNP–SNP interaction on LDH risk.
Figure 2. The dendogram (a) and fruchterman Rheingold (b) of MIR31HG SNP-SNP interaction for LDH risk. (a) Short connections among nodes represent stronger redundant interactions. (b) A negative value for the two-locus entropy indicates that it is an antagonistic effect, and a positive value indicates that it is a synergistic effect.
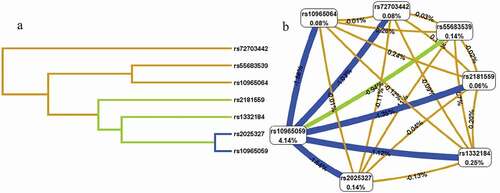
4. Discussion
Intervertebral disc degeneration is a common multifactorial and polygenic disease [Citation36], and some studies have found some genes related to LDH [Citation4,Citation8,Citation37], such as Interleukin6 [Citation11] and ALDH2 [Citation10]. However, the effect of SNPs MIR31HG gene on LDH is unclear. Our results showed that rs10965059 was found to be concerned with susceptibility to LDH in the overall analysis, age, male, and complication stratification study. As far as we know, this is the first time that a relationship between the MIR31HG gene polymorphisms and LDH risk has been discovered in the Chinese Han population.
LDH represents a historically well-known disease. At present, mainly through surgical treatment and non-surgical treatment, expensive treatment costs have brought a huge burden to patients and society [Citation38], but the specific treatment mechanism of LDH is still unclear. Previous studies have shown that the susceptibility to LDH is related to genetic polymorphisms. For example, Dong Q et al. [Citation10] showed the relationship between ALDH2 polymorphisms and susceptibility of LDH. Yang X et al. [Citation39] found that in the men subgroup, the risk of LDH was 0.46 times higher in individuals with the rs8040868 CT genotype than in individuals with the rs8040868 TT genotype (OR = 0.46, 95%CI = 0.25–0.84, P = 0.012). However, as far as we know, whether polymorphisms of MIR31HG are related to the emotivity of LDH has not been reported before.
Compared with mRNAs, long non-coding RNAs (lncRNAs) usually do not have a protein-encoding open reading frames and has a relatively low expression level. However, there is a higher degree of conservation in the promoter and exon regions of lncRNAs, suggesting that they have important functions in biological processes [Citation40]. In addition, some studies have shown that the abnormal expression of lncRNAs is related to many orthopedic diseases such as LDH [Citation41] MIR31HG produces a incRNA that serves as a host gene for MIR31. A research by Walter M. Hodges et al. showed that MIR31 played a role in inhibiting the various steps of bone morphogenetic protein 2 (BMP2)-mediated osteogenesis [Citation42]. MIR31 is related to mechanisms, such as promoting muscle regeneration, inhibiting ubiquitin ligase, and resisting muscle atrophy during hibernation [Citation43] Studies have shown that MIR31 is involved in the pathogenesis of neurodegenerative diseases [Citation44]. Jin C et al. [Citation18] demonstrated that the down-regulation of MIR31HG can not only promote osteogenic differentiation, but also fully reverse the inhibitory effect of osteogenesis in an inflammatory environment. These results indicate that MIR31HG plays an important role in the occurrence and development of a variety of orthopedic diseases. But there is no related report on the exploration of the relationship between MIR31HG and LDH. We speculate that to a certain extent, the MIR31HG gene may have to do with the risk of LDH. We selected the rs1332184, rs72703442, rs2025327, rs55683539, rs2181559, rs10965059, rs10965064 locus on the MIR31HG gene for research. Among these loci, we only found that rs1332184 has been reported in a piece of literature, which found that the rs1332184 mutation had a strong correlation with that in alcohol (with P value 0.001 or lower) [Citation45]. However, as for rs1332184, there were also no distinctly significant differences between the patients and the control group (P > 0.05) in our study, which may be caused by different research methods, distinctive research samples and various environments
In general, according to age and male stratified analyses, we all found that rs10965059 was concerned with a lower risk of LDH. However, in a stratified analysis of complications, the CT and TT genotypes of rs10965059 were concerned with an incremental susceptibility of LDH compared to the CC genotype after adjustments for age and gender under the multiple models. Our findings supported the rationality of our previous guess. Therefore, these results showed that the variation of MIR31HG may influence the risk of LDH in Chinese Han population. More samples and functional tests are required to carry out our results, and we will be working on them.
MDR analysis is a new method for analyzing interactions developed in recent years [Citation46]. This study explored the impact of SNP–SNP interaction on the risk of LDH through MDR analysis. The results showed that the best single-locus model for LDH risk prediction was rs10965059, and the best multi-locus model is the seven-locus model, which increases the risk of LDH. There are some limitations in our research. First, a larger sample is worth considering to validate our results. Second, there were still selection biases during the survey. Third, this study did not assess the relationship between MIR31HG polymorphisms and types of clinical pathological diseases. The mechanism of LDH remains to be further studied.
5. Conclusion
In conclusion, our results indicate that the MIR31HG gene polymorphism is associated with the risk of LDH in the Chinese population, which has laid a certain genetic theoretical basis for the early screening of LDH high-risk populations.
Ethics approval and consent to participate
Written informed consent was signed before all subjects participated in the research. The Institutional Ethical Committee of Xi’an Jiaotong University approved the study protocol, which was based on the ethical principles of medical research applicable to the human body in the Declaration of Helsinki.
Acknowledgments
We are very grateful to the individuals who participated in this study. We also thank the clinicians and hospital staff for their samples and data collection for this study. We would also like to thank all participants and collaborators for this manuscript.
Disclosure statement
No potential conflict of interest was reported by the author(s).
Additional information
Funding
References
- Han L, Zhao P, Guo W, et al. Short-term study on risk-benefit outcomes of two spinal manipulative therapies in the treatment of acute radiculopathy caused by lumbar disc herniation: study protocol for a randomized controlled trial. Trials. 2015;16. DOI:10.1186/s13063-015-0634-0
- Chen B-L, Guo J-B, Zhang H-W, et al. Surgical versus non-operative treatment for lumbar disc herniation: a systematic review and meta-analysis. Clin Rehabil. 2018;32:146–160.
- Rajasekaran S, Bajaj N, Tubaki V, et al. ISSLS prize winner: the anatomy of failure in lumbar disc herniation. Spine (Phila Pa 1976). 2013;38:1491–1500.
- Eskola PJ, Lemmela S, Kjaer P, et al. Genetic association studies in lumbar disc degeneration: a systematic review. PLoS One. 2012;7:e49995.
- Bjornsdottir G, Benonisdottir S, Sveinbjornsson G, et al. Sequence variant at 8q24.21 associates with sciatica caused by lumbar disc herniation. Nat Commun. 2017;8:14265.
- Ml S. Epidemiology of low back pain. Bailliere’s clin rheumatol. 1992;6:559–573.
- Suzuki S, Fujita N, Hosogane N, et al. Excessive reactive oxygen species are therapeutic targets for intervertebral disc degeneration. Arthritis Res Ther. 2015;17. DOI:10.1186/s13075-015-0834-8.
- Zhang Y-G, Zhang F, Sun Z, et al. A controlled case study of the relationship between environmental risk factors and apoptotic gene polymorphism and lumbar disc herniation. The American Journal of Pathology. 2013;182(1):56–63.
- Battie MC, Videman T, Levalahti E, et al. Genetic and environmental effects on disc degeneration by phenotype and spinal level: a multivariate twin study. Spine (Phila Pa 1976). 2008;33:2801–2808.
- Dong Q, Ren G, Zhang K, et al. Genetic polymorphisms of ALDH2 are associated with lumbar disc herniation in a Chinese Han population. Scientific Reports. 2018;8. DOI:10.1038/s41598-018-31491-6
- Huang X, Chen F, Zhao J, et al. Interleukin 6 (IL-6) and IL-10 promoter region polymorphisms are associated with risk of lumbar disc herniation in a Northern Chinese Han population. Genet Test Mol Biomarkers. 2017;21:17–23.
- Yang X, Li F, Xin D, et al. Investigation of the STOX1 polymorphism on lumbar disc herniation. Mol Genet Genomic Med. 2019;8:e1038.
- Wapinski O, Chang HY. Corrigendum: long noncoding RNAs and human disease. Trends Cell Biol. 2011;21:354–361.
- Liao ZW, Fan ZW, Huang Y, et al. Long non-coding RNA MT1DP interacts with miR-365 and induces apoptosis of nucleus pulposus cells by repressing NRF-2-induced anti-oxidation in lumbar disc herniation. Ann Transl Med. 2021;9:151.
- Tang N, Dong Y, Xiao T, et al. LncRNA TUG1 promotes the intervertebral disc degeneration and nucleus pulposus cell apoptosis through modulating miR-26a/HMGB1 axis and regulating NF-κB activation. Am J Transl Res. 2020;12:5449–5464.
- Stepicheva NA, Song JL. Function and regulation of microRNA-31 in development and disease. Mol Reprod Dev. 2016;83:654–674.
- Dai Y, Liu S, Xie X, et al. MicroRNA‑31 promotes chondrocyte proliferation by targeting C‑X‑C motif chemokine ligand 12. Mol Med Rep. 2019;19:2231–2237.
- Jin C, Jia L, Huang Y, et al. Inhibition of lncRNA MIR31HG promotes osteogenic differentiation of human adipose-derived stem cells. Stem Cells. 2016;34:2707–2720.
- Kreiner DS, Hwang SW, Easa JE, et al. An evidence-based clinical guideline for the diagnosis and treatment of lumbar disc herniation with radiculopathy. Spine J. 2014;14:180–191.
- Messner A, Stelzeneder D, Trattnig S, et al. Does T2 mapping of the posterior annulus fibrosus indicate the presence of lumbar intervertebral disc herniation? A 3.0 Tesla magnetic resonance study. European spine journal: official publication of the European Spine Society, the European Spinal Deformity Society, and the European Section of the Cervical Spine Research Society 2017;26:877–883.
- Amin RM, Andrade NS, Neuman BJ. Lumbar disc herniation. Curr Rev Musculoskelet Med. 2017;10:507–516.
- Zhou C, Zhang G, Panchal RR, et al. Unique complications of percutaneous endoscopic lumbar discectomy and percutaneous endoscopic interlaminar discectomy. Pain Physician. 2018;21:E105–e12.
- Daly MJ, Rioux JD, Schaffner SF, et al. High-resolution haplotype structure in the human genome. Nat Genet. 2001;29:229–232.
- Gabriel SB, Schaffner SF, Nguyen H, et al. The structure of haplotype blocks in the human genome. Science (New York, NY). 2002;296:2225–2229.
- Villa-Angulo R, Matukumalli LK, Gill CA, et al. High-resolution haplotype block structure in the cattle genome. BMC Genet. 2009;10:19.
- Wang J, Jin T, Mo F, et al. Association between IL-1 gene polymorphisms and tuberculosis susceptibility in the ChineseTibetan population. Int J Clin Exp Pathol. 2018;11:5441–5449.
- Geng TT, Xun XJ, Li S, et al. Association of colorectal cancer susceptibility variants with esophageal cancer in a Chinese population. World J Gastroenterol. 2015;21:6898–6904.
- Yang Q, Guo CY, Cupples LA, et al. Genome-wide search for genes affecting serum uric acid levels: the Framingham Heart Study. Metabolism. 2005;54:1435–1441.
- Gabriel S, Ziaugra L, Tabbaa D. SNP genotyping using the sequenom MassARRAY iPLEX platform. Curr Protoc Hum Genet. 2009;60: Chapter 2:Unit 2 12. 10.1002/0471142905.hg0212s60.
- Adamec C. Example of the use of the nonparametric test. Test x2 for comparison of 2 independent examples. Ceskoslovenske zdravotnictvi. 1964;12:613–619.
- Bland JM, Altman DG. Statistics notes. The odds ratio. BMJ. 2000;320:1468.
- Sole X, Guino E, Valls J, et al. SNPStats: a web tool for the analysis of association studies. Bioinformatics. 2006;22:1928–1929.
- Barrett JC, Fry B, Maller J, et al. Haploview: analysis and visualization of LD and haplotype maps. Bioinformatics. 2005;21:263–265.
- Song MK, Lin FC, Ward SE, et al. Composite variables: when and how. Nurs Res. 2013;62:45–49.
- Faul F, Erdfelder E, Lang AG, et al. G*Power 3: a flexible statistical power analysis program for the social, behavioral, and biomedical sciences. Behav Res Methods. 2007;39:175–191.
- Battie MC, Videman T. Lumbar disc degeneration: epidemiology and genetics. J Bone Joint Surg-Am Vol. 2006;88A:3–9.
- Mayer JE, Iatridis JC, Chan D, et al. Genetic polymorphisms associated with intervertebral disc degeneration. Spine J. 2013;13:299–317.
- Benzakour T, Igoumenou V, Mavrogenis AF, et al. Current concepts for lumbar disc herniation. Int Orthop. 2019;43:841–851.
- Yang X, Guo X, Huang Z, et al. CHRNA5/CHRNA3 gene cluster is a risk factor for lumbar disc herniation: a case-control study. J Orthop Surg Res. 2019;14:243.
- Ulitsky I, Bartel DP. lincRNAs: genomics, evolution, and mechanisms. Cell. 2013;154:26–46.
- Esteller M. Non-coding RNAs in human disease. Nat Rev Genet. 2011;12:861–874.
- Hodges WM, O’Brien F, Fulzele S, et al. Function of microRNAs in the osteogenic differentiation and therapeutic application of adipose-derived stem cells (ASCs). Int J Mol Sci. 2017;18(12);2597.
- Luu BE, Lefai E, Giroud S, et al. MicroRNAs facilitate skeletal muscle maintenance and metabolic suppression in hibernating brown bears. J Cell Physiol. 2020;235:3984–3993.
- Yan JH, Hua P, Chen Y, et al. Identification of microRNAs for the early diagnosis of Parkinson’s disease and multiple system atrophy. J Integr Neurosci. 2020;19:429–436.
- Yuan A, Yue QQ, Apprey V, et al. An extension of the weighted dissimilarity test to association study in families. Hum Genet. 2007;122:83–94.
- Lou XY, Chen GB, Yan L, et al. A generalized combinatorial approach for detecting gene-by-gene and gene-by-environment interactions with application to nicotine dependence. Am J Hum Genet. 2007;80:1125–1137.