ABSTRACT
N-acetylserotonin (NAS) exerts neuroprotective, antioxidant, and anti-apoptotic effects. Oxidative stress and apoptosis are the primary causes of spinal cord injury (SCI). Herein, we explored potential protective effects and mechanisms of NAS in a neuron oxidative damage model in vitro. We established an oxidative damage model in PC12 cells induced by hydrogen peroxide (H2O2) and treated these cells with NAS. NAS enhanced the activity of superoxide dismutase and halted the increase in reactive oxygen species (ROS) and the expression of inducible nitric oxide synthase. Additionally, NAS promoted protein expression of Bcl-2, but inhibited protein expressions of Fas, FADD, cytochrome c, Bax, cleaved caspase-9, and cleaved caspase-3, namely, decreasing protein expression of the Fas and mitochondrial pathways. Furthermore, it reduced the rate of apoptosis and necroptosis-related protein expressions of MLKL and p-MLKL. Moreover, NAS promoted the protein expression of p-PI3K and p-AKT, and the addition of the PI3K inhibitor LY294002 partially attenuated the antioxidant stress and anti-apoptotic effects of NAS in H2O2 stimulated PC12 cells. In conclusion, NAS protected PC12 cells from apoptosis and oxidative stress induced by H2O2 by inhibiting ROS activity and activating the PI3K/AKT signaling pathway.
Introduction
Spinal cord injury (SCI) is a permanent or temporary change in spinal cord function after traumatic (including traffic accidents, falls, sports-related injuries) or non-traumatic factors (including tumors, intervertebral disc degeneration, inflammation) [Citation1]. Approximately 780,000 new cases of traumatic SCI are reported worldwide every year, half of which require surgical treatment [Citation2]. Patients with SCIs incur high medical expenses. The lifetime economic burden of patients with complete SCI in Canada is approximately 3 million dollars [Citation3]. The pathophysiological process after SCI can be divided into primary and secondary SCI. Primary injury immediately leads to spinal cord compression or transection, which is an irreversible process [Citation1]. Secondary injury, which occurs after primary injury, has multiple triggers, including glutamate-mediated excitotoxicity, increased free radical release, oxidative stress, inflammation, apoptosis, and necrosis [Citation4–6]. Excessive accumulation of ROS in the process of secondary SCI leads to oxidative stress [Citation7] and oxidative stress-induced apoptosis [Citation8,Citation9], which participate in the continuous cascade of secondary injury and further cause an adverse microenvironment after injury [Citation4]. Therefore, effective methods to reduce secondary injury, including oxidative stress, apoptosis, and inflammation, are crucial for neurological function recovery after SCI.
N-acetylserotonin (NAS), an intermediate in the conversion of serotonin to melatonin, is mainly synthesized in the pineal gland and retina of mammals. NAS has proven to be a more effective antioxidant than melatonin, which can effectively reduce ROS and inducible nitric oxide synthase (iNOS) expression in macrophages [Citation10]. NAS can regulate the activation of the promyosin-associated kinase receptor B (TrkB)/protein kinase B (AKT) pathway to reduce oxidative stress-induced neuronal apoptosis and exert its neuroprotective effect [Citation11–13]. NAS can exert a neuroprotective activity through antioxidant stress, anti-apoptosis, and anti-inflammatory effects and has protective influences in a variety of diseases. However, it is not clear whether NAS plays a role in an in vitro model of SCI.
The phosphatidylinositol 3-kinase (PI3K)/AKT signal transduction pathway plays an important role in neuronal survival and growth and in neurodegenerative diseases [Citation14]. PI3K can be divided into three subtypes (class I, class II, and class III). Class I is the most widely studied at present. Class I heterodimers are composed of a P85 type regulatory subunit and a P110 type catalytic subunit, in which the P110 catalytic subunit consists of P110 α, P110 β, P110 γ, and P110 δ [Citation15]. When an upstream signal is received, the P85 subunit is recruited to a site near the plasma membrane and binds to the P110 subunit to phosphorylate phosphatidylinositol (4,5) diphosphate (PIP2) to become phosphatidylinositol (3,4,5) triphosphate (PIP3). Subsequently, PIP3 binds to AKT and transfers it from the cytoplasm to the cell membrane for activation [Citation16], thereby phosphorylating a variety of downstream effector proteins, including apoptotic regulatory proteins and transcription factors [Citation17]. Under physiological and pathological conditions, it can regulate cellular proliferation, metabolism, oxidative stress, and apoptosis [Citation18]. The PI3K/AKT signal transduction pathway plays an important role in redox homeostasis and is anti-apoptotic and anti-inflammatory after SCI [Citation19–21], NAS and its derivatives can play a neuroprotective role by activating the PI3K/AKT signaling pathway [Citation13,Citation22,Citation23]. Therefore, we conclude that NAS may have a protective effect in a neuron oxidative damage model in vitro and that the PI3K/AKT signal transduction pathway may play a role in it.
ROS can effectively induce oxidative stress injury and participate in regulating cellular functions, such as apoptosis [Citation24]. The PC12 cell line is commonly used in neuroscience research for neuroprotection, neurotoxicity, and neuro-inflammation [Citation25]. The PC12 cell model induced by H2O2 is often used in in vitro studies of nervous system diseases, such as SCI [Citation26,Citation27]. Therefore, we chose the H2O2-induced oxidative injury PC12 cell model as our research object. Herein, we aimed to investigate the effects of NAS on cell viability, apoptosis, and oxidative stress of PC12 cells, stimulated by H2O2, and to elucidate its possible molecular mechanism.
Materials and methods
Materials
NAS (high-performance liquid chromatographic purity ≥ 99%) was obtained from Sigma-Aldrich (St. Louis, Missouri, USA). Dulbecco’s Modified Eagle Medium (DMEM), fetal bovine serum (FBS), 1 × phosphate buffered saline (PBS) and 0.25% trypsin Ethylenediamine tetraacetic acid (EDTA) were purchased from Gibco/BRL (Gaithersburg, USA). 2, 7-Dichlorofluorescein diacetate (DCFH-DA) ROS fluorescent probe was obtained from Maokang Biotechnology Company (Shanghai, China). Cell counting kit (CCK-8) and annexin V-fluorescein isothiocyanate (FITC)/propidium iodide (PI) apoptosis detection kits were obtained from Yeason Biotechnology Company (Shanghai, China). LY294002 (PI3K inhibitor) was obtained from Medchem Express (Shanghai, China). Specific antibodies against cleaved caspase-3, cleaved caspase-9, Bax, Bcl-2, Fas-associating protein with a novel death domain (FADD) and iNOS were obtained from Proteintech Company (Wuhan, China). Specific antibodies against β-actin and 4’, 6‐diamidino‐2‐phenylindole (DAPI) were purchased from Beyotime (Hangzhou, China). Specific antibodies against p-AKT (Ser473) and AKT were purchased from Cell Signaling Technology (Danvers, MA, USA). Specific antibodies against p-PI3K p85 (Tyr458) [Tyr467], Phospho-mixed lineage kinase domain-like (MLKL) (Ser358) and PI3K were obtained from Affinity Biosciences (Jiangsu, China). Specific antibodies against Cytochrome C (Cyt-c) and MLKL were obtained from Abcam (Shanghai, China). Specific antibodies against Fas were obtained from Santa Cruz Biotechnology (Shanghai, USA). All chemicals used in this study were of analytical grade.
Cell culture and treatment
PC12 cells were purchased from Fu Heng Biology (Shanghai, China) and cultured in high-glucose DMEM supplemented with 10% FBS. Cells were cultured at 37°C in a humidified 5% CO2 atmosphere. The complete medium was replaced every alternate day. When the PC12 cell density reached 80–90%, cells were subcultured with 0.25% trypsin-EDTA. PC12 cells in the logarithmic phase were divided into the control group, the 200 μM H2O2 group, the 200 μM NAS+ H2O2 group, and the 300 μM NAS+ H2O2 groups. The cells in the control group were treated with complete medium, and those in the H2O2 group were treated with 200 μM H2O2 for six hours. Cells in NAS+ H2O2 group were pre-treated with NAS for 24 h and then treated with 200 μM H2O2 for six hours. In the second half of the experiment, cells were pre-treated with a PI3K inhibitor (LY29400220; 10 μM) for 12 h, pre-treated with 300 μM NAS for 24 h, and then treated with 200 μM H2O2 for six hours.
Cell viability assay
Cell viability was determined using the CCK-8 kit. PC12 cells were inoculated into 96-well plates at a density of 1 × 104 and incubated in a humidified 5% CO2 atmosphere at 37°C for 24 h, then treated with different concentrations of H2O2 (0, 50, 100, 150, 200, 250, and 300 μM) for 6 h. Subsequently, 10 μL CCK-8 reagent was added to each well and incubated in the above environment for another hour, and the absorbance of each well at 450 nm was measured using a microwell plate reader (Bio-Bio). Cells were cultured for 24 h according to the above method, treated with different concentrations of NAS (0,100,200, 300,400,500, and 600 μM) for 24 h, and then the absorbance was measured using the above method. Cells were cultured again at a density of 1 × 103 for 24 h, treated with NAS (200 and 300 μM) for 24 h, treated with 200 μM H2O2 for 6 h, and the absorbance was measured again.
Detection of superoxide dismutase (SOD) content
A total SOD activity detection kit (Nanjing Jiancheng, China) was used to detect the intracellular SOD activity. In brief, PC12 cells were inoculated into six-well plates at a density of 1 × 104 cells/well and treated with different concentrations of NAS and H2O2, as aforementioned. The cell culture medium was collected using a 15 mL centrifugation tube, and the supernatant was collected by 1200 rpm centrifugation for 5 min. Supernatants were added to SOD detection reagent and incubated in a 37°C water bath for 30 min. Finally, the OD value was measured at 450 nm and SOD activity was calculated.
Annexin V-FITC/PI apoptosis detection
PC12 cells were inoculated into six-well plates at a density of 1 × 104 cells/well and treated as described previously. After treatment, cells were digested using 0.25% trypsin without EDTA and then were collected using centrifugation at 4°C and 1200 rpm for 5 min. Cells were washed twice with precooled PBS and centrifuged at 4°C, 1200 rpm for 5 min. In total, 1–5 × 105 cells were collected. After discarding the PBS, 100 μL of 1× binding buffer was added to re-suspend cells. Then, 5 μL Annexin V-FITC and 10 μL PI staining solution were added and mixed gently, and then reacted at room temperature in the dark for 10 min. At the end of the reaction, 400 μL of 1 × binding buffer was added, gently mixed with the sample, and placed on ice. Finally, an FC500 flow cytometer (Beckman Coulter, California, USA) was used for detection, and CytExpert2.4 acquisition and analysis software (Beckman Coulter, Florida, USA) was used for analysis. Living cells exhibited very low-intensity background fluorescence. Early apoptotic cells were labeled with Annexin-V and showed green fluorescence. Late apoptotic cells were stained with FITC and PI simultaneously to show green and red fluorescence signals, respectively.
ROS detection
ROS were detected to determine oxidative stress levels using a DCFH-DA reactive oxygen fluorescent probe. PC12 cells were seeded in a six-well plate at a density of 1 × 104 cells/well and treated as previously described. According to the product specification, the 10 μM dyeing working solution was configured, and after cell treatment, the culture medium was discarded, 2 mL of 10 μM dyeing working solution was added to each well, and incubated at room temperature for 30 min. Thereafter, the dye working solution was washed twice with preheated PBS, re-added in the wells, and the fluorescence intensity of cells was measured under the excitation wavelength of 485 nm using a fluorescence microscope (Olympus, Japan). In another experiment, after administration of different concentrations of NAS and H2O2, cells were digested with 0.25% trypsin without EDTA and then centrifuged at 4°C, 1200 rpm for 5 min. The cells were then washed twice with precooled PBS. After each wash, they were centrifuged at 4°C at 1200 rpm for 5 min. In total, 1–5 × 105 cells were collected. Then 10 μM staining working solution 1 mL was added and incubated at room temperature for 30 min, after which the staining working solution was discarded, washed twice with precooled PBS, and intracellular ROS fluorescence intensity was detected by flow cytometry.
Immunofluorescence staining
PC12 cells were inoculated into a six-well plate at a density of 1 × 104 cells/well and treated as described above. After discarding the culture medium, cells were washed with PBS thrice, fixed with 4% paraformaldehyde for 15 min, and then permeabilised with 0.5% Triton X-100 for 30 min. After washing with PBS thrice again, 1 mL of 10% goat serum was added to each well and sealed in a 37°C water bath for one hour. Then, the solution was incubated overnight with primary antibody against iNOS (1:300) at 4°C. The next day, cells were incubated with the corresponding fluorescent secondary antibodies in a 37°C water bath for one hour. Finally, nuclei were stained with DAPI and observed under a fluorescence microscope (Olympus, Japan).
Western blot analysis
After cells were treated as described above, these were lysed with a precooled radioimmunoprecipitation assay cell lysis buffer containing phenylmethylsulfonyl fluoride protease inhibitor for 20 min, and centrifuged at 12,000 rpm at 4°C for 20 min after the lysate was collected. After centrifugation, the supernatant was collected, and the protein concentration was determined using an enhanced BCA protein concentration determination kit (Beyotime, China). The supernatant was mixed with 1/3 volume of 4 × protein buffer and boiled for 8 min. Total protein was separated using 10% sodium dodecyl sulfate polyacrylamide gel electrophoresis and then transferred to a polyvinylidene fluoride membrane. Quick Block Blocking Buffer for Western Blot (Beyotime, China) was used at room temperature for one hour to reduce nonspecific binding of antibodies. We incubate the membrane with the first antibodies against the following proteins overnight at 4°C: cleaved caspase-3 (Anti-Rabbit, 1:2000), cleaved caspase-9 (Anti-Rabbit, 1:2000), Bax (Anti-Rabbit, 1:5000), Bcl-2 (Anti-Rabbit, 1:2000), Fas (Anti-mouse, 1:500), FADD (Anti-Rabbit, 1:2000), Cyt-c (Anti-Rabbit, 1:5000), MLKL (Anti-mouse, 1:2000), p-MLKL (Anti-Rabbit, 1:2000), PI3K (Anti-Rabbit, 1:2000), p-PI3K (Anti-Rabbit, 1:2000), AKT (Anti-rabbit, 1:2000), p-AKT (Anti-rabbit, 1:2000), and β-actin (Anti-mouse, 1:2000). On day two, the membrane was washed thrice at room temperature for 10 min each time and then incubated at room temperature for one hour with goat anti-rabbit antibody bound to horseradish peroxidase or goat anti-mouse antibody bound to horseradish peroxidase. The membrane was washed with TBST again thrice at room temperature for 10 min each. The signal intensity of the above proteins was detected using a hypersensitive ECL chemiluminescence kit (Beyotime, China) and ChemiDoc XRS imaging system (Bio-Rad), and the gray value of each protein band was measured using ImageJ software.
Statistical analysis
GraphPad Prism 8.0 (GraphPad, San Diego, CA, USA) was used for drawing and SPSS Version 26.0 (IBM, Armonk, NY, USA) was used for statistical analysis. Student’s T-Test was used to evaluate the difference between the two groups. When more than two groups were compared, one-way analysis of variance (ANOVA) was used, and then Tukey posttest was performed. The data were expressed as percentage, mean ±standard deviation (SD), and all experiments were repeated at least three times. P < 0.05 is considered to be statistically significant.
Results
NAS reversed the changes of viability and morphology of PC12 cells induced by H2O2
To study the effects of NAS and H2O2 on PC12 cells, we first used a CCK-8 kit to determine cell viability. After cells were treated with 50, 100, 150, 200, 250, and 300 μM H2O2 for six hours, the cell viability decreased to 81.56 ± 0.90%, 77.66 ± 2.27%, 65.89 ± 3.47%, 51.32 ± 2.96%, 40.90 ± 2.44%, and 19.99 ± 2.09% respectively (), p < 0.05). When treated with 200 μM H2O2, cell viability decreased to 51.32 ± 2.96% (), p < 0.001); therefore, we chose the oxidative damage model of PC12 cells induced by 200 μM H2O2. We treated cells with 100, 200, 300, 400, 500, and 600 μM NAS for 24 h ()). When 400 μM NAS was used, cell viability was not significantly affected. However, when 100, 200 and 300 μM NAS were used, the cell viability increased by 2.67 ± 1.20%, 7.11 ± 1.44% and 12.11 ± 1.68%, respectively, compared with that of the control group (), p < 0.05). Compared with that of the control group, the cell viability of 500 and 600 μM NAS decreased to 90.10 ± 4.66% and 73.71 ± 4.64%, respectively (), p < 0.05). Therefore, 200 and 300 μM NAS with more obvious changes in cell viability, were selected as the working concentrations. We first pre-treated cells with 200 and 300 μM NAS for 24 h and then treated cells with 200 μM H2O2 for six hours. NAS pre-treatment increased the viability of PC12 cells treated with H2O2 in a dose-dependent manner (), p < 0.05).
Figure 1. NAS weakened the effect of H2O2 on PC12 cell viability and cell morphology (a) Chemical structure of NAS. (b) Cell counting Kit experiment was used to determine the cell viability of PC12 cells treated with different concentrations of H2O2 for 6 hours. (c) Effect of NAS on PC12 cell viability. (d) NAS alleviated the toxic effect of H2O2 on PC12 cells. (e) PC12 cell morphology under 40X magnification microscope and 4 times local field magnification after the interaction of NAS and H2O2. The results are expressed as mean ± standard deviation (SD) (n = 3). ns p > .05 vs. Control group; #p < .05 or ##p < .01 or ###p < .001 vs. Control group; *p < .05 or**p < .01 vs. H2O2 group.
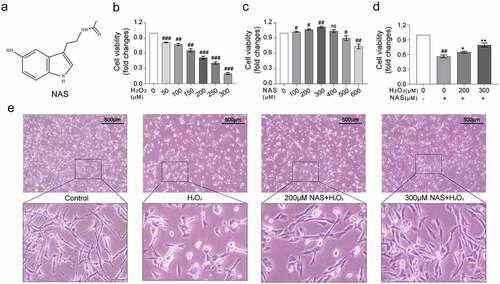
Then, we observed morphological changes of the cells under a microscope after co-treatment with 200 μM NAS and 200 μM H2O2. Cells in the control group exhibited long fusiform shapes. After treatment with 200 μM H2O2 for six hours, the processes of the cells shortened, cell bodies became round and swollen, and some dead cells fell off. The morphological changes in cells treated with 200 μM NAS and 300 μM NAS were reversed ()).
NAS reduced the level of oxidative stress in H2O2 stimulated PC12 cells
To investigate whether the protective effect of NAS on H2O2 stimulated PC12 cells was related to oxidative stress, we evaluated levels of intracellular ROS, SOD, and iNOS. First, we used a DCFH-DA ROS fluorescence probe to detect ROS, and the immunofluorescence results were consistent with those of flow cytometry. The results of the two detection methods showed that the intracellular ROS level increased significantly after H2O2 stimulation (), p < 0.05), whereas the ROS level decreased in a dose-dependent manner after pre-treatment with NAS (), p < 0.05). Subsequently, immunofluorescence staining was used to detect iNOS expression in cells. The expression of iNOS in the H2O2 group was significantly increased (), p < 0.001). After NAS pre-treatment, iNOS expression decreased in a dose-dependent manner (), p < 0.01). The activity of SOD in the H2O2 group decreased significantly (), p < 0.001), while that of SOD in the NAS-pre-treated group was higher than that that of SOD in the H2O2 group (), p < 0.01). In summary, NAS plays a protective role by reducing the level of oxidative stress in PC12 cells stimulated by H2O2.
Figure 2. NAS decreased the level of reactive oxygen species (ROS) in PC12 cells induced by H2O2 (The following fluorescence pictures were taken under an inverted fluorescence microscope with 100X magnification) (a, c) Immunostaining showed the level of ROS in each group. (b, d) A scatter diagram of the DCFH-DA result showed the level of ROS in PC12 cells through flow cytometry. The results are expressed as mean ± SD (n = 3). ##p < .01 or ###p < .001 vs. Control Group; *p < .05 or **p < .01 or ***p < .001 vs. H2O2 group.
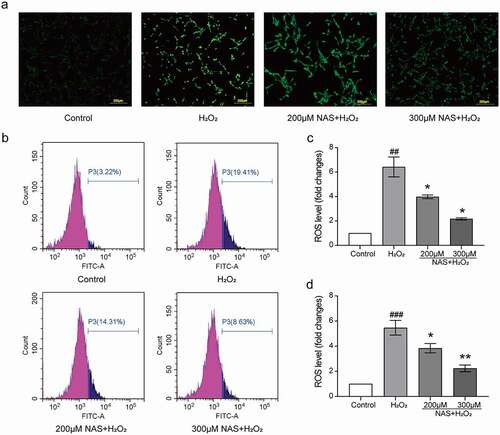
Figure 3. Effect of NAS on inducible nitric oxide synthase (iNOS) expression and superoxide dismutase (SOD) activity in PC12 cells induced by H2O2 (a, b) Immunofluorescence staining was used to detect the effect of NAS on the content of iNOS in PC12 cells and its quantitative analysis. (c) Effect of NAS on SOD activity in PC12 cells. The results are expressed as mean ± SD (n = 3). ###p < .001 vs. Control group; **p < .01 or***p < .001 vs. H2O2 group.
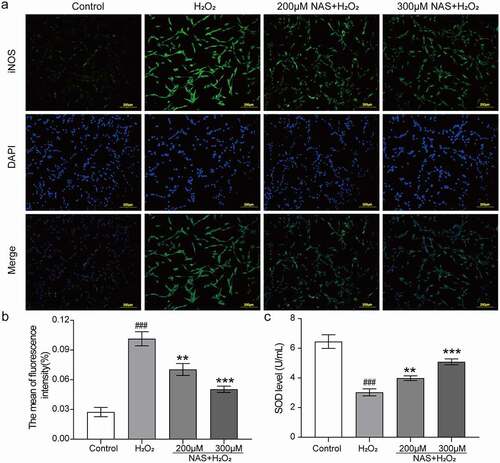
NAS effectively inhibited H2O2-induced apoptosis and necroptosis of PC12 cells
To study whether NAS can reduce the apoptosis and necroptosis in PC12 cells induced by H2O2, we used Annexin V/PI staining and western blotting to detect them. Only 5.71 ± 0.57% apoptotic cells were detected in the control group and 51.81 ± 1.86% apoptotic cells were detected in the H2O2 group (), p < 0.001). However, pre-treatment with 200 and 300 μM NAS effectively reduced the apoptosis rate to 27.68 ± 1.69% and 14.87 ± 0.64% (), p < 0.001), respectively, and effectively reduced the proportion of late apoptotic cells ()). Western blotting analysis showed that after H2O2 stimulation, the protein expressions of MLKL, p-MLKL, Fas, FADD, Cyt-c (Supplementary Figure. 1(a-f), p < 0.05), Bax, cleaved caspase-9, and cleaved caspase-3 was upregulated and that of anti-apoptotic protein Bcl-2 was downregulated in PC12 cells (), p < 0.05), while NAS pre-treatment reversed the expression of these proteins in a dose-dependent manner (), Supplementary Figure.1 (a, d-f), p < 0.05). In summary, NAS effectively reduced the apoptosis and necroptosis of PC12 cells induced by H2O2.
Figure 4. NAS reduced the apoptosis of PC12 cells induced by H2O2 (a) The apoptosis rate of PC12 cells was detected by Annexin V-FITC and PI staining. (b) Percentage of apoptotic cells. (c) The levels of apoptosis related proteins (Bax, Bcl-2, cleaved caspase-3 and cleaved caspase-9) were detected by Western blotting. (d) Quantitative analysis of Bax level. (e) Quantitative analysis of Bcl-2 level. (f) Quantitative analysis of cleaved caspase-3 level. (g) Quantitative analysis of cleaved caspase-9 level. The results are expressed as mean ± SD (n = 3). ##p < .01 or ###p < .001 vs. Control group; *p < .05 or **p < .01 or***p < .001 vs. H2O2 group.
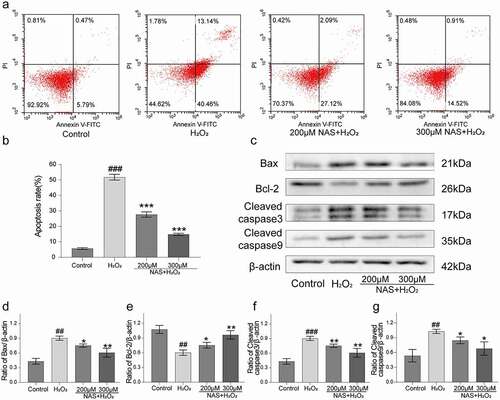
NAS activates the PI3K/AKT signaling pathway in H2O2-induced PC12 cells
To study whether the PI3K/AKT signaling pathway plays a role in H2O2 induced injury and the protective effect of NAS, we detected the expression of key proteins p-PI3K, PI3K, p-AKT, and AKT in the signaling pathway by western blot analysis. The expressions of p-PI3K and p-AKT in the H2O2 group were significantly lower than those in the control group (), p < 0.05), and the expressions of p-PI3K and p-AKT in the NAS group were higher than those in the H2O2 group (), p < 0.05). This indicates that the PI3K/AKT signaling pathway is involved in the protective effect of NAS on PC12 cells stimulated by H2O2.
Figure 5. NAS affected the expression of PI3K/AKT signaling pathway proteins in H2O2-induced PC12 cells (a) The protein levels of PI3K, p-PI3K, AKT and p-AKT in each group were detected by Western blotting. (b) Quantitative analysis of p-PI3K/PI3K ratio. (c) Quantitative analysis of p-AKT/AKT ratio. The results were expressed as mean ±SD (n = 3). ##p < .01 vs. Control group; *p < .05 or **p < .01 vs. H2O2group.
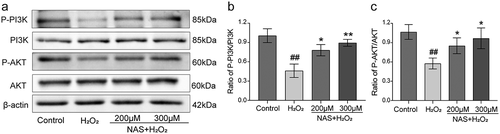
PI3K/AKT signaling pathway plays a role in NAS reducing H2O2-induced oxidative stress in PC12 cells
To study whether the PI3K/AKT signaling pathway is involved in the effect of NAS on oxidative stress, we measured the levels of ROS, SOD, and iNOS in cells after adding the PI3K inhibitor LY294002 (a small molecule known to inhibit PI3K α/δ/β). The immunofluorescence results were consistent with those of flow cytometry. The level of ROS in the inhibitor group was significantly higher than that in the NAS+ H2O2 group (), p < 0.001). After adding inhibitors, we detected the expression of iNOS in cells using immunofluorescence staining, and these additions significantly increased iNOS expression (), p < 0.001). The SOD activity decreased significantly after the addition of the inhibitors (), p < 0.001). In summary, the supplementation of the PI3K inhibitor LY294002 reversed the effect of NAS on oxidative stress, and the PI3K/AKT signaling pathway played a role in NAS by reducing the level of oxidative stress induced by H2O2 in PC12 cells.
Figure 6. LY294002 (inhibitor of PI3K/AKT signaling pathway) affected the level of intracellular ROS induced by H2O2 in PC12 cells induced by NAS (a, c) immunostaining showed the expression level of ROS in each group. (b, d) A scatter diagram of the DCFH-DA result shows the level of ROS in PC12 cells through flow cytometry. The results are expressed as mean ± SD (n = 3). ##p < .01 or ###p < .001 vs. Control group; ***p < .001 vs. H2O2 group; ^^^p < .001 vs. NAS+ H2O2 group.
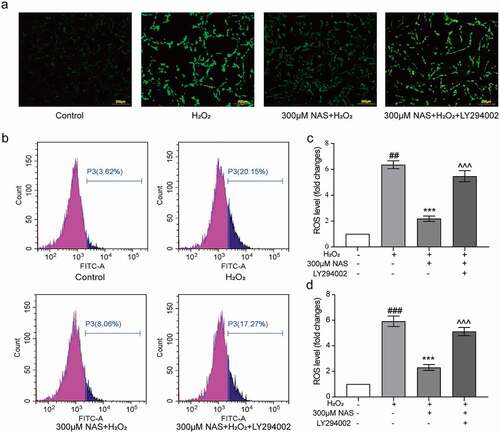
Figure 7. Effect of NAS on iNOS expression and SOD activity in PC12 cells induced by H2O2 after adding LY294002 (a, b) The expression of iNOS in PC12 cells was detected by immunofluorescence staining. (c) SOD activity of each group after adding inhibitor. The results are expressed as mean ± SD (n = 3). ###p < .001 vs. Control group; ***p < .001 vs. H2O2 group; ^^^p < .001 vs. NAS+ H2O2 group.
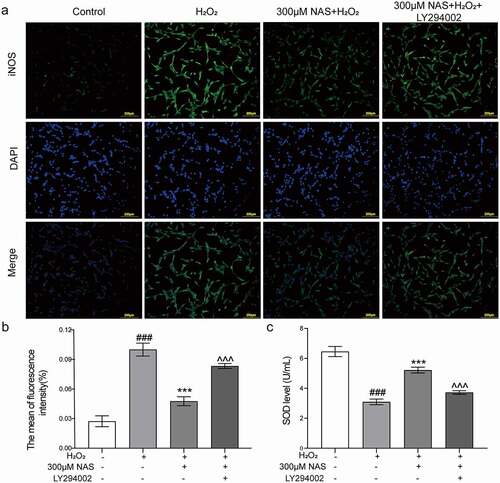
PI3K/AKT signaling pathway plays a role in the inhibitory effect of NAS on H2O2-induced apoptosis in PC12 cells
To study whether the PI3K/AKT signaling pathway is involved in the inhibitory effect of NAS on apoptosis, we detected the expression of the pathway and apoptosis-related proteins and the apoptosis rate of cells after the addition of LY294002. Expressions of p-PI3K and p-AKT decreased significantly after the addition of the inhibitors, which partially attenuated the increase in p-PI3K and p-AKT expressions in cells pre-treated with NAS (), p < 0.01). The protein expressions of Fas, FADD, Cyt-c, Bax, cleaved caspase-9, and cleaved caspase-3 were downregulated, and that of anti-apoptotic protein Bcl-2 was upregulated in PC12 cells induced by H2O2 preconditioned with NAS, which could be partially attenuated by the addition of inhibitors (), Supplementary Figure 1(a, d-f), p < 0.05). However, the addition of the inhibitors did not affect the expression of MLKL and p-MLKL proteins (Supplementary Figure 1(a-c), p > 0.05).
Figure 8. LY294002 affected the expression of PI3K/AKT signaling pathway related proteins and apoptosis of PC12 cells induced by H2O2 under the action of NAS (a) The protein levels of PI3K, p-PI3K, AKT, p-AKT, Bax, Bcl-2, cleaved caspase-3 and cleaved caspase-9 were detected by Western blotting. (b) Quantitative analysis of p-PI3K/PI3K ratio. (c) Quantitative analysis of p-AKT/AKT ratio. (d) Quantitative analysis of Bax level. (e) Quantitative analysis of Bcl-2 level. (f) Quantitative analysis of cleaved caspase-3 level. (g) Quantitative analysis of cleaved caspase-9 level. (h) The apoptosis rate of PC12 cells was detected by Annexin V-FITC and PI staining. (i) Percentage of apoptotic cells. The results are expressed as mean ± standard deviation (n = 3). ###p < .001 vs. Control group; ***p < .001 vs. H2O2 group; ^^^p < .001 vs. NAS+ H2O2 group.
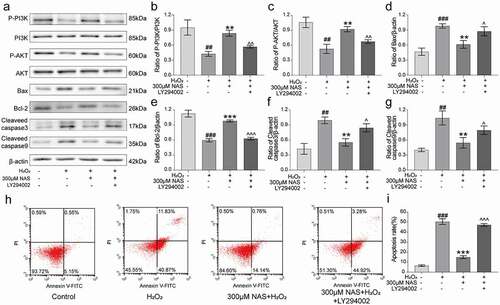
Similarly, the apoptosis rate was determined by Annexin V/PI staining. The apoptosis rate increased to 47.19 ± 1.33% after the addition of inhibitors (), p < 0.001), and the proportion of late apoptotic cells decreased compared to that of the H2O2 group ()), indicating that the addition of inhibitors partially attenuated the anti-apoptotic effect of NAS. In summary, the addition of the PI3K inhibitor LY294002 reversed the inhibitory effect of NAS on apoptosis, and the PI3K/AKT signaling pathway played a role in the inhibition of H2O2-induced apoptosis of PC12 cells by NAS.
Discussion
SCI is a neurological disease with a high mortality and disability rate [Citation28], which imposes enormous psychological and financial pressure on patients and their families. Primary SCI is irreversible. In the pathophysiological process of secondary SCI, the accumulation of ROS can lead to lipid peroxidation and oxidative damage of mitochondrial DNA, which in turn leads to neuronal cell apoptosis, while the oxidative damage of mitochondrial DNA and the destruction of the electron transfer chain will further increase ROS [Citation1,Citation29]. Therefore, finding a method to effectively block this injury cascade process may limit the development of secondary injury and become an effective method for SCI treatment [Citation30,Citation31]. NAS is a more effective antioxidant than melatonin. It has strong antioxidant, anti-apoptotic, anti-inflammatory, and neuroprotective effects both in vivo and in vitro [Citation10–13,Citation32]. We used an H2O2 stimulated PC12 cell model to study the protective effects of NAS and its molecular mechanisms. NAS treatment effectively reduced variations in cellular viability and morphology of H2O2-stimulated PC12 cells, inhibited oxidative stress, and induced apoptosis and necroptosis of H2O2-stimulated PC12 cells. Meanwhile, the ROS-mediated PI3K/AKT signaling pathway played an important role ().
Figure 9. Schematic diagram of NAS alleviating oxidative stress-induced apoptosis of PC12 cells through ROS mediated PI3K/AKT pathway.
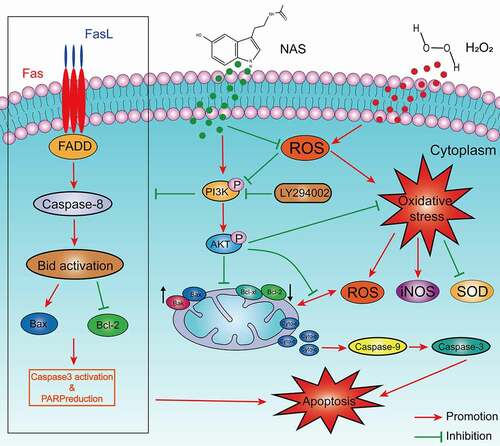
Oxidative stress refers to a state of imbalance between cell oxidation and antioxidation, in which a large number of oxidation intermediates are produced, resulting in cytotoxicity. Oxidative stress plays an important role in secondary SCI pathogenesis [Citation33], leading to a series of cascade reactions, such as inflammation and apoptosis. High ROS concentrations destroy the basic structure of nucleic acids, lipids, and proteins, resulting in structural damage, such as mitochondrial DNA damage, and functional changes, such as apoptosis [Citation34]. SOD accelerates the reaction of superoxide (O2•−) to form H2O2 and oxygen, thus regulating the levels of ROS and reactive nitrogen, thereby controlling damage and regulating signal transduction [Citation35]. As a member of the nitric oxide synthase family, iNOS is expressed only when cells are stimulated by internal and external factors. L-arginine can be oxidized to L-citrulline to produce NO, which reacts with O2•− to produce peroxynitrite (ONOO −) to participate in oxidative stress [Citation36,Citation37]. Dysregulated iNOS eventually leads to toxicity and is closely related to various diseases [Citation38]. Therefore, ROS, SOD, and iNOS are often used as biomarkers for the response to oxidative stress [Citation39]. The cascade of secondary injury induced by oxidative stress during SCI can be effectively reduced by reducing the excessive ROS production and regulating redox balance [Citation40,Citation41]. Our research showed that NAS can effectively reduce the excessive accumulation of ROS induced by H2O2 stimulation in PC12 cells, enhance the antioxidant activity of SOD, and reduce iNOS expression after H2O2 stimulation, thus playing an effective role in resisting oxidative stress.
Apoptosis can be divided into internal (mitochondrial-mediated) and external (death receptor-mediated) [Citation42] mainly involve the Bcl-2 family (Bcl-2, Bax, etc.) and caspase family (caspase-3, caspase-9, etc.) [Citation43,Citation44]. The external pathways are triggered by tumor necrosis factor (TNF) family death receptors on the cell surface [Citation45]. They have intracellular death domains through their respective ligands (such as FAS activated by FAS ligands) and lead to the recruitment of FADD. FADD further activates caspase-8 and further induces apoptosis [Citation46]. Neurological deficits after SCI may be caused by apoptosis of a large number of neurones and oligodendrocytes. Therefore, reducing apoptosis induced by oxidative stress after SCI is an effective strategy for SCI treatment [Citation47]. Our study found that after H2O2 stimulation, the protein expressions of Fas, FADD, Cyt-c, Bax, cleaved caspase-9, and cleaved caspase-3 was upregulated, while that of anti-apoptotic protein Bcl-2 was downregulated. Moreover, the apoptosis rate was significantly increased. After NAS pre-treatment, the expression of the aforementioned proteins and the increase in the apoptosis rate were effectively reversed, indicating that NAS may reduce the apoptosis of PC12 cells induced by oxidative stress through both internal and external apoptosis pathway.
Necroptosis is a programmed cell death that depends on the kinase activity of Receptor Interacting Kinase 1 (RIP1) and RIP3 and the expression of pseudokinase MLKL. At present, it is believed that the phosphorylation of MLKL and the formation of necrotizing bodies mainly occur during necroptosis, so it is considered to be a cellular marker of necroptosis [Citation46]. Our study found that the increase of MLKL and p-MLKL protein expressions induced by H2O2 can be reversed by NAS, which indicates that NAS has a neuroprotective effect to some extent by inhibiting necroptosis.
We further studied the possible mechanisms of action of NAS in terms of antioxidant stress and anti-apoptosis. NAS and its derivatives can play a neuroprotective role by activating the PI3K/AKT signal pathway [Citation13,Citation22,Citation23], and the PI3K/AKT signal transduction pathway plays an important role in redox homeostasis, anti-apoptosis, and anti-inflammatory effects after SCI [Citation19–21]. Similarly, ROS cause apoptosis and oxidative stress by inhibiting the PI3K/AKT pathway [Citation48,Citation49]. We detected the key proteins in the PI3K/AKT signaling pathway and found that the expression of p- PI3K and p-AKT was significantly inhibited after H2O2 stimulation, whereas the antioxidant NAS prevented the inhibition of p- PI3K and p-AKT expression, indicating that the generation of H2O2 -stimulated ROS was a previous inhibition event of the PI3K/AKT signaling pathway. We then blocked the pathway with the PI3K inhibitor LY294002. The antioxidant stress and anti-apoptotic effects of NAS were partially eliminated after blocking the pathway, which further showed that the PI3K/AKT signal transduction pathway was involved in the process of NAS exerting antioxidant stress and anti-apoptotic effects. In conclusion, inhibition of ROS activity and activation of the PI3K/AKT pathway play important roles in NAS antioxidant stress and apoptosis inhibition. Our research has some limitations, including the lack of research on the model of SCI and the therapeutic effect of NAS in clinical SCI patients. Oxidative stress is also closely related to programmed cell death such as pyroptosis [Citation50,Citation51]. Therefore, NAS may play a protective role through other mechanisms. Moreover, a more comprehensive analysis is required in the future.
Conclusion
In conclusion, NAS protected PC12 cells from apoptosis and oxidative stress induced by H2O2 by inhibiting ROS activity and activating the PI3K/AKT signaling pathway. This study elucidates the neuroprotective mechanism of NAS and provides a basis for a follow-up study of NAS in SCI.
Authors’ contributions
Jihe Kang, Yidian Wang, and Xudong Guo contributed equally to this work. We would like to thank Editage (www.editage.cn) for English language editing.
Supplemental Material
Download TIFF Image (3.4 MB)Disclosure statement
No potential conflict of interest was reported by the author(s).
Data availability statement
The datasets generated during and/or analysed during the current study are available from the corresponding author on reasonable request.
Supplementary material
Supplemental data for this article can be accessed online at https://doi.org/10.1080/15384101.2022.2092817
Additional information
Funding
References
- Ahuja CS, Wilson JR, Nori S, et al. Traumatic spinal cord injury. Nat Rev Dis Primers. 2017;3:17018.
- Kumar R, Lim J, Mekary RA, et al. Traumatic spinal injury: global epidemiology and worldwide volume. World Neurosurg. 2018;113:e345–e363.
- Krueger H, Noonan VK, Trenaman LM, et al. The economic burden of traumatic spinal cord injury in Canada. Chronic Diseases In Canada. 2013;33(3):113–122.
- Anjum A, Yazid MD, Fauzi Daud M, et al. Spinal cord injury: pathophysiology, multimolecular interactions, and underlying recovery mechanisms. Int J Mol Sci. 2020;21(20):7533.
- Alizadeh A, Dyck SM, Karimi-Abdolrezaee S. Traumatic spinal cord injury: an overview of pathophysiology, models and acute injury mechanisms. Front Neurol. 2019;10:282.
- McDonald JW, Sadowsky C. Spinal-cord injury. Lancet. 2002;359(9304):417–425.
- Dizdaroglu M, Jaruga P, Birincioglu M, et al. Free radical-induced damage to DNA: mechanisms and measurement. Free Radic Biol Med. 2002;32(11):1102–1115.
- Beattie MS. Inflammation and apoptosis: linked therapeutic targets in spinal cord injury. Trends Mol Med. 2004;10(12):580–583.
- Kim DH, Vaccaro AR, Henderson FC, et al. Molecular biology of cervical myelopathy and spinal cord injury: role of oligodendrocyte apoptosis. Spine J. 2003;3(6):510–519.
- Vašíček O, Lojek A, Číž M. Serotonin and its metabolites reduce oxidative stress in murine RAW264.7 macrophages and prevent inflammation. J Physiol Biochem. 2020;76(1):49–60.
- Rui T, Wang Z, Li Q, et al. A TrkB receptor agonist N-acetyl serotonin provides cerebral protection after traumatic brain injury by mitigating apoptotic activation and autophagic dysfunction. Neurochem Int. 2020;132:104606.
- Yoo JM, Lee BD, Sok DE, et al. Neuroprotective action of N-acetyl serotonin in oxidative stress-induced apoptosis through the activation of both TrkB/CREB/BDNF pathway and Akt/Nrf2/Antioxidant enzyme in neuronal cells. Redox Biol. 2017;11:592–599.
- Li Q, Wang P, Huang C, et al. N-Acetyl serotonin protects neural progenitor cells against oxidative stress-induced apoptosis and improves neurogenesis in adult mouse hippocampus following traumatic brain injury. J Mol Neurosci. 2019;67(4):574–588.
- Manning BD, Toker A. AKT/PKB signaling: navigating the network. Cell. 2017;169(3):381–405.
- Vanhaesebroeck B, Guillermet-Guibert J, Graupera M, et al. The emerging mechanisms of isoform-specific PI3K signalling. Nat Rev Mol Cell Biol. 2010;11(5):329–341.
- Wymann MP, Zvelebil M, Laffargue M. Phosphoinositide 3-kinase signalling–which way to target? Trends Pharmacol Sci. 2003;24(7):366–376.
- Revathidevi S, Munirajan AK. Akt in cancer: mediator and more. Semin Cancer Biol. 2019;59:80–91.
- Long HZ, Cheng Y, Zhou ZW, et al. PI3K/AKT signal pathway: a target of natural products in the prevention and treatment of alzheimer’s disease and Parkinson’s disease. Front Pharmacol. 2021;12:648636.
- Chen J, Wang Z, Zheng Z, et al. Neuron and microglia/macrophage-derived FGF10 activate neuronal FGFR2/PI3K/Akt signaling and inhibit microglia/macrophages TLR4/NF-κB-dependent neuroinflammation to improve functional recovery after spinal cord injury. Cell Death Dis. 2017;8(10):e3090.
- Abbaszadeh F, Fakhri S, Khan H. Targeting apoptosis and autophagy following spinal cord injury: therapeutic approaches to polyphenols and candidate phytochemicals. Pharmacol Res. 2020;160:105069.
- Li F, Song X, Xu J, et al. Morroniside protects OLN-93 cells against H(2)O(2)-induced injury through the PI3K/Akt pathway-mediated antioxidative stress and antiapoptotic activities. Cell Cycle (Georgetown, Tex). 2021;20(7):661–675.
- Luo X, Zeng H, Fang C, et al. N-acetylserotonin derivative exerts a neuroprotective effect by inhibiting the NLRP3 inflammasome and activating the PI3K/Akt/Nrf2 pathway in the model of hypoxic-ischemic brain damage. Neurochem Res. 2021;46(2):337–348.
- Choudhury A, Singh S, Palit G, et al. Administration of N-acetylserotonin and melatonin alleviate chronic ketamine-induced behavioural phenotype accompanying BDNF-independent and dependent converging cytoprotective mechanisms in the hippocampus. Behav Brain Res. 2016;297:204–212.
- Giorgio M, Migliaccio E, Orsini F, et al. Electron transfer between cytochrome c and p66Shc generates reactive oxygen species that trigger mitochondrial apoptosis. Cell. 2005;122(2):221–233.
- Wiatrak B, Kubis-Kubiak A, Piwowar A, et al. PC12 cell line: cell types, coating of culture vessels, differentiation and other culture conditions. Cells. 2020;9(4):958.
- Zhang Q, Li RL, Tao T, et al. Antiepileptic effects of cicadae periostracum on mice and its antiapoptotic effects in H(2)O(2)-stimulated PC12 cells via regulation of PI3K/Akt/Nrf2 signaling pathways. Oxid Med Cell Longev. 2021;2021:5598818.
- Li RL, Zhang Q, Liu J, et al. Hydroxy-α-sanshool possesses protective potentials on H(2)O(2)-stimulated PC12 cells by suppression of oxidative stress-induced apoptosis through regulation of PI3K/Akt signal pathway. Oxid Med Cell Longev. 2020;2020:3481758.
- Spinal cord injury (SCI) 2016 facts and figures at a glance. J Spinal Cord Med. 2016;39(4):493–494.
- Circu ML, Aw TY. Reactive oxygen species, cellular redox systems, and apoptosis. Free Radic Biol Med. 2010;48(6):749–762.
- Silva NA, Sousa N, Reis RL, et al. From basics to clinical: a comprehensive review on spinal cord injury. Prog Neurobiol. 2014;114:25–57.
- Jia Z, Zhu H, Li J, et al. Oxidative stress in spinal cord injury and antioxidant-based intervention. Spinal Cord. 2012;50(4):264–274.
- Zhou H, Wang J, Jiang J, et al. N-acetyl-serotonin offers neuroprotection through inhibiting mitochondrial death pathways and autophagic activation in experimental models of ischemic injury. J Neurosci. 2014;34(8):2967–2978.
- Fatima G, Sharma VP, Das SK, et al. Oxidative stress and antioxidative parameters in patients with spinal cord injury: implications in the pathogenesis of disease. Spinal Cord. 2015;53(1):3–6.
- Pisoschi AM, Pop A. The role of antioxidants in the chemistry of oxidative stress: a review. Eur J Med Chem. 2015;97:55–74.
- Wang Y, Branicky R, Noë A, et al. Superoxide dismutases: dual roles in controlling ROS damage and regulating ROS signaling. J Cell Biol. 2018;217(6):1915–1928.
- Cinelli MA, Do HT, Miley GP, et al. Inducible nitric oxide synthase: regulation, structure, and inhibition. Med Res Rev. 2020;40(1):158–189.
- Lind M, Hayes A, Caprnda M, et al. Inducible nitric oxide synthase: good or bad? Biomed Pharmacothe. 2017;93:370–375.
- Minhas R, Bansal Y, Bansal G. Inducible nitric oxide synthase inhibitors: a comprehensive update. Med Res Rev. 2020;40(3):823–855.
- Marrocco I, Altieri F, Peluso I. Measurement and clinical significance of biomarkers of oxidative stress in humans. Oxid Med Cell Longev. 2017;2017:6501046.
- Rao S, Lin Y, Du Y, et al. Designing multifunctionalized selenium nanoparticles to reverse oxidative stress-induced spinal cord injury by attenuating ROS overproduction and mitochondria dysfunction. J Mat Chem B. 2019;7(16):2648–2656.
- Visavadiya NP, Patel SP, VanRooyen JL, et al. Cellular and subcellular oxidative stress parameters following severe spinal cord injury. Redox Biol. 2016;8:59–67.
- Grilo AL, Mantalaris A. Apoptosis: a mammalian cell bioprocessing perspective. Biotechnol Adv. 2019;37(3):459–475.
- Singh R, Letai A, Sarosiek K. Regulation of apoptosis in health and disease: the balancing act of BCL-2 family proteins. Nat Rev Mol Cell Biol. 2019;20(3):175–193.
- Van Opdenbosch N, Lamkanfi M. Caspases in cell death, inflammation, and disease. Immunity. 2019;50(6):1352–1364.
- Moujalled D, Strasser A, Liddell JR. Molecular mechanisms of cell death in neurological diseases. Cell Death Differ. 2021;28(7):2029–2044.
- Fricker M, Tolkovsky AM, Borutaite V, et al. Neuronal cell death. Physiol Rev. 2018;98(2):813–880.
- Lu J, Ashwell KW, Waite P. Advances in secondary spinal cord injury: role of apoptosis. Spine (Phila Pa 1976). 2000;25(14):1859–1866.
- Xie C, Yi J, Lu J, et al. N-Acetylcysteine reduces ROS-Mediated oxidative DNA damage and PI3K/Akt pathway activation induced by Helicobacter pylori infection. Oxid Med Cell Longev. 2018;2018:1874985.
- Zhu X, Liu S, Cao Z, et al. Higenamine mitigates interleukin-1β-induced human nucleus pulposus cell apoptosis by ROS-mediated PI3K/Akt signaling. Mol Cell Biochem. 2021;476(11):3889–3897.
- Yu W, Qin X, Zhang Y, et al. Curcumin suppresses doxorubicin-induced cardiomyocyte pyroptosis via a PI3K/Akt/mTOR-dependent manner. Cardiovasc Diagn Ther. 2020;10(4):752–769.
- Al Mamun A, Wu Y, Monalisa I, et al. Role of pyroptosis in spinal cord injury and its therapeutic implications. J Adv Res. 2021;28:97–109.