ABSTRACT
Liver injury from any number of causes (e.g. chemical material, drugs and diet, viral infection) is a global health problem, and its mechanism is not clearly understood. MicroRNAs (miRNAs) expression profiling is gaining popularity because miRNAs, as key regulators in gene expression networks, can influence many biological processes and have also shown promise as biomarkers for disease. Previous studies reported the regulation effects of miRNAs in liver injury, whereas function and molecular mechanisms of miR-322-5p were still unclear. Therefore, our study focused on the biological role of miR-322-5p in carbon tetrachloride (CCl4)-induced liver injury proliferation, apoptosis, and cell cycle. A mouse model of CCl4-induced liver injury was established, and the transcriptomes and miRNAs transcriptomes of 2d and 5d liver tissues after injury were sequenced. The expression of miR-322-5p and the cell cycle genes were detected in liver tissues and Hepa1-6 cell line by miRNA RT-PCR, qRT-PCR. The effects of miR-322-5p on liver cell proliferation, cell cycle and apoptosis were evaluated using MTS assays and flow cytometry analysis. The relationship between miR-322-5p and Wee1 was predicted and confirmed by bioinformatics analysis and a dual luciferase reporter assay. Functional experiments, including an MTS assay and flow cytometric analysis, were performed to study the effects of Wee1. MiR-322-5p was upregulated in injury liver tissues, and downregulated miR-322-5p was proved to inhibit proliferation, apoptosis and arrest cell cycle at G2/M in vitro. The dual-luciferase reporter assay results indicated that miR-322-5p has a binding site at position 285 in the Wee1 3´UTR. The effects of miR-322-5p in proliferation and cell cycle regulation can be abolished by Wee1 through rescue experiments. By directly targeting Wee1 influenced the expression of several cell cycle factors, including Cyclin dependent kinase 1 (Cdk1), cyclin B1 (Ccnb1) and Cell division cyclin 25C (Cdc25C). MiR-322-5p may function as a suppressive factor by negatively controlling Wee1, thus, highlighting the potential role of miR-322-5p as a therapeutic target for liver injury.
Abbreviations: ALT: Alanine aminotransferase; AST: Aspartate aminotransferase; GSH: Glutathione, γ-glutamyl cysteinel + glycine; CCl4: Carbon tetrachloride; HE: Haematoxylin and eosin; KEGG: Kyoto Encyclopedia of Genes and Genomes
KEYWORDS:
Introduction
The liver is known as the primary organ important for drug and chemical substance metabolism [Citation1]. Liver injury may be induced by drug abuse, viral infection and heavy alcohol consumption, and is considered to be a common clinical disease [Citation2]. Current studies have shown that compensatory growth is initiated after liver injury, which induces liver parenchymal cells to reenter the cell cycle; hepatocytes transition from the dormant G0 phase to prophase for DNA synthesis and then undergo mitosis [Citation3]. In the process of compensation, if the cell cycle progresses too fast or too slow, the repair and regeneration of liver cells will be disordered, the apoptotic cells will increase, and it may even lead to liver failure and canceration. Therefore, it is of great significance to deeply explore the regulation mechanism of the cell cycle after liver injury and find potential molecular therapeutic targets.
MicroRNAs (miRNAs) are short (19–25 nucleotides) noncoding RNAs that regulate target gene expression by influencing messenger RNA (mRNA) degradation and/or inhibiting RNA expression, and they have been found in different organisms [Citation4]. Previous data have shown that approximately 30% of mammalian mRNAs seem to be regulated by miRNAs [Citation5]. Recently, increasing evidence has indicated that miRNAs are promising diagnostic biomarkers for many diseases and disorders, including gastric cancer [Citation6], renal cell carcinoma [Citation7] and atherogenesis [Citation8]. Furthermore, miRNAs are also potential therapeutic targets. The role of miR-322-5p, previously known as miR-322, has been investigated in multiple biological processes. Inhibition of miR-322-5p protects cardiac myoblast cells against hypoxia-induced apoptosis and injury through regulating cytokine-induced apoptosis inhibitor 1 (CIAPIN1) [Citation9]. MiR-322-5p targets insulin-like growth factor 1 (IGF-1) and is suppressed in the heart of rats with pulmonary hypertension [Citation10]. MiR-322-5p alleviates vascular dementia development by targeting tetraspanin 5 (TSPAN5) which may provide a potential therapeutic target for dement [Citation11]. However, there has been no study that focused on the role of miR-322-5p in liver injury.
Wee1 is a tyrosine kinase that is a crucial component of the G2/M cell cycle checkpoint [Citation12]. Wee1 is a nuclear protein essential for a cell cycle transition into mitosis: by catalyzing the inhibitory phosphorylation of a tyrosine residue on the Cdc2/cyclin B kinase complex, Wee1 negatively regulates mitotic entry by deactivating the complex’s ability to regulate critical cell cycle proteins [Citation13]. Here, the downstream target gene of miR-322-5p may be Wee1. MiR-322-5p can affect the proliferation, apoptosis, and cell cycle progression of damaged cells by regulating Wee1 in liver injury process.
In conclusion, we investigated the functions of miR-322-5p and its correlations with Wee1 in liver injury. Our findings demonstrated that miR-322-5p downregulation has vital effects in regulating cell cycle and inhibiting proliferation and apoptosis of liver cells, providing miR-322-5p the role as a potential novel therapeutic target in liver injury.
Materials and methods
Animal models and treatment
C57BL/6 mice (male, 6–8 weeks old, 20–22 g, 80 mice) were purchased from Weitonglihua Co. (Beijing, China). The mice were given access to food and water. The room was maintained at a temperature of 20 ± 2°C with 60–70% relative humidity and a 12-hour light/dark cycle. The mice were divided into two groups (Grouping: Control, CCl4 injury group. Forty mice in the control group. Forty mice in the injury group. n = 5). Mice in the control group were injected with olive oil. Mice in all groups except the control group received CCl4 (10 mL/kg BW, diluted in olive oil, intraperitoneal injection). At the indicated time points after the injection, mice were anaesthetized with isoflurane, and blood was collected from the ophthalmic vein. Then, the mice were euthanized. The liver was removed and quickly sectioned and was then immediately frozen in liquid nitrogen. Then, the separated liver sections were stored at ‒80°C until analysis.
Determination of ALT and AST activity
The levels of ALT and AST in serum were assessed using commercially available diagnostic kits (NanJing Jiancheng Bio Co. Ltd, China) to evaluate ALT and AST activity.
HE staining and Masson’s trichrome staining
Liver tissue sections from the same part of the liver lobe were cut, fixed with 4% buffered formalin at room temperature for 24 hours, embedded in paraffin, and sliced into 4 μm thick sections. HE staining: Tissue sections were obtained, and paraffin was melted at 65°C for 20 min in a tissue slicer. After dewaxing and hydration, the sections were stained with hematoxylin for 3 min. The sections were immersed in 1% hydrochloric acid and ethanol for 3 s, stained with 0.5% eosin for 3 min, dehydrated and sectioned at room temperature. Images were acquired after drying.
Masson’s trichrome staining: After sections were deparaffinized and hydrated, they were fixed with Bouin’s solution for 2 hours, after which lapis lazuli staining solution was dripped onto the sections for 3 min, Mayer’s hematoxylin staining solution was dripped onto the sections for 3 min, and the sections were differentiated in 1% hydrochloric acid–ethanol differentiation solution for 8 s. Acid fuchsin staining solution was dripped onto the sections for 10 min, and the sections were then treated with phosphomolybdic acid solution for 10 min. The liquid was discarded, and aniline blue staining solution was directly dripped onto the sections for staining for 5 min, after which the sections were treated with a weak acid solution for 2 min, dehydrated and dried for imaging.
Transcriptome data analysis
Based on the transcriptome sequencing results, differentially expressed genes were compared, cell cycle pathways were screened on the analysis platform, enrichment of differentially expressed genes and pathways was analyzed with the Metascape online tool (http://metascape.org/), and the results were visualized using Cytoscape software. Based on the results of the noncoding RNA sequencing and differential gene expression mapping for the cell cycle pathway obtained via interaction analysis with Cytoscape software, TargetScan 7.2 (http://www.targetscan.org/), miRDB (http://mirdb.org/), Miranda, etc., were used to take the intersection of the miRNAs predicted by the online data analysis tools and obtain the overlapping prediction results.
Quantitative real-time PCR
Total RNA was extracted from tissues and Hepa1-6 cells to verify the mRNA expression level (TRIzol solution, TaKaRa). The relative mRNA levels were determined using the comparative Ct method with β-actin or U6 as the reference gene, and the formula 2 -ΔΔCt. All primers are listed in .
Table 1. Primer sequences
Immunohistochemical analysis
Immunohistochemical analysis was performed using deparaffinized liver sections. The sections were blocked with 3% goat-serum at room temperature for 30 minutes. Then add the primary antibody and incubate overnight at 4°C. After washing with PBS, the sections were treated with a secondary antibody for 50 minutes at room temperature. Then, they were immersed in PBS for 5 minutes, and then stained with DAB and hematoxylin. The section was examined in a blinded manner. The percentage of positive areas and the intensity of the cell staining were determined based on three randomly selected regions per section. Staining was scored according to intensity and percentage of positive cells. The staining intensity was scored according to 4 grades: 0 (No staining), 1 (weak staining), 2 (intermediate staining), or 3 (strong staining). The product (percentage of positive cells and respective intensity scores) was used as the final staining score (a minimum value of 0 and a maximum of 300).
Construction of sh-Wee1
Use the online tool BLOCK-iT™ RNAi Designer (https://rnaidesigner.thermofisher.com/rnaiexpress/sort.do) on the Life Technologies website to design shRNA sequences and add restriction site sequence synthesis. The vector (pGPU6/GFP/Neo) was ligated to the desired fragment that contained the same restriction sites at the ends. The pGPU6/GFP/Neo plasmid (Kan+) was purchased from Wuhan Miaoling Biotechnology Co., Ltd. The sequence of sh-Wee1 is shown in .
Table 2. shRNA–Wee1 sequence
Mimics & inhibitor
For miR-322-5p measurement, RNA was reverse transcribed (RT) using TaqMan primers specific for miR-322-5p and U6 (Applied Biosciences) suspended in 1x TE buffer in accordance with the manufacturer’s instructions. cDNA was used for qPCR with TaqMan fluorescent probes for miR-322-5p and U6 according to the manufacturer’s instructions.
RNA oligos (miR-322-5p mimics and inhibitor) were designed and synthesized by Suzhou Gemma. .
Table 3. Sequences of the miR-322-5p mimic and inhibitor.
Cell culture
The mouse hepatocyte line Hepa1-6 was used in this study and routinely cultured in DMEM. Hepa1-6 cells were cultures in medium supplemented with 10% FBS and 1% penicillin/streptomycin at 37°C in a humidified atmosphere containing 5% CO2.
Cell cycle assay
Transfected cells were harvested via trypsinization and washed with cold PBS. Precooled 70% ethanol (500 μL) was added to the cells for overnight fixation at 4°C. Cells were centrifuged in PBS to remove the fixative before the experiment; then, 100 μL of RNaseA solution was added to the cell pellet, and the cells were resuspended and incubated in a 37°C water bath for 30 minutes. PI (Solarbio) staining solution (400 μL) was added and incubated for 30 minutes at 4°C in the dark; then, staining was analyzed with a computer, and the red fluorescence intensity was recorded at an excitation wavelength of 488 nm.
Cell proliferation assay
Cell proliferation was evaluated using an MTS assay in Hepa1-6 cells. Approximately 2 × 103 transfected cells per well were seeded in 96-well plates and cultured at 37°C. After incubation for 24 h, 48 h, 72 h, and 96 h, 20 uL of MTS (Sigma) reagent was added incubation for 4 hours and determined at a wavelength of 490 nm.
Target prediction and luciferase reporter assay
MiRBase sequence information for microRNAs potentially binding to the NCBI Wee1 3´UTR sequence was uploaded to the online prediction tool RNAhybrid (https://bibiserv.cebitec.uni-bielefeld.de/rnahybrid/). The binding energy and binding sites were analyzed and predicted. Randomly mutate the sequences at positions 285 and 556 in the 3ʹUTR region of the Wee1 gene. WT-285 construct does not contain WT-556 and WT-556 construct does not contain WT-285 too. MU-285 construct does not contain MU-556 and MU-556 construct does not contain MU-285 too. The vectors of Wee1 wild type (GP-miRGLO-Wee1-W) or mutant (GP-miRGLO-Wee1-M) were framed and co-transfected, respectively, with miR-322-5p mimics or corresponding control (miR-NC). After 48 hours, we used Dual-Luciferase Reporter Assay System (Promega) to measure luciferase activity differences.
Statistical analysis
All data were analyzed with GraphPad Prism 7.0 software (La Jolla, CA, USA) and are shown as the mean ± SD values unless otherwise stated. One-way analysis of variance (ANOVA) was used to compare differences among more than three groups. Statistical analyses to compare differences between two groups were performed using Student’s t-test. P < 0.05 was considered to indicate a significant difference.
Results
Liver cells were actively proliferating after liver injury
Time-course assays showed that serum ALT, AST, and ALT/AST markedly increased on the 2d after CCl4 injection ()). A decrease in the GSH content is a potential activation signal of early apoptosis. The GSH content in the livers of mice decreased to a minimum on the 2d after injury ()). Liver tissue morphology was analyzed on 2d, 5d, 7d, and 14d after injury by HE staining and Masson’s trichrome staining. HE staining showed that CCl4-induced damage peaked on the 2d, which caused coagulative necrosis in more than 1/2 of the liver area ()). Masson’s trichrome staining showed that the liver began to synthesize a large amount of collagen to fill the damaged area at 48 h after injury ()). The above results indicated that the CCl4 induced-liver injury model was successfully constructed.
Figure 1. Liver cells were actively proliferating after liver injury.Average optical density of ALT/AST tested of the serum levels and average optical density of GSH tested of the liver tissue (2d, 5d, 7d, 14d) (n = 5); (b) The liver histopathology was analyzed by HE staining and Masson staining (Scale bar, 50 μm), (2d, 5d, 7d, 14d) (n = 5); (c) Hepatic histopathological examination by PCNA immunohistochemistry (Scale bar, 50 μm), (1d, 2d, 3d, 5d, 7d, 14d) (n = 5); The area of positive staining was marked by arrows; (d) The mRNA expression of PCNA, P21, p53 was detected using qPCR and β-actin was used as a loading control at different time points after injury (1d, 2d, 3d, 5d, 7d, 14d) (n = 5). The data are presented as the mean ± SD of three independent experiments. *P < 0.05, **P < 0.01.
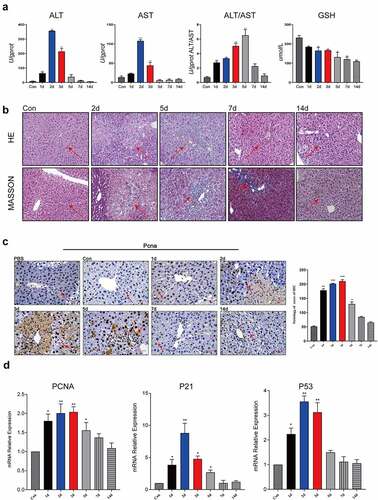
Proliferating cell nuclear antigen (PCNA) expression is a good indicator of the cell proliferation status. PCNA immunohistochemistry results showed that the nuclear expression of PCNA in liver cells in the injured area was significantly increased, and these liver cells were actively proliferating. On the 1d after injury, obvious hepatocyte damage was observed in the area from the hepatic portal vein to the central vein. On the 2d after injury, a large number of hepatocytes in the injured area exhibited coagulative necrosis, and nuclear PCNA expression in the liver cells at the edge of the necrotic area was still high. On the 3d after injury, PCNA was still highly expressed in the nuclei of liver cells at the edge of the injured area, and nuclear PCNA expression in the distal liver cells was also significantly increased. On the 5d after injury, the expression of PCNA began to diminish, and the injured area was repaired by the proliferated liver cells, but there was still obvious inflammatory cell infiltration near the hepatic vein after this repair, and the nuclear PCNA expression level in the liver cells was relatively high. On the 7d and 14d after injury, damage repair was basically completed, and PCNA was basically no longer expressed ()). The mRNA levels of proliferation-related genes PCNA, P21, and P53 were also significantly elevated during liver injury ()). The study showed that liver cells were actively proliferating after liver injury.
The cell cycle pathway was activated after liver injury
We performed transcriptome sequencing of liver tissue (Con, 2d, and 5d). The results of KEGG pathway and GO enrichment analysis showed that the top 10 pathways included the cell cycle pathway ()). Further overlap analysis between groups found that a total of 66 genes in cell cycle pathways were significantly changed in the CCl4-induced liver injury ()). The heat map of 66 differential genes showed that the transcription factor E2f family genes involved in cell cycle and apoptosis pathway, Mcm family genes involved in DNA replication and some genes of cell cycle checkpoint were actively expressed on 2d. The expression of some cyclins was up-regulated on 5d ()). The key nodes of the protein interaction network are cyclins such as Ccnb1, Ccna1, and Ccna2, and cyclin-dependent kinases such as Cdc20, Cdk2, and Cdkn1a. These key genes are involved in the regulation of key cycle kinase activities in cell mitosis, especially in G1/S and G2/M phases ()). The levels of Ccnb1, Cdc25c, and Chk1 cell cycle-related genes were further verified, and the results were consistent with the transcriptome data (). The study showed that the cell cycle pathway was activated after liver injury.
Figure 2. The cell cycle pathway was activated in liver injury. The top 10 up-regulated signaling pathways in the Con and CCl4 group in KEGG enrichment and GO enrichment; (b) Circos overlap analysis of differentially expressed genes; (c) Heatmaps of differentially expressed genes related to the cell cycle signaling pathway. The abscissa represents the sample name and the clustering results of the samples, and the ordinate represents the differential genes and the clustering results of the genes. Different columns in the figure represent different samples, and different rows represent different genes. The color represents the gene expression level log2 in the sample; (d) Module in protein–protein interaction network of cell cycle-related genes; (e) The mRNA expression of Ccnb1, Cdc25c and Chk1 was detected using qPCR and β-actin was used as a loading control at different time points after injury (1d, 2d, 3d, 5d, 7d, 14d) (n = 5). Sequenced samples were divided into the Con group, 2d injury group and 5d injury group (n = 3 in each group), and P < 0.05 was used as the significance criterion for differential gene expression. The data are presented as the mean ± SD of three independent experiments. *P < 0.05, **P < 0.01.
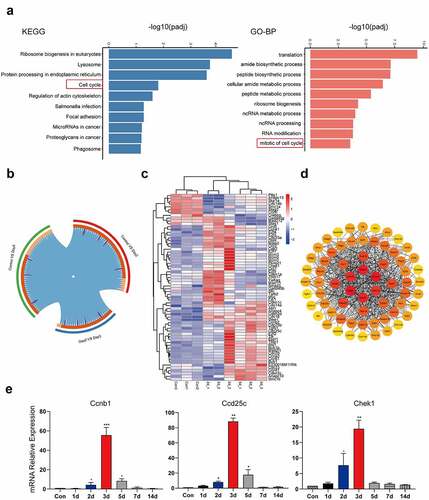
miR-322-5p was upregulated in liver tissues after liver injury
In order to investigate the expression level of miR-322-5p after liver injury, we performed miRNA transcriptome sequencing of liver tissue (Con, 2d, and 5d). The results showed that 93 miRNAs were up-regulated on the 2d after liver injury, and 125 miRNAs were up-regulated on the 5d after liver injury ()). There were 47 miRNAs that were simultaneously up-regulated at these two time points ()). Interestingly, these 47 miRNAs included miRNA-322-5p ()). q-PCR results also showed that miR-322-5p was significantly decreased after liver injury ()).
Figure 3. miR-322-5p was upregulated in liver tissues after liver injury. Volcano plot of differential miRNAs on the 2d after liver injury and Volcano plot of differential miRNAs on the 5d after liver injury; (b) Venn diagram showing intersection of up-regulated miRNAs on 2d and 5d; (c) Heatmaps of differentially expressed miRNAs (Con, 2d, 5d). The abscissa represents the sample name and the clustering results of the samples, and the ordinate represents the differential genes and the clustering results of the genes. Different columns in the figure represent different samples, and different rows represent different genes. The color represents the gene expression level log2 in the sample; (d) Transcriptome data showed the expression of miRNA-322-5p on the 2d and 5d after liver injury; (e) The mRNA expression of miRNA-322-5p was detected using qPCR at different time points after injury (1d, 2d, 3d, 5d, 7d, 14d) (n = 5). Sequenced samples were divided into the Con group, 2d injury group and 5d injury group (n = 3 in each group), and P < 0.05 was used as the significance criterion for differential gene expression. The data are presented as the mean ± SD of three independent experiments. *P < 0.05, **P < 0.01.
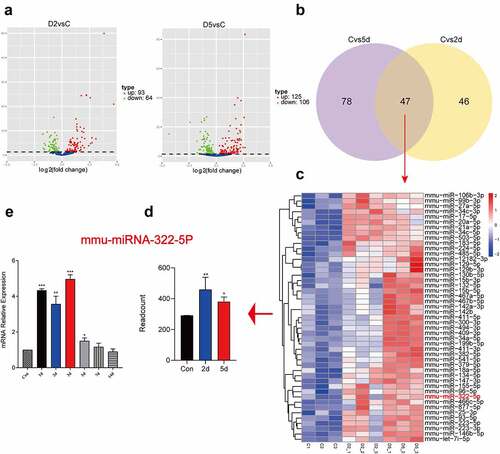
MiR-322-5p promotes the proliferation and apoptosis reduces cell cycle at G2/M phase
To transfect the miR-322-5p mimics or inhibitor, respectively, and analyze the function of miR-322-5p in Hepa1-6 cells. MiRNA RT-PCR confirmed the transfection efficiency. The results were shown in ()), relative expression of miR-322-5P in Hepa1-6 mimics and inhibitor was significant higher and lower, respectively, than the control group. We carried out MTS assay to evaluate the influence of miR-322-5p in proliferation. As shown in ()), results of MTS showed that upregulated miR-322-5P promoted the proliferation while downregulated miR-322-5P tends out the opposite effects in Hepa1-6. We used flow cytometry to analyze the effects of dysregulated miR-322-5p in cell cycle, results were shown in ()), overexpressed miR-322-5P presented a significant reduce in the percentage of cells in the G2/M phase while downregulated miR-322-5p indicated cell cycle was arrest in G2/M phase in Hepa1-6. Results of apoptosis were shown in ()), the number of Hepa1-6 cells apoptotic ratio in the miR-322-5p mimics treated group was distinctly increased compared with the number in the control group, while the cells transfected with the miR-322-5p inhibitor revealed a significant decreased in cell apoptotic compared with that of the control group. These findings demonstrated that overexpressed miR-322-5P promoted Hepa1-6 cell proliferation, apoptotic and reduces cell cycle arrest in G2/M phase.
Figure 4. MiR-322-5p promotes the proliferation and apoptosis reduces cell cycle at G2/M phase.(a) MiRNA RT-PCR was used to confirm the expression of miR-322-5P in cells transfected with miR-322-5P mimics and miR-322-5P inhibitor in turn; (b) Effects of miR-322-5P on proliferation in Hepa1-6 cell lines were detected by MTS; (c) Effects of miR-322-5P on regulating cell cycle in Hepa1-6 cell lines; (d) Effects of miR-322-5p on regulating apoptosis in Hepa1-6 cell lines. The data are presented as the mean ± SD of three independent experiments. *P < 0.05, **P < 0.01.
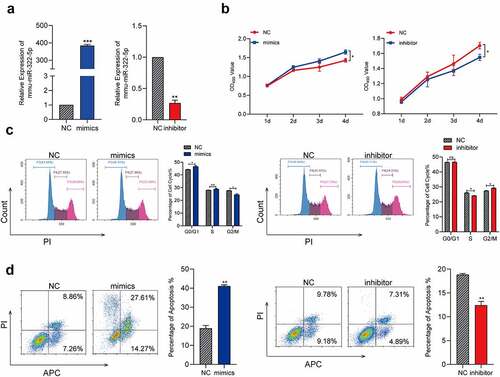
MiR-322-5p suppresses Wee1 expression via interacting directly with a hypothetic binding site of Wee1 3´UTR
To predict the potential target of miR-322-5p, we used bioinformatics tools in public database including TargetScan, miRanda, miRDB combined with the sequencing results ()). Wee1 was identified as the target gene of miRNA-322-5p. The association analysis of miRNA transcriptome data and differentially expressed gene transcriptome data also showed that Wee1 was identified as the target gene of miRNA-322-5p ()). Based on the sequence information of the Wee1 3´UTR and the sequence information of miR-322-5p, the sequence structure and binding energy of the possible seed region were plotted. The binding regions in the Wee1 3´UTR were predicted to be located at 285 bp and 556 bp ()). We further utilized dual-luciferase reporter assays to verify our prediction. Mutant-type (MUT) and wild-type (WT) Wee1-3’-UTR sequences (the containing site including 285 bp and 556 bp sites) were cloned into reporter plasmids. The dual-luciferase reporter assay showed that miR-322-5p bound to the site at positions 285 in the Wee1 3´UTR ()). However, no significant reduction was observed about luciferase activity in group co-transfected with pmirGLO-MUT-Wee1-3’-UTR-556 and miR-322-5p mimics ()). Expression of miRNA-322-5P and its correlation with Wee1 were also detected. In Hepa1-6 transfected with miR-322-5p mimics, we identified Wee1 is downregulated compared with miR-NC treated group through RT-PCR assay. The reverse changes of Wee1 were also observed in Hepa1-6 transfected with miR-322-5p inhibitor ()). The above results indicate that miR-322-5p has a binding effect on positions 285 in the 3´UTR region of Wee1.
Figure 5. MiR-322-5p suppresses Wee1 expression via interacting directly with a hypothetic binding site of Wee1 3´UTR.(a) A Venn diagram shown that sequencing analysis was combined with online tools to predict miRNA-322-5p targeting Wee1; (b) Potential interaction network of the differentially expressed miRNAs and differentially expressed genes in the cell cycle pathway. The red circle indicates the selected pair Wee1 and miR-322-5p, and they have a targeted effect; (c) RNAhybrid was used to predict the binding energy for miR-322-5p and its target Wee1, the wild-type and mutated binding sites in the Wee1 3ʹUTR; WT, wild-type; Mu, mutated, red + green indicates the predicted binding seed sequences, and green indicates random mutated sequences; (d) The dual luciferase reporter assay showed that miR-322-5p bound to the site in the Wee1 3ʹUTR; (e) Expression of Wee1 in Hepa1-6 cell lines transfected with relevant plasmid was detected by qRT-PCR. The data are presented as the mean ± SD of three independent experiments. *P < 0.05, **P < 0.01.
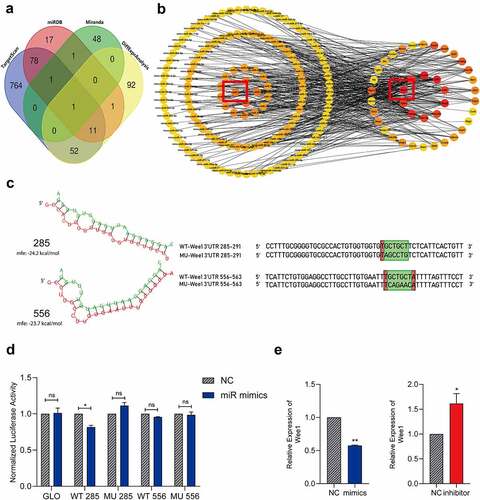
MiR-322-5p promotes the proliferation and apoptosis and regulates cell cycle by targeting Wee1
To identify the effect of Wee1 on the cell cycle, we knocked down Wee1 in Hepa1-6 cells ()). The results showed that the proliferation of Hepa1-6 cells was significantly enhanced after Wee1 was knocked down ()). The cell cycle analysis results showed that the proportion of cells in G2/M phase was significantly decreased ()), suggesting that knockdown of Wee1 can promote the G2/M transition. Previous studies have revealed that Wee1 as a G2/M checkpoint can influence the cell cycle by interacting with some key factors. In our study, we wonder whether Wee1 could influence any key factors in cell cycle in Hepa1-6, we detected the expression of some key factors in cell cycle by qRT-PCR, including Pcna, P21, P53, CDK1, CCNB1, and Cdc25c. The results showed that cell cycle-related genes were significantly upregulated ()). We also found that after knocking down Wee1, the proportion of apoptosis increased ()). Apoptosis genes are upregulated, anti-apoptotic genes are downregulated ()). We found in vitro experiments that the inhibition of Wee1 significantly reduced cell G2/M retention, and improved the proliferation ability of cells, but increased the number of apoptotic cells. The increase in the proportion of apoptosis may be due to the rapid passage of cells in the G2/M phase, which reduces the preparation time for cell mitosis and reduces the process of DNA replication inspection. In summary, the expression of Wee1 after injury is essential for cell proliferation, cell cycle, and apoptosis of liver cells.
Figure 6. MiR-322-5p promotes the proliferation and apoptosis and regulates cell cycle by targeting Wee1.(a) RT-PCR was used to confirm the expression of Wee1 in cells transfected with plasmid sh-Wee1; (b) Effects of Wee1 on proliferation in Hepa1-6 cell lines were detected by MTS; (c) Effects of Wee1 on regulating cell cycle in Hepa1-6 cell lines; (d) The mRNA expression of Cdk1, Cyclin B1, Cdc25c, Pcna, P21 and P53 gene after knocking down Wee1 by RT-PCR; (e) Effects of Wee1 on regulating apoptosis in Hepa1-6 cell lines. (f) The mRNA expression of Caspase3, Caspase7, Bax, Bcl-2, Bcl-w and Bcl-xl gene after knocking down Wee1 by RT-PCR. The data are presented as the mean ± SD of three independent experiments. *P < 0.05, **P < 0.01.
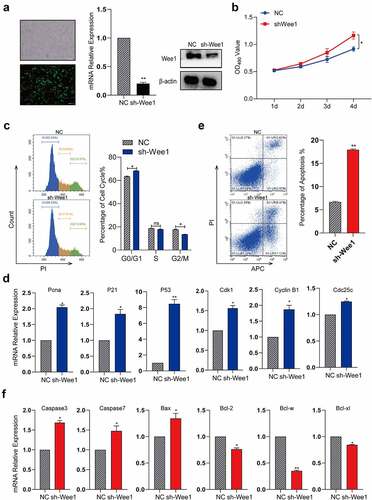
MiR-322-5p promotes the proliferation and apoptosis and regulates cell cycle through Wee1
To further investigate the influence of interaction between miR-322-5p and Wee1 in Hepa1-6, we conducted the rescue experiments. In our rescue experiments, we designed one comparisons, the comparison: Hepa1-6 cells transfected with NC vs. Hepa1-6 cells sh-Wee1 vs. Hepa1-6 cells co-transfected with miR-322-5p inhibitor and sh-Wee1. Effect of increasing Hepa1-6 proliferation made by downregulated Wee1 can be counteracted by downregulated miRNA-322-5P ()). We also used flow cytometric to explore whether the effects of miR-322-5p on cell cycle were mediated by the regulation of Wee1. It turned out that downregulated miR-322-5p can abolish cell cycle G2/M arrest caused by downregulated Wee1 in Hepa1-6 cells ()). Likewise, downregulated miR-322-5p abolished the effect of downregulated Wee1 in promoting Hepa1-6 apoptosis ()). These findings suggested that miR-322-5P promotes the proliferation and apoptosis and regulates cell cycle by directly targeting Wee1.
Figure 7. MiR-322-5p promotes the proliferation and apoptosis and regulates cell cycle through Wee1.(a) After simultaneous miR-322-5p inhibitor and Wee1 shRNA down-regulation was transferred into Hepa1-6 cells, the cell proliferation did not change significantly; (b) After simultaneous miR-322-5p inhibitorand Wee1 shRNA down-regulation was transferred into Hepa1-6 cells, cell cycle did not change significantly; (c) After simultaneous miR-322-5p inhibitorand Wee1 shRNA down-regulation was transferred into Hepa1-6 cells, the apoptosis did not change significantly. The data are presented as the mean ± SD of three independent experiments. *P < 0.05, **P < 0.01.
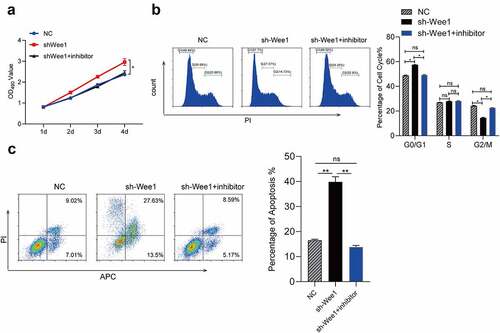
Discussion
Acute massive or chronic persistent liver injuries can lead to liver failure. Developing a cell-based treatment to reduce damage, prevent progression, and restore liver function is of important clinical relevance. CCl4 is the chemical most commonly used to induce liver injury [Citation14]. In severe cases, CCl4 can cause tissue lesions such as large-scale hepatocyte necrosis, ballooning degeneration, inflammatory cell infiltration, vascular occlusion, loss of hepatic trabecular structure, hepatic fibrosis, vascular degeneration, and calcification [Citation15–18]. In our study, it was also observed that a large area of diffuse degeneration and necrosis along the central hepatic vein, with a disordered arrangement of hepatocytes and edema in most cases. Under microscopic examination of hepatic cords, the injured area was found to encompass 2/3 of the region, and some inflammatory cell infiltration was visible. This indicates that the CCl4-induced liver injury model was successfully constructed. It is of great importance to fully understand the changes in pathways after liver injury via the CCl4 model, a classical model, to prevent the progression of liver disease.
Oxidative stress caused by CCl4 can also trigger the cellular stress response and control the G2/M transition in the cell cycle, resulting in an imbalance between cell proliferation and apoptosis and eventually leading to cell damage and even cell death [Citation19]. CCl4 toxicity can also activate p53 and P21, leading to cell growth arrest, and liver polyploidy can be seen [Citation20]. Wang XH et al. [Citation21] found that CCl4-induced injury to the mouse liver not only mediated accelerated hepatocyte entry into mitosis through p21 via the same mechanism as partial liver resection (PH) with expression of the same M-phase cyclin genes (Ccnb1, Ccnb2, and Ccnf) but also promoted M-phase entry by stimulating Cdc25b expression. In addition, Bangen JM et al. [Citation22] proposed that continuous blockade of Ccne1 expression in hepatocytes prevented early compensatory proliferation of CCl4-damaged hepatocytes and rapidly triggered the proliferative tissue repair function. In this study, the transcriptome sequencing results showed significant changes in key genes involved in the cell cycle, apoptosis, carbon metabolism, inflammatory response and other pathways, and the RT–PCR results showed that changes in genes involved in apoptosis, the cell cycle and other functions occurred mainly within 72 h after injection of CCl4, a finding that was basically consistent with the results of model studies in the existing literature [Citation23,Citation24]. Caspase-3 plays a catalytic role in apoptosis and is responsible for chromatin condensation and DNA fragmentation, while Bcl-2, located in the outer mitochondrial membrane, inhibits proapoptotic proteins and promotes cell survival [Citation25,Citation26]. The RT–PCR results showed that Casp3 expression was significantly up-regulated and Bcl2 expression was significantly down-regulated on Day 1 after injury, indicating that liver apoptosis was active at that time. Studies have shown that PCNA is involved in the key steps of DNA synthesis and repair [Citation27]. The RT–PCR analysis results have shown that PCNA is always activated within 3 days after injury. According to the results of tissue section staining, large areas of necrotic tissue appeared around the central vein after injury, and immunohistochemical staining showed a large number of pyknotic cells in the necrotic area. Binucleated cells appeared at the junction with adjacent normal tissues, and PCNA was highly expressed, suggesting that hepatocytes at the edges of the damaged tissues were actively proliferating. The above results indicated that apoptosis and repair of cells might occur simultaneously during liver injury.
MiR-322 is an miRNA located on chromosome X, which is abundantly expressed in various types of species [Citation28]. MiR-322 has previously been investigated as a vital regulator in the cell cycle, and cell proliferation, formation, and differentiation [Citation29,Citation30]. Current studies of miR-322-5p have focused on its roles in cardiac hypertrophy [Citation31], cardiac cell differentiation [Citation32], and atherosclerosis [Citation33] and other diseases. Its homologue, miR-424-5p, has been indicate to regulate cell proliferation and apoptosis in liver cancer [Citation34], but its role in liver injury has not been reported. In the present study, we found that miR-322-5p was significantly elevated on the second and fifth days after liver injury in miRNA sequencing date. Stepwise investigation revealed the function of miR-322-5p in proliferation, apoptosis, and cell cycle both in vitro. The results showed that upregulated miR-322-5p can promote proliferation and apoptosis in vitro and reduce cell cycle arrest in G2/M phase. In contrast, the cell arrest in G2/M phase and other reverse effects can be observed in downregulated miR-322-5P groups. These findings demonstrated that upregulated miR-322-5P can serve as a regulator in the process of liver injury. We found many miRNAs that were up- and down-regulated during liver injury, unfortunately the functions and roles of other miRNAs were not reflected in our study. Although this ability was not reflected in our article, it has been incorporated into our next research plan.
To further research the mechanism of miR-322-5p in liver injury, we used bioinformatics tools to predict the putative targets of miR-322-5p. Among these candidate target mRNAs, we chose to investigate Wee1. Wee1 is a kinase that regulates replication stress and cell cycle arrest at the G2/M checkpoint. During liver regeneration, the G2/M transition controls the periodic proliferation of hepatocytes. After completion of DNA replication, cells regulate the activity of the MPF complex through the regulation of Wee1 transcription, affecting the G2/M transition [Citation35]. Inhibition of Wee1 activity also leads to high levels of replication pressure and premature entry into mitosis [Citation36,Citation37]. Chen F et al. [Citation38]reported that PH activates the cell cycle reentry program in hepatocytes but at the same time limits cell cycle progression and delays liver regeneration by triggering the expression of negative cell cycle regulators such as p53, CHK1, and Wee1. Based on differential gene expression analysis of the cell cycle pathway, the expression of Wee1 decreased on Day 3, and the downstream regulation of Cdk1 activity increased significantly on Day 3, indicating that Wee1 expression was negatively correlated with Cdk1 expression after injury. In the early stage of damage, high expression promotes the DNA damage repair process, and lower expression then promotes the activation of the MPF complex and induces mitosis. Further in vitro experiments were conducted to explore the effects of Wee1, and it was found that inhibition of Wee1 significantly reduced the retention of cells in G2/M phase, and improved the cell proliferation, apoptosis ability. The dual-luciferase reporter assay showed that the seed sequence located at bp 285 in the Wee1 3´UTR was a binding site for miR-322-5p and that miR-322-5p might exert a targeted regulatory effect on Wee1 through this site. After increasing/decreasing miR-322-5p expression, the expression of Wee1 was inversely regulated. Subsequent cell proliferation, cell cycle, and apoptosis showed that an increase in miR-322-5p expression promoted cell proliferation, apoptosis and decreased the proportion of G2/M cells, possibly through the targeting of Wee1. Liver injury can cause a series of complex pathway changes. In our study, we only focused on the pathway that miR-322-5p affects cell cycle pathways by targeting Wee1, thereby affecting cell proliferation and apoptosis. Although Wee1 is fundamental for proper cell cycle progression and has been extensively investigated in this regard, the global effects of regulating this kinase remain to be elucidated in liver injury.
In conclusion, our study indicated that miR-322-5p can suppress the cell proliferation and apoptosis of liver injury through influencing cell cycle by targeting Wee1 directly and provided miR-322-5p the role as a novel therapeutic target for liver injury.
Authors’ contributions
All authors read and approved the final manuscript. H.T. and L.W. contributed to the conception and design of the work, acquired the majority of the data and drafted the manuscript. J.S. and H.J. contributed to the conception and design of the work, and acquired some of the data. K.Z. and Y.B. and Y.W. and Y.C. contributed to the design of the work and to interpretation of data. C.W. and P.L. contributed to the design of the work, to interpretation of data and substantively revised the manuscript.
Data Availability
All data generated or analysed during this study are included in this manuscript.
Ethics approval
The study was conducted according to the guidelines of the Declaration of Helsinki. All animal related experimental protocols applied in this study were conducted under the standards of Ethics Committee of Inner Mongolia Medical University (SCXK2016-0001).
Supplemental Material
Download Zip (769.9 KB)Acknowledgments
We thank Spring Nature. Co., Ltd. for the language modification.
Disclosure statement
No potential conflict of interest was reported by the authors.
Supplementary material
Supplemental data for this article can be accessed online at https://doi.org/10.1080/15384101.2022.2108128
Additional information
Funding
References
- Trefts E, Gannon M, Wasserman DH. The liver. Curr Biol. 2017;27(21):R1147–r1151.
- Aghemo A, Alekseeva OP, Angelico F, et al. Role of silymarin as antioxidant in clinical management of chronic liver diseases: a narrative review. Ann Med. 2022;54(1):1548–1560.
- Miyaoka Y, Ebato K, Kato H, et al. Hypertrophy and unconventional cell division of hepatocytes underlie liver regeneration. Curr Biol. 2012;22(13):1166–1175.
- Lu TX, Rothenberg ME. MicroRNA. J Allergy Clin Immunol. 2018;141(4):1202–1207.
- Lewis BP, Burge CB, Bartel DP. Conserved seed pairing, often flanked by adenosines, indicates that thousands of human genes are microRNA targets. Cell. 2005;120(1):15–20.
- Kao CK, Liebovitz DM. Consumer mobile health apps: current state, barriers, and future directions. PM & R. 2017;9:S106–s115.
- Petrozza JC. Value of versatility in fibroid surgery. Fertil Steril. 2017;108(3):453–454.
- Vilahur G, Gutiérrez M, Casani L, et al. Response by Vilahur et al to letters regarding article, “protective effects of ticagrelor on myocardial injury after infarction”. Circulation. 2017;135(17):e1004–e1005.
- Cai X, Wang S, Hong L, et al. Inhibition of miR-322-5p protects cardiac myoblast cells against hypoxia-induced apoptosis and injury through regulating CIAPIN1. J Cardiovasc Pharmacol. 2021;77(2):200–207.
- Connolly M, Garfield BE, Crosby A, et al. miR-322-5p targets IGF-1 and is suppressed in the heart of rats with pulmonary hypertension. FEBS Open Bio. 2018;8(3):339–348.
- Zheng W, Zhang J, Zhou B, et al. MiR-322-5p alleviates cell injury and impairment of cognitive function in vascular dementia by targeting TSPAN5. Yonsei Med J. 2022;63(3):282–291.
- Al-Jamaei AAH, de Visscher J, Ramadugula VS, et al. WEE1 kinase inhibitor MK-1775 sensitizes oral tongue squamous cell carcinoma cells to radiation irrespective of TP53 status. Oral Dis. 2022. DOI:10.1111/odi.14269.
- Dinavahi SS, Chen YC, Punnath K, et al. Targeting WEE1/AKT restores p53-dependent natural killer-cell activation to induce immune checkpoint blockade responses in “cold” melanoma. Cancer Immunol Res. 2022;10(6):757–769.
- Mohi-Ud-Din R, Mir RH, Sawhney G, et al. Possible pathways of hepatotoxicity caused by chemical agents. Curr Drug Metab. 2019;20(11):867–879.
- Dutta S, Chakraborty AK, Dey P, et al. Amelioration of CCl4 induced liver injury in swiss albino mice by antioxidant rich leaf extract of croton bonplandianus baill. PloS one. 2018;13(4):e0196411.
- Z H, Viswanathan R, Cheng H, et al. Inhibiting WEE1 and IKK-RELA crosstalk overcomes TNFα resistance in head and neck cancers. Mol Cancer Res. 2022;20(6):867–882.
- Huang X, Wang L, Meng M, et al. Extract of averrhoacarambola L. (oxalidaceae) roots ameliorates carbon tetrachloride-induced hepatic fibrosis in rats. Biomed Pharmacothe. 2020;121:109516.
- Sahreen S, Khan MR, Khan RA. Ameliorating effect of various fractions of rumex hastatus roots against hepato- and testicular toxicity caused by CCl4. Oxid Med Cell Longev. 2013;2013:325406. 2013.
- Meyer NJ, Huang Y, Singleton PA, et al. GADD45a is a novel candidate gene in inflammatory lung injury via influences on Akt signaling. FASEB J. 2009;23(5):1325–1337.
- Gentric G, Maillet V, Paradis V, et al. Oxidative stress promotes pathologic polyploidization in nonalcoholic fatty liver disease. J Clin Invest. 2015;125(3):981–992.
- Wang X, Hung NJ, Costa RH. Earlier expression of the transcription factor HFH-11B diminishes induction of p21(CIP1/WAF1) levels and accelerates mouse hepatocyte entry into S-phase following carbon tetrachloride liver injury. Hepatology. 2001;33(6):1404–1414.
- Bangen JM, Hammerich L, Sonntag R, et al. Targeting CCl 4 -induced liver fibrosis by RNA interference-mediated inhibition of cyclin E1 in mice. Hepatology. 2017;66(4):1242–1257.
- Gonzales KAU, Fuchs E. Skin and its regenerative powers: an alliance between stem cells and their niche. Dev Cell. 2017;43(4):387–401.
- Michalopoulos GK. Principles of liver regeneration and growth homeostasis. Compr Physiol. 2013;3:485–513.
- Hardwick JM, Soane L. Multiple functions of BCL-2 family proteins. cold spring harbor perspectives in biology. 2013;5:a008722. DOI:10.1101/cshperspect.a008722.
- Walters J, Pop C, Scott FL, et al. A constitutively active and uninhibitable caspase-3 zymogen efficiently induces apoptosis. Biochem J. 2009;424(3):335–345.
- Essers J, Theil AF, Baldeyron C, et al. Nuclear dynamics of PCNA in DNA replication and repair. Mol Cell Biol. 2005;25(21):9350–9359.
- Cao S, Xiao L, Rao JN, et al. Inhibition of Smurf2 translation by miR-322/503 modulates TGF-β/Smad2 signaling and intestinal epithelial homeostasis. Mol Biol Cell. 2014;25(8):1234–1243.
- Gámez B, Rodríguez-Carballo E, Bartrons R, et al. MicroRNA-322 (miR-322) and its target protein Tob2 modulate osterix (Osx) mRNA stability. J Biol Chem. 2013;288(20):14264–14275.
- Merlet E, Atassi F, Motiani RK, et al. miR-424/322 regulates vascular smooth muscle cell phenotype and neointimal formation in the rat. Cardiovasc Res. 2013;98(3):458–468.
- Long Y, Wang L, Li Z. SP1-induced SNHG14 aggravates hypertrophic response in in vitro model of cardiac hypertrophy via up-regulation of PCDH17. J Cell Mol Med. 2020;24(13):7115–7126.
- Q W, Zhan J, Y L, et al. Differentiation-associated microRNA alterations in mouse heart-derived Sca-1(+)CD31(-) and Sca-1(+)CD31(+) cells. Stem Cells Int. 2016;2016:9586751.
- Nie P, Yang F, Wan F, et al. Analysis of microRNAs associated with carotid atherosclerotic plaque rupture with thrombosis. Front Genet. 2021;12:599350.
- J W, Yang B, Zhang Y, et al. miR-424-5p represses the metastasis and invasion of intrahepatic cholangiocarcinoma by targeting ARK5. Int J Biol Sci. 2019;15(8):1591–1599.
- Reppert SM, Weaver DR. Coordination of circadian timing in mammals. Nature. 2002;418(6901):935–941.
- Bukhari AB, Lewis CW, Pearce JJ, et al. Inhibiting Wee1 and ATR kinases produces tumor-selective synthetic lethality and suppresses metastasis. J Clin Invest. 2019;129(3):1329–1344.
- Davies KD, Cable PL, Garrus JE, et al. Chk1 inhibition and Wee1 inhibition combine synergistically to impede cellular proliferation. Cancer Biol Ther. 2011;12(9):788–796.
- Chen F, Huang D, Shi H, et al. Capn3 depletion causes Chk1 and Wee1 accumulation and disrupts synchronization of cell cycle reentry during liver regeneration after partial hepatectomy. Cell Regener. 2020;9(1):8. (London, England).