ABSTRACT
The study was designed to evaluate the efficiency of microwave ablation (MWA) in combination with anti-programmed death receptor 1 (anti-PD-1)/cytotoxic T lymphocyte-associated antigen 4 (CTLA-4) in renal cell carcinoma (RCC) treatment. After tumors were established on C57/BL6 mice, MWA treatment and/or immune checkpoint inhibitor (ICI) treatment to the mice were performed. Tumor volume was recorded every 7 days. A rechallenge test was conducted on mice with tumors in the left kidney to explore the systemic establishment of antitumor immunity on day 7. In this study, during the 21-day observation period, tumors were continued to grow in all groups. However, compared with the tumor growth rate in MWA or control group, the rate in the ICI or MWA+ICI groups was decreased. Moreover, the population of CD8+T-cells was increased only in the MWA+ICI group, while that of regulatory T cells was decreased in the MWA, ICI, and MWA+ICI groups. Additionally, the MWA+ICI group had the highest interferon-γ level among all groups. Furthermore, histopathological examination revealed that CTLA-4 expression in distant tumors was reduced in the ICI and MWA + ICI groups. MWA treatment increased PD-L1/PD-1 expression; however, after the combination treatment with ICI, PD-L1/PD-1 expression was decreased. According to the rechallenge test, mice (16.7%) in the MWA group, ICI group (50%), and MWA+ICI group (66.7%) exhibited successful tumor rejection, whereas no mice in the control group exhibited the capability of tumor rejection. Overall, the systemic antitumor immunity induced by MWA was boosted when combined with anti-PD-1/CTLA-4 treatment in an RCC murine model.
1. Introduction
Renal cell carcinoma (RCC), the third type of urologic cancer, accounts for 90% of all types of kidney cancer [Citation1,Citation2]. The percentage of patients with RCC who suffered synchronous metastases was up to 30%, and there also estimated 30% of patients who experienced recurrence after radical surgery [Citation3,Citation4]. Recently, targeted therapies have transformed the treatment landscape of RCC, and immune checkpoint inhibitors (ICIs) have become a new class of immunotherapy drugs that can improve the treatment of many types of cancer, such as metastatic RCC, non-small lung cancer and melanoma [Citation5–7]. Although systemic therapy is the first-line treatment for metastatic RCC, there still exist challenges in therapeutic choice [Citation8,Citation9]. Therefore, more efforts are required for achieving better overall survival and symptom control in RCC.
Thermal ablation has been frequently used to treat primary and metastatic cancers [Citation10–12], and indirect systemic effects such as antitumor immune responses have gained attention. Ablation can induce an abscopal effect, which is the spontaneous regression of a remote no-target tumor, and the abscopal effect may be dependent on the activation of the systemic antitumor immune response [Citation13]. After ablation, various immune-modulating factors are released or expressed. Moreover, increased release of tumor antigens induced by ablation is processed and presented by antigen-presenting cells, leading to the recognition and subsequent destruction of distant tumor cells [Citation13]. For example, lung metastasis was reported to be subsided after radio-frequency ablation in clinical practice [Citation14]. However, the effect of ablation alone has been proven to be either counterproductive or insufficient [Citation15,Citation16]. Recently, researchers have focused on the possible synergy between ablation and immunotherapy. They aimed to overcome issues related to negative immune regulation and systemically boost the long-term antitumor response. Microwave ablation (MWA) has several advantages over other ablation modalities, but MWA induces a weaker T-cell response than radiofrequency ablation and cryoablation [Citation17]. In addition, studies focused on the combination of MWA and immunotherapies are scarce.
ICIs have recently been extensively used in clinical practice [Citation18–20]. The programmed death receptor 1 (PD-1) and cytotoxic T lymphocyte-associated antigen 4 (CTLA-4) pathways are related to the ability of a tumor to evade the host immune system [Citation21,Citation22]. Specifically, the two most representative immune checkpoint pathways can modulate T cell immune function in a negative manner during different phases of T-cell activation [Citation21]. Inhibition of these targets contributes to the activation of the immune system [Citation22]. For example, upon CTLA-4 blockade, a broadening of circulating tumor-reactive CD8+T cell response was seen in melanoma [Citation23]. Blockade of PD-1 or PD-L1 results in rejection of ID8 tumors in most experimental mice as well as the increased proliferation of tumor antigen-specific effector CD8+T cells and the inhibition of myeloid-derived suppressor cells (MDSCs) and regulatory T cells (Tregs) in ovarian cancer [Citation24]. Combination therapy of anti-CTLA-4 and anti-PD-1 sufficiently induce increased frequency of T helper type 1 (Th1)-like CD4 effector T cells in malignant melanoma [Citation25]. Whether the PD-1 and CTLA-4 axes regulate MWA-induced antitumor T-cell immune responses in RCC remains obscure. Here, we aimed to investigate the changes of immune responses caused by MWA and ICI combination therapy in tumor-bearing mice to explore the clinical value of MWA combining with dual ICIs for metastatic RCC treatment.
2. Materials and methods
2.1. Cell line and cell culture
Murine renal cell carcinoma line (RENCA) was acquired from the American Type Culture Collection (Manassas, USA). The cells were cultured in Dulbecco’s modified Eagle’s medium (Thermo Fisher Scientific, Waltham, USA) with 10% fetal calf serum, 1% penicillin/streptomycin with nonessential amino acids, and 1% L-glutamine at 37°C and 5% CO2.
2.2. Animal care and tumor model establishment
The Institutional Animal Care Committee approved the experiments. A total of 48 C57/BL6 mice and 24 Rag1−/− mice were used for the study, and all mice were kept in the same environment. C57/BL6 mice (6–8 weeks) were obtained from the Institute of Laboratory Animals, Chinese Academy of Medical Science (Beijing, China). Immunodeficient C57/BL6J Rag1−/− mice were purchased from GemPharmatech Corporation (Nanjing, China). Mice were anesthetized using isoflurane inhalation. For the subcapsular implantation of RENCA cells, 1 × 105 cells were injected into the left kidney capsule. Approximately 7–10 days after inoculation, orthotopic tumor models were successfully established when the subcapsular tumors reached a diameter of 5–8 mm. For subcutaneous implantation of RENCA cells, 5 × 106 cells were injected to the right flank of these mice. When a mouse displayed symptoms and signs of pain and suffering or when the tumor in a mouse grew to 20 mm (the maximum diameter), the mouse was euthanized by CO2 inhalation.
2.3. Animal study design
Tumors were established on the left kidney and right flank of mice. First, before further treatment, tumors in mice were evaluated to determine baseline growth (). These mice were randomly assigned into four groups: control group (no treatment), MWA group (MWA of the left kidney tumor alone), ICI group (anti-PD-1/CTLA-4 treatment alone), and MWA+ICI group (MWA of the left kidney tumor in combination with anti-PD-1/CTLA-4 immunotherapy). Six mice were allocated to each group because the sample size is of statistical significance when n > = 6. People who allocate mice, conduct the experiments, and analyze the data are different. Evaluation of right flank tumors, flow cytometric analysis, and detection of serum interferon-γ (IFN-γ) levels were performed according to the scheme. Survival was observed within 21 days after MWA and/or ICI treatment.
Figure 1. Experimental design and schedule. (a) the assessment of tumor growth at each time point. (b) the arrangement of the rechallenge test. MWA: microwave ablation.
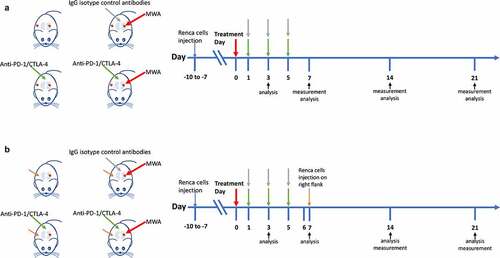
Next, we performed a tumor rechallenge test to determine the establishment of antitumor immunity (). The mice with tumor implantation in the left kidney were divided into four groups (n = 6/group): control, MWA, ICI, and MWA+ICI and were treated as described above. On day 7, the mice were injected with RENCA cells in their right flank. According to this scheme, reimplantation of the right flank tumor was evaluated, and flow cytometric analysis as well as the detection of serum IFN-γ levels were performed. Tumor-free survival was observed from re-implantation day (day 7) to the final day 21. After 21 days, all mice were sacrificed for histopathological analysis.
The same combination treatment was performed on immunodeficient Rag1−/− mice (n = 6/group).
2.4. MWA procedure
After anesthesia, tumor-bearing mice underwent MWA. A single 17-gauge water-cooled MWA antenna (Yigao Microwave Institute, Nanjing, China) with the active length of 1 cm was inserted into the tumor. A 5W output power was used through a microwave generator (ECO-100A, Yigao Microwave Institute) with 2450 MHz. Based on preliminary findings, MWA (5W, 3 min) can ablate 8–10 mm diameter tumors completely. Therefore, each mouse was subjected to MWA treatment under the same condition.
2.5. Antibody injection
For mice in the ICI and MWA+ICI groups, intraperitoneally injection of 2 mg/kg anti-PD-1 antibody (clone RMP1–14, BioXcell) and 10 mg/kg anti-CTLA-4 antibody (clone 9H10, BioXcell) suspended in 0.2 ml phosphate buffered saline (PBS) was performed on day 1, 3, and 5. For mice in the MWA group, intraperitoneally administration of 200 μg hamster IgG isotype control antibodies (BioXCell, West Lebanon, USA) in 0.2 ml PBS was performed.
2.6. Tumor evaluation
Tumors without any treatment were evaluated using Vernier calipers, and tumor volume was calculated using the following formula: (largest diameter × shortest diameter2)/2. The specific growth rate (SGR) was calculated according to the formula: (ln (Vend) – ln (VDay0))/T [Citation26]. Vend is the tumor volume at the end of observation (day 21) or before death, and VDay0 refers to the volume on the beginning day. T is the total days that a mouse survived. For the rechallenge test, tumor rejection was considered when the maximum diameter of a tumor was less than 5 mm at the end of the observation period.
2.7. Flow cytometry analysis
The populations of CD8+T-cells, myeloid-derived suppressor cells (MDSCs), and regulatory T cells (Tregs) were subjected to flow cytometry analyses. After washed in FACS buffer, the single-cell suspensions were incubated with Fc block (clone 2.4 G, CD16/32, BD Biosciences, Franklin Lakes, USA) followed by 25 min of staining at 4°C with the anti-mouse antibodies. Flow cytometry was performed using a FACSCalibur flow cytometer (BD Biosciences), and FlowJo software (TreeStar, Ashland, USA) was utilized for data analysis. Dead and adherent cells were excluded from the study. CD8+T-cells, MDSCs, and Tregs were gated as CD45+CD3+CD8+, CD11b+Gr1+, and CD4+CD25+Foxp3+, respectively.
2.8. Immunohistochemistry (IHC)
IHC were performed to detect the expression of CTLA-4, PD-1, and PD-L1 in tumor tissues. Tumor tissues were fixed, embedded, and then sectioned into 5 μm-thick slices. Tissue sections were deparaffinized with xylene and then rehydrated with different grades of ethanol. Antigen retrieval was performed using the provided solution (Vector Laboratories). Then, 0.2% Triton X-100 was applied to permeabilize the sections, and then the sections were blocked with serum followed by incubation with BLOXALL reagent (Vector Laboratories, USA) to quench endogenous peroxidase. Finally, the tissue sections were incubated with primary and secondary antibodies, as well as DAB substrate in succession. The positive cells on each slide were observed in 5 randomly selected visual fields and were analyzed by ImageJ software (National Institutes of Health, Bethesda, USA).
2.9. Detection of serum IFN-γ levels
Serum IFN-γ levels were detected using enzyme-linked immunosorbent assay (ELISA) kits (Abcam, UK). After mouse blood was put on ice for 30 min, centrifugation at 3600 RPM for 5 min was performed to aspirate the serum. Briefly, each well was added with 100 μL sample or standard reagent diluent (1:1). Next, an adhesive strip was used to cover the plate for 2 h incubation at room temperature (RT). Then, each well was fulfilled with 100 μL detection antibody (diluted in the reagent diluent), and the plate was covered with a new adhesive strip for another 2 h incubation. Afterward, the plate was supplemented with 100 μL streptavidin-HRP for 20 min incubation in the darkness. Subsequently, the plate was added with 100 μL substrate solution for 20 min of incubation followed by supplementation of 50 μL stop solution. At last, optical density was measured utilizing an automatic ELISA reader (Biobase, Nanjing, China) at the wavelength of 450 nm.
2.10. Statisticalanalysis
The Statistical Package for the Social Sciences version 26.0 (IBM, Armonk, USA) was used for data analysis. Data are expressed as the mean ± standard deviation or count (%). Student’s t-test or one-way ANOVA for parametric measures, followed by Bonferroni correction were applied for difference comparison between groups. A P value less than 0.05 was defined as statistically significant.
3. Results
3.1. MWA+ICI combination treatment suppresses tumor growth rate
For mice with tumors in the left kidney and right flank, the scheme of RENCA cell injection, MWA treatment, anti-PD-1/CTLA-4 treatment, and measurement analysis is shown in . A rechallenge test was conducted in mice with tumors in the left kidney, and the scheme of cell injection is provided in . Tumor volume was calculated every 7 days, and mean values of tumor volumes in different groups are provided in . The growth of untreated tumors is illustrated as in . To evaluate the antitumor impacts mediated by MWA, ICI, and MWA+ICI in RCC treatment, tumor growth in different groups were compared. Tumor volumes at initial treatment were 0.143 cm3, 0.133 cm3, 0.128 cm3, and 0.151 cm3 in the control, MWA, ICI, and MWA+ICI group, respectively (). Within the 21-day observation period, tumor volume was increased in all mice (). On day 21, 5 of 6 mice in control group and MWA group survived, while all 6 mice in ICI and MWA+ICI groups survived (). The specific growth rates (SGRs) were 0.180 ± 0.013, 0.159 ± 0.011, 0.150 ± 0.015, and 0.137 ± 0.027 in the control, MWA, ICI, and MWA + ICI groups, respectively (). The SGRs of the ICI and MWA+ICI groups were repressed (P = .0433 and P = .0027) compared with those of the control group. The findings revealed that MWA and ICI treatment significantly suppresses tumor growth.
Figure 2. MWA+ICI combination treatment obviously suppresses tumor growth rate. (a) the volume of untreated tumors within 21 days. (b) Specific growth rate (SGRs) of tumors in control, MWA, ICI, and MWA+ICI groups. *P<.05, **P<.01. MWA: microwave ablation; ICI: immune checkpoint inhibitor; SGR: specific growth rate.
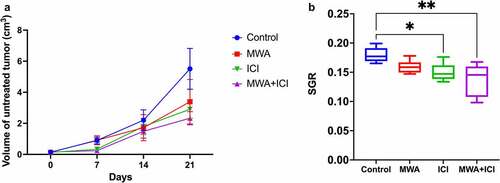
Table 1. Growth of untreated tumors.
3.2. MWA+ICI combination treatment increases CD8+T-cell percentage and decreasesTreg cell population
To better ascertain the synergistic antitumor immune responses induced by MWA, ICI, MWA+ICI, the T cell response in mice of each group was measured. CD8+T-cell levels were elevated only in the MWA+ICI group (vs. control PDay21 = .0021; vs. MWA PDay21 = .0233; and Supplementary-Figure S1). On days 7, 14, and 21, the percentage of Tregs was lowered in MWA, ICI, and MWA+ICI groups compared to those in control group ( and Supplementary-Figure S2). Myeloid-derived suppressor cell (MDSC) percentage among the groups showed no significant differences ( and Supplementary-Figure S3). The CD8+T/Treg ratio was obviously higher in the ICI group (vs. control PDay21 <.0001; vs. PDay21 = .0002) and the MWA+ICI group (vs. control PDay14 = .0032; vs. MWA PDay14 = .0114) than in control group and MWA group (). CD8+T-cells are key members of the adaptive immune response against cancer and infection [Citation27]. In addition, Tregs and MDSCs are important immunosuppressive cells in mouse tumors and in patients with cancer, and removing the two cell populations enhances antitumor immunity [Citation28]. Therefore, in the context of MWA and ICI combination treatment, the increase in CD8+T cell percentage and the reduction of Treg level suggested the enhancement of antitumor immunity caused by the MWA in combination with ICI. IFN-γ levels were further analyzed using ELISA (). On day 3, IFN-γ levels were obviously increased in MWA-treated (20.391 ng/ml, P < .0001), ICI-treated (20.174 ng/ml, P < .0001), and MWA+ICI-treated mice (20.029 ng/ml, P < .0001) compared to the levels in untreated mice (15.681 ng/ml). The MWA+ICI group showed the highest IFN-γ levels among the four groups on days 7 (35.971 ng/ml), 14 (30.193 ng/ml), and 21 (27.474 ng/ml). Since IFN-γ is essential for the activation of cellular immunity and subsequent antitumor immune response [Citation29], the decrease in serum IFN-γ level suggests that MWA and ICI treatment exerts effective antitumor immune response.
Figure 3. MWA+ICI combination treatment increases CD8+T-cell percentage and decreases Treg cell population. (a) CD8+T-cell percentage in control, MWA, ICI, and MWA+ICI groups. (b) Treg population by group. (c) MDSC population by group. (d) CD8+T/Treg ratio by group. (e) IFN-γ levels by group. *P<.05, **P<.01, ***P<.001, ****P<.0001.
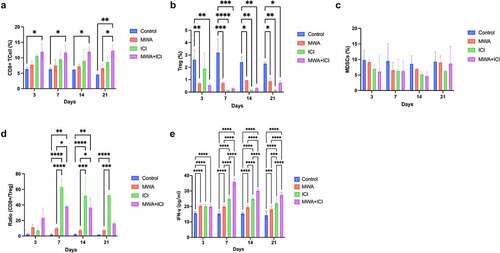
3.3. Expression of CTLA-4, PD-L1 and PD-1 in distant tumors
On day 21, we examined the expression of CTLA-4, PD-L1, and PD-1 in the tumor microenvironment. The activation of ICIs, including CTLA-4, PD-1, and PD-L1, can help cancer cells evade immune surveillance [Citation30]. CTLA-4 belongs to the CD28-B7 superfamily and regulates T-cell activation in a negative manner [Citation31]. As a co-inhibitory receptor on the T-cell surface, PD-1 maintains peripheral tolerance by inhibiting the activation of T-cells [Citation32]. The PD-1 receptor transmits inhibitory signals to T cells through binding to its ligands PD-L1 and PD-L2 [Citation33]. CTLA-4 expression in distant tumors was downregulated in ICI (P = .0285) and MWA+ICI groups (P = .0334) compared to that in control group (). Additionally, MWA treatment led to the upregulation of PD-L1 expression (vs. control, P = .0366) and that MWA combined with ICI treatment decreased PD-L1 expression (vs. control, P = .0232; vs. MWA, P = .0023; vs. ICI, P = .0294; ). Similarly, MWA increased PD-1 expression (vs. control, P = .0292); however, in MWA+ICI group, PD-1 expression was decreased (MWA+ICI vs. MWA, P = .0203; ). The above results implied that ICI levels are successfully reduced after anti-PD-1/CTLA-4 treatment, and the combining treatment of ICI and MWA further enhances the reduction of CTLA4, PD-L1, and PD-1 expression.
Figure 4. Detection of CTLA4, PD-L1 and PD-1 expression levels in distant tumors. (a) Mean density of CTLA4 expression in tumor tissues of control, MWA, ICI, and MWA+ICI groups. (b) Mean density of PD-L1 expression by group. (c) Mean density of PD-1 expression by group. *P<.05, **P<.01. MWA: microwave ablation; ICI: immune checkpoint inhibitor.
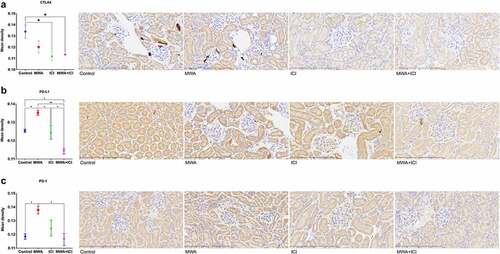
3.4. MWA+ICI combination treatment boosts tumor rejection upon rechallenge
To evaluate the establishment of antitumor immunity, a rechallenge test was performed. In the tumor rechallenge test, 1 mouse (16.7%) in the MWA group, 3 mice (50%) in the ICI group, and 4 mice (66.7%) in the MWA+ICI group exhibited successful tumor rejection, whereas none of mice in control group exhibited tumor rejection. The growth rates of the distant tumors are shown in . The SGRs were 0.286 ± 0.013, 0.179 ± 0.0882, 0.110 ± 0.036, and 0.062 ± 0.038 in control, MWA, ICI, and MWA+ICI groups, respectively. SGRs of the MWA, ICI, and MWA+ICI groups were significantly repressed (), and the SGR of the MWA group was higher than that of the MWA+ICI group (P = .0028). Hence, we concluded that combination of MWA and ICIs elicits tumor-specific immune responses.
Figure 5. Upon rechallenge test, MWA+ICI combination treatment boosts tumor rejection. (a) Volumes of distant tumors in control, MWA, ICI, and MWA+ICI groups. (b) SGRs by group. (c) CD8+T-cell percentage by group. (d) Treg cell population by group. (e) MDSC population by group. (f) CD8+T/Treg ratio by group. (g) IFN-γ levels by group. *P<.05, **P<.01, ***P<.001, ****P<.0001.
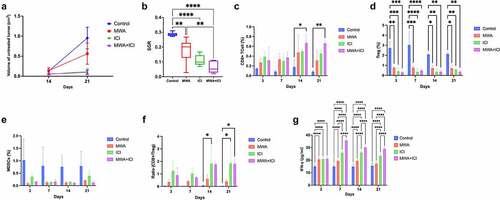
We also compared the levels of CD8+T-cells, Tregs, MDSCs, and CD8+T/Treg ratio among the groups. CD8+T-cell percentage was greatly elevated in the MWA+ICI group ( and Supplementary-Figure S4). During the 21-day observation period, the percentage of Tregs was reduced in the MWA, ICI, and MWA+ICI groups ( and Supplementary-Figure S5). Additionally, no significant differences were found in the MDSC levels among all groups ( and Supplementary-Figure S6). The CD8+T/Treg ratio was obviously increased in the ICI and MWA+ICI groups (). The MWA+ICI group showed the highest IFN-γ levels among the groups on days 7, 14, and 21 (). As evidenced by the above results, the T cell response is enhanced after MWA and ICIs treatment.
3.5. Immuno-deficient mice exhibits no tumor rejection after the combination therapy
To further verify the antitumor immune response of the combination therapy, immunodeficient Rag1−/− mice lacking both T cells and B cells were used to establish a tumor model. As shown by , the combination therapy failed to significantly reduce tumor volume and growth rates. Moreover, the population of CD8+T cells, Tregs, and MDSCs, as well as the ratio of CD8+T-cell/Treg were not significantly altered by combined MWA and ICI (). The findings revealed that the restriction of tumor growth mediated by the combination therapy call for an intact adaptive immune response, and T cells and/or B cells play essential roles in the process.
Figure 6. Immunodeficient mice exhibits no tumor rejection after the combination therapy. (a) Volumes of tumors in Rag1−/− mice of control, MWA, ICI, and MWA+ICI groups at the time point of day 0, day 7, day 14, and day 21. (b) SGRs of tumors in Rag1−/− mice from above four groups. (c) CD8+T-cell percentage by group. (d) Treg cell population by group. (e) MDSC population by group. (f) CD8+T/Treg ratio by group. (g) IFN-γ levels by group.
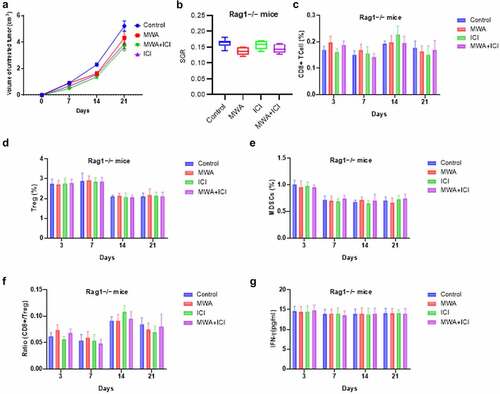
4. Discussion
This study revealed that combining MWA with anti-PD-1/CTLA-4 therapy increased CD8+T-cell percentage, decreased Treg cell population, and inhibited the growth of distant tumors. Furthermore, animals treated with the combined modalities showed potential tumor rejection upon rechallenge.
MWA destroys cancer cells via hyperthermic injury, and this action results in the disruption of cell membranes, protein denaturation, and coagulative necrosis with cellular death [Citation34,Citation35]. While interleukin-1 and heat shock proteins are all increased after MWA, the magnitude of their induction is less than that after radiofrequency ablation and cryoablation [Citation36]. Perhaps because of its relatively poor immune stimulation, the combination of MWA and immunotherapy has not been explored as extensively as other ablative technologies [Citation37]. A detailed understanding of the immune-mediated effects of MWA is essential to determine the role of this treatment in the context of emerging ICI therapy [Citation38]. Clinical trials to validate the efficacy of PD-1 or CTLA-4 inhibitors have demonstrated a potential option for the treatment of a variety of cancers [Citation20,Citation25,Citation39]. However, many patients still experience disease recurrence after immunotherapy. One explanation is that cancer stem cells are largely responsible for cancer relapse.
Therefore, it was hypothesized that combining MWA with dual ICIs could synergistically affect the immune response. MWA activates antitumor immunity by generating tumor antigens and danger signals. The sublethal zone around necrosis area also generates inflammatory cytokines [Citation40]. This study showed that combining MWA and ICIs boosted antitumor immunity. The combination therapy increased IFN-γ production compared to the use of monotherapy and decreased the SGR of untreated tumors compared to that of the controls. After rechallenge test, 66.7% of the mice treated with the combination therapy did not exhibit secondary tumors, whereas all the control mice exhibited secondary tumors, and only 16.7% of the mice treated with MWA alone exhibited secondary tumors. The combination therapy also obviously increased CD8+T-cells and decreased Treg cells, consistent with previous research [Citation41], while the combination therapy did not obviously decrease MDSC levels. Levy et al. demonstrated that cryoablation combined with cyclophosphamide caused systemic antitumor immunity in animal models with metastases, and the deficiency of CD8+T-cells obviously attenuated the adoptive antitumor immune response [Citation42].
As to the underlying mechanism, immunogenic cell death (ICD) might be the explanation for the antitumor impacts. ICD is a type of regulated cancer cell death that can be induced by radiotherapy [Citation43], which can activate adaptive immunity and thus form long-term immunological memory [Citation44]. The definition of ICD is based on the communication between dying and immune cells, which refers to a successful dialog between a dying cell and an appropriately disposed immune system [Citation45]. For example, Nizar Hijaze et al. induces the ICD of mouse renal cell carcinoma cells and colon carcinoma cells using anti-161-pAb and thus shift the mode of macrophage activation by inducing necrosis of tumor cells [Citation46]. Specifically, damage‐associated molecular patterns (DAMPs) are released from dying tumor cells, leading to the activation of tumor‐specific immune responses, and thereby induce long-term efficacy of anticancer drugs through the combination of direct cancer cell killing and antitumor immunity [Citation47]. Iuliia Efimova et al. found that the release of ATP, one of critical DAMPs, was observed temporally followed the cell death process [Citation48]. ICD-associated DAMPs can reactivate anticancer immunity by triggering the maturation of dendritic cells, and then these dendritic cells prime naïve CD8+T-cells [Citation44]. As evidenced by a previous study, modified vaccinia virus Ankara leads to the autophagy and death of murine bone marrow-derived dendritic cells, which promotes CD8+T-cell proliferation [Citation49]. Moreover, MWA was also reported to cause ICD in osteosarcoma and elevates CD8+T-cell percentage [Citation34]. Additionally, depletion of CD8+T cells reversed the antitumor impacts exerted by MWA, indicating the key role of CD8+T-cells in reducing osteosarcoma cells [Citation34]. Moreover, CTLA4 and PD-1 are important checkpoint pathways that can attenuate T-cell activation, and the blockade of the two pathways contributes to T-cell reinvigoration and tumor rejection [Citation50,Citation51]. When combining MWA and anti-CTLA4/PD-1 therapy, the increase in CD8+T cell population might be benefit for the elimination of tumor cells that have not been ablated and thus protect the host from tumor recurrence or the invasion of new tumor cells [Citation34].
Moreover, the expression of PD-L1 and PD-1 was found to be increased after MWA treatment and decreased after combination treatment with ICIs, which indicated the important role of PD-L1/PD-1 axis in suppressing MWA-induced antitumor immunity. However, PD-1 inhibitors show limited benefit for the majority of patients with low or no PD-L1 expression [Citation52], which indicates that the efficacy of PD-1 inhibitors is intimately related to preexisting antitumor immune responses [Citation53]. In preclinical studies, radiotherapy combined with PD-L1/PD-1 inhibitors boosted antitumor immunity synergistically, indicating that local antitumor therapies that can induce immune responses may provide opportunities for PD-1/PD-L1 inhibitors [Citation54]. Tartarone et al. found that PD-1 inhibitors are slightly better than PD-L1 inhibitors [Citation55]. As hypothesized by Passiglia et al., the major effects observed with PD-1 inhibitors could be due to their ability to synchronously interfere the binding capacity of PD-1 and PD-L1/PD-L2, while PD-L1 inhibitors may not affect PD-1 and PD-L2 interaction [Citation56].
CTLA-4 was revealed to suppress ablation-caused antitumor immunity [Citation57]. In our study, CTLA-4 expression did not change significantly after MWA but obviously decreased after ICI therapy and MWA+ICI treatment. Victor et al. revealed that combining treatment of radiotherapy and a CTLA-4 inhibitor boosted antitumor immunity but failed to prevent the exhaustion of T-cells in a mouse model of melanoma [Citation54]. According to Sobhani et al., CTLA-4 inhibitors do not change the level of CTLA-4 in Tregs and suggest that they could be more effective in eradicating tumor cells, as CTLA-4 inhibitors do not lead to the degradation of CTLA-4 and therefore do not interfere with Treg function in preventing autoimmunity [Citation58]. Similar to our dual ICI approach, PD-L1 inhibition reversed T cell exhaustion while promoting T cell expansion, mitigating CD8+T/Treg ratio depression, and promoting an optimal response in the animal model.
There are several advantages to combining MWA and dual ICI therapy. First, thermal ablation is recommended for patients with poor physical condition, bilateral tumors, and those with one kidney [Citation59]; thus, it is feasible to explore the role of MWA in combined therapy. Second, MWA leads to the instant release of large levels of tumor antigens in the milieu of “danger” signals. Third, anti-PD-1 and anti-CTLA-4 treatments have shown promising results in metastatic renal cell carcinoma, demonstrating the lowest toxicity among first-line treatments [Citation60]. Nevertheless, this study has the following limitations. First, because of the differences between mice and human beings, more experiments need to be conducted for further application. Second, we did not compare MWA to other thermal ablation techniques. Additionally, the PD-1/CTLA-4 antibodies were hamster anti-mouse PD-1/CTLA-4 antibodies, and thus immune reactions against hamster-derived antibodies may cause antibody deactivation. Finally, the overall incidence of immune-related adverse events (irAEs) with dual ICIs might increase when compared with monotherapy, and thereby further studies are warranted.
5. Conclusions
Systemic antitumor immunity could be boosted in a murine model by administering anti-PD-1/CTLA-4 therapy in combination with MWA. Mice that received combination treatment demonstrated suppressed tumor growth and rejection. The combination strategy may be a promising clinical option to boost systemic antitumor immunity in patients with metastatic RCC.
Author contributions
R-Q.G. contributed to the conception and design of the study, the analysis and interpretation of the data, performed the experimental study, and drafted the article. J-Z.P. performed the experimental study and drafted the manuscript. Y-M.L. contributed to data analysis and interpretation. X-G.L. contributed to the conception and design of the study, and supervision. All authors have read and approved the final version of the manuscript.
Ethic approval
All procedures performed in studies involving animals were in accordance with the ethical standards of the institution or practice at which the studies were conducted.
Supplemental Material
Download Zip (13.4 MB)Disclosure statement
No potential conflict of interest was reported by the author(s).
Supplementary material
Supplemental data for this article can be accessed online at https://doi.org/10.1080/15384101.2022.2112007.
Additional information
Funding
References
- Deleuze A, Saout J, Dugay F, et al. Immunotherapy in renal cell carcinoma: the future is now. Int J Mol Sci. 2020 Apr 5;21(7):2532.
- Gray RE, Harris GT Renal cell carcinoma: diagnosis and management. Am Fam Physician. 2019 Feb 1;99(3):179–184.
- Sung H, Ferlay J, Siegel RL, et al. Global cancer statistics 2020: globocan estimates of incidence and mortality worldwide for 36 cancers in 185 countries. CA Cancer J Clin. 2021 May;71(3):209–249.
- Siegel RL, Miller KD, Fuchs HE, et al. Cancer statistics, 2021. CA Cancer J Clin. 2021 Jan;71(1):7–33.
- Kahlmeyer A, Stöhr CG, Hartmann A, et al. Expression of PD-1 and CTLA-4 are negative prognostic markers in renal cell carcinoma. J Clin Med. 2019 May 24;8(5):743.
- Schoenfeld JD, Giobbie-Hurder A, Ranasinghe S, et al. Durvalumab plus tremelimumab alone or in combination with low-dose or hypofractionated radiotherapy in metastatic non-small-cell lung cancer refractory to previous PD(L)-1 therapy: an open-label, multicentre, randomised, phase 2 trial. Lancet Oncol. 2022 Feb;23(2):279–291. 10.1016/S1470-2045(21)00658-6
- Zhou J, Mahoney KM, Giobbie-Hurder A, et al. Soluble PD-L1 as a biomarker in malignant melanoma treated with checkpoint blockade. Cancer Immunol Res. 2017 Jun;5(6):480–492.
- Choueiri TK, Motzer RJ Systemic therapy for metastatic renal-cell carcinoma. N Engl J Med. 2017 Jan 26;376(4):354–366.
- Bex A, Mulders P, Jewett M, et al. Comparison of immediate vs deferred cytoreductive nephrectomy in patients with synchronous metastatic renal cell carcinoma receiving sunitinib: the SURTIME randomized clinical trial. JAMA Oncol. 2019 Feb 1;5(2):164–170.
- Löffler MW, Nussbaum B, Jäger G, et al. A non-interventional clinical trial assessing immune responses after radiofrequency ablation of liver metastases from colorectal cancer. Front Immunol. 2019;10:2526.
- Acksteiner C, Steinke K Percutaneous microwave ablation for early-stage non-small cell lung cancer (NSCLC) in the elderly: a promising outlook. J Med Imaging Radiat Oncol. 2015 Feb;59(1):82–90.
- Matsui Y, Hiraki T, Gobara H, et al. Percutaneous thermal ablation for renal cell carcinoma in patients with birt-hogg-dubé syndrome. Diagn Interv Imaging. 2019 Nov;100(11):671–677.
- Takaki H, Cornelis F, Kako Y, et al. Thermal ablation and immunomodulation: from preclinical experiments to clinical trials. Diagn Interv Imaging. 2017 Sep;98(9):651–659.
- Rao P, Escudier B, de Baere T Spontaneous regression of multiple pulmonary metastases after radiofrequency ablation of a single metastasis. Cardiovasc Intervent Radiol. 2011 Apr;34(2):424–430.
- Su T, Huang M, Liao J, et al. Insufficient radiofrequency ablation promotes hepatocellular carcinoma metastasis through N6-methyladenosine mRNA methylation-dependent mechanism. Hepatology. 2021 Sep;74(3):1339–1356. 10.1002/hep.31766
- Yan P, Lyu X, Wang S, et al. Insufficient ablation promotes the metastasis of residual non-small cell lung cancer (NSCLC) cells via upregulating carboxypeptidase A4. Int J Hyperthermia. 2021;38(1):1037–1051.
- Ahmad F, Gravante G, Bhardwaj N, et al. Changes in interleukin-1beta and 6 after hepatic microwave tissue ablation compared with radiofrequency, cryotherapy and surgical resections. Am J Surg. 2010 Oct;200(4):500–506.
- Dolladille C, Ederhy S, Sassier M, et al. Immune checkpoint inhibitor rechallenge after immune-related adverse events in patients with cancer. JAMA Oncol. 2020 Jun 1;6(6):865–871.
- Wang H, Guo X, Zhou J, et al. Clinical diagnosis and treatment of immune checkpoint inhibitor-associated pneumonitis. Thorac Cancer. 2020 Jan;11(1):191–197.
- Amaria RN, Reddy SM, Tawbi HA, et al. Neoadjuvant immune checkpoint blockade in high-risk resectable melanoma. Nat Med. 2018 Nov;24(11):1649–1654.
- Zhang H, Dai Z, Wu W, et al. Regulatory mechanisms of immune checkpoints PD-L1 and CTLA-4 in cancer. J Exp Clin Cancer Res. 2021 Jun 4;40(1):184.
- Buchbinder EI, Desai A CTLA-4 and PD-1 pathways: similarities, differences, and implications of their inhibition. Am J Clin Oncol. 2016 Feb;39(1):98–106.
- Gangaev A, Rozeman EA, Rohaan MW, et al. Differential effects of PD-1 and CTLA-4 blockade on the melanoma-reactive CD8 T cell response. Proc Natl Acad Sci U S a. 2021 Oct 26;118(43):e2102849118.
- Duraiswamy J, Freeman GJ, Coukos G Therapeutic PD-1 pathway blockade augments with other modalities of immunotherapy T-cell function to prevent immune decline in ovarian cancer. Cancer Res. 2013 Dec 1;73(23):6900–6912.
- Wei SC, Anang NAS, Sharma R, et al. Combination anti-CTLA-4 plus anti-PD-1 checkpoint blockade utilizes cellular mechanisms partially distinct from monotherapies. Proc Natl Acad Sci U S a. 2019 Nov 5;116(45):22699–22709.
- Hamamoto S, Okuma T, Yamamoto A, et al. Radiofrequency ablation and immunostimulant OK-432: combination therapy enhances systemic antitumor immunity for treatment of VX2 lung tumors in rabbits. Radiology. 2013 May;267(2):405–413.
- Raud B, McGuire PJ, Jones RG, et al. Fatty acid metabolism in CD8(+) T cell memory: challenging current concepts. Immunol Rev. 2018 May;283(1):213–231.
- Tanaka A, Sakaguchi S Regulatory T cells in cancer immunotherapy. Cell Res. 2017 Jan;27(1):109–118.
- Jorgovanovic D, Song M, Wang L, et al. Roles of IFN-γ in tumor progression and regression: a review. Biomark Res. 2020;8:49.
- Lee HT, Lee SH, Heo YS Molecular interactions of antibody drugs targeting PD-1, PD-L1, and CTLA-4 in immuno-oncology. Molecules. 2019 Mar 26;24(6):1190.
- Wei SC, Levine JH, Cogdill AP, et al. Distinct cellular mechanisms underlie anti-CTLA-4 and anti-PD-1 checkpoint blockade. Cell. 2017 Sep 7;170(6):1120–1133.e17.
- Okazaki T, Iwai Y, Honjo T New regulatory co-receptors: Inducible co-stimulator and PD-1. Curr Opin Immunol. 2002 Dec;14(6):779–782.
- Okazaki T, Honjo T PD-1 and PD-1 ligands: from discovery to clinical application. Int Immunol. 2007 Jul;19(7):813–824.
- Yu Z, Geng J, Zhang M, et al. Treatment of osteosarcoma with microwave thermal ablation to induce immunogenic cell death. Oncotarget. 2014 Aug 15;5(15):6526–6539.
- Yu J, Liang P, Yu XL, et al. US-Guided percutaneous microwave ablation versus open radical nephrectomy for small renal cell carcinoma: intermediate-term results. Radiology. 2014 Mar;270(3):880–887.
- Ahmad F, Gravante G, Bhardwaj N, et al. Renal effects of microwave ablation compared with radiofrequency, cryotherapy and surgical resection at different volumes of the liver treated. Liver Int. 2010 Oct;30(9):1305–1314.
- Takahashi H, Berber E Role of thermal ablation in the management of colorectal liver metastasis. Hepatobiliary Surg Nutr. 2020 Feb;9(1):49–58.
- Flynn MJ, Sayed AA, Sharma R, et al. Challenges and opportunities in the clinical development of immune checkpoint inhibitors for hepatocellular carcinoma. Hepatology. 2019 May;69(5):2258–2270.
- Delaunay M, Cadranel J, Lusque A, et al. Immune-Checkpoint inhibitors associated with interstitial lung disease in cancer patients. Eur Respir J. 2017 Aug;50(2):1700050.
- Slovak R, Ludwig JM, Gettinger SN, et al. Immuno-Thermal ablations - boosting the anticancer immune response. J Immunother Cancer. 2017 Oct 17;5(1):78.
- den Brok MHMGM, Sutmuller RPM, Nierkens S, et al. Efficient loading of dendritic cells following cryo and radiofrequency ablation in combination with immune modulation induces anti-tumour immunity. Br J Cancer. 2006 Oct 9;95(7):896–905.
- Levy MY, Sidana A, Chowdhury WH, et al. Cyclophosphamide unmasks an antimetastatic effect of local tumor cryoablation. J Pharmacol Exp Ther. 2009 Aug;330(2):596–601.
- Kong Y, Zhao X, Xu M, et al. PD-1 inhibitor combined with radiotherapy and GM-CSF (PRaG) in patients with metastatic solid tumors: an open-label phase II study. Front Immunol. 2022;13:952066.
- Lin RA, Lin JK, Lin SY. Mechanisms of immunogenic cell death and immune checkpoint blockade therapy. Kaohsiung J Med Sci. 2021 Jun;37(6):448–458.
- Legrand AJ, Konstantinou M, Goode EF, et al. The diversification of cell death and immunity: memento mori. Mol Cell. 2019 Oct 17;76(2):232–242.
- Hijaze N, Ledersnaider M, Simanovich E, et al. Inducing regulated necrosis and shifting macrophage polarization with anti-EMMPRIN antibody (161-pAb) and complement factors. J Leukoc Biol. 2021 Aug;110(2):343–356.
- Ahmed A, Tait SWG. Targeting immunogenic cell death in cancer. Mol Oncol. 2020 Dec;14(12):2994–3006.
- Efimova I, Catanzaro E, Van der Meeren L, et al. Vaccination with early ferroptotic cancer cells induces efficient antitumor immunity. J Immunother Cancer. 2020 Nov;8(2):e001369.
- Tappe KA, Budida R, Stankov MV, et al. Immunogenic cell death of dendritic cells following modified vaccinia virus ankara infection enhances CD8(+) T cell proliferation. Eur J Immunol. 2018 Dec;48(12):2042–2054.
- O’Malley DM, Neffa M, Monk BJ, et al. Dual PD-1 and CTLA-4 checkpoint blockade using balstilimab and zalifrelimab combination as second-line treatment for advanced cervical cancer: an open-label phase II study. J Clin Oncol. 2022 Mar 1;40(7):762–771.
- Curran MA, Montalvo W, Yagita H, et al. PD-1 and CTLA-4 combination blockade expands infiltrating T cells and reduces regulatory T and myeloid cells within B16 melanoma tumors. Proc Natl Acad Sci U S a. 2010 Mar 2;107(9):4275–4280.
- Topalian SL, Hodi FS, Brahmer JR, et al. Safety, activity, and immune correlates of anti-PD-1 antibody in cancer. N Engl J Med. 2012 Jun 28;366(26):2443–2454.
- Taube JM, Klein A, Brahmer JR, et al. Association of PD-1, PD-1 ligands, and other features of the tumor immune microenvironment with response to anti-PD-1 therapy. Clin Cancer Res. 2014 Oct 1;20(19):5064–5074.
- Twyman-Saint Victor C, Rech AJ, Maity A, et al. Radiation and dual checkpoint blockade activate non-redundant immune mechanisms in cancer. Nature. 2015 Apr 16;520(7547):373–377.
- Tartarone A, Roviello G, Lerose R, et al. Anti-PD-1 versus anti-PD-L1 therapy in patients with pretreated advanced non-small-cell lung cancer: a meta-analysis. Future Oncol. 2019 Jul;15(20):2423–2433.
- Passiglia F, Galvano A, Rizzo S, et al. Looking for the best immune-checkpoint inhibitor in pre-treated NSCLC patients: an indirect comparison between nivolumab, pembrolizumab and atezolizumab. Int J Cancer. 2018 Mar 15;142(6):1277–1284.
- Zhang L, Xu L, Wang Y, et al. Antitumor immunity augmented by combining radiofrequency ablation with anti-CTLA-4 therapy in a subcutaneous murine hepatoma model. J Vasc Interv Radiol. 2020 Jul;31(7):1178–1186.
- Sobhani N, Tardiel-Cyril DR, Davtyan A, et al. CTLA-4 in regulatory T cells for cancer immunotherapy. Cancers (Basel). 2021 Mar 22;13(6):1440.
- NCCN clinical practice guidelines in oncology (NCCN Guidelines®) kidney cancer, version 1. 2022- July 1, 2021: National Comprehensive Cancer Network; 2021 [cited 2021 July 11]. Available from: https://www.nccn.org/professionals/physician_gls/pdf/kidney.pdf
- Nocera L, Karakiewicz PI, Wenzel M, et al. Clinical outcomes and adverse events after first-line treatment in metastatic renal cell carcinoma: a systematic review and network meta-analysis. J Urol. 2021 Sep 21; 207(1):16–24.